- 1Soil Ecology Lab, College of Resources and Environmental Sciences, Nanjing Agricultural University, Nanjing, China
- 2Jiangsu Collaborative Innovation Center for Solid Organic Waste Resource Utilization, Nanjing, China
- 3Sanya Institute of Nanjing Agricultural University, Sanya, China
Applications of the key plant nutrient nitrogen (N) increase the uptake and accumulation of pollutants such as polycyclic aromatic hydrocarbons (PAHs). However, it is unclear how a plant’s preference for a particular form of N in the soil affects the uptake and accumulation of PAHs. In this study, we investigated the physiological mechanisms involved in fluoranthene uptake by rice (Oryza sativa L.) and wheat (Triticum aestivum L.) and examined how these mechanisms were affected by different forms of N treatment under an equivalent N supply. Both N form and plant species affected plant fluoranthene uptake. Rice accumulated more fluoranthene than wheat under an equivalent N supply, while the transfer coefficient of fluoranthene in wheat was higher than that in rice. Fluoranthene accumulation in rice and wheat was positively correlated with plant root morphology parameters, and the transfer coefficient was positively correlated with transpiration. Of the treatments examined, ammonium (NH4+-N)-treated rice and nitrate (NO3−-N)-treated wheat accumulated the most fluoranthene at equivalent N supply. Fluoranthene accumulation was positively correlated with plant growth, total nitrogen N content, total protein content, and antioxidant enzyme activities. Based on a partial least squares path model (PLS-PM) analysis, total plant N was the main factor influencing fluoranthene uptake by rice and wheat treated with different forms of N. Overall, ammonium-preferring rice and nitrate-preferring wheat had the highest nutrient content in their preferred N forms, which also promoted fluoranthene uptake. Therefore, regulating the form of N applied to the soil could be a suitable strategy to improve the safety of agricultural products.
Introduction
Polycyclic aromatic hydrocarbons (PAHs) such as fluoranthene, phenanthrene, and pyrene, are organic pollutants with strong toxic, mutagenic, and carcinogenic properties. These pollutants are ubiquitous in agroecosystems worldwide (Tian et al., 2018; Wang et al., 2021). PAHs can be absorbed by crops grown in PAH-contaminated soils. Thus, food chain contamination is an important entry route into the human body for these contaminants (Zhan et al., 2013; Liao et al., 2018). Rice (Oryza sativa L.) and wheat (Triticum aestivum L.) cultivated in China represent 29% and 18% of global rice and wheat yields, respectively (Aslam et al., 2021). Rice and wheat are important food crops in China, together accounting for 51.6% of China’s total grain yield (Zhang et al., 2022). Dietary intake accounts for 88–98% of PAH exposure in humans worldwide (Singh & Agarwal, 2018). Therefore, deciphering the physiological mechanisms underlying PAH accumulation in rice and wheat and developing strategies to minimize absorption are important to improve the safety of agricultural products.
Nitrogen (N) is an essential macronutrient for protein biosynthesis and is also a component of various structural, metabolic, and genetic molecules. N plays crucial roles in plant growth and development and even influences pollutant accumulation in plants (Cheng et al., 2018; Yang et al., 2020; Mussarat et al., 2021). Nitrate-N (NO3−-N) and ammonium-N (NH4+-N) are the two major forms of N absorbed and assimilated by plants (Yi et al., 2019). N application increases heavy metal accumulation in plants (Hassan et al., 2005; Cheng et al., 2020), and the accumulation of heavy metals in plants varies depending on the form of N fertilizer applied. Compared with NH4+-N, NO3−-N increased Cd uptake and accumulation in plants such as tomato (Solanum lycopersicum) (Luo et al., 2012), potato (Solanum tuberosum) (Jönsson and Asp, 2013), and Arabidopsis thaliana (Guan et al., 2015). Conversely, NH4+-N enhances Cd uptake and accumulation in Carpobrotus rossi (Cheng et al., 2016) and Kandelia obovata (Chai et al., 2018). However, the accumulation efficiency of heavy metals by the same plant also varied under different N forms. A previous study reported that rice supplied with NO3−-N increased Cd uptake in grain (Jalloh et al., 2009), whereas another study reported that NH4+-N application increased Cd uptake in rice (Wu et al., 2018). These inconsistent findings may be partly due to differences in cultivation type, N application rate and timing, and the particular growth conditions used in each study (Cheng et al., 2017). Furthermore, the form of nitrogen present in the soil affects the physicochemical properties of plants and further influences heavy metal accumulation. In rice, application of NH4+-N promotes the uptake and accumulation of Cd by enhancing antioxidant enzyme activity (Hassan et al., 2008). Another study found that NO3−-N application, which significantly enhanced the total nitrogen (TN) content of dwarf polish wheat (Triticum polonicum L.), promoted the uptake and accumulation of Cd (Cheng et al., 2018).
In addition to influencing heavy metal accumulation, N addition to the soil increases PAH uptake by plants (Thompson et al., 2008; Chen et al., 2015). Different N forms also have different effects on PAH uptake. For instance, NH4+-N application promoted PAH uptake in alfalfa (Medicago sativa L.) (Li et al., 2022), whereas NH4+ addition increased PAH uptake in wheat and lettuce (Lactuca sativa L.) (Zhan et al., 2015). This increase in PAH uptake is due to the proton efflux induced by ammonium uptake, which enhances the symport of H+ and PAH (Yin et al., 2015). Wang et al. (2021) established that overexpression of the nitrate (NO3−) transporter gene OsNRT2.3b in rice increased the TN content of the plant and decreased phenanthrene uptake. Moreover, the effect of ammonium and nitrate treatment on PAH uptake differed even in the same plant. Yang et al. (2012) showed that NH4+-N promoted phenanthrene uptake mainly by enhancing antioxidant enzyme activity and total plant nitrogen, while NO3−-N promoted phenanthrene uptake mainly by enhancing the biosynthesis of soluble proteins.
The plant preference for nutrients is reflected in the promotion of plant growth, such as plant height and dry weight. Moreover, while rice generally prefers NH4+-N (Zhang et al., 2018), especially in the early growth stages (Wu et al., 2018), wheat prefers NO3− (Zhao & Shen, 2018). Thus, plant nutrient preferences could affect the uptake and accumulation of pollutants.
Fluoranthene is a dominant PAH in the Yangtze River Delta region of China, being present in 73.3% of soil samples from this region (Cai et al., 2008). Indeed, more than 20% of major crop plants in China exceed the relative control limit for PAHs (Zhan et al., 2015); PAH uptake in plants was influenced by the form of N fertilizer applied (Yang et al., 2012; Zhan et al., 2015). In this study, we sought to 1) examine the uptake characteristics of fluoranthene in rice and wheat under three different N forms (NH4+-N, NO3−-N, and NH4+-N + NO3−-N), 2) evaluate the response characteristics of plants under different N forms, and 3) reveal the relationship between PAH uptake and plant response characteristics. Plant preference for nutrients affects the PAH uptake. These results could provide a theoretical basis for PAH safety control in crops by nitrogen application management.
Materials and methods
Plant material and growth conditions
Rice (Oryza sativa L.) and wheat (Triticum aestivum L.) seeds were surface sterilized with 5% (v/v) NaClO for 10 min, soaked in deionized water for 24 h, and then germinated on wet filter paper in the dark at 20°C for 4 days. Seedlings were cultivated in modified full-strength Hoagland’s nutrient solution (4 mM Ca (NO3)2·4H2O, 6 mM KNO3, 1 mM NH4H2PO4, 2 mM MgSO4·7H2O, 10 μM H3BO3, 1.8 μM MnSO4, 0.2 μM NaMoO4, 0.31 μM CuSO4, 5 μM ZnSO4, and 50 μM Fe-EDDHA [pH, 5.5]). The rice and wheat seedlings were grown in a climate chamber under controlled conditions (photoperiod 16 h light/8 h dark; light intensity 400 μmol m−2 s−1; day/night temperature of 25/20°C; relative humidity 60%). After a 30-days growth in Hoagland nutrient solution, the rice and wheat were used in the subsequent experiments.
To investigate the effects of different N forms on PAH uptake, 3 N treatments were established: NH4+-N (1 mM), NO3−-N (14 mM), and NH4+-N (1 mM) + NO3−-N (14 mM). The amount of NO3− and NH4+ used in the treatments was selected so that the TN concentration was the same as that of full-strength Hoagland’s nutrient solution (2.86 mmol L−1). To maintain the balance between calcium (Ca) and potassium (K), 4 mM Ca(NO3)2·4H2O and 6 mM KNO3 were replaced with 4 mM CaCl2 and 6 mM KCl, respectively, for the NH4+-N treatment; for the NO3−-N treatment, 1 mM KCl and 1 mM NH4H2PO4 were replaced with 1 mM KH2PO4; for the NH4+-N + NO3−-N treatment, 1 mM KCl was added. The composition of other nutrients in the modified Hoagland’s solution was the same as that of the basal nutrient solution. The final concentration of fluoranthene in the nutrient solution was 10 mg/L with methanol as a solvent. The methanol concentration was less than 0.1% and did not impact root growth (Chapin et al., 1993). A nitrification inhibitor (dicyandiamide, 7 μmol/L) was added to each nutrient solution to prevent ammonium oxidation. The pH of the nutrient solutions was adjusted to 5.5 each day with 0.1 mol/L HCl and 0.1 mol/L NaOH. Four biological replicates were prepared for further analyses.
