- School of Economics, College of Business and Economics, University of Johannesburg, Johannesburg, South Africa
The asymmetric impact of technological innovation on carbon dioxide (CO2) emissions in South Africa from 1960 to 2020 is evaluated in this study. We apply the newly established quantile autoregressive distributed lag (QARDL) methodology to deal with distributional asymmetry based on the location of CO2 emissions within its own distribution. This distinguishes our analysis from earlier studies in the following way. In contrast to other studies, this research uses the QARDL technique to assess the long-term stability across the quantiles, resulting in a more adaptable econometric analysis than the traditional frameworks. In order to capture the trade share in South Africa’s GDP and the quantity of trade compared to world trade, we employ a novel measure of trade openness. We find that 1) technological innovation helps reduce CO2 emissions in the short term and over the long term; 2) the scale effect worsens CO2 emissions, whereas the technique effect enhances it, supporting the existence of an environmental Kuznets curve (EKC) hypothesis; 3) energy consumption, foreign direct investment (FDI), and industrial added value degrade environmental quality; and 4) increasing trade openness is glaringly harmful to the environment over the long term, despite being beneficial in the short term; 5) there are long-term, asymmetric linkages between CO2 emissions, scale effect, technique effect, technological innovation, energy use, FDI, and trade openness; 6) industrial value-added, scale effect, technique effect, technical innovation, energy usage, FDI, and trade openness Granger-cause CO2 emissions over the medium, long, and short terms indicate the significance of these variables in determining CO2 emissions. Based on our empirical findings, this study makes the case that South Africa’s government and policymakers should consider the importance of innovative technologies as a sustainable source of advancements in attaining energy security and promoting ecological integrity in the nation.
Introduction
Governments all over the world are becoming more and more concerned about environmental degradation as it has an impact on global warming and has the potential to disrupt the planet’s carbon cycle. Climate change is currently one of the most important issues facing humanity. Unparalleled threats to growth and human existence are shown by climate change brought on by greenhouse gas emissions (GHGs), primarily carbon dioxide (CO2) pollution. These risks include extreme weather, the extinction of species, and a food crisis (Bales and Sovacool, 2021; Udeagha and Ngepah, 2022a). The main human activity that contributes to CO2 emissions is the combustion of fossil fuels (coal and natural gas) for transportation and energy. However, some manufacturing processes and alterations to land usage still result in CO2 emissions. Extreme weather occurrences, such as floods, heatwaves, storms, droughts, rising sea levels, disruptions to water systems, and stunted plant growth are just a few of the many possible health, physical, and ecological repercussions of climate change and global warming (Udeagha and Muchapondwa, 2022). A nation may endure environmental deterioration as a result of the enormous cost of saving endangered species and cleaning up landfills, which might have detrimental economic repercussions. Thus, one of the contemporary global challenges included in the political frameworks of many countries is environmental protection. With the expectation that these studies will shed light on the macroeconomic drivers of climate change, several scientific investigations have been conducted to identify the components that influence pollution to comprehend the economic aspects of environmental degradation.
In recent years, climate change and ecological degradation have become the most urgent economic issues. The fundamental causes of climate change and global warming are GHGs. Conversely, as the primary greenhouse gas, CO2 emissions have attracted considerable attention in the environmental literature (Abid et al., 2022). As shown by the International Energy Agency, rising fossil fuel consumption has caused CO2 emissions to soar, necessitating a swift transition to lower CO2 emissions and meet sustainable goals. Additionally, the sustainable development goals (7, 8, 9, 12, and 13) established by the United Nations, which must be achieved by the year 2030, emphasize the urgent need for solutions to combat climate change, including affordable clean energy, sustainable economic growth, technological innovation, sustainable consumption, and production (Wang et al., 2022a). As a result, many nations now place a high priority on lowering carbon emissions, and the advancement of technology has also played an important role in promoting changes to the direction of global economic development (Udeagha and Ngepah, 2022b). It is recognized that the idea of technological innovation is a factor that may reduce energy consumption, cut pollutant emissions, enhance environmental quality, and encourage the growth of a greener economy (Li et al., 2022). The use of innovative methods to create eco-friendly products that consume less energy and pollute the environment is referred to as technological innovation. Creating clean energy, using renewable energies, and manufacturing techniques that are less environmentally destructive than fossil fuels are all examples of technological breakthroughs (Chhabra et al., 2022). Additionally, technological advancement helps governments maximize their use of renewables and develop alternative fuels (Adebayo et al., 2022). It has been stated that the advancement of technological improvements and alternative energies in emerging economies will be an effective tool for reducing environmental emissions and achieving long-term environmental sustainability (Udeagha and Breitenbach, 2021; Kuang et al., 2022). Consequently, investing in eco-friendly technological innovations can increase the sustainability of production and economic engagement and offer a viable remedy for lowering carbon emissions in emerging economies such as South Africa. Therefore, technological innovation is acknowledged as one of the tactics the nation may employ to improve the sustainability of the environment and accomplish sustainable economic development because it provides an opportunity to drastically cut energy consumption (EC) and increase energy efficiency.
Technological innovations are important in increasing energy efficiency, reducing energy consumption, and minimizing CO2 emissions (Erdogan, 2021). Fisher-Vanden et al. (2004), Hang and Tu (2007), Zhou et al. (2010), and Jiahua et al. (2010) showed that technological innovation provides strong opportunities to fulfill the energy mandate by allowing the country to switch from exhaustible energy sources to renewable ones, allows the country to achieve higher production levels with a minimum of energy, and improves innovation, which leads to more entrepreneurship via improved market access and increased competition. Technological innovation by opening up international commodity markets is a way to generate new investment, increase productivity, and improve employment and real wages (Berg and Krueger, 2003). It also promotes resource allocation efficiency, which brings about better economic growth. This could ultimately lead to massive factor accumulation, information spillovers, and the spread of technology (Das and Paul, 2011; Zahonogo, 2017; Udeagha and Ngepah, 2020; Udeagha MC. and Ngepah, N. 2021). Improved use of technological innovations is important in promoting a green economy and reducing emissions of growing CO2. Improved use of electric vehicles, hybrid technology, and renewable energy sources reduces pollution and fossil fuels. In the process of becoming a reality, sustainable innovation highlights the interplay between scientific and technical advancement and the atmosphere. Moreover, the country’s natural environment and economic gains will be directly impacted by innovation capacity. In order to “decouple” the rate of scientific and technical advancement from resource utilization, the catalyst for environmental sustainability in South Africa is to encourage sustainable urban economic innovation and competitiveness.
Decoupling theory is the fundamental hypothesis put out by the Organization for Economic Co-operation and Development (OECD) to break the relationship between economic development and resource use or ecological damage (OECD, 2002). The phrase “decoupling” refers to a break in the link between economic development and resource usage or environmental damage (Enevoldsen et al., 2007). The environmental Kuznets curve (EKC) may be used to describe how the decoupling trajectory manifests. The EKC theory argues that early economic development is accompanied by an increase in environmental pressure. Notwithstanding, in the medium term, under the combined effects of economic, structural, technological, and governmental environmental regulations, a progression trend appears after environmental pollution attains a maximum point, finally realizing the best state of strong decoupling between economic development and environmental pollution (Xia and Zhong, 2016). The burden of resource extraction on the atmosphere is measured by this idea’s ability to assess the coupling fracture connection between human pursuits (the driving force) and that load. In academics, the study of decoupling has recently gained popularity. Economic development and transportation (Tapio, 2005), environmental quality and economic growth (Yang and Meng, 2019), and energy utilization and economic growth (Roman-Collado et al., 2018) have been the key themes of decoupling investigations. The level of reliance between innovation and resource consumption is primarily what is meant by the decoupling between innovation capabilities and resource consumption. The act of dependence building shifts from a strong to a weak connection, diminishing with time, before eventually showing a reversal change. The precise occurrence of this process is the progressive strengthening of innovation in the urban economy and the shift in the urban economy’s growth pattern to a green mode. For instance, Balsa-Barreiro et al. (2019) demonstrated how some pertinent human indicators (e.g., wealth production, GDP, environmental costs, and CO2 emissions) described different trajectories at a global level, highlighting the decoupling effect brought on by technological innovation at this point. At the global level, the shifting of the GDP and CO2 emissions traces from west to east may be explained by the decreasing importance of the western nations, where wealth is typically accumulated, as well as the growing significance of Southeast Asia. Grether and Mathys (2009) stated that it is crucial to underline that the CO2 emission trace is found farther to the east. This concerns the economy’s prospective coupling or decoupling with the energy sector. The western nations have gone through an industrial and economic transition, concentrating their economies on the services sector. In an international setting, several nations have transplanted their traditional industries in emerging nations to reduce prices and ecological deterioration (Balsa-Barreiro et al., 2019). As a result, most of them have entered what is known as the “strong decoupling phase,” during which they are growing their GDP while simultaneously lowering their ecological impact in absolute terms (Szigeti et al., 2017).
In recent years, the impact of technological innovation on the environment has attracted considerable attention from various researchers and scholars around the world. The innovation activities can be defined as the production of modern and best products (goods and services) or processes, a new marketing plan, or a modern organizational approach to business activities, workplace organization, or close relationships (Destek and Manga, 2021; Ibrahim and Vo, 2021). The minimum requirement for innovation is that the production process, marketing approach must be new or highly developed by the company. In this context, some empirical works have found that technological innovation improves the quality of the environment (Udeagha and Ngepah, 2021a; Udeagha and Ngepah, 2021b). However, some studies have concluded that technological innovations have added to the growing levels of environmental degradation (Atsu et al., 2021). According to a report by the Intergovernmental Panel on Climate Change (IPCC 2018), the number of anthropogenic emissions from the air could be reduced through measures to develop technological innovations and environmental policies. However, the most widely used and improved indicators are research and development activities (R&D) and patent applications. Technological innovations are an important factor in this dynamic integration that can contribute to transforming energy resources from non-renewable sources to more efficient and sustainable sources (Zameer et al., 2020; Usman and Hammar, 2021).
Numerous studies have examined how South Africa’s environmental quality is impacted by trade openness, energy intensity, foreign direct investment (FDI), and an enhanced financial system. For instance, Adebayo and Odugbesan (2021), who used ARDL-based bounds and wavelet coherence methods to examine the relationship between financial development, real growth, urbanization, and CO2 emissions in South Africa, discovered that financial development and real growth worsen environmental quality, whereas urbanization helps lower CO2 emissions. In a multivariate framework, Joshua and Bekun (2020) evaluated the long-term relationships between several factors and environmental quality and discovered feedback causation among the variables analyzed. Joshua and Bekun (2020) used the dynamic autoregressive distributed lag method to investigate the link between FDI and economic growth in carbon emissions, considering the effect of urbanization and coal consumption in South Africa. The results show that long-term and short-term CO2 emissions increase with economic expansion. A bidirectional causal relationship between urbanization and FDI is also Joshua and Bekun (2020), who hypothesized that urban growth encourages FDI in South Africa. Bekun et al. (2019) used Bayer and Hanck’s (2013) combined cointegration framework, Pesaran et al.’s (2001) bounds analysis, Kripfganz and Schneider’s (2018) critical values, and an approximate p-value to examine the relationship between EC and economic growth in South Africa from 1960 to 2020. The findings corroborate the theory of energy-led growth in South Africa by showing a one-way causal relationship between EC and economic growth.
However, the role of technological innovation in promoting environmental quality has been largely ignored, especially in South Africa. Although a few studies have examined the link between innovation and environmental quality, such as EU countries (Anser et al., 2021), the BRICS economics (Erdogan, 2021; Yang et al., 2021), Turkey (Shan et al., 2021), Egypt (Ibrahiem, 2020), US (Dinda, 2018), Brazil (Ahmad and Raza, 2020), OECD countries (Baloch et al., 2020), India (Zameer et al., 2020), China (Khan et al., 2020a), Asia-Pacific Economic Cooperation (APEC) countries (Guo et al., 2021; Usman and Hammar, 2021), G7 countries (Khan et al., 2020b), and Big Emerging Market (BEM) economies (Faisal and Idris, 2020; Destek and Manga, 2021; Ibrahim and Vo, 2021), to our knowledge, no study has examined this relationship in South Africa. Therefore, this paper aims to fill this important gap in the literature.