Measurement of plant growth and root morphology parameters
Fresh roots were scanned using an Epson Expression 11000XL scanner (Epson Seiko Corporation, Nagano, Japan). Total root length, surface area, and root tips were further analyzed using a WinRHIZO instrument (Regent Instruments Canada Inc., Quebec, Canada). Dry weights (DWs) of roots and shoots were measured after deactivation at 105°C for 0.5 h and drying to a constant weight at 80°C.
Determination of photosynthetic and transpiration parameters
The photosynthetic rate (Pn) and transpiration rate (Tr) of upper fully expanded leaves were measured using a portable photosynthesis system (LI-6400; LICOR, Lincoln, NE, United States) from 9:00 a.m. to 11:00 a.m. Measurements were always performed at a constant flow rate of 500 μmol s−1, a PFD of 500 μmol m−2 s−1, an ambient CO2 concentration of approximately 350 μmol mol−1, and a temperature of about 25°C.
Enzyme activity and malondialdehyde content assay
Superoxide dismutase activity (SOD) was measured using the photochemical method, as described previously by Beauchamp & Fridovich (1971). One unit of SOD activity was defined as the amount of enzyme required to inhibit the reduction of nitro blue tetrazolium (NBT) by 50% at 560 nm in the presence of riboflavin and light.
Peroxidase (POD) activity was determined by monitoring the increase in absorbance at 470 nm due to guaiacol oxidation (1 EU = 1 μmol guaiacol oxidized in 1 min) (Upadhyaya et al., 1985).
Catalase (CAT) activity was assayed (Upadhyaya et al., 1985) by monitoring the decomposition of H2O2 at 240 nm (1EU = 1 μmol H2O2 decomposed in 1 min).
MDA content was quantified using the thiobarbituric acid method (Wills. 1987) and expressed as nmol thiobarbituric acid-reactive substance (TBARS)/mg protein.
Total protein and total nitrogen contents
The weight digestion method was used to measure the TN content (mg N g−1 DW) by colorimetric method using an assortment of H2SO4 and HClO4 acids (10:1; w/v) and determined using Nessler’s reagent (Polley, 1954). Total protein content was determined via a bicinchoninic acid (BCA) assay (Smith et al., 1985).
Analysis of fluoranthene in plant tissues
Plant roots and shoots were freeze-dried, ground, and homogenized. Each plant sample (0.5 g) was extracted by ultrasonication for 30 min in 10 ml acetone and hexane (1:1 [v/v]). The supernatant was collected, and the precipitate was re-extracted using the same solution; this process was repeated three times. All supernatants were combined and passed through an anhydrous Na2SO4 column, and the residue was eluted with 1:1 (v/v) acetone: hexane. The collected eluent was evaporated in a rotary evaporator and dissolved in 2 ml methanol. A 1-ml aliquot of each sample was filtered through a silica gel column (2 g), and the residue was washed and eluted with 11 ml of hexane and dichloromethane (1:1 [v/v]). The collected liquids were evaporated and dissolved in 2 ml methanol. After filtration through a 0.22-μm filter unit, the extracts were analyzed by HPLC. The recovery of Flu for this procedure was reported to be 89.5% (n = 3, relative standard deviation [RSD] < 1.6%).
An HPLC system (LC-20AT, Shimadzu, Japan) coupled to a UV detector and a 4.6 × 250 mm reverse phase C18 column was used for Flu analysis. Methanol was used as the mobile phase at a flow rate of 1 ml min−1 (30°C). The detection wavelength was 254 nm. Aliquots (20 μl) of each sample were injected into the HPLC system with an auto sampler. The average recovery obtained by spiking Arabidopsis samples with fluoranthene was 90.4% (n = 4) for the entire procedure. The fluoranthene detection limit by HPLC was 65.7 pg. Transfer coefficient (TF) was calculated as the ratio of fluoranthene content in shoot versus root (Zhan et al., 2015).
Statistical analysis
The analyses included one-way analysis of variance (ANOVA) and Duncan’s test (p < 0.05) to test the possible differences among treatments. Two-way analysis of variance (ANOVA) was used to assess the effects and interactions of species type and N form (SPSS 20.0, SPSS Inc., Chicago, IL, United States). Values are means ± standard error (SE; n = 4). The data were visualized by ImageGP (Chen et al., 2022). The relationship between total protein content (TPC), total nitrogen (TN), enzyme activity, root growth biomass, photosynthetic rates, transpiration rates, transfer coefficients (TF), and fluoranthene concentration was explored using a partial least squares path model (PLS-PM), a particularly useful statistical method for demonstrating cause and effect relationships among observed and latent variables (Tenenhaus et al., 2005). The estimates of path coefficients and the coefficients of determination (R2) in our path model were validated in R (v. 3.6.3) using the plspm package (1,000 bootstraps). Path coefficients (i.e., direct effects) represent the strength and direction of the linear relationships between variables, whereas indirect effects represent the multiplied path coefficients between a predictor and response variable, adding the product of all possible paths except the direct effect (Barberán et al., 2014). The final model was chosen from all constructed models based on the Goodness of Fit (GoF) statistic, a measure of the model’s overall predictive power. The correlation between potential fluoranthene accumulation and plant physicochemical properties were analyzed and visualized by R package ggClusterNet (Wen et al., 2022).
Results
Fluoranthene and N uptake
To determine the influence of N uptake on Flu uptake in rice and wheat, we measured the fluoranthene and TN content in the plants. Rice absorbed more fluoranthene than wheat under an equivalent N supply (Table 1). The fluoranthene concentration in the rice leaf and root was highest under the NH4+-N treatment and lowest under the NO3−-N treatment. By contrast, the fluoranthene concentration in wheat leaf and root was highest under NO3−-N treatment. Notably, TF of wheat was higher than that of rice under an equivalent N supply. Rice showed the highest TF for Flu under NH4+-N treatment, while wheat showed the highest TF under NO3−-N treatment. The TF resulting from NH4+-N + NO3−-N treatment was intermediate between that obtained for both species. Moreover, the Flu concentration and TF in rice or wheat were affected by plant species, N form, as well as the interaction of N form and species (Table 1).
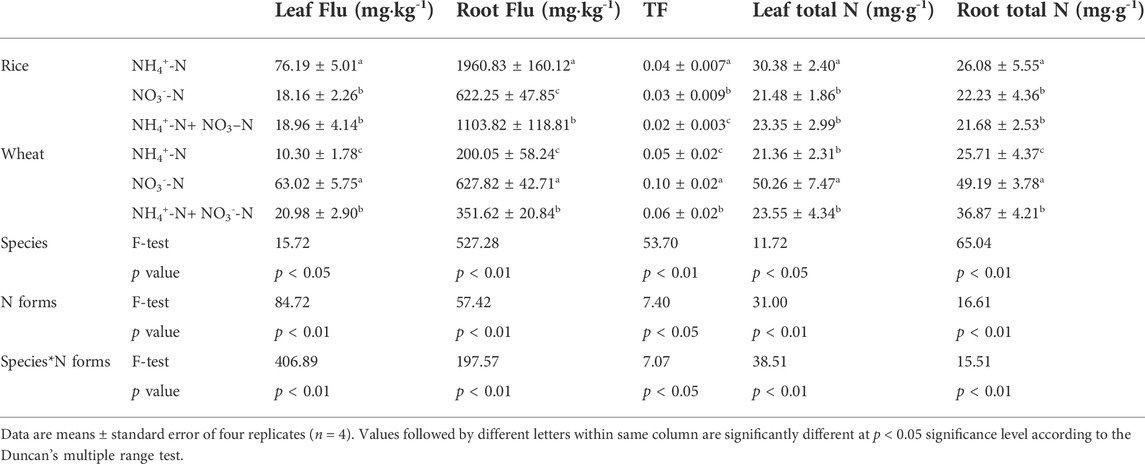
TABLE 1. The direct and indirect relationships between variables. The path coefficients are calculated by PLS-PM after 1,000 bootstraps.
The root TN content of wheat was higher than that of rice at equivalent applied N levels. The TN content of rice under NH4+-N treatment was higher than under NO3−-N treatment. Opposite trends were observed in wheat: The TN content was higher under NH4+-N treatment than under NO3−-N treatment. The TN content under NH4+-N + NO3−-N treatment was intermediate in both rice and wheat. Two-way analysis of variance (ANOVA) showed that the TN content in plants was influenced by plant species and N forms individually, but also by the interaction of both variables (Table 1). Thus, application of different N forms affects the TN content of the plants.
Plant growth and root morphology parameters
Plant growth in hydroponic solution varied for rice and wheat under different N forms (Figure 1). Rice treated with NH4+-N accumulated 74% higher biomass than rice treated with NO3−-N. The biomass of wheat under NO3−-N was 338% higher than that under NH4+-N treatment. Wheat biomass under NH4+-N + NO3−-N treatment was intermediate between the NH4+-N treatment and NO3−-N treatment (Figure 1A).
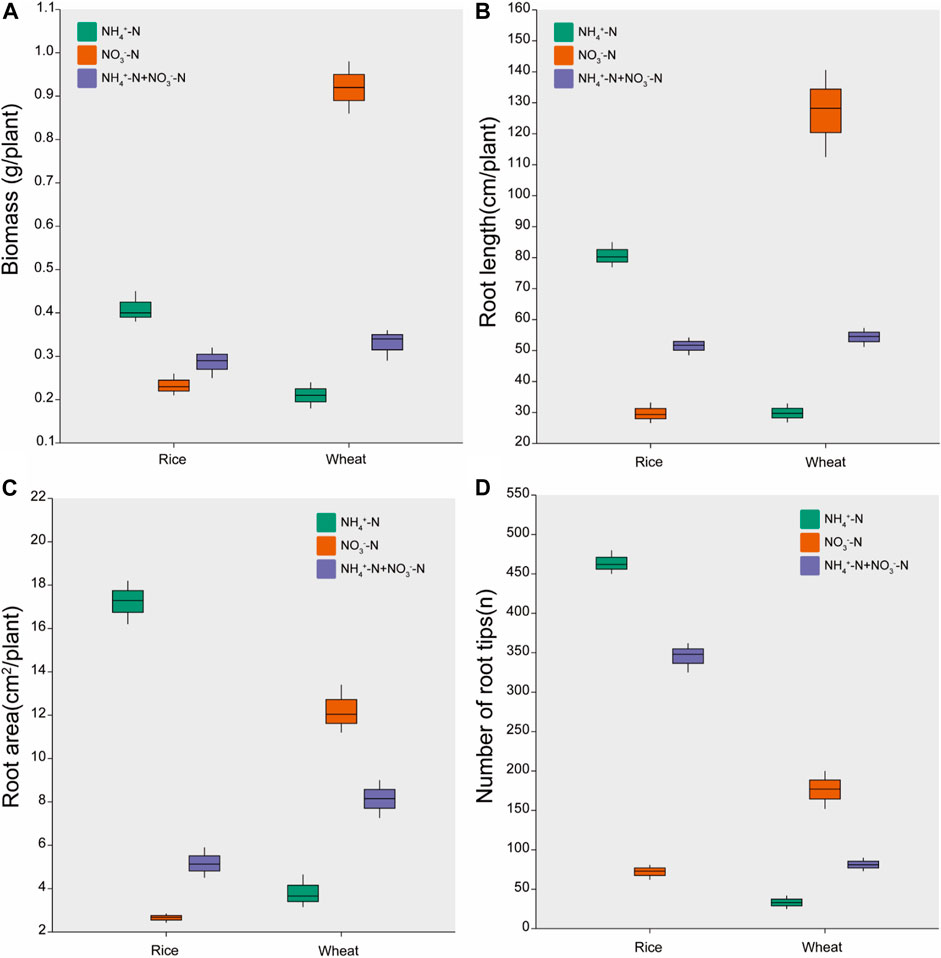
FIGURE 1. Effects of different nitrogen forms on the growth responses. Data are presented for (A) Plant biomass, (B) Root length, (C) Root area, and (D) number of root tips. Data are means ± standard error of four replicates (n = 4).
The root length, root surface area, and number of root tips were higher in rice treated with NH4+-N than with NO3−-N, but were higher in wheat treated with NO3−-N than with NH4+-N. These parameters were intermediate between the two different N treatments for the combined NH4+-N + NO3−-N treatment in both rice and wheat (Figures 1B–D).
Photosynthesis and transpiration
The photosynthetic and transpiration rates of rice under NH4+-N treatment were 164% and 114% higher than those observed under the NO3−-N treatment, respectively (Figure 2). For wheat, the photosynthetic and transpiration rates under the NO3−-N treatment were 243% and 77% higher than that under the NH4+-N treatment, respectively. The transpiration rate of wheat was higher than that of rice at equivalent nitrogen levels. The photosynthetic and transpiration rates in both rice and wheat were intermediate under the NH4+-N + NO3−-N treatment (Figure 2).
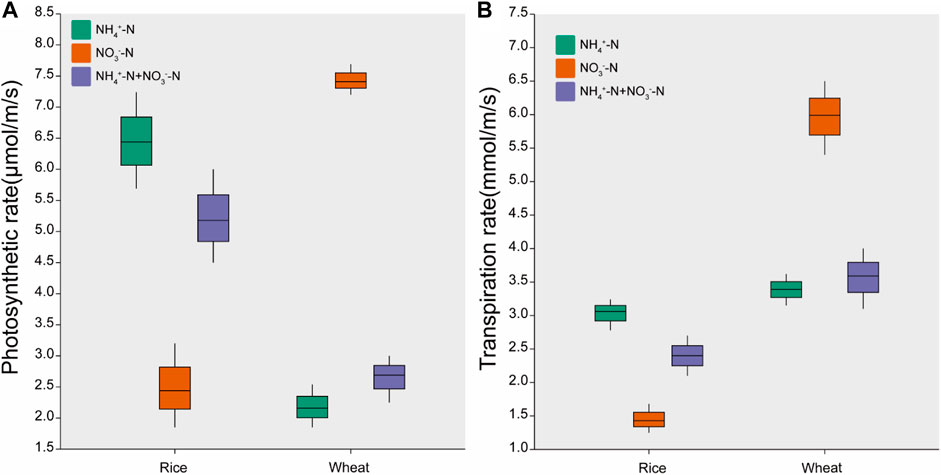
FIGURE 2. Effects of different nitrogen forms on (A) photosynthesis rate and (B) transpiration rate. Data are means ± standard error of four replicates (n = 4).
Antioxidant enzyme activities and MDA and total protein content
In both leaf and root tissue, superoxide dismutase (SOD) and catalase (CAT) antioxidant enzyme activities in rice were higher than those in wheat. In addition, the peroxidase (POD) activity of rice leaf was higher than that of wheat, but not the root (Table 2). Antioxidant enzyme activity was higher in rice under the NO3−-N treatment than the NH4+-N treatment. By contrast, the activity of these enzymes in wheat was higher under the NO3−-N treatment.
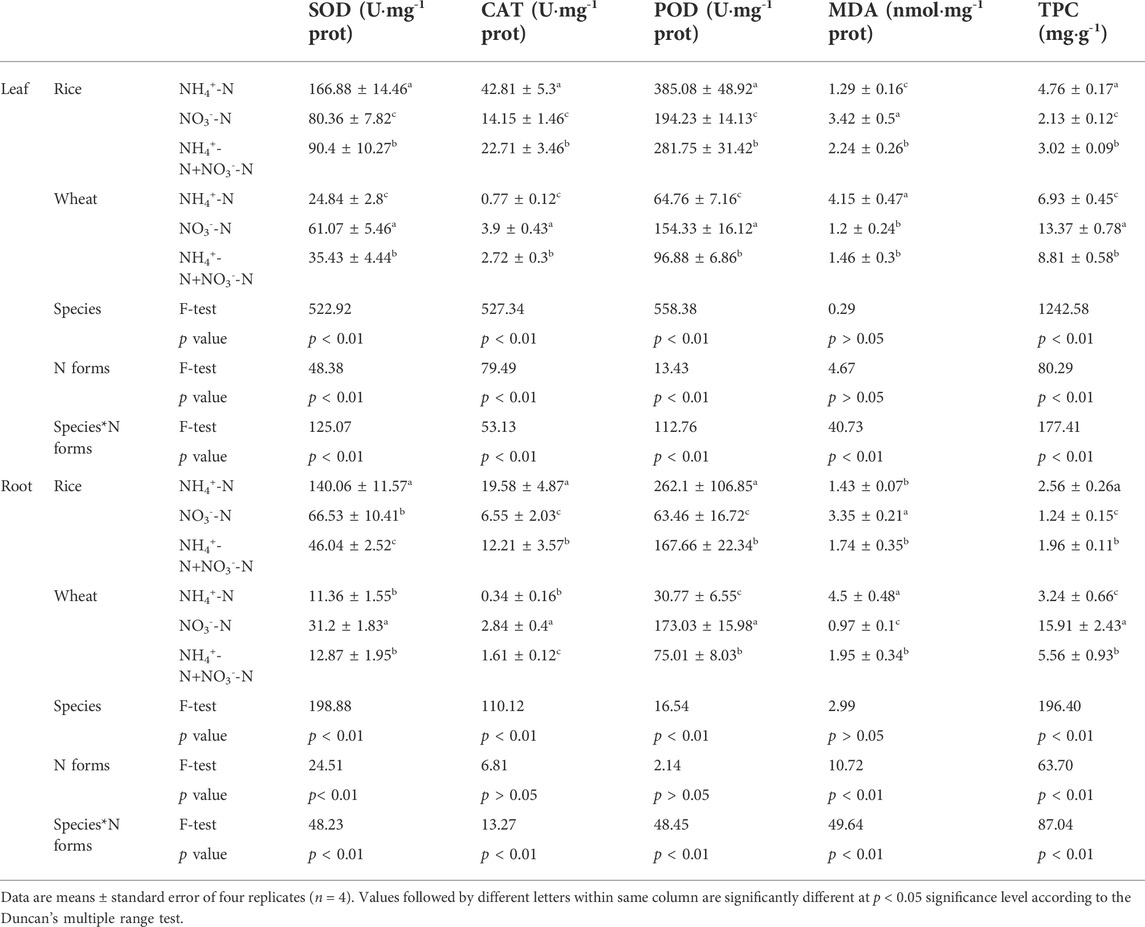
TABLE 2. Effect of different nitrogen forms on plant antioxidative enzymes activities, malondialdehyde and total protein content.