The following are justifications for technological innovation and climate change in this study: first, understanding the interconnectedness of sociotechnical elements in the context of climate change and innovation is made possible by insights from energy policy and the broader business literature. One finding is that, in recent years, the deployment of novel technologies, such as distributed energy storage, smart grids, and renewable energy (particularly solar photovoltaics and microinverters), has resulted in significant cost savings and climate change mitigation (IRENA, 2021). Second, in addition to energy efficiency, low-carbon and non-carbon, carbon reduction, and carbon capture and storage technologies are advancements in preventing climate change (Khalfaoui et al., 2022). More contentious technologies include “geoengineering” techniques that aim to halt or lessen global warming by purposefully altering the environment on a big scale (Sovacool, 2021). The geoengineering concepts include introducing reflecting particles into the atmosphere, burying CO2 beneath the surface, or erecting massive mirrors in space to reflect sunlight (Sovacool, 2021). Third, Figure 1 helps conceptualize these various climate routes or approaches. Basically, mitigation and geoengineering possible alternatives attempt to “prevent the uncontrollable” by effectively reducing CO2 or comparable greenhouse gases or improving the ability of natural and technical sinks to store them; adaptation strives to “handle the inevitable” by increasing adaptability and reducing vulnerability to cater for climate variability currently in progress, consistent with earlier levels of pollution and probable emission levels. Therefore, each pathway incorporates different commercial marketplaces, established actors, and underlying management logic. Fourth, mitigation is frequently seen as a public good with little-to-no financial value outside the direct selling of energy technology or services. The business model is based on fuel substitution or encouraging low-carbon alternatives to replace fossil fuel systems. The extractive and mining sectors, the hydrocarbon industry, retrofit businesses, and energy service providers are the main protagonists in this scenario. Emerging renewable energy and electric vehicle companies are also important players. When it comes to improving resilience, investing in infrastructure, or diversifying other local assets such as agriculture or buildings, climate adaptation is frequently seen as having significant local co-benefits and a market value; incumbent actors here include those already pushing large development or community benefit projects. With no established actors and the least robust commercial model, geoengineering has the prospect of upending the fundamental economic principles that underlie mitigation and adaptation. Last but not least, addressing the environmental threats brought on by climate change would be made easier with an awareness of its inconsistencies and dynamics. Adopting suitable technological innovation should be emphasized as a possible route in that direction. Most of the factors influencing the course and effects of climate change are human-related. Mitigation and control of the impacts of global warming would be exceedingly difficult without reputable instruments and systems for constant assessments and evaluation. Without management, humanity would be forced to deal with the looming cruelty that it has unintentionally caused via its own actions and inactions. This demonstrates the importance of contemporary technological innovation as a game-changing method for reducing carbon emissions and addressing climate change.
In contrast, the Technological Innovation Agency (TIA) of South Africa was established on 29 October 2010 to assist the government in promoting and accelerating technological innovation so that it may be developed and utilized to boost economic growth and the standard of living for all South Africans. The agency’s mission is to foster innovation to meet the particular circumstances in South Africa and the whole African continent because the creation of fresh, context-specific knowledge is a crucial component of innovation. The young population of South Africa, with a median age of roughly 20 years, is a crucial component of this exceptional circumstance. This distinctive cohort offers fascinating potential for innovation in the format and subject matter of postsecondary education and training. Additionally, it emphasizes the significance of a government-industry-led consolidated technical education system. The developing ties between South Africa and Brazil, China, Russia, and India, which were formalized by the recent request of South Africa to join these BRIC nations, suggest another move for rapid technological innovation and transformation of the country. Healthcare policy, how global change impacts community livelihoods, and how the financial crisis in the “developed world” affects the economy and aid are further areas where domestic technological innovation could have an influence. South Africa has addressed these issues in several ways. First, in order to “help drive South Africa’s transformation toward a knowledge-based economy, in which the production and dissemination of knowledge lead to economic benefits and enrich all fields of human endeavor,” the Department of Science and Technology came up with the “Ten-Year Innovation Plan of South Africa” in 2008. Second, the government’s broad industrialization strategy is outlined in the 2007 National Industrial Policy Framework Industrial Policy Action Plan, which also set the goal of halving unemployment and poverty by 2014 through accelerated growth of at least 6% starting in 2010. This strategy is part of South Africa’s Accelerated and Shared Growth Initiative. Lastly, the need to “accelerate economic growth and change the economy to generate decent jobs and improved standards of living” is one of the ten strategic goals included in the Medium-Term Strategic Framework of the Presidency, which was announced in July 2009. The TIA might be seen as a body that facilitates communication between the main knowledge creators and the social and business innovators. As stated in its mission statement, the organization will use various tools to close this gap. These tools include “adequately designed financial intervention strategies, the transformation of human potential, harnessing of domestic and global collaborations, and the establishment of the national dynamic capabilities.” Meanwhile, in South Africa, technological innovations and development have significantly led to a reduction in CO2 emissions in the following ways: 1) the development of end-to-end pipeline technologies that are important in reducing carbon emissions, 2) the use of energy-efficient production technologies, and 2) changes in fuel mixing and transformation of oil mixtures. Technological innovation via all these channels increases energy efficiency, which greatly reduces carbon emissions in the country. More importantly, South Africa’s significant investment in R&D and technological changes are some of the reasons why technological advances have significantly contributed to improving the country’s environmental sustainability. Moreover, as part of a major key to addressing environmental degradation, the country has adopted several policies to develop strong technologies critical to minimizing the intensity of emissions from manufacturing processes and other economic activities that involve high emissions. Due to these features, South Africa is a prime candidate for this study, which examines the asymmetric impact of technological innovation on environmental sustainability.
On the contrary, South Africa is one of the biggest developing markets and a member of the BRICS (Brazil, Russia, India, and China) alliance. The basic and secondary sectors, such as mining, manufacturing, and transportation, continue to provide a sizable contribution to the country’s gross domestic product even while its tertiary service industries (e.g., banking, real estate, and business services) have expanded in importance (Statistics South Africa, 2019). Compared to other BRICS nations, South Africa’s economy has significantly emphasized coal as a source of energy. Coal accounts for more than 80% of South Africa’s power, whereas renewable sources make up barely 7%. (African Development Bank Group, 2019). The replacement of all the coal-fired power plants in South Africa is extremely difficult, although international organizations have mandated the use of renewable energy and a decrease in coal mining. The assessment of an alternative power source also entails revising energy policy in light of the current political, social, economic, and environmental circumstances (Pathak and Shah, 2019). However, comparing South Africa’s greenhouse gas (GHG) emissions globally reveals that it has one of the most carbon-intensive economies in the world. In reality, excluding island nations and based on per capita CO2 equivalent emissions in 2010, South Africa is the most carbon-intensive developing nation that does not produce any oil (EIA, 2010). Moreover, South Africa alone accounts for 42% of all GHG emissions on the African continent, making it the continent’s top emitter. Additionally, South Africa emits more CO2 than the entirety of the Sub-Saharan African (SSA) region (EIA, 2010). The total estimated GHGs of South Africa in 2000 were 461 million tons CO2 equivalent, of which 83% were related to energy supply and consumption, 7% were from industrial operations, 8% were related to agriculture, and 2% were related to trash. Therefore, with 380,988 Gg CO2, the energy sector is by far the greatest contributor to emissions in the country, with fuel combustion accounting for 81% of the industry’s emissions and fugitive emissions from fuel accounting for the remaining 19%. The intentional promotion of investment in energy-intensive sectors of the economy, such as aluminum and other non-ferrous metal beneficiation (the so-called “mineral-energy complex,” identified by Fine and Rustomjee (1996), by the pre-democratic government prior to 1994, is a factor that has made a significant contribution to South Africa’s extremely large energy-related emission levels. The carbon intensity of a largely coal-based electricity generation base, which accounts for 90% of total emissions, is another factor responsible for high emissions in South Africa (Udeagha and Ngepah, 2022a; Udeagha and Ngepah, 2022b). South Africa ranks as the 14th greatest GHG emitter in the world, and most of its CO2 emissions are caused by a significant reliance on coal. However, a newly unveiled draft power plan suggests a considerable move away from fuel to gas and renewable energy sources. The plan calls for no new plants to be built after 2030 and the closure of four-fifths of the capacity by 2050, even though coal will keep playing a role for decades. Additionally, the nation has committed to peaking its emissions between 2020 and 2025, enabling them to level off for around 10 years before beginning to decline. The US, UK, France, Germany, and the EU offered South Africa $8.5 billion to help the nation lessen its dependence on coal during the Conference of the Parties (COP26) in Glasgow (https://www.bbc.com/news/world-africa-59135169). This is a paradigm-shifting event that can help the nation progressively shut down its coal-fired power plants and make the switch to renewable energy sources, resulting in a decrease in GHGs. Therefore, South Africa presents a compelling case for consideration in a separate study that examines how technological innovation influences pollutant emissions based on the analyses presented above.
Additionally, earlier research on the relationship between technical advancement and CO2 emissions in a global setting while including trade openness uses the same definition and presentation of trade openness. These publications have employed the trade intensity (TI) or the ratio of trade (exports plus imports) to GDP to measure trade openness. This proxy solely considers a country’s status compared to the performance of its internal commerce. This means that the true influence of trade openness on environmental quality is not adequately portrayed and that a country’s openness to international commerce is neglected. Because developing nations such as Togo, Nigeria, Ghana, Uganda, Venezuela, Zambia, and Zimbabwe are categorized as open economies due to their low GDP, the use of the TI-based proxy is detrimental to larger economies such as South Africa, Japan, China, France, the US, and Germany because they are grouped as closed economies due to their higher GDP (Squalli and Wilson, 2011). Different methodological stances and issues with model misspecification are also responsible for the inconsistent findings and lack of empirical agreement in these few works on the effect of technological innovation on CO2 emissions.
The primary objective of this study is to investigate the asymmetric effect of technological innovation on environmental quality in South Africa. Our investigation in this work is motivated by all the previously mentioned factors, including the lack of scientific consensus on the relationship between technological innovation and CO2 emissions. The significant contributions of this study are summarized below.
Firstly, by considering the aforementioned statistics, this work is crucial in examining the asymmetric impact of technological innovation on CO2 emissions in the context of South Africa using the quantile autoregressive distributed lag (QARDL) model developed by Cho et al. (2015). Again, for the aforementioned relationship, one of the most daunting challenges was to provide a dynamic concept for the association’s future development that would help policymakers with further planning. In light of contemporary realities, the current research differs from the earlier efforts in terms of the methods used to further assess the composite behavior of technological innovation-CO2 nexus. The best strategy to avoid challenges or gaps was carefully chosen after thorough scrutiny. Practically, past research emphasized basic correlation or conventional approaches to describe the connection without carefully considering the magnitude (i.e., the quantiles). In order to give a more adaptable econometric framework than the conventional ones to examine the linkages under examination, this investigation employs the QARDL approach to evaluate the long-term stability of the nexus across the quantiles. Furthermore, it was challenging to determine the main characteristics of their changes due to the chaotic and nonlinear behavior of our involved variable. Because the QARDL framework also looks at the asymmetric and nonlinear relationship between technological innovation and CO2, we used it to further understand the technological innovation-CO2 nexus.
Secondly, it is important to consider that various levels of policy instruments may have varying impacts on all levels of the target policy parameters when describing the study’s policy-level contribution. This relationship must be investigated simultaneously for both short-run and long-run settings, as the results will be used to make policy decisions. The QARDL technique has been used in this endeavor. This strategy offers a variety of advantages. 1) This method allows for examining both long-term associations and short-term dynamics throughout a range of quantiles of the constrained distribution of the target policy parameter. 2) Unlike traditional methods, the novel QARDL model offers an excellent econometric methodology by efficiently and effectively assessing the relationship’s long-term stability across quantiles. 3) In order to select the target policy parameter within its constrained distribution, it allows for locational asymmetry among the model parameters. 4) This method also helps us to identify nonlinearity in the relationship between technological advancement and pollutant emissions because the information provided by linear frameworks is insufficient to draw valid conclusions and provide accurate predictions. Consequently, this evidence suggests that the presumed linearity by earlier studies using the simple ARDL model and other cointegration frameworks is severely constrained in various economic manifestations, particularly for the connection between technological innovation and CO2 emissions. Evidence from the literature suggests the link between technological innovation and CO2 emissions could be asymmetric and nonlinear. If this happens, the policy implications will differ considerably from when this connection is linear. To the best of our knowledge, previous research on the relationship between technological innovation and CO2 emissions, particularly in the context of South Africa, has not used this approach. 5) The methodological adaptation now complements the study’s policy-level contribution from the perspective of policymaking. Diverse degrees of technological innovation are anticipated to have a range of effects on CO2 emissions. As a result, the QARDL methodology may address the issue of formulating policies, accordingly contributing to the advancement of environmental economics literature from a methodological standpoint driven by contextual factors.