The malondialdehyde (MDA) content (an indicator of stress-induced damage to plant membranes) in rice under the NH4+-N treatment was lower than under the NO3−-N treatment (Table 2). The opposite trend was observed in wheat, where the MDA content under the NO3−-N treatment was lower than under the NH4+-N treatment.
The total protein content (TPC) of wheat was higher than that of rice under an equivalent nitrogen supply. In rice, the TPC was higher under the NH4+-N conditions than under NO3−-N conditions, while the TPC of wheat was higher under the NO3−-N conditions.
Enzymatic activity, MDA content, and TPC in rice and wheat under the combined NH4+-N + NO3−-N treatment were intermediate between the NH4+-N and NO3−-N treatments. Moreover, antioxidant enzyme activities, MDA content, and TPC were affected by plant species and N form, as well as by the interaction between N form and species (Table 2).
Correlation between fluoranthene uptake and plant physicochemical properties
According to Mantel tests, fluoranthene uptake by the plant was correlated with its physicochemical properties (Figure 3). Fluoranthene uptake in both rice and wheat exhibited a significant correlation with antioxidant enzyme activity, TN, TPC, photosynthetic and transpiration rates, and plant growth (p < 0.05). Among these physicochemical properties correlated with fluoranthene uptake, TN showed the strongest correlation with fluoranthene accumulation in rice and wheat.
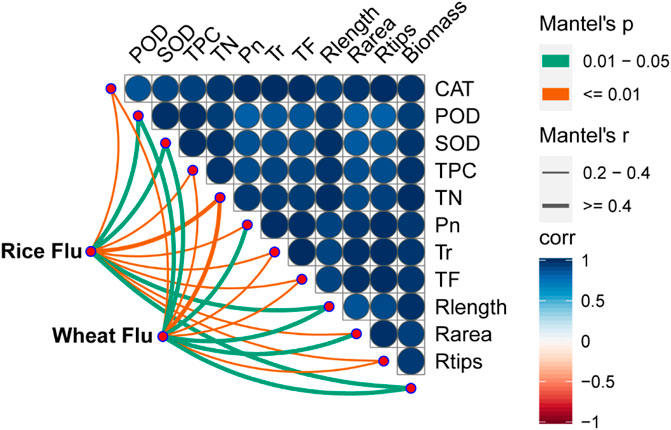
FIGURE 3. Parameters of plant drivers of the potentially fluoranthene accumulation in rice and wheat. Association of the potential fluoranthene accumulation (Bray-Curtis distance) and plant physicochemical properties were analyzed with Mantel tests. CAT: Catalase; POD: Peroxidase; SOD: Superoxide dismutase; TPC: Total protein content; TN: Total nitrogen; Pn: Photosynthesis; Tr: Transpiration; TF: Transfer coefficient; Rlength: Root length; Rarea: Root area; Rtips: Root tips. The edge width corresponds to the R value, and edge color denotes the statistical significance. The color gradient indicates Pearson correlation coefficients among the plant physicochemical properties and indicates no significant correlation at 0.05 level.
We next sought to further explore the effects of TPC, TN, enzyme activities, and plant growth on Flu accumulation. To this end, a partial least squares path model (PLS-PM) was constructed to assess direct and indirect effects between the observed indicators (Figure 4). We established that the NH4+-N treatment directly affected plant growth, TN, enzyme activities, and TPC in rice; the greatest effect was on TN, with a path coefficient of 0.85 (Figure 4A, Supplementary Table S1). In wheat, the NO3−-N treatment had a substantial effect on TN, with a path coefficient of 0.82 (Figure 4B, Supplementary Table S1). While plant growth, TN, enzyme activity, and TPC had direct effects on root fluoranthene uptake in rice and wheat, TN was the dominant factor affecting fluoranthene uptake in both species, with path coefficients of 0.74 for rice and 0.71 for wheat (Figures 4A,B). Root fluoranthene concentration had a direct effect on leaf fluoranthene concentration in rice and wheat with path coefficients of 0.78 and 0.75, respectively.
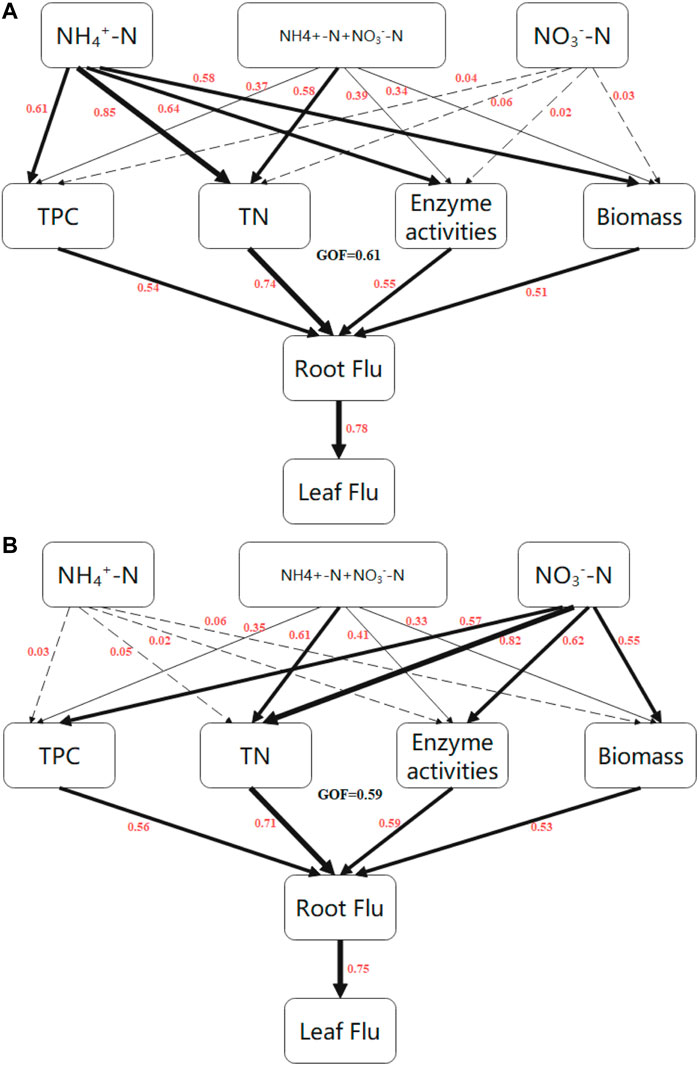
FIGURE 4. Directed graph of the partial least squares path model (PLS-PM) of rice (A) and wheat (B). Each box represents an observed variable (i.e., measured) or latent variable (i.e., constructs). PLS-PM describing the relationships between N forms, TPC, TN, enzyme activities, biomass, and Flu concentration. Flu: Fluoranthene concentration; T1\ Total nitrogen; TPC: Total protein concentration; Enzyme activities: Peroxidase, superoxide dismutase and catalase. Path coeffic ients are calculated after 1000 bootstraps and reflected in the width of the arrow, with hick indicating positive effects. Dashed arrows show that coefficients did not differ significantly from 0 (P >0.05). The model is assessed using the Goodness of Fit(GoF) statistic, and the GoF value was OA (A) and 0.59 (B), respectively.
Discussion
N supply modifies plant growth and root morphology, influencing fluoranthene uptake in plants
Plant biomass under various stresses is an important indicator of plant stress tolerance (Tarigholizadeh et al., 2021). PAH uptake is associated with biomass accumulation (Su et al., 2010). In this study, rice had the highest biomass under the NH4+-N treatment (Figure 1A). As an ammonium-preferring plant, increasing the ratio of NH4+-N under different NO3−/NH4+ ratios in rice could increase plant biomass (Wu et al., 2018). For wheat, biomass was highest under the NO3−-N treatment (Figure 1A). Similar results have shown that supplying wheat with NO3−-N led to a greater increase in biomass compared with NH4+-N when plants were exposed to Cd stress (Cheng et al., 2020). Our data showed that NH4+-N-treated rice and NO3−-N-treated wheat had the highest fluoranthene concentration in both the leaf and root tissues (Table 1). The increase in biomass facilitated the enhancement of photosynthetic rate and an increase in photosynthetic products, which in turn accelerated fluoranthene uptake, as supported by the correlation analysis (Figure 3). Similar results have been reported in cucumber (Cucumis sativus L.), demonstrating that an increase in biomass could promote phenanthrene uptake (Yang et al., 2012).