Thirdly, this study makes a theoretical contribution by revalidating the EKC theory in South Africa. In terms of applications, the findings provide strategies to enhance environmental quality by implementing and conducting effective initiatives. These findings are essential for the South African government and policymakers to implement policies aimed at protecting the environment from the damaging consequences of CO2 emissions.
Lastly, in order to capture the magnitude of trade compared to global trade and the trade share in GDP, this research also makes a further contribution by using a novel measure of trade openness provided by Squalli and Wilson (2011). As a result, our study significantly differs from prior ones that mostly employed TI-based measures of trade openness by using the Squalli and Wilson proxy of trade openness.
The remainder of the paper is organized as follows: the literature review and contributions of the study section reviews the relevant literature on the nexus between technological innovation and CO2 emissions; the material and methods section outlines the material and methods, and the empirical results and their discussion section discusses the results. The Conclusions and policy implications section concludes with policy implications.
Literature review and contributions of the study
This part is divided into three subsections: the first section discusses the theoretical framework regarding CO2 emissions and environmental regulations, the second section explores and provides empirical research on the connection between technological innovation and environmental quality, and the last section outlines the gaps in the literature and highlights how the current study adds to the existing knowledge on this topic.
Theoretical framework of CO2 emissions and environmental regulations
Researchers have been interested in the trending topics of environmental regulation and CO2 emissions. The green paradox and forced emission reduction are two dominant positions on these topics (Yin et al., 2022). The green paradox contends that CO2 emissions cannot be successfully reduced by environmental regulation. Energy exploitation quickens as fossil energy producers anticipate that the green legislation may hurt their earning potential. A rise in supply lowers energy prices while raising energy demand. Consequently, environmental control measures have the reverse impact, leading to an increase in CO2 emissions and pollution (Ngo, 2022). Forced emission reduction is the other viewpoint. This perspective maintains that the fundamental tenet of the green paradox is unrealistic.
The overall amount of fossil fuel reserves is finite and has a finite shelf life. Prices and demand for energy could also not be related, and both might rise simultaneously. Cost increases lead to a decrease in pollution and CO2 emissions (Hassan et al., 2022). How would environmental regulation affect CO2 emissions as a result? Will it be favorable or unfavorable? We consider the direct and indirect consequences in order to respond to these two queries.
Environmental regulation has two implications for CO2 emissions. On the one hand, CO2 emission is directly impacted by environmental regulation (Figure 2). Environmental regulation is a crucial component of social regulation, which suggests that in order to achieve sustainable economic growth and the atmosphere, the government regulates the manufacturing and operating processes of industry players through governmental actions, carbon pollution authorizations, regulatory fines, and the collection of emission taxes. Although there are many different environmental regulatory mechanisms, they may be loosely grouped into two categories: command control and market incentive (Chen et al., 2022). All parties involved must strictly abide by the required instructions under the command-control environmental regulation, where the state agency establishes the goals and specifications of environmental management in the form of laws or regulations and guidelines (Liu et al., 2022). Command-control environmental regulation is more stringently enforced than the market incentive regulatory style. Carbon emitters can accept the different pollutants’ emissions requirements to avoid the environmental protection agency’s stiff penalties. By using market-oriented measures such as sanitation surcharges, carbon pollution trading costs, and environmental taxes, the state, through market incentive environmental regulation, supports different market entities to proactively maintain a sustainable environment because of the benefits this has for society as a whole (Xu and Xu, 2022). In general, market-based environmental regulation increases ecological integrity indirectly by employing financial means to increase polluters’ financial costs (Wang L. et al., 2022c). As a result, the state creates environmental standards focused on taxing fossil fuel consumption and manufacturing. Consequently, the demand for fossil fuels will be reduced because it will become more expensive to produce energy using fossil fuels. Commercial organizations will conduct research to create green technology and raise their levels of technological innovation as a result. The state also has the power to influence environmental regulation. The state uses administrative measures to limit pollution discharges from industries, such as requiring some high-polluting corporations to shut down and some businesses to adopt low-carbon technologies to effectively reduce CO2 emissions. These measures are enforced through statutes and rules that maintain industry requirements. The “green paradox” argument, which suggests that stringent environmental regulations will hasten the extraction and sale of fossil fuels and raise CO2 emissions, may also be considered simultaneously (Gu et al., 2022).
Contrarily, environmental regulation affects CO2 emissions indirectly through four conductive channels, including FDI, technological innovation, industrial structure, and energy structure (Yin et al., 2022). Environmental regulations have increased the regulatory limit for polluting enterprises and limited their development, as shown in Figure 3 from an examination of the indirect functional route of industrial structure. The cost of conducting energy-intensive enterprises also rises as a result, which encourages upgrading the industrial structure and further cuts CO2 emissions. An examination of the energy utilization structure reveals that environmental regulation will cause businesses to use less fossil fuel and emit less CO2. Environmental regulation’s impact on energy structure, however, may have a contradictory result (Wang et al., 2022c). According to some researchers, the adoption of environmental regulations will expedite the expansion and deployment of fossil fuels as a source of energy. The “Porter hypothesis” impact and the “following cost” influence are two consequences of environmental regulation on technological innovation, as shown by research. Moderate environmental regulation can encourage companies to employ technological innovation, which will help reduce CO2 emissions. However, excessively stringent environmental standards would drive up the cost of pollution and limit the capacity for technical R&D (Xie et al., 2022). Therefore, it is difficult to lower CO2 emissions. A host nation will benefit from FDI’s sophisticated managerial and technological capabilities, which will help reduce CO2 emissions. However, if some nations accept enterprises that produce much pollution by reducing the environmental regulatory requirement, CO2 emissions will not be reduced. However, the technological spillover effect of FDI and the absorption of cutting-edge technology and knowledge would not be possible if the environmental standards of a host country were significantly tighter than those in other nations (Yirong, 2022). The indirect impact of environmental regulations on CO2 emissions is the primary goal of this research.
Review of previous literature
Several studies have examined the role technological innovation plays in enhancing environmental quality. However, across a variety of methodological frameworks and nations investigated, the results are often ambiguous and conflicting. Although some studies found that environmental quality is improved by technological innovation through various routes (Rafique et al., 2022), several other works argued that technological advancement exacerbates the state of the environment (Atsu et al., 2021).
For an illustrative sample of 76 Belt and Road economies, Rafique et al. (2022) evaluated the empirical interactions between the consumption of renewable energy, FDI, medium and high-tech industries, economic complexity, human capital, power distance, uncertainty avoidance, and masculinity versus femininity. A comprehensive framework for econometric testing was created using a series that covered the years 1996–2019 and included the generalized method of moments and the technique of moment quantile regression. Associated findings supported the authors’ initial hypotheses that medium and high-tech industries, as opposed to FDI, caused the diffusion of low-carbon energy across sectors. Changes in human capital have a detrimental impact on the implementation of renewable energy. The authors included several policy recommendations and a methodological comment to incorporate those findings into future energy planning. Likewise, Lin and Ma. (2022) investigated the influence of the urban innovation environment on the effect of technological advances on CO2 emissions using data on 264 prefecture-level Chinese cities from 2006 to 2017. The empirical findings showed that different types of cities are affected differently by technological advancements. Although the impact is minimal in Chinese cities prior to 2010, technological advancements can help reduce CO2 emissions after 2010. Second, through improving industrial structure, technical advancements can indirectly lower CO2 emissions. Thirdly, government spending cannot considerably affect the marginal impact of technical advances when the urban innovation environment is considered. Similarly, Obobisa et al. (2022), who recognized institutional excellence and technical innovation as efficient ways to decrease carbon emissions and advance sustainable development, examined how each contributed to emissions reductions in 25 African nations between 2000 and 2018. The use of renewable energy and technical progress, according to the authors, massively reduces CO2 emissions. On the contrary, CO2 emissions are adversely affected by institutional quality, economic expansion, and the use of fossil fuels as an energy source. The authors suggested that, in order to meet their goals for sustainable development, African nations expand their investment in technical innovation and renewable energy initiatives. Moreover, the analysis of the relationship between technical advancement, renewable energy, and CO2 emissions from 1990 to 2018 in China by Kuang et al. (2022) using panel data demonstrated that these variables had a long-term, significant negative influence on CO2 emissions. Additionally, there is no evidence of a short-term relationship between technical innovation and economic development. The authors conclude that putting technological innovation to use has positive externalities. During the 1990–2018 data period, Rahman et al. (2022) investigated the role of contributing factors for CO2 emissions reduction in the 22 most industrialized countries worldwide. The authors found that reducing CO2 emissions is aided by export quality and renewable energy. The positive stimuli of technological innovation, as measured by R&D investment and export quality index, reduce these emissions in contradiction to the negative shocks or counter incentives of these variables, which increase CO2 emissions. Additionally, Habiba et al. (2022) examined the effects of financial development, technological breakthroughs, and the use of renewable energy on carbon emissions for the top twelve emitters using data from 1991 to 2018. In the future, technological developments and the use of renewable energy will be the primary factors in lowering CO2 emissions, whereas the usage of non-renewable energy will continuously drop. Based on their findings, the authors suggested actions to reduce CO2 emissions in order to achieve sustainable development. Vitenu-Sackey and Acheampong (2022) examined the impact of economic policy uncertainty (EPU) and technological development on CO2 emissions in a panel of 18 industrialized countries from 2005 to 2018 using second-generation time-series panel data techniques. The authors used three trustworthy long-run estimators to handle heterogeneity, endogeneity, and simultaneity in the panels: two-stage least squares, panel generalized method of moments (GMM), and generalized least squares (GLS). They discovered that economic growth had a significant and favorable influence on CO2 emissions, but this benefit peaked at a certain rate of growth and then decreased, demonstrating that the sample had an inverted U-shaped EKC relationship. Second, the impact of EPU on CO2 emissions varies by country. For example, high levels of EPU have little impact in low-pollution countries while having a considerable impact in high-pollution countries. Thirdly, R&D, FDI, urbanization, and the utilization of renewable energy sources all have varied effects on CO2 emissions (RE). The authors stressed that there is a heterogeneous relationship between carbon emissions and economic indices, even in advanced economies. This relationship is known as the pollution haven hypothesis (PHH), which is true in high-pollution nations, whereas the pollution halo effect is true for low-pollution ones. This study contends that a one-size-fits-all approach to emission reduction is not the best course of action because not every country’s rate of urbanization, FDI inflows, R&D spending, and use of renewable energy directly influence CO2 emissions in the face of unpredictable economic policies.
Furthermore, Adebayo et al. (2022) used cutting-edge Morlet wavelet analysis to provide a new understanding of the dynamic relationship between CO2 emission and economic development, the use of renewable energy, trade openness, and technical innovation in the Portuguese economy. The study used a dataset between 1980 and 2019 to apply continuous wavelet transform, wavelet correlation, multiple and partial wavelet coherence, and frequency domain causality (FDC) analysis to the variables under study. The linkage between the markers advances with time and frequency, according to the authors. In addition, they discovered considerable lead and lag linkages and wavelet coherence in the frequency domain but competing interactions between the variables were discovered in the time domain. The wavelet analysis supports the economic position that the use of renewable energy reduces CO2 emissions whereas trade openness, technical advancement, and economic expansion increase CO2 emissions. The findings suggested that the usage of renewable energy will reduce CO2 in Portugal over the long term. Portugal’s government ought to encourage investment in renewable energy sources, enact limiting legislation, and promote energy innovation. Chhabra et al. (2022) investigated how trade openness and technical advancement helped middle-income nations reduce their CO2 emissions to improve the quality of the environment. For a sample of 23 middle-income nations from 1994 to 2018, the generalized method of moments (GMM) approach and the Dumitrescu–Hurlin causality test were used to estimate the long-run relationship between variables and investigate causality, respectively. The inverted u-shape association between innovation and CO2 emissions was disproved by their research. In terms of commerce, it was discovered that lower middle-income countries experienced environmental deterioration at a more pronounced rate than upper middle-income nations. In contrast, the data also confirmed the existence of the EKC theory for both nation groups; however, the decline in the EKC curve is negligible for low- and middle-income countries suggesting that in order to minimize the steadily growing CO2 emissions, poor and medium income nations must focus on a higher degree of green innovation than they have in the past. The authors proposed setting a pollution level standard for the industrial and trading sectors, which produce the most polluted waste, and encouraging economic growth through knowledge spillovers. Additionally, according to Li et al. (2022), technological innovation unquestionably contributes significantly to creating job possibilities, enhancing green economic activity, and boosting environmental sustainability. The authors used nonlinear autoregressive distributed lag (NARDL) to examine the impact of technical advancement and energy efficiency on CO2 emissions in China from 1991 to 2019. Their studies demonstrated how China’s CO2 emissions might be decreased through technical innovation and energy efficiency. Innovation in technology and energy efficiency are significant nonlinear factors of CO2 emissions. Energy efficiency and technology advancements help reduce CO2 emissions, but their decline has a long-term negative impact on CO2 emissions in China.
The high technology (high-tech) industry in China has advanced to a critical strategic position in the Chinese economic objectives, according to Wang et al. (2022a). This posture has led to the rise of FDI and technical innovation as powerful cornerstones of the high-tech sector. Although it is still up for debate, there are rising worries about the industry’s carbon emissions. Wang et al. (2022b) examined the impact of FDI and technical advancement on carbon emissions in the high-tech industry from 28 Chinese provinces in this context. China’s province statistics from 2000 to 2018 were used in the study. The authors employed quantile regression to estimate long-run correlations among research variables in addition to looking at unit root characteristics, structural breakdowns, and cointegration. The results showed that FDI has a negative effect on carbon emissions. The first three quantiles of technological innovation are favorably impacted, whereas the next six quantiles are negatively impacted. According to the authors, FDI and technical advancement have altered the energy intensity in the high-tech sector, which affects how much CO2 is emitted over time. Their analysis suggested that policymakers should focus on the diverse effects of FDI and technology-led emissions at different quantiles during the process of CO2 emission reduction after controlling the effects of urbanization, energy intensity, and economic growth. Based on data from 1990 to 2019, Abid et al. (2022) investigated the effects of technical advancement, financial growth, FDI, energy usage, and urbanization on carbon emissions in G8 member nations. Within the panel nations, their findings showed a substantial cross-sectional reliance. FDI, financial development, and technical innovation in G8 nations have all been found to have a statistically significant long-run and adverse correlation with CO2 according to the FMLOS estimator. Economic growth, financial development, urbanization, trade openness, CO2 emissions, and energy usage have been found to have long-term bidirectional causal relationships. In contrast, there is a one-way causal relationship between carbon emissions and FDI. The authors suggested that the current requirement for the growth of industries, technical innovation, and financial development for the G8 nations is a quality FDI. Additionally, urbanization contributes significantly to environmental deterioration, necessitating the need for stronger regulations in these nations.
Moreover, Anser et al. (2021), who investigated the effect of innovation on environmental quality in EU countries using the panel fixed effect and panel quantile regression over the period 2000–2017 found that innovation has contributed immensely to reducing CO2 emissions. This evidence is supported by Yang et al. (2021) and Erdogan (2021) in the case of BRICS economies. Moreover, Shan et al. (2021) reached a similar conclusion for Turkey using the ARDL framework over the period 1990–2018. Similarly, Guo et al. (2021), who examined the role of technological innovation in improving environmental quality in Asian countries found that innovation is good for the environment and has contributed tremendously to reducing CO2 emissions in those countries under review.
In contrast, Dauda et al. (2021), who assessed the effect of technological innovation on environmental quality in SSA countries over the period 1990–2018 concluded that innovation increases CO2 emissions. Using the feasible generalized least square (FGLS) and augmented mean group (AMG) frameworks over the period 1990–2017, Usman and Hammar (2021) found evidence that innovation escalates environmental dilapidation for APEC countries.
Therefore, Table 1 presents a summary of selected literature on the nexus between technological innovation and CO2 emissions to reflect more comparisons against different regions.
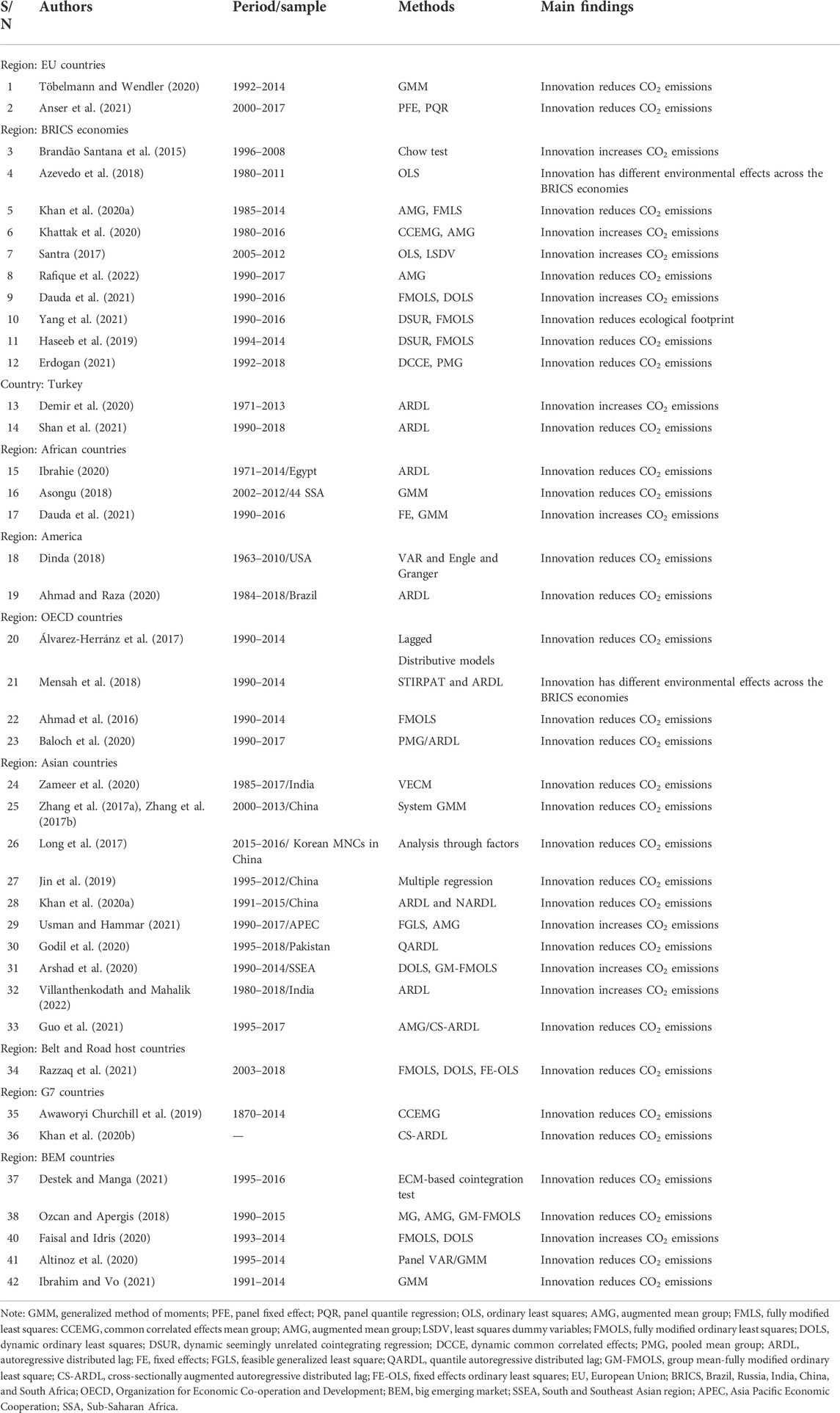
TABLE 1. A summary of the selected articles on the innovation–CO2 emissions nexus based on different regions.
Literature gap and contributions of the study
Based on the literature review, the environmental impact of technological innovation is controversial and has substantially generated more heat than light. Because of its complex nature, we do not precisely know the future effect of technological innovation, and our decision today affects the direction of sustainable development. Therefore, revisiting the role technological innovation plays in fostering environmental quality, especially in the context of South Africa has been an area that requires further analysis. This is because the constant application of technological innovation determines if it could lead to improvement in environmental quality, thus enhancing better lives with good environmental quality in South Africa.
In light of this, we make three contributions to the literature on how technological advancement affects CO2 emissions. 1) Several studies have looked into how South Africa’s CO2 emissions are impacted by financial development, FDI, trade openness, and energy use. The role of technological innovation in promoting environmental quality in the context of South Africa, however, is less thoroughly examined in empirical research. As a result, this research aims to close this significant vacuum in the body of knowledge on South Africa. 2) The simple ARDL approach suggested by Pesaran et al. (2001) and other cointegration frameworks, which can only estimate and explore the long- and short-run relationships between the variables under review, have been widely used in studies that investigated the relationship between technological innovation and CO2 emissions in the global context. The newly designed QARDL model presented by Cho et al. (2015), which circumvents the shortcomings of the conventional ARDL technique, is used in this work instead. In contrast to traditional methods, the innovative QARDL model offers a flexible econometric framework by effectively and efficiently assessing the relationship’s long-term stability across quantiles. To the best of our knowledge, previous research on the relationship between technological innovation and CO2 emissions, particularly in the context of South Africa, has not used this approach. 3) There have been criticisms of the definition and measurement of trade openness in a few empirical studies that examined the impact of technical innovation on CO2 emissions while accounting for trade openness. In order to capture the magnitude of trade compared to global trade and the trade share in GDP, this research also makes a further contribution by using a novel measure of trade openness provided by Squalli and Wilson. (2011). As a result, our study significantly differs from prior ones that mostly employed conventional TI measures of trade openness by using the Squalli and Wilson proxy of trade openness.
Material and methods
This study examines the relationship between technological innovation and CO2 emissions in South Africa from 1960 to 2020 using the innovative QARDL framework developed by Cho et al. (2015), which circumvents the shortcomings of the straightforward ARDL method. In contrast to traditional methods, the unique QARDL model offers a flexible econometric framework by effectively and efficiently assessing the relationship’s long-term stability across quantiles. To the best of our knowledge, previous research on the relationship between technological innovation and CO2 emissions, particularly in the context of South Africa, has not used this approach. It is crucial to perform a stationarity test on the variables to determine their order of integration before using the innovative QARDL model. As a result, we use the standard Dickey–Fuller GLS (DF-GLS), Phillips–Perron (PP), Augmented Dickey–Fuller (ADF), and Kwiatkowski–Phillips–Schmidt–Shin (KPSS) unit root tests. Because structural breaks are persistent and have an impact on many macroeconomic variables, including CO2 emissions and technological innovation, the Narayan and Popp structural break unit root test is utilized.
Functional form
This study revisits the relationship between technological innovation and CO2 emissions in South Africa using the standard EKC hypothesis framework, which is a strong empirical technique that has been widely employed in past research. The EKC theory argues that economic expansion significantly worsens environmental quality because, throughout the earlier stages of society’s development, reaching greater income levels received more attention than minimizing environmental deterioration. As a result, aggressive efforts were made to achieve faster economic development at the price of reducing carbon emissions, which ineluctably led to the deterioration of the environmental state.