Rice root growth (root length, area, and number of root tips) significantly increased under the NH4+-N treatment compared with the NO3−-N treatment (Figures 1B–D). This may be associated with plant N accumulation under the different N treatments. In this study, total N in rice was higher under NH4+-N treatment than under NO3−-N treatment (Table 1). An increase in total N can promote rice root growth (Lucob-Agustin et al., 2020). Similarly, rice root growth was reported to be higher under NH4+-N conditions than under NO3−-N and mixed N conditions in response to Cd stress (Hassan et al., 2008).
In contrast with rice, the root growth of wheat treated with NO3−-N was higher than that of wheat treated with NH4+-N (Figures 1B–D). This may be associated with the enhanced activity of nitrate reductase in wheat, which could enhance N accumulation and facilitate root growth (Garnett and Smethurst, 1999; Jampeetong and Box, 2009). In wheat, NO3−-N could facilitate nitrate reductase activity to a greater extent than NH4+-N (Yuan et al., 2014). A previous study also showed that, under Cd stress, wheat root growth was higher in plants treated with NO3−-N than with NH4+-N (Cheng et al., 2020).
The highest fluoranthene concentrations were detected in rice under the NH4+-N treatment and in wheat under the NO3−-N treatment (Table 1). The Mantel test showed that root length, root area, and root tip number were positively correlated with root fluoranthene concentration (Figure 3). Plant root morphology influences the efficiency of nutrient acquisition, thus affecting PAH uptake (Liu et al., 2014). Zhan et al. (2013) revealed that the major factor promoting phenanthrene uptake was root-specific surface area. In addition, a previous study reported that longer roots increased root surface area, thereby providing a spatial location for PAH absorption (Parrish et al., 2004).
Interestingly, rice took up more fluoranthene than wheat under an equivalent N supply (Table 1). A similar study reported that PAH accumulation in rice was higher than in wheat (Li et al., 2017). Root surface area and root tip numbers are the most important factors influencing PAH uptake (Tao et al., 2004; Li et al., 2021). Rice roots with higher numbers of root tips than those of wheat under an equivalent N supply might be responsible for this (Figure 1D). Thus, different N applications affect the root morphology and, hence, the uptake of Flu by plants.
N supply improves plant N accumulation, increasing fluoranthene uptake
The TN of rice under NH4+-N treatment was higher than NO3−-N treatment, and the highest TN was found in wheat treated with NO3−-N (Table 1). The total N content in plants was correlated with the N form (Figure 4). A similar study reported that TN accumulation in rice was significantly correlated with NH4+-N in the soil (Chen et al., 2012). Huo et al. (2020) showed that total plant N increased in rice under Cu stress when N was supplied as NH4+-N rather than NO3−-N. Wheat TN was found to be positively correlated with soil NO3−-N content (Zhang et al., 2021).
In this study, rice treated with NH4+-N and wheat treated with NO3−-N had higher Flu concentrations at equivalent N levels. Correlation analysis showed a positive correlation between total N and fluoranthene uptake (Figure 3), and PLS-PM analysis further suggested that total N was the main factor affecting fluoranthene uptake (Figure 4). Similar studies also confirmed the contribution of total N content on PAH uptake. Wang et al. (2021) revealed that elevated total N content in rice could promote phenanthrene uptake. Similarly, Yang et al. (2012) reported that increased total N content in cucumber facilitated phenanthrene uptake. Different nitrogen applications affect the TN content of plants to influence the uptake of fluoranthene.
N supply enhances plant photosynthesis and transpiration, facilitating fluoranthene uptake and translocation
The photosynthetic rate of rice was higher under NH4+-N than under NO3−-N, while that of wheat was higher under NO3−-N (Figure 2A). Different N forms vary in their effect on crop plant photosynthesis (Shelp et al., 1991). A previous study reported that the photosynthetic rate of rice treated with NH4+-N was higher than that of rice treated with NO3−-N under Cd stress (Alpha et al., 2009). Another study reported that the photosynthetic rate of wheat treated with NO3−-N was higher than that of wheat treated with NH4+-N under Cd stress (Cheng et al., 2020).
The increased photosynthetic rate of wheat and rice by N application could enhance fluoranthene uptake. The positive correlation between photosynthetic rate and fluoranthene uptake was confirmed through a Mantel test (Figure 3). A previous study reported that an increased photosynthetic rate in tomato could promote the uptake of phenanthrene and pyrene (Ahammed et al., 2012). Another study reported that increased photosynthetic rate in cucumber also promoted phenanthrene uptake (Jin et al., 2017).
The transpiration rate of wheat was higher than that of rice. This may be due to smaller stomata and lower stomatal density in rice compared to wheat (Eberbach et al., 2011). The transpiration rate of rice under NH4+-N was higher than that under NO3−-N, and the transpiration rate of wheat under NO3−-N was the highest (Figure 2B). Research has shown that transpiration rate is positively correlated with photosynthetic rate, due to stomatal opening resulting from an enhanced photosynthetic rate (Tanaka et al., 2013; Wang et al., 2019).
An increased transpiration rate could promote fluoranthene transport in plants. Meudec et al. (2006) reported that transpiration was the main motive force for the transport of organic pollutants in plants. Another previous study showed that phenanthrene transport from root to shoot proceeded mainly through the transpiration stream (Gao & Zhu, 2004).
The transfer coefficient of fluoranthene was highest in rice under NH4+-N treatment and highest in wheat under NO3−-N treatment (Table 1). Correlation analysis showed that the fluoranthene transfer coefficient was positively correlated with transpiration rate (p < 0.01) (Figure 3). A previous study reported that the transfer coefficient of phenanthrene was higher in wheat under NO3−-N than under NH4+-N (Zhan et al., 2015). Another study reported that the transfer coefficient of phenanthrene in rice was higher under NH4+-N than under NO3−-N (Wang et al., 2021). It is worth noting that the transfer coefficient of wheat is higher than that of rice at the equivalent N level (Table 1) likely because the transpiration rate of wheat is higher than that of rice at the equivalent N level. Therefore, different N applications affect the photosynthetic and transpiration rates of plants to influence the uptake and translocation of fluoranthene.
N supply promotes antioxidant enzyme activities to alleviate oxidative stress and boost plant fluoranthene uptake
Plants possess efficient systems for scavenging reactive oxygen species to protect themselves from destructive oxidative reactions (Debnath et al., 2021). Antioxidant enzymes are key elements of this system. There have been many reports suggesting that the form of nitrogen supplied to plants not only influences their growth characteristics under non-stress conditions, but also affects their stress tolerance (Rios-Gonzalez et al., 2002). We observed that the antioxidant enzyme (SOD, POD, and CAT) activities in rice under NH4+-N treatment and wheat under NO3−-N treatment were higher than those at equivalent N levels (Table 2). A previous study showed that the antioxidant enzyme activity of rice under NH4+-N was higher than that under NO3−-N during Cd stress (Jalloh et al., 2009). However, another study also showed that the antioxidant enzyme activity in wheat was higher under NH4+-N than under NO3−-N under Cu stress (Zeng et al., 2021). This opposite result may be due to differences in pH; rhizosphere pH increases through the application of NO3−-N, which decreases the uptake of mineral nutrients and reduces resistance to stress pollutants (Xu et al., 2012). In this experiment, we adjusted the pH of the nutrient solutions to 5.5 each day. Thus, it is most likely that the antioxidant enzyme activity in wheat was indeed higher under NO3−-N than under NH4+-N (Table 2).
The elevated antioxidant enzyme activities promoted fluoranthene uptake. PLS-PM analysis showed that pollution caused an increase in antioxidant enzyme activity in plants, and the increase in antioxidant enzyme activity promoted the uptake of fluoranthene by plants (Figure 4). Previous studies have demonstrated that antioxidant enzyme activity increased with elevated PAH concentrations in plants (Yang et al., 2012; Shen et al., 2018). SOD plays a role in scavenging the superoxide anion free radical, converting it into peroxide (H2O2), while CAT and POD then break down H2O2 (Ahmad et al., 2010). Moreover, an increase in antioxidant enzyme activity improves the ability of plants to scavenge reactive oxygen species generated by PAHs entering the cells (Yang et al., 2012). Different nitrogen applications affect the antioxidant enzyme activity of plants. Ammonium-loving rice had the highest increase in antioxidant enzyme activity under NH4+-N condition, suggesting that the plant was better adapted to uptake and accumulate more fluoranthene. Similarly for wheat, which had higher antioxidant enzyme activity under NO3−-N condition and consequently was able to uptake and accumulate more fluoranthene.