This evidence logically justifies the reason why the scale effect, a stand-in for economic expansion, and environmental quality have a positive association. People grew increasingly ecologically sensitive as society developed, particularly during the advanced industrial era, and governments passed environmental regulations intended to boost environmental quality. As a result, throughout this stage of development, as income rose, the environment got better due to people’s propensity for a clean environment and the implementation of stricter environmental norms. The reasoning behind the negative association between the technology impact (square of economic growth) and environmental quality is thus intuitively explained by this argument. The typical EKC hypothesis is thus stated in accordance with Udeagha and Breitenbach (2021), Udeagha and Ngepah. (2019), Cole and Elliott (2003), and Ling et al. (2015) as follows:
where CO2 represents CO2 emissions, an environmental quality measure; SE denotes scale effect, a proxy for economic growth; and TE represents technique effect, which captures the square of economic growth. Log-linearizing Eq. 1 yields the following:
As income rises, the scale effect (economic expansion) causes environmental quality to decline. In contrast, the method effect—following the adoption of environmental regulations and people’s propensity for a clean environment—improves environmental quality (Cole and Elliott, 2003; Ling et al., 2015). With this context, the conceptual predictions demand that
where
Measuring trade openness
Following Squalli and Wilson (2011), the composite trade intensity (CTI) is employed in this study as a measure of trade openness to adequately account for trade’s contribution to GDP and its magnitude in relation to global trade. We can successfully overcome the drawbacks of the conventional TI extensively employed in past research by using this method of measuring trade openness. More crucially, the innovative CTI offers more significant data about a nation’s trade contribution to the world economy. Additionally, because it includes both aspects of a nation’s relationships with the rest of the world, it reflects the reality of trade outcomes. The CTI is shown as follows:
where South Africa is represented by i and its trading partners are denoted by j. The first part of Eq. 4 signifies the global trade share, and the second part represents the South African trade share.
Variables and data sources
The data used in this study are annual times series data from 1960 to 2020. The dependent variable is CO2 emissions, which act as a stand-in for environmental quality. To confirm the existence of the EKC hypothesis, economic growth as measured by scale effect and the square of economic growth as measured by technique effect are utilized. Gross domestic investment in R&D is used as a proxy for technological innovation. Following the literature, the additional factors that were considered were as follows: EC, FDI, trade openness (OPEN), and industrial value-added to GDP (IGDP). Therefore, the variable definition and data sources are summarized in Table 2.
Narayan and Popp’s structural break unit root test
It is crucial to perform a stationarity test on the variables under consideration in order to determine their order of integration before using the innovative QARDL model. As a result, this study utilizes the unit root tests DF-GLS, PP, ADF, and KPSS. As empirical data demonstrate that structural breaks are persistent in the sense that numerous macroeconomic variables, such as CO2 emissions and technological innovation, are impacted by structural breaks, the Narayan and Popp structural break unit root test is further applied.
Quantile autoregressive distributed lag framework
The simple ARDL approach put forth by Pesaran et al. (2001) and other cointegration frameworks, which can only estimate and explore the short- and long-run relationships between the variables, have been widely used in previous studies that examined the impact of technological innovation on CO2 emissions. The recently designed QARDL model established by Cho et al. (2015), which circumvents the shortcomings of the conventional ARDL technique, is used in this work instead. In contrast to traditional methods, the innovative QARDL model offers a flexible econometric framework by effectively and efficiently assessing the relationship’s long-term stability across quantiles. This method also helps us identify the nonlinearity in the relationship between technological advancement and CO2 emissions because the information provided by linear frameworks is insufficient to draw valid conclusions and provide accurate predictions. Consequently, this evidence suggests that the assumed linear relationship by earlier studies using the traditional ARDL model and other cointegration frameworks is severely constrained in a wide range of economic phenomena, especially for the relationship between technological innovation and CO2 emissions. According to the studies reviewed above, there is cause for concern that the link between technological advancement and CO2 emissions may be nonlinear and asymmetric. If this were to occur, the policy implications would be considerably different than when this connection is linear. To the best of our knowledge, previous research on the relationship between technological innovation and CO2 emissions, particularly in the context of South Africa, has not used this approach. The conventional linear ARDL bounds testing approach, following Pesaran et al. (2001), is presented as follows:
where
Following the above-mentioned linear ARDL framework, the novel QARDL model is presented as follows:
where
Following Cho et al. (2015), the dynamic quantile error correction model of QARDL is presented as follows:
By using the
Frequency domain causality test
Lastly, this study explores the causal connections between the variables under investigation using the FDC technique, a reliable testing procedure recommended by Breitung and Candelon (2006). FDC makes it possible to predict the response variable at a given time-frequency, which is virtually impossible with the traditional Granger causality approach. It also enables capturing permanent causality for medium-, short-, and long-term relationships among the variables being studied. In this study, the robustness of the test is also checked.
Empirical results and their discussion
Summary statistics
Before analyzing the findings, the summary statistics of the variables employed in this work are examined and analyzed. The summary of information in Table 3 shows that the CO2 emissions average value is 0.264. The square of GDP per capita, the technique effect, has an average mean that is 60.316 times bigger than other variables. FDI, which has 13.203, comes next. Table 2 characterizes the summary statistics and depicts the peak using kurtosis, whereas the Jarque–Bera test statistic is utilized to determine if our data series is normal. The table demonstrates that although the method impact has a negative tendency, scale effect, trade openness, energy consumption, FDI, industrial value-added, and technical innovation all have positive trends. Technique effect (TE) has the largest variation of all of the variables, indicating a significant degree of instability. Because there is less variation in CO2 emissions than there is in method effect, CO2 emissions are far steadier. Additionally, there are far higher variances in technical innovation (TECH), scale impact, and trade openness (OPEN). The Jarque–Bera statistics also demonstrates the non-normal distribution of our data series. Our evidence confirms the nonlinearity of the variables in our dataset, and the choice of the QARDL model in this study is supported by this evidence.
Order of integration of the respective variables
All variables that are nonstationary at the level become stationary at I(1) after the first differencing, according to the findings of Table 4' from the DF-GLS, PP, ADF, and KPSS tests. This suggests that none of the series under consideration are I(2) and that all are either I(1) or I(0). The conventional unit root tests mentioned above do not consider structural breaks. Therefore, a testing technique that may consider two structural breakdowns in the variables is used in this work. In the right-hand panel of Table 3, the outcomes of the Narayan and Popp unit root test with two structural breaks are also presented. The empirical data demonstrate that the variables are stationary in the presence of structural breaks. All data series are therefore integrated into order one.
Quantile co-integration test results
To support the cointegration connection between the variables under consideration, this research applies the quantile co-integration technique suggested by Xiao (2009). The fallouts of the quantile co-integration for the variables being studied are shown in Table 5. These findings show the supremum ordinary measures of β and γ coefficients and CV10, CV5, and CV1 of a measurably significant level at 10%, 5%, and 1%, respectively. We reject the null hypothesis because the supremum measures of β and γ values are larger than all the CVs at various significance levels of 10%, 5%, and 1%. Therefore, cointegration between the variables under discussion is supported by our empirical results.
Quantile autoregressive distributed lag model results
Table 6 presents the QARDL model’s findings. The speed of adjustment is captured by the parameter
From quantiles 0.30 to 0.95, the long-run predicted coefficient on technological innovation is statistically significant and negative. Our empirical research demonstrates that, over the long term, a 1% increase in technological innovation results in a 0.31% reduction in CO2 emissions (from the 0.30th quantile). Technology advancements reduce carbon emissions in South Africa by promoting efficient energy use and producing renewable energy sources at lower costs. Following are some ways that technological innovation benefits South Africa’s environment: implementing end-of-pipe technology is essential for reducing carbon emissions, along with using energy-efficient industrial techniques and altering the fuel mix. Through these routes, technological advancements boost energy efficiency, which significantly improves environmental quality. Technology advancements have greatly improved the environmental quality of South Africa, in part due to the country’s massive spending on R&D and advances in technology. Additionally, South Africa has implemented several policies aimed at developing strong technologies necessary to minimize the intensity of emissions from production processes and other economic activities linked to high levels of emissions as part of the major key to mitigating the rising levels of carbon emissions. According to Sohag et al. (2015), technical innovation opens up a door for lower energy use, which in turn promotes energy efficiency and significantly lowers carbon emissions. Our conclusion is backed by earlier research, including that of Ahmed et al. (2016) and Yii and Greetha (2017).
From the 0.20th to the 0.80th quantiles, the estimated coefficient over the long run on trade openness (InOPEN) is found to be statistically significant and positive, indicating that a 1% increase in trade openness results in a 0.121% increase in CO2 emissions (from the 0.20th quantile). The conclusion is backed by Baek et al. (2009), who argued that trade harms developing nations’ environments and has significantly worsened them. Our empirical data reveal that long-term access to the global market for commodities does not benefit South Africa’s environmental quality. Contrary to the short-term findings, which indicate that trade openness may significantly enhance the nation’s environmental quality from the 0.50th to the 0.95th quantiles, unquestionably, the long-term negative impact of openness on South Africa’s environmental situation reinforces the opposition to economic liberalization. The majority of a country’s exports are made up of certain types of goods, which is one of the potential explanations for why trade openness harms the environment. Because South Africa has a comparative advantage in the export and production of goods that require a lot of natural resources, such as fuelwood, arsenate, canister, base metals, nickel-cobalt mineral deposits, trace elements, molybdenum, valuable minerals, natural gas, chromite, mineral ores, dimes, coal, chromium, gemstones, palladium, and precious metals, an increase in demand for these goods will undoubtedly worsen the country’s environmental situation. This is because constant harvesting of them damages the ecology. The empirical results are supported by Shahbaz et al. (2013a), Shahbaz et al. (2013c), Shahbaz et al. (2014a), Shahbaz et al. (2014b), Ngepah and Udeagha (2018), and Ngepah and Udeagha (2019). However, our empirical findings are different from Destek et al. (2021), who used annual frequency data from 1970 to 2016 and continuously updated fully modified and continuously updated bias-corrected panel estimation techniques that control for cross-section dependence among sampled countries, finding that trade openness reduces both ecological footprint and CO2 emissions by 0.34–0.55% across the top five biomass energy-consuming countries: Brazil, China, Germany, India, and the US.
In the case of energy consumption (InEC), the estimated coefficients for the short run (from the 0.05th to 0.70th quantiles) and long run (from the 0.50th to 0.95th quantiles) are statistically significant and positive, indicating that the increasing level of CO2 emissions in South Africa is significantly exacerbated by the country’s energy use. Energy utilization is essential for sustaining output and it promotes economic growth. An increase in energy use results in a rise in CO2 emissions because producing things requires a significant amount of energy. Ling et al. (2015) and Saboori et al. (2012), who made similar observations using data from Malaysia, bolster our empirical findings.
From the 0.05th to 0.60th quantiles, the short-run estimated coefficient on foreign direct investment (InFDI) is statistically significant and positive. The calculated long-run coefficient on foreign direct investment, which ranges from quantiles 0.05 to 0.40, is also discovered to be substantial and favorable. Our findings thus imply that an increase in FDI causes environmental deterioration in South Africa. In the case of MENA nations, Abdouli and Hammami (2017) come to the same conclusion that FDI has significantly increased CO2 emissions and that there is evidence of the pollution haven theory. Similarly, Omri et al. (2014) found that in the case of 54 nations, the level of pollution has increased due to the influx of FDI. However, Destek and Okumus (2019), who examined the pollution haven hypothesis’ applicability for the years 1982–2013 in ten newly industrialized nations, observed that the significant signs of the coefficients of FDI and the square of FDI are opposite. As a result, the validity of both the pollution haven hypothesis and the pollution halo hypothesis is thoroughly debunked, and the U-shaped relationship between FDI and ecological footprint is maintained. According to the authors’ empirical findings, environmental deterioration decreases up to a certain point with increased FDI, but after that point, environmental degradation grows with increased FDI. In the case of individual country results, the findings revealed that in Brazil, China, Malaysia, Thailand, and Turkey, the sign of the coefficient of FDI is negative and the sign of the coefficient of the square of FDI is positive. Therefore, it is discovered in these nations that there is a U-shaped link between FDI and ecological footprint. Similarly, in the case of BRICS and Next Eleven countries, the Shahbaz et al. (2018) study of the key interactions between foreign capital, financial development, and environmental deterioration over the period 1992–2016 found that economic expansion promotes clean EC, whereas financial development decreases it. On the contrary, it does not seem that foreign capital inflows have a statistically significant impact on renewable energy. The authors made the case that while financial development, economic expansion, and foreign capital inflows all lead to an increase in CO2 emissions, the BRICS nations’ use of sustainable energy prevents environmental damage by reducing carbon emissions. Empirical findings further showed that economic growth and foreign investment had a positive impact on the use of clean energy in the Next Eleven nations. However, CO2 emissions in the Next Eleven nations rose as a result of economic and financial progress.
The long-run estimated coefficient on industrial value-added (InIGDP) is statistically significant and positive from quantiles 0.40 to 0.95 showing that industrial sector growth significantly contributes to the deterioration of South Africa’s environment in the long run. Our findings are supported by the results of Udeagha and Ngepah (2021) and Sohag et al. (2017). However, Destek (2021), who investigated how structural changes affected environmental deterioration in Turkey from 1970 to 2017, revealed that deindustrialization reduces carbon emissions but has little-to-no effect on ecological footprint. The authors also found that although industrialization and reindustrialization result in a decline in environmental quality, reindustrialization can be less environmentally detrimental due to technological developments.
This study further applies the structural stability evaluation of the model to validate its robustness and its dynamic stability. To this end, this study uses the Wald test to examine the constancy (linearity) of parameters approximated as presented in Table 7. Our results show that the null hypothesis for parameter constancy for the speed of adjustment parameter is rejected at a 1% significance level. Moreover, our empirical results reject the null hypothesis of linearity across different tails of every quantile for long-term parameters InSE, InTE, InTECH, InEC, InFDI, and InOPEN, except for InIGDP. As a result, this evidence validates the presence of asymmetric long-run relationships between these variables and InCO2 in South Africa, and the study concludes that long-term parameters are dynamic in various quantiles. Additionally, Table 7 shows that the null of linearity for the short-term cumulative impact of previous levels of InSE, InTE, InTECH, InEC, and InOPEN is rejected by the Wald test, except for InFDI and InIGDP. This evidence further suggests that there are asymmetric short-run relationships between these variables and InCO2 in South Africa.
The FDC test developed by Breitung and Candelon (2006) is also used in this study to investigate the relationship between South Africa’s InSE, InTE, InTECH, InEC, InFDI, InOPEN, InIGDP, and InCO2. According to Table 8, for frequencies wi = 0.05, wi = 1.50 and wi = 2.50, InSE, InTE, InTECH, InEC, InFDI, InOPEN, and InIGDP Granger-cause InCO2 in the short, medium, and long term. This suggests that short-, medium-, and long-term CO2 emissions in South Africa are considerably impacted by InSE, InTE, InTECH, InEC, InFDI, InOPEN, and InIGDP. Our empirical data agree with those of Udeagha and Ngepah (2019).
Conclusion and policy implications
This study investigated the asymmetric impact of technological innovation on CO2 emissions in South Africa between 1960 and 2020 using the recently created QARDL framework by Cho et al. (2015). This framework allows us to evaluate the long-term stability across the quantiles and account for the distributional asymmetry based on the position of CO2 emissions within its own distribution, making it possible to perform an econometric analysis that is more adaptable than that provided by traditional frameworks. Breitung and Candelon’s (2006) robust testing technique, the FDC approach, which enables us to capture permanent causation for the medium, short, and long term among variables under consideration, was utilized to assess robustness. By using an innovative measure of trade openness proposed by Squalli and Wilson (2011) that accounts for trade share in GDP and the magnitude of trade relative to global trade for South Africa, this study made an additional contribution to the empirical literature. We employed the unit root tests: KPSS, ADF, PP, and DF-GLS. Additionally, the Narayan and Popp structural break unit root test was employed because empirical data demonstrate that the structural breakdowns are persistent in the sense that they have an impact on several macroeconomic variables, including CO2 emissions and technological innovation. The data series was integrated into order one, or I(1), according to our empirical evidence from all the tests, and there was no indication of any I(2). In order to determine the ideal lag length, SBIC was used. Our empirical findings for South Africa showed that while an increase in method impact is ecologically beneficial, an increase in scale effect worsens the environmental state. As a result, this evidence supported the EKC theory for South Africa. Environmental quality is harmed by trade openness, FDI, industry value addition, and energy use. Our results confirmed the presence of asymmetric long-run relationships between the scale effect, technique effect, technological innovation, energy consumption, FDI, trade openness, and CO2 emissions. The FDC results also showed that in the medium, long, and short terms, the scale effect, technique effect, technological innovation, energy consumption, FDI, trade openness, and industrial value-added Granger cause CO2 emissions, indicating the significance of these variables in influencing CO2 emissions in South Africa.
Moreover, with regard to the relationship between technological innovation and CO2 emissions, our empirical results showed that in the short and long term, an upsurge in technological innovation improves environmental quality by reducing CO2 emissions in South Africa. Our findings are consistent with the results of Sohag et al. (2015), who have shown that technological innovations create a mechanism that helps reduce energy consumption, thus allowing energy efficiency to significantly improve environmental quality. In South Africa, technological innovations and development have significantly led to a reduction in CO2 emissions in the following ways: 1) the development of end-to-end pipeline technologies that are important in reducing carbon emissions, 2) the use of energy-efficient production technologies, and 3) changes in fuel mixing and transformation of oil mixtures. Technological innovation via all these channels increases energy efficiency, which greatly reduces carbon emissions in the country. More importantly, South Africa’s significant investment in R&D and technological change are some of the reasons why technological advances have made a significant contribution to improving the country’s environment. Moreover, as part of a major key to addressing environmental degradation, the country has adopted several policies to develop strong technologies that are critical to minimizing the intensity of emissions from manufacturing processes and other economic activities that involve high emissions.
On the basis of our findings, the following recommendations for policy are made: first, in order to improve environmental quality, South Africa should support economic policies that encourage innovation and investment in energy-efficient machinery and appliances and capital investment in energy-efficient technologies and the use of hydroelectricity, solar, water, wind, and other clean energy sources (Udeagha and Breitenbach, 2022). South Africa should take steps to limit energy usage and promote renewable energy sources, which would lessen the intensity of fossil fuel-based energy consumption, in order to fully support economic growth.
Second, the government should strengthen its regulations in order to improve the environment. The long-term detrimental impact of trade openness on the nation’s environment, however, does not justify ongoing actions to restrict the borders because of certain benefits to South Africa’s economy. Instead, proper measures should be made to ensure that international commerce significantly lowers South Africa’s growing carbon emissions. In this regard, South Africa’s policymakers should step up efforts to adopt cutting-edge, environmentally friendly, and non-polluting technologies that could help the country make the transition from non-renewable to sustainable, consume less carbon-intensive energy sources, and guarantee the competence of its manufacturing processes. Meanwhile, alternative energy sources such as solar power will take the place of non-renewable energy sources, which produce roughly 90% of the nation’s energy (Udeagha and Ngepah, 2022c). Furthermore, in order to address the growing transnational environmental degradation as well as other knock-on consequences, international cooperation in climate change mitigation is required. In this sense, the South African government should make efforts to forge significant ties with the rest of the globe, particularly to exchange technology and lessen pollution. In order to promote the transition to environmentally friendly industries and a low-carbon economy, which encourages the creation of sustainable goods and services, South African authorities should, more crucially, include chapters on pollution avoidance in their trade deal policies. To further stimulate long-term value for GHG emission reductions and consistently support the development of innovative technologies that improve South Africa’s environmental position and safeguard the global environment, trade policy may be supplemented with additional policies.
Third, South Africa’s energy plans should incorporate renewable sources as an attractive alternative to reduce CO2 emissions. The use of renewable energy has recently received a strong economic promotion in South Africa. However, the nation still does not use enough renewable energy. More than 80% of South Africa’s entire primary energy supply comes from fossil fuels (Udeagha and Ngepah, 2022d). Even if the use of fossil fuels is decreasing, this proportion is still substantial. The study’s findings showed that a 1% increase in NREC increased environmental degradation by 0.42%, whereas REC decreased emissions by 0.35%. Increased energy usage in South Africa is a result of the growing human effect on the environment. This highlights how crucial it is to switch out NREC with renewable energy sources and promote sustainable energy sources via green technology. Despite recent major financial assistance from South Africa for the development of alternative energy sources, total energy use still pollutes the environment. In light of this, the government should enhance its management of natural resources by boosting the proportion of renewable energy in the total energy mix. The nation should also increase tax exemptions for businesses that use clean energy, reinforce incentives for low-carbon energy consumption, and boost energy efficiency and reduce energy intensity. South Africa should provide further assistance to businesses engaged in R&D to reduce the cost of implementing renewable energy sources.
Lastly, as an additional step, South Africa’s government could support the expansion of companies that produce energy-saving technology by offering low-interest funding to firms that want to use it in their production processes. The use of tax breaks or other non-price incentives that do not affect the price of fossil fuels can be utilized to promote energy efficiency. Additional incentives, tax breaks, and assistance should be provided to ecologically friendly energy sources in order to move the energy structure away from fossil fuels. In order for alternative energy sources to compete with non-renewable ones, they should receive more attention. Innovations in energy storage technology should be seen as a vital policy tool and managed alongside renewable energy programs. The potential importance of energy technology in reducing GHGs must also be highlighted. To reduce the social costs of utilizing fossil fuels, energy policy should concentrate on energy advancements.
Although the current work has yielded significant useful findings and important policy recommendations in the case of South Africa, one of the major limitations of this work is the use of CO2 emissions as the only environmental quality. Therefore, further research should examine other environmental quality proxies such as ecological footprint, sulfur dioxide emissions, nitrogen oxide emissions, and organic water pollutants to gain a better understanding of broader coverage.
Data availability statement
The original contributions presented in the study are included in the article/supplementary material. Further inquiries can be directed to the corresponding author.
Author contributions
MU and NN conceptualized the study idea, drafted the paper, collected data, analyzed data, wrote the introduction section, organized the literature review, drafted the methodology section, interpreted the results, provided the discussions, concluded the study with policy implications, and organized the reference list.
Conflict of interest
The authors declare that the research was conducted in the absence of any commercial or financial relationships that could be construed as a potential conflict of interest.
Publisher’s note
All claims expressed in this article are solely those of the authors and do not necessarily represent those of their affiliated organizations or those of the publisher, the editors, and the reviewers. Any product that may be evaluated in this article, or claim that may be made by its manufacturer, is not guaranteed or endorsed by the publisher.
References
Abdouli, M., and Hammami, S. (2017). Investigating the causality links between environmental quality, foreign direct investment and economic growth in MENA countries. Int. Bus. Rev. 26 (2), 264–278. doi:10.1016/j.ibusrev.2016.07.004
Abid, A., Mehmood, U., Tariq, S., and Haq, Z. U. (2022). The effect of technological innovation, FDI, and financial development on CO2 emission: Evidence from the G8 countries. Environ. Sci. Pollut. Res. 29 (8), 11654–11662. doi:10.1007/s11356-021-15993-x
Adebayo, T. S., and Odugbesan, J. A. (2021). Modeling CO2 emissions in South Africa: Empirical evidence from ARDL based bounds and wavelet coherence techniques. Environ. Sci. Pollut. Res. 28 (8), 9377–9389. doi:10.1007/s11356-020-11442-3
Adebayo, T. S., Oladipupo, S. D., Adeshola, I., and Rjoub, H. (2022). Wavelet analysis of impact of renewable energy consumption and technological innovation on CO2 emissions: Evidence from Portugal. Environ. Sci. Pollut. Res. 29 (16), 23887–23904. doi:10.1007/s11356-021-17708-8
African Development Bank Group (2019). South Africa economic outlook. Available at: https://www.afdb.org/en/countries/southern-africa/south-africa/south-africa-econ omic-outlook.