In this study, the MDA content in rice under NH4+-N treatment and in wheat under NO3−-N treatment was lower than that under the equivalent N levels (Table 2). MDA is the direct production of lipid peroxidation, and its content is often used as an indicator of the extent of lipid peroxidation and is negatively correlated with antioxidant enzyme activity (Yang et al., 2012). A previous study reported a negative correlation between antioxidant enzyme activity and MDA content in tomato under phenanthrene and pyrene stress (Ahammed et al., 2012). Another study reported that antioxidant enzyme activity in wheat under phenanthrene stress was negatively correlated with MDA (Shen et al., 2018).
N supply increases TPC to improve fluoranthene uptake and translocation in plants
The TPC of rice was highest under NH4+-N treatment, while the TPC of wheat was highest under NO3−-N treatment. Accordingly, a previous study showed that the TPC of rice treated with NH4+-N was higher than that of NO3−-N-treated rice (Balkos et al., 2010). Another study reported that the TPC of wheat under NO3−-N treatment was higher than that of wheat under NH4+-N treatment (Carillo et al., 2005).
TPC was positively correlated with Flu uptake (Figure 3), suggesting that PAH uptake by plants requires the synthesis of proteins that mediate PAH transport. Indeed, Shen et al. (2020) reported that PAH transport in the xylem is an active energy-consuming process mediated by transport proteins. Another study reported that active uptake of phenanthrene by wheat roots may be mediated by transporters that remain uncharacterized (Zhan et al., 2010). Thus, different N applications affected the TPC of plants to influence fluoranthene uptake.
Taken together, the nutrient preference of plants influences fluoranthene uptake. A similar pattern has been reported for the heavy metal Cd (Cheng et al., 2016; Cheng et al., 2020). In this study, plant TN content was the main factor affecting fluoranthene uptake (Figure 4). Therefore, regulating the N forms applied to crops in the field could be an effective and safe strategy to reduce fluoranthene uptake and ensure agricultural product safety.
Conclusion
Fluoranthene uptake by rice and wheat is influenced by their nutrient preferences. NH4+-N-treated rice and NO3−-N-treated wheat absorb more fluoranthene than the corresponding plants subjected to equivalent nitrogen levels. The N form present in the growth medium affects plant growth, root morphology, photosynthetic and transpiration rates, TPC, TN content, and antioxidant enzyme activity and thereby facilitates fluoranthene uptake. Among these parameters, TN content was the key factor influencing fluoranthene uptake.
Data availability statement
The original contributions presented in the study are included in the article/Supplementary Material, further inquiries can be directed to the corresponding authors.
Author contributions
YX: conceptualization, methodology, writing—original draft. ZX and XZ: supervision, project administration, data curation, writing—review and editing. CZ and YL: data curation, visualization, investigation. XZ and SJ: investigation formal analysis. HL: data curation, validation, formal analysis. LX and FH: writing—reviewing and editing, supervision. All authors have read and agreed to the published version of the manuscript.
Funding
This study was financially supported by the National Natural Science Foundation (No. 41977123). The research was supported by the Hainan Provincial Joint Project of Sanya Yazhou Bay Science and Technology City, Grant No: 2021JJLH0093. Key R & D Program of Jiangsu province, China (BE2021378). Project of Sanya Yazhou Bay Science and Technology City, Grant No: SCKJ-JYRC-2022-79.
Conflict of interest
The authors declare that the research was conducted in the absence of any commercial or financial relationships that could be construed as a potential conflict of interest.
Publisher’s note
All claims expressed in this article are solely those of the authors and do not necessarily represent those of their affiliated organizations, or those of the publisher, the editors and the reviewers. Any product that may be evaluated in this article, or claim that may be made by its manufacturer, is not guaranteed or endorsed by the publisher.
Supplementary material
The Supplementary Material for this article can be found online at: https://www.frontiersin.org/articles/10.3389/fenvs.2022.987743/full#supplementary-material
References
Ahammed, G. J., Yuan, H.-L., Ogweno, J. O., Zhou, Y.-H., Xia, X.-J., Mao, W.-H., et al. (2012). Brassinosteroid alleviates phenanthrene and pyrene phytotoxicity by increasing detoxification activity and photosynthesis in tomato. Chemosphere 86 (5), 546–555. doi:10.1016/j.chemosphere.2011.10.038
Ahmad, P., Umar, S., and Sharma, S. (2010). Mechanism of free radical scavenging and role of phytohormones in plants under abiotic stresses. Plant Adapt. phytoremediation, 99–118. doi:10.1007/978-90-481-9370-7_5
Alpha, J. M., Chen, J., and Zhang, G. (2009). Effect of nitrogen fertilizer forms on growth, photosynthesis, and yield of rice under cadmium stress. J. plant Nutr. 32 (2), 306–317. doi:10.1080/01904160802608635
Aslam, M. S., Xue, P. H., Bashir, S., Alfakhri, Y., Nurunnabi, M., and Nguyen, V. C. (2021). Assessment of rice and wheat production efficiency based on data envelopment analysis. Environ. Sci. Pollut. Res. 28 (29), 38522–38534. doi:10.1007/s11356-021-12892-z
Balkos, K. D., Britto, D. T., and Kronzucker, H. J. (2010). Optimization of ammonium acquisition and metabolism by potassium in rice (Oryza sativa L. cv. IR‐72). Plant Cell. Environ. 33 (1), 23–34. doi:10.1111/j.1365-3040.2009.02046.x
Barberán, A., Ramirez, K. S., Leff, J. W., Bradford, M. A., Wall, D. H., and Fierer, N. (2014). Why are some microbes more ubiquitous than others? Predicting the habitat breadth of soil bacteria. Ecol. Lett. 17 (7), 794–802. doi:10.1111/ele.12282
Beauchamp, C., and Fridovich, I. (1971). Superoxide dismutase: Improved assays and an assay applicable to acrylamide gels. Anal. Biochem. 44 (1), 276–287. doi:10.1016/0003-2697(71)90370-8
Cai, Q.-Y., Mo, C.-H., Wu, Q.-T., Katsoyiannis, A., and Zeng, Q.-Y. (2008). The status of soil contamination by semivolatile organic chemicals (SVOCs) in China: A review. Sci. Total Environ. 389 (2–3), 209–224. doi:10.1016/j.scitotenv.2007.08.026
Carillo, P., Mastrolonardo, G., Nacca, F., and Fuggi, A. (2005). Nitrate reductase in durum wheat seedlings as affected by nitrate nutrition and salinity. Funct. Plant Biol. 32 (3), 209–219. doi:10.1071/fp04184
Chai, M., Li, R., Shen, X., Tam, N. F. Y., Zan, Q., and Li, R. (2018). Does ammonium nitrogen affect accumulation, subcellular distribution and chemical forms of cadmium in Kandelia obovata? Ecotoxicol. Environ. Saf. 162, 430–437. doi:10.1016/j.ecoenv.2018.07.031
Chapin, F. S., Moilanen, L., and Kielland, K. (1993). Preferential use of organic nitrogen for growth by a non-mycorrhizal arctic sedge. Nature 361 (6408), 150–153. doi:10.1038/361150a0
Chen, J., Zhou, H. C., Wang, C., Zhu, C. Q., and Tam, N. F.-Y. (2015). Short-term enhancement effect of nitrogen addition on microbial degradation and plant uptake of polybrominated diphenyl ethers (PBDEs) in contaminated mangrove soil. J. Hazard. Mater. 300, 84–92. doi:10.1016/j.jhazmat.2015.06.053
Chen, T., Liu, Y., and Huang, L. (2022). ImageGP: An easy‐to‐use data visualization web server for scientific researchers. iMeta 1, e5. doi:10.1002/imt2.5
Chen, X., Li, Y., Liu, L., Fang, S., Fang, P., and Lin, X. (2012). Effects of water management patterns and nitrogen fertilizer levels on nitrogen use efficiency of rice. Plant Nutr. Fertilizer Sci. 18 (2), 283–290.
Cheng, M., Wang, A., and Tang, C. (2017). Ammonium-based fertilizers enhance Cd accumulation in Carpobrotus rossii grown in two soils differing in pH. Chemosphere 188, 689–696. doi:10.1016/j.chemosphere.2017.09.032
Cheng, M., Wang, P., Kopittke, P. M., Wang, A., Sale, P. W. G., and Tang, C. (2016). Cadmium accumulation is enhanced by ammonium compared to nitrate in two hyperaccumulators, without affecting speciation. J. Exp. Bot. 67 (17), 5041–5050. doi:10.1093/jxb/erw270
Cheng, Y., Bao, Y., Chen, X., Yao, Q., Wang, C., Chai, S., et al. (2020). Different nitrogen forms differentially affect Cd uptake and accumulation in dwarf Polish wheat (Triticum polonicum L.) seedlings. J. Hazard. Mater. 400, 123209. doi:10.1016/j.jhazmat.2020.123209
Cheng, Y., Wang, C., Chai, S., Shuai, W., Sha, L., Zhang, H., et al. (2018). Ammonium N influences the uptakes, translocations, subcellular distributions and chemical forms of Cd and Zn to mediate the Cd/Zn interactions in dwarf polish wheat (Triticum polonicum L.) seedlings. Chemosphere 193, 1164–1171. doi:10.1016/j.chemosphere.2017.11.058
Debnath, S., Chandel, R. K., Devi, K., and Khan, Z. (2021). “Mechanism and molecular response of induced genotoxicity and oxidative stress in plants,” in Induced genotoxicity and oxidative stress in plants, 213–227.