Ahmad, M., and Raza, M. Y. (2020). Role of public-private partnerships investment in energy and technological innovations in driving climate change: Evidence from Brazil. Environ. Sci. Pollut. Res. 27 (24), 30638–30648. doi:10.1007/s11356-020-09307-w
Ahmed, A., Uddin, G. S., and Sohag, K. (2016). Biomass energy, technological progress and the environmental Kuznets curve: Evidence from selected European countries. Biomass Bioenergy 90, 202–208. doi:10.1016/j.biombioe.2016.04.004
Anser, M. K., Ahmad, M., Khan, M. A., Zaman, K., Nassani, A. A., Askar, S. E., et al. (2021). The role of information and communication technologies in mitigating carbon emissions: Evidence from panel quantile regression. Environ. Sci. Pollut. Res. 28 (17), 21065–21084. doi:10.1007/s11356-020-12114-y
Atsu, F., Adams, S., and Adjei, J. (2021). ICT, energy consumption, financial development, and environmental degradation in South Africa. Heliyon 7 (7), e07328. doi:10.1016/j.heliyon.2021.e07328
Altinoz, B., Apergis, N., and Aslan, A. (2020). Energy consumption, carbon dioxide emissions and economic growth: Fresh evidence from panel quantile regressions. Energy Res. Lett. 1 (3), 17075. doi:10.46557/001c.17075
Álvarez-Herránz, A., Balsalobre, D., Cantos, J. M., and Shahbaz, M. (2017). Energy innovations-GHG emissions nexus: Fresh empirical evidence from OECD countries. Energy Policy 101, 90–100. doi:10.1016/j.enpol.2016.11.030
Arshad, Z., Robaina, M., and Botelho, A. (2020). The role of ICT in energy consumption and environment: An empirical investigation of Asian economies with cluster analysis. Environ. Sci. Pollut. Res. 27 (26), 32913–32932. doi:10.1007/s11356-020-09229-7
Asongu, S. A. (2018). ICT, openness and CO2 emissions in Africa. Environ. Sci. Pollut. Res. 25 (10), 9351–9359. doi:10.1007/s11356-018-1239-4
Awaworyi Churchill, S. A., Inekwe, J., Smyth, R., and Zhang, X. (2019). R&D intensity and carbon emissions in the G7: 1870–2014. Energy Econ. 80, 30–37. doi:10.1016/j.eneco.2018.12.020
Azevedo, V. G., Sartori, S., and Campos, L. M. (2018). CO2 emissions: A quantitative analysis among the BRICS nations. Renew. Sustain. Energy Rev. 81, 107–115. doi:10.1016/j.rser.2017.07.027
Baek, J., Cho, Y., and Koo, W. W. (2009). The environmental consequences of globalization: A country-specific time-series analysis. Ecol. Econ. 68 (8-9), 2255–2264. doi:10.1016/j.ecolecon.2009.02.021
Bales, K., and Sovacool, B. K. (2021). From forests to factories: How modern slavery deepens the crisis of climate change. Energy Res. Soc. Sci. 77, 102096. doi:10.1016/j.erss.2021.102096
Baloch, M. A., Khan, S. U. D., Ulucak, Z. Ş., and Ahmad, A. (2020). Analyzing the relationship between poverty, income inequality, and CO2 emission in Sub-Saharan African countries. Sci. Total Environ. 740, 139867. doi:10.1016/j.scitotenv.2020.139867
Balsa-Barreiro, J., Li, Y., Morales, A., and Pentland, A. S. (2019). Globalization and the shifting centers of gravity of world's human dynamics: Implications for sustainability. J. Clean. Prod. 239, 117923. doi:10.1016/j.jclepro.2019.117923
Bayer, C., and Hanck, C. (2013). Combining non-cointegration tests. J. Time Ser. analysis 34 (1), 83–95. doi:10.1111/j.1467-9892.2012.00814.x
Bekun, F. V., Emir, F., and Sarkodie, S. A. (2019). Another look at the relationship between energy consumption, carbon dioxide emissions, and economic growth in South Africa. Sci. Total Environ. 655, 759–765. doi:10.1016/j.scitotenv.2018.11.271
Berg, A., and Krueger, A. O. (2003). “Trade, growth, and poverty: A selective survey,” in Annual world bank conference on development economics 2003: The new reform agenda (Washington, DC: World Bank), 1047.
Brandão Santana, N., Rebelatto, D. A. D. N., Périco, A. E., Moralles, H. F., and Leal Filho, W. (2015). Technological innovation for sustainable development: An analysis of different types of impacts for countries in the BRICS and G7 groups. Int. J. Sustain. Dev. World Ecol. 22 (5), 425–436. doi:10.1080/13504509.2015.1069766
Breitung, J., and Candelon, B. (2006). Testing for short-and long-run causality: A frequency-domain approach. J. Econ. 132 (2), 363–378. doi:10.1016/j.jeconom.2005.02.004
Chen, Y., Yao, Z., and Zhong, K. (2022). Do environmental regulations of carbon emissions and air pollution foster green technology innovation: Evidence from China's prefecture-level cities. J. Clean. Prod. 350, 131537. doi:10.1016/j.jclepro.2022.131537
Chhabra, M., Giri, A. K., and Kumar, A. (2022). Do technological innovations and trade openness reduce CO2 emissions? Evidence from selected middle-income countries. Environ. Sci. Pollut. Res. 29, 65723–65738. doi:10.1007/s11356-022-20434-4
Cho, J. S., Kim, T.-H., and Shin, Y. (2015). Quantile cointegration in the autoregressive distributed-lag modeling framework. J. Econ. 188, 281–300. doi:10.1016/j.jeconom.2015.05.003
Cole, M. A., and Elliott, R. J. (2003). Determining the trade–environment composition effect: The role of capital, labor and environmental regulations. J. Environ. Econ. Manag. 46 (3), 363–383. doi:10.1016/s0095-0696(03)00021-4
Das, A., and Paul, B. P. (2011). Openness and growth in emerging Asian economies: Evidence from GMM estimations of a dynamic panel. Econ. Bull. 31, 2219–2228.
Dauda, L., Long, X., Mensah, C. N., Salman, M., Boamah, K. B., Ampon-Wireko, S., et al. (2021). Innovation, trade openness and CO2 emissions in selected countries in Africa. J. Clean. Prod. 281, 125143. doi:10.1016/j.jclepro.2020.125143
Demir, C., Cergibozan, R., and Ari, A. (2020). Environmental dimension of innovation: Time series evidence from Turkey. Environ. Dev. Sustain. 22 (3), 2497–2516. doi:10.1007/s10668-018-00305-0
Destek, M. A. (2021). Deindustrialization, reindustrialization and environmental degradation: Evidence from ecological footprint of Turkey. J. Clean. Prod. 296, 126612. doi:10.1016/j.jclepro.2021.126612
Destek, M. A., and Manga, M. (2021). Technological innovation, financialization, and ecological footprint: Evidence from BEM economies. Environ. Sci. Pollut. Res. 28 (17), 21991–22001. doi:10.1007/s11356-020-11845-2
Destek, M. A., and Okumus, I. (2019). Does pollution haven hypothesis hold in newly industrialized countries? Evidence from ecological footprint. Environ. Sci. Pollut. Res. 26 (23), 23689–23695. doi:10.1007/s11356-019-05614-z
Destek, M. A., Sarkodie, S. A., and Asamoah, E. F. (2021). Does biomass energy drive environmental sustainability? An SDG perspective for top five biomass consuming countries. Biomass Bioenergy 149, 106076. doi:10.1016/j.biombioe.2021.106076
Destek, M. A., Shahbaz, M., Okumus, I., Hammoudeh, S., and Sinha, A. (2020). The relationship between economic growth and carbon emissions in G-7 countries: Evidence from time-varying parameters with a long history. Environ. Sci. Pollut. Res. 27 (23), 29100–29117. doi:10.1007/s11356-020-09189-y
Dinda, S. (2018). Production technology and carbon emission: Long-run relation with short-run dynamics. J. Appl. Econ. 21 (1), 106–121. doi:10.1080/15140326.2018.1526871
Energy Information Administration (EIA) (2010). Energy statistics for countries. Washington, DC: U.S. Department of Energy Office of Scientific and Technical Information.
Enevoldsen, M. K., Ryelund, A. V., and Andersen, M. S. (2007). Decoupling of industrial energy consumption and CO2-emissions in energy-intensive industries in scandinavia. Energy Econ. 29 (4), 665–692. doi:10.1016/j.eneco.2007.01.016
Erdogan, S. (2021). Dynamic nexus between technological innovation and building sector carbon emissions in the BRICS countries. J. Environ. Manag. 293, 112780. doi:10.1016/j.jenvman.2021.112780
Faisal, S. M., and Idris, S. (2020). Innovation factors influencing the supply chain technology (SCT) adoption: Diffusion of innovation theory. Int. J. Soc. Sci. Res. 2 (2), 128–145.
Fine, B., and Rustomjee, Z. (1996). The political economy of South Africa: From Minerals-energy complex to industrialisation. London: Hurst.
Fisher-Vanden, K., Jefferson, G. H., Liu, H., and Tao, Q. (2004). What is driving China’s decline in energy intensity? Resour. Energy Econ. 26 (1), 77–97. doi:10.1016/j.reseneeco.2003.07.002
Godil, D. I., Sharif, A., Rafique, S., and Jermsittiparsert, K. (2020). The asymmetric effect of tourism, financial development, and globalization on ecological footprint in Turkey. Environ. Sci. Pollut. Res. 27 (32), 40109–40120. doi:10.1007/s11356-020-09299-7
Grether, J. M., and Mathys, N. (2009). How fast are CO2 emissions moving to Asia? Vox CEPR policy portal. AvaliableAt: https://voxeu.org/article/global-distribution-carbonemissions (Accessed June 9, 2019).
Gu, G., Zheng, H., Tong, L., and Dai, Y. (2022). Does carbon financial market as an environmental regulation policy tool promote regional energy conservation and emission reduction? Empirical evidence from China. Energy Policy 163, 112826. doi:10.1016/j.enpol.2022.112826
Guo, J., Zhou, Y., Ali, S., Shahzad, U., and Cui, L. (2021). Exploring the role of green innovation and investment in energy for environmental quality: An empirical appraisal from provincial data of China. J. Environ. Manag. 292, 112779. doi:10.1016/j.jenvman.2021.112779
Habiba, U. M. M. E., Xinbang, C., and Anwar, A. (2022). Do green technology innovations, financial development, and renewable energy use help to curb carbon emissions? Renew. Energy 193, 1082–1093. doi:10.1016/j.renene.2022.05.084
Hang, L., and Tu, M. (2007). The impacts of energy prices on energy intensity: Evidence from China. Energy policy 35 (5), 2978–2988. doi:10.1016/j.enpol.2006.10.022
Haseeb, A., Xia, E., Saud, S., Ahmad, A., and Khurshid, H. (2019). Does information and communication technologies improve environmental quality in the era of globalization? An empirical analysis. Environ. Sci. Pollut. Res. 26 (9), 8594–8608. doi:10.1007/s11356-019-04296-x
Hassan, T., Khan, Y., He, C., Chen, J., Alsagr, N., Song, H., et al. (2022). Environmental regulations, political risk and consumption-based carbon emissions: Evidence from OECD economies. J. Environ. Manag. 320, 115893. doi:10.1016/j.jenvman.2022.115893
Ibrahiem, D. M. (2020). Do technological innovations and financial development improve environmental quality in Egypt? Environ. Sci. Pollut. Res. 27 (10), 10869–10881. doi:10.1007/s11356-019-07585-7
Ibrahim, M., and Vo, X. V. (2021). Exploring the relationships among innovation, financial sector development and environmental pollution in selected industrialized countries. J. Environ. Manag. 284, 112057. doi:10.1016/j.jenvman.2021.112057
IPCC (2018). “Summary for policymakers,” in Global warming of 1.5 ◦c. An IPCC special report on the impacts of global warming of 1.5 ◦c above pre-industrial levels and related global greenhouse gas emission pathways, in the context of strengthening the global response to the threat of climate change, sustainable development, and efforts to eradicate poverty. Eds. V. Masson-Delmotte, P. Zhai, H. O. Pörtner, D. Roberts, J. Skea, P. R. Shuklaet al. (Geneva, Switzerland: World Meteorological Organization), 32.
IRENA (2021). Renewable power generation costs in 2020. Abu Dhabi: International Renewable Energy Agency.
Jiahua, P., Guiyang, Z., Yan, Z., ShouXian, Z., and Qianyi, X. (2010). Clarification of the concept of low-carbon economy and analysis of its core elements [J]. Int. Econ. Rev. 4, 88–102.