Eberbach, P. L., Humphreys, E., and Kukal, S. S. (2011). The effect of rice straw mulch on evapotranspiration, transpiration and soil evaporation of irrigated wheat in Punjab. India. Agric. Water Manag. 98 (12), 1847–1855.
Gao, Y., and Zhu, L. (2004). Plant uptake, accumulation and translocation of phenanthrene and pyrene in soils. chemosphere 55 (9), 1169–1178. doi:10.1016/j.chemosphere.2004.01.037
Garnett, T. P., and Smethurst, P. J. (1999). Ammonium and nitrate uptake by Eucalyptus nitens: Effects of pH and temperature. Plant Soil 214 (1), 133–140. doi:10.1023/a:1004740204876
Guan, M. Y., Fan, S. K., Fang, X. Z., and Jin, C. W. (2015). Modification of nitrate uptake pathway in plants affects the cadmium uptake by roots. Plant Signal. Behav. 10 (3), e990794. doi:10.4161/15592324.2014.990794
Hassan, M. J., Shafi, M., Zhang, G., Zhu, Z., and Qaisar, M. (2008). The growth and some physiological responses of rice to Cd toxicity as affected by nitrogen form. Plant Growth Regul. 54 (2), 125–132. doi:10.1007/s10725-007-9235-6
Hassan, M. J., Wang, F., Ali, S., and Zhang, G. (2005). Toxic effect of cadmium on rice as affected by nitrogen fertilizer form. Plant Soil 277 (1), 359–365. doi:10.1007/s11104-005-8160-6
Huo, K., Shangguan, X., Xia, Y., Shen, Z., and Chen, C. (2020). Excess copper inhibits the growth of rice seedlings by decreasing uptake of nitrate. Ecotoxicol. Environ. Saf. 190, 110105. doi:10.1016/j.ecoenv.2019.110105
Jalloh, M. A., Chen, J., Zhen, F., and Zhang, G. (2009). Effect of different N fertilizer forms on antioxidant capacity and grain yield of rice growing under Cd stress. J. Hazard. Mater. 162 (2–3), 1081–1085. doi:10.1016/j.jhazmat.2008.05.146
Jampeetong, A., and Brix, H. (2009). Nitrogen nutrition of Salvinia natans: Effects of inorganic nitrogen form on growth, morphology, nitrate reductase activity and uptake kinetics of ammonium and nitrate. Aquat. Bot. 90 (1), 67–73. doi:10.1016/j.aquabot.2008.06.005
Jin, L., Che, X., Zhang, Z., Li, Y., Gao, H., and Zhao, S. (2017). The mechanisms by which phenanthrene affects the photosynthetic apparatus of cucumber leaves. Chemosphere 168, 1498–1505. doi:10.1016/j.chemosphere.2016.12.002
Larsson Jönsson, E. H., and Asp, H. (2013). Effects of pH and nitrogen on cadmium uptake in potato. Biol. plant. 57 (4), 788–792. doi:10.1007/s10535-013-0354-9
Li, N., Możdżeń, K., Zhang, Z., Liu, C., Zandi, P., Sardar, M. F., et al. (2021). Rhizosphere effect on removal and bioavailability of PAHs in contaminated agricultural soil. Biologia 76 (3), 841–851. doi:10.1007/s11756-021-00716-x
Li, Y., Long, L., Ge, J., Yang, L., Cheng, J., Sun, L., et al. (2017). Presence, distribution and risk assessment of polycyclic aromatic hydrocarbons in rice-wheat continuous cropping soils close to five industrial parks of Suzhou, China. Chemosphere 184, 753–761. doi:10.1016/j.chemosphere.2017.06.055
Li, Y., Ma, J., Li, Y., Xiao, C., Shen, X., Chen, J., et al. (2022). Nitrogen addition facilitates phytoremediation of PAH-Cd cocontaminated dumpsite soil by altering alfalfa growth and rhizosphere communities. Sci. Total Environ. 806, 150610. doi:10.1016/j.scitotenv.2021.150610
Liao, C., Kim, U.-J., and Kannan, K. (2018). A review of environmental occurrence, fate, exposure, and toxicity of benzothiazoles. Environ. Sci. Technol. 52 (9), 5007–5026. doi:10.1021/acs.est.7b05493
Liu, H., Meng, F., Tong, Y., and Chi, J. (2014). Effect of plant density on phytoremediation of polycyclic aromatic hydrocarbons contaminated sediments with Vallisneria spiralis. Ecol. Eng. 73, 380–385. doi:10.1016/j.ecoleng.2014.09.084
Lucob-Agustin, N., Hasegawa, T., Jinno, K., Suralta, R. R., Niones, J. M., Kano-Nakata, M., et al. (2020). Enhanced root system development responses of a newly identified mutation gene promoting lateral root development to various nitrogen conditions in rice. J. Int. Coop. Agric. Dev. 18, 48–55.
Luo, B. F., Du, S. T., Lu, K. X., Liu, W. J., Lin, X. Y., and Jin, C. W. (2012). Iron uptake system mediates nitrate-facilitated cadmium accumulation in tomato (Solanum lycopersicum) plants. J. Exp. Bot. 63 (8), 3127–3136. doi:10.1093/jxb/ers036
Meudec, A., Dussauze, J., Deslandes, E., and Poupart, N. (2006). Evidence for bioaccumulation of PAHs within internal shoot tissues by a halophytic plant artificially exposed to petroleum-polluted sediments. Chemosphere 65 (3), 474–481. doi:10.1016/j.chemosphere.2006.01.058
Mussarat, M., Shair, M., Muhammad, D., Mian, I. A., Khan, S., Adnan, M., et al. (2021). Accentuating the role of nitrogen to phosphorus ratio on the growth and yield of wheat crop. Sustainability 13 (4), 2253. doi:10.3390/su13042253
Parrish, Z. D., Banks, M. K., and Schwab, A. P. (2004). Effectiveness of phytoremediation as a secondary treatment for polycyclic aromatic hydrocarbons (PAHs) in composted soil. Int. J. Phytoremediation 6 (2), 119–137. doi:10.1080/16226510490454803
Polley, J. R. (1954). Colorimetric determination of nitrogen in biological materials. Anal. Chem. 26 (9), 1523–1524. doi:10.1021/ac60093a039
Rios-Gonzalez, K., Erdei, L., and Lips, S. H. (2002). The activity of antioxidant enzymes in maize and sunflower seedlings as affected by salinity and different nitrogen sources. Plant Sci. 162 (6), 923–930. doi:10.1016/s0168-9452(02)00040-7
Shelp, B. J., Taylor, D. C., and Nelson, L. M. (1991). Carbon and nitrogen partitioning in young nodulated pea (wild type and nitrate reductase-deficient mutant) plants exposed to NO3− or NH4+. Can. J. Bot. 69 (8), 1780–1786. doi:10.1139/b91-226
Shen, Y., Li, J., Gu, R., Yue, L., Wang, H., Zhan, X., et al. (2018). Carotenoid and superoxide dismutase are the most effective antioxidants participating in ROS scavenging in phenanthrene accumulated wheat leaf. Chemosphere 197, 513–525. doi:10.1016/j.chemosphere.2018.01.036
Shen, Y., Sheng, Y., Li, J., Zhu, J., Shi, S., and Zhan, X. (2020). The role of temperature in phenanthrene transfer and accumulation in crop leaves. Environ. Pollut. 258, 113827. doi:10.1016/j.envpol.2019.113827
Singh, L., and Agarwal, T. (2018). Polycyclic aromatic hydrocarbons in diet: Concern for public health. Trends food Sci. Technol. 79, 160–170. doi:10.1016/j.tifs.2018.07.017
Smith, P. K. etal, Krohn, R. Il, Hermanson, G. T., Mallia, A. K., Gartner, F. H., Provenzano, Md., et al. (1985). Measurement of protein using bicinchoninic acid. Anal. Biochem. 150 (1), 76–85. doi:10.1016/0003-2697(85)90442-7
Su, Y.-H., McGrath, S. P., and Zhao, F.-J. (2010). Rice is more efficient in arsenite uptake and translocation than wheat and barley. Plant Soil 328 (1), 27–34. doi:10.1007/s11104-009-0074-2
Tanaka, Y., Sugano, S. S., Shimada, T., and Hara‐Nishimura, I. (2013). Enhancement of leaf photosynthetic capacity through increased stomatal density in Arabidopsis. New Phytol. 198 (3), 757–764. doi:10.1111/nph.12186
Tao, S., Cui, Y. H., Xu, F. L., Li, B. G., Cao, J., Liu, W. X., et al. (2004). Polycyclic aromatic hydrocarbons (PAHs) in agricultural soil and vegetables from Tianjin. Sci. Total Environ. 320 (1), 11–24. doi:10.1016/s0048-9697(03)00453-4
Tarigholizadeh, S., Motafakkerazad, R., Salehi- Lisar, S. Y., and Kazemi, E. M. (2021). High resistance of Panicum miliaceum L. to phenanthrene toxicity based on growth response and antioxidant system assessment. Acta Agric. Slov. 117 (2), 1–13. doi:10.14720/aas.2021.117.2.1987
Tenenhaus, M., Vinzi, V. E., Chatelin, Y.-M., and Lauro, C. (2005). PLS path modeling. Comput. statistics data analysis 48 (1), 159–205. doi:10.1016/j.csda.2004.03.005
Thompson, O. A., Wolf, D. C., Mattice, J. D., and Thoma, G. J. (2008). Influence of nitrogen addition and plant root parameters on phytoremediation of pyrene-contaminated soil. Water Air Soil Pollut. 189, 37–47. doi:10.1007/s11270-007-9552-4
Tian, K., Bao, H., Zhang, X., Shi, T., Liu, X., and Wu, F. (2018). Residuals, bioaccessibility and health risk assessment of PAHs in winter wheat grains from areas influenced by coal combustion in China. Sci. Total Environ. 618, 777–784. doi:10.1016/j.scitotenv.2017.08.174
Upadhyaya, A., Sankhla, D., Davis, T. D., Sankhla, N., and Smith, B. N. (1985). Effect of paclobutrazol on the activities of some enzymes of activated oxygen metabolism and lipid peroxidation in senescing soybean leaves. J. plant physiology 121 (5), 453–461. doi:10.1016/s0176-1617(85)80081-x
Wang, H., Yan, S., Xin, H., Huang, W., Zhang, H., Teng, S., et al. (2019). A subsidiary cell-localized glucose transporter promotes stomatal conductance and photosynthesis. Plant Cell. 31 (6), 1328–1343. doi:10.1105/tpc.18.00736
Wang, X., Jain, A., Huang, X., Lan, X., Xu, L., Zhao, G., et al. (2021). Reducing phenanthrene uptake and translocation, and accumulation in the seeds by overexpressing OsNRT2.3b in rice. Sci. Total Environ. 761, 143690. doi:10.1016/j.scitotenv.2020.143690
Wen, T., Xie, P., Yang, S., Niu, G., Liu, X., Ding, Z., et al. (2022). ggClusterNet: An R package for microbiome network analysis and modularity‐based multiple network layouts. Nanjing, China: iMeta, e32.
Wills, E. D. (1987). Biochemical toxicology: A practical approach.Evaluation of lipid peroxidation in lipids and biological membranes
Wu, Z., Zhang, W., Xu, S., Shi, H., Wen, D., Huang, Y., et al. (2018). Increasing ammonium nutrition as a strategy for inhibition of cadmium uptake and xylem transport in rice (Oryza sativa L.) exposed to cadmium stress. Environ. Exp. Bot. 155, 734–741. doi:10.1016/j.envexpbot.2018.08.024
Xu, G., Fan, X., and Miller, A. J. (2012). Plant nitrogen assimilation and use efficiency. Annu. Rev. Plant Biol. 63, 153–182. doi:10.1146/annurev-arplant-042811-105532
Yang, X., Guo, S., Guo, L., and Wei, C. (2012). Ammonium enhances the uptake, bioaccumulation, and tolerance of phenanthrene in cucumber seedlings. Plant Soil 354 (1), 185–195. doi:10.1007/s11104-011-1054-x
Yang, Y., Xiong, J., Tao, L., Cao, Z., Tang, W., Zhang, J., et al. (2020). Regulatory mechanisms of nitrogen (N) on cadmium (Cd) uptake and accumulation in plants: A review. Sci. Total Environ. 708, 135186. doi:10.1016/j.scitotenv.2019.135186
Yi, J., Gao, J., Zhang, W., Zhao, C., Wang, Y., and Zhen, X. (2019). Differential uptake and utilization of two forms of nitrogen in japonica rice cultivars from north-eastern China. Front. Plant Sci. 10, 1061. doi:10.3389/fpls.2019.01061
Yin, X., Liang, X., Zhang, R., Yu, L., Xu, G., Zhou, Q., et al. (2015). Impact of phenanthrene exposure on activities of nitrate reductase, phosphoenolpyruvate carboxylase, vacuolar H+-pyrophosphatase and plasma membrane H+-ATPase in roots of soybean, wheat and carrot. Environ. Exp. Bot. 113, 59–66. doi:10.1016/j.envexpbot.2015.02.001
Yuan, J., Yi, X., Gu, S., Zhan, X., Zhou, L., and Xu, R. (2014). Nitrate reductase activity of crop roots in the presence of phenanthrene and NH4+-n, no3--n. J. Nanjing Agric. Univ. 37 (1), 101–107.
Zeng, J., Tang, J., Zhang, F., Wang, Y., Kang, H., Chen, G., et al. (2021). Ammonium regulates redox homeostasis and photosynthetic ability to mitigate copper toxicity in wheat seedlings. Ecotoxicol. Environ. Saf. 226, 112825. doi:10.1016/j.ecoenv.2021.112825
Zhan, X.-H., Ma, H.-L., Zhou, L.-X., Liang, J.-R., Jiang, T.-H., and Xu, G.-H. (2010). Accumulation of phenanthrene by roots of intact wheat (Triticum acstivnm L.) seedlings: Passive or active uptake? BMC Plant Biol. 10 (1), 52–58. doi:10.1186/1471-2229-10-52
Zhan, X., Liang, X., Xu, G., and Zhou, L. (2013). Influence of plant root morphology and tissue composition on phenanthrene uptake: Stepwise multiple linear regression analysis. Environ. Pollut. 179, 294–300. doi:10.1016/j.envpol.2013.04.033
Zhan, X., Yi, X., Yue, L., Fan, X., Xu, G., and Xing, B. (2015). Cytoplasmic pH-stat during phenanthrene uptake by wheat roots: A mechanistic consideration. Environ. Sci. Technol. 49 (10), 6037–6044. doi:10.1021/acs.est.5b00697
Zhan, X., Yuan, J., Yue, L., Xu, G., Hu, B., and Xu, R. (2015). Response of uptake and translocation of phenanthrene to nitrogen form in lettuce and wheat seedlings. Environ. Sci. Pollut. Res. 22, 6280–6287. doi:10.1007/s11356-014-3834-3
Zhang, G., Zhang, J., Xu, L., Zhou, Y., Hou, P., Yang, F., et al. (2022). Study on the nutrient optimal management strategy of high and stable Annual yield in the rice–wheat system: A 10-year term experiment. Agronomy 12 (3), 698. doi:10.3390/agronomy12030698
Zhang, J., Cai, Z., and Müller, C. (2018). Terrestrial N cycling associated with climate and plant‐specific N preferences: A review. Eur. J. Soil Sci. 69 (3), 488–501. doi:10.1111/ejss.12533
Zhang, J., Wei, Q., Xiong, S., Shi, L., Ma, X., Du, P., et al. (2021). A spectral parameter for the estimation of soil total nitrogen and nitrate nitrogen of winter wheat growth period. Soil Use Manag. 37 (4), 698–711. doi:10.1111/sum.12639
Keywords: fluoranthene, nitrogen form, rice, wheat, PAHs
Citation: Xu Y, Xiao Z, Zhang X, Zhao C, Li Y, Che T, Li Y, Zang X, Jiang S, Li H, Xu L and Hu F (2022) The nutrient preferences of rice and wheat influence fluoranthene uptake. Front. Environ. Sci. 10:987743. doi: 10.3389/fenvs.2022.987743
Received: 06 July 2022; Accepted: 27 July 2022;
Published: 31 August 2022.
Edited by:
Xijuan Chen, Institute of Applied Ecology (CAS), ChinaReviewed by:
Xiao-San Luo, Nanjing University of Information Science and Technology, ChinaYong-Xin Liu, Institute of Genetics and Developmental Biology (CAS), China
Copyright © 2022 Xu, Xiao, Zhang, Zhao, Li, Che, Li, Zang, Jiang, Li, Xu and Hu. This is an open-access article distributed under the terms of the Creative Commons Attribution License (CC BY). The use, distribution or reproduction in other forums is permitted, provided the original author(s) and the copyright owner(s) are credited and that the original publication in this journal is cited, in accordance with accepted academic practice. No use, distribution or reproduction is permitted which does not comply with these terms.
*Correspondence: Li Xu, eHVsaTYwMkBuamF1LmVkdS5jbg==; Feng Hu, ZmVuZ2h1QG5qYXUuZWR1LmNu