Jin, P., Peng, C., and Song, M. (2019). Macroeconomic uncertainty, high-level innovation, and urban green development performance in China. China Econ. Rev. 55, 1–18. doi:10.1016/j.chieco.2019.02.008
Joshua, U., and Bekun, F. V. (2020). The path to achieving environmental sustainability in South Africa: The role of coal consumption, economic expansion, pollutant emission, and total natural resources rent. Environ. Sci. Pollut. Res. 27 (9), 9435–9443. doi:10.1007/s11356-019-07546-0
Khalfaoui, R., Stef, N., Wissal, B. A., and Sami, B. J. (2022). Dynamic spillover effects and connectedness among climate change, technological innovation, and uncertainty: Evidence from a quantile VAR network and wavelet coherence. Technol. Forecast. Soc. Change 181, 121743. doi:10.1016/j.techfore.2022.121743
Khan, Z., Ali, M., Kirikkaleli, D., Wahab, S., and Jiao, Z. (2020a). The impact of technological innovation and public-private partnership investment on sustainable environment in China: Consumption-based carbon emissions analysis. Sustain. Dev. 28 (5), 1317–1330. doi:10.1002/sd.2086
Khan, Z., Ali, S., Umar, M., Kirikkaleli, D., and Jiao, Z. (2020b). Consumption-based carbon emissions and international trade in G7 countries: The role of environmental innovation and renewable energy. Sci. Total Environ. 730, 138945. doi:10.1016/j.scitotenv.2020.138945
Khattak, S. I., Ahmad, M., Khan, Z. U., and Khan, A. (2020). Exploring the impact of innovation, renewable energy consumption, and income on CO2 emissions: New evidence from the BRICS economies. Environ. Sci. Pollut. Res. 27 (12), 13866–13881. doi:10.1007/s11356-020-07876-4
Kim, T. H., and White, H. (2003). “Estimation, inference, and specification testing for possibly misspecified quantile regression,” in Maximum likelihood estimation of misspecified models: Twenty years later (Bradford, United Kingdom: Emerald Group Publishing Limited).
Kripfganz, S., and Schneider, D. C. (2018). Ardl: Estimating autoregressive distributed lag and equilibrium correction models. Avaliable at: www.stata.com/meeting/uk18/slides/uk18_Kripfganz.pdf.
Kuang, H., Akmal, Z., and Li, F. (2022). Measuring the effects of green technology innovations and renewable energy investment for reducing carbon emissions in China. Renew. Energy 197, 1–10. doi:10.1016/j.renene.2022.06.091
Lau, L. S., Choong, C. K., and Eng, Y. K. (2014). Carbon dioxide emission, institutional quality, and economic growth: Empirical evidence in Malaysia. Renew. Energy 68, 276–281. doi:10.1016/j.renene.2014.02.013
Li, Y., Zhang, C., Li, S., and Usman, A. (2022). Energy efficiency and green innovation and its asymmetric impact on CO2 emission in China: A new perspective. Environ. Sci. Pollut. Res. 29, 47810–47817. doi:10.1007/s11356-022-19161-7
Lin, B., and Ma, R. (2022). Green technology innovations, urban innovation environment and CO2 emission reduction in China: Fresh evidence from a partially linear functional-coefficient panel model. Technol. Forecast. Soc. Change 176, 121434. doi:10.1016/j.techfore.2021.121434
Ling, C. H., Ahmed, K., Muhamad, R. B., and Shahbaz, M. (2015). Decomposing the trade-environment nexus for Malaysia: What do the technique, scale, composition, and comparative advantage effect indicate? Environ. Sci. Pollut. Res. 22 (24), 20131–20142. doi:10.1007/s11356-015-5217-9
Liu, C., Xin, L., and Li, J. (2022). Environmental regulation and manufacturing carbon emissions in China: A new perspective on local government competition. Environ. Sci. Pollut. Res. 29 (24), 36351–36375. doi:10.1007/s11356-021-18041-w
Long, X., Chen, Y., Du, J., Oh, K., and Han, I. (2017). Environmental innovation and its impact on economic and environmental performance: Evidence from Korean-owned firms in China. Energy Policy 107, 131–137. doi:10.1016/j.enpol.2017.04.044
MacKinnon, J. G. (1996). Numerical distribution functions for unit root and cointegration tests. J. Appl. Econ. Chichester. Engl. 11 (6), 601–618. doi:10.1002/(sici)1099-1255(199611)11:6<601::aid-jae417>3.0.co;2-t
Mensah, C. N., Long, X., Boamah, K. B., Bediako, I. A., Dauda, L., and Salman, M. (2018). The effect of innovation on CO2 emissions of OCED countries from 1990 to 2014. Environ. Sci. Pollut. Res. 25 (29), 29678–29698. doi:10.1007/s11356-018-2968-0
Ngepah, N., and Udeagha, M. C. (2018). African regional trade agreements and intra-African trade. J. Econ. Integration 33 (1), 1176–1199. doi:10.11130/jei.2018.33.1.1176
Ngepah, N., and Udeagha, M. C. (2019). Supplementary trade benefits of multi-memberships in African regional trade agreements. J. Afr. Bus. 20 (4), 505–524. doi:10.1080/15228916.2019.1584719
Ngo, T. Q. (2022). How do environmental regulations affect carbon emission and energy efficiency patterns? A provincial-level analysis of Chinese energy-intensive industries. Environ. Sci. Pollut. Res. 29 (3), 3446–3462. doi:10.1007/s11356-021-15843-w
Obobisa, E. S., Chen, H., and Mensah, I. A. (2022). The impact of green technological innovation and institutional quality on CO2 emissions in African countries. Technol. Forecast. Soc. Change 180, 121670. doi:10.1016/j.techfore.2022.121670
OECD (2002). Indicators to measure decoupling of environmental pressures from economic growth. Paris: OECD.
Omri, A., Nguyen, D. K., and Rault, C. (2014). Causal interactions between CO2 emissions, FDI, and economic growth: Evidence from dynamic simultaneous-equation models. Econ. Model. 42, 382–389. doi:10.1016/j.econmod.2014.07.026
Ozcan, B., and Apergis, N. (2018). The impact ofinternet use on air pollution: Evidence from emerging countries. Environ. Sci. Pollut. Res. 25 (5), 4174–4189. doi:10.1007/s11356-017-0825-1
Pathak, L., and Shah, K. (2019). Renewable energy resources, policies and gaps in BRICS countries and the global impact. Front. Energy 13, 506–521. doi:10.1007/s11708-018-0601-z
Pesaran, M. H., Shin, Y., and Smith, R. J. (2001). Bounds testing approaches to the analysis of level relationships. J. Appl. Econ. Chichester. Engl. 16 (3), 289–326. doi:10.1002/jae.616
Rafique, M. Z., Schneider, N., Shahzad, U., and Song, M. (2022). High-tech industries, financial expansion, and low-carbon energy deployment along the Belt and Road Initiative. New York, United States: John Wiley & Sons, Inc.
Rahman, M. M., Alam, K., and Velayutham, E. (2022). Reduction of CO2 emissions: The role of renewable energy, technological innovation and export quality. Energy Rep. 8, 2793–2805. doi:10.1016/j.egyr.2022.01.200
Razzaq, A., An, H., and Delpachitra, S. (2021). Does technology gap increase FDI spillovers on productivity growth? Evidence from Chinese outward FDI in Belt and Road host countries. Technol. Forecast. Soc. Change 172, 121050. doi:10.1016/j.techfore.2021.121050
Roman-Collado, R., Cansino, J. M., and Botia, C. (2018). How far is Colombia from decoupling? Two-level decomposition analysis of energy consumption changes. Energy 148, 687–700. doi:10.1016/j.energy.2018.01.141
Saboori, B., Sulaiman, J., and Mohd, S. (2012). Economic growth and CO2 emissions in Malaysia: A cointegration analysis of the environmental Kuznets curve. Energy policy 51, 184–191. doi:10.1016/j.enpol.2012.08.065
Santra, S. (2017). The effect of technological innovation on production-based energy and CO2 emission productivity: Evidence from BRICS countries. Afr. J. Sci. Technol. Innovation Dev. 9 (5), 503–512. doi:10.1080/20421338.2017.1308069
Shahbaz, M., Khan, S., and Tahir, M. I. (2013a). The dynamic links between energy consumption, economic growth, financial development and trade in China: Fresh evidence from multivariate framework analysis. Energy Econ. 40, 8–21. doi:10.1016/j.eneco.2013.06.006
Shahbaz, M., Destek, M. A., and Polemis, M. L. (2018). Do foreign capital and financial development affect clean energy consumption and carbon emissions? Evidence from BRICS and next-11 countries. SPOUDAI-Journal Econ. Bus. 68 (4), 20–50.
Shahbaz, M., Khraief, N., Uddin, G. S., and Ozturk, I. (2014a). Environmental Kuznets curve in an open economy: A bounds testing and causality analysis for Tunisia. Renew. Sustain. Energy Rev. 34, 325–336. doi:10.1016/j.rser.2014.03.022
Shahbaz, M., Lean, H. H., and Shabbir, M. S. (2012b). Environmental Kuznets curve hypothesis in Pakistan: Cointegration and granger causality. Renew. Sustain. Energy Rev. 16 (5), 2947–2953. doi:10.1016/j.rser.2012.02.015
Shahbaz, M., Sbia, R., Hamdi, H., and Ozturk, I. (2014b). Economic growth, electricity consumption, urbanization and environmental degradation relationship in United Arab Emirates. Ecol. Indic. 45, 622–631. doi:10.1016/j.ecolind.2014.05.022
Shahbaz, M., Tiwari, A. K., and Nasir, M. (2013c). The effects of financial development, economic growth, coal consumption and trade openness on CO2 emissions in South Africa. Energy Policy 61, 1452–1459. doi:10.1016/j.enpol.2013.07.006
Shan, S., Genç, S. Y., Kamran, H. W., and Dinca, G. (2021). Role of green technology innovation and renewable energy in carbon neutrality: A sustainable investigation from Turkey. J. Environ. Manag. 294, 113004. doi:10.1016/j.jenvman.2021.113004
Sohag, K., Al Mamun, M., Uddin, G. S., and Ahmed, A. M. (2017). Sectoral output, energy use, and CO2 emission in middle-income countries. Environ. Sci. Pollut. Res. 24 (10), 9754–9764. doi:10.1007/s11356-017-8599-z
Sohag, K., Begum, R. A., Abdullah, S. M. S., and Jaafar, M. (2015). Dynamics of energy use, technological innovation, economic growth and trade openness in Malaysia. Energy 90, 1497–1507. doi:10.1016/j.energy.2015.06.101
Sovacool, B. K. (2021). Who are the victims of low-carbon transitions? Towards a political ecology of climate change mitigation. Energy Res. Soc. Sci. 73, 101916. doi:10.1016/j.erss.2021.101916
Squalli, J., and Wilson, K. (2011). A new measure of trade openness. World Econ. 34 (10), 1745–1770. doi:10.1111/j.1467-9701.2011.01404.x
Statistics South Africa (2019). Economic growth. Available at: http://www.statssa.gov.za/ ?page_id=735&id=1.
Szigeti, C., Toth, G., and Szabo, D. R. (2017). Decoupling–shifts in ecological footprint intensity of nations in the last decade. Ecol. Indic. 72, 111–117. doi:10.1016/j.ecolind.2016.07.034
Tapio, P. (2005). Towards a theory of decoupling: Degrees of decoupling in the EU and the case of Road traffic in Finland between 1970 and 2001. Transp. Policy 12 (2), 137–151. doi:10.1016/j.tranpol.2005.01.001
Töbelmann, D., and Wendler, T. (2020). The impact of environmental innovation on carbon dioxide emissions. J. Clean. Prod. 244, 118787. doi:10.1016/j.jclepro.2019.118787
Udeagha, M. C., and Breitenbach, M. C. (2021). Estimating the trade-environmental quality relationship in SADC with a dynamic heterogeneous panel model. Afr. Rev. Econ. Finance 13 (1), 113–165.
Udeagha, M. C., and Breitenbach, M. C. (2022). Exploring the moderating role of financial development in environmental Kuznets curve for South Africa: Fresh evidence from the novel dynamic ARDL simulations approach. Financ. Innov. 2022, 1–38. doi:10.1186/s40854-022-00396-9
Udeagha, M. C., and Muchapondwa, E. (2022). Investigating the moderating role of economic policy uncertainty in environmental Kuznets curve for South Africa: Evidence from the novel dynamic ARDL simulations approach. Environ. Sci. Pollut. Res. 29, 77199–77237. doi:10.1007/s11356-022-21107-y
Udeagha, M. C., and Ngepah, N. (2022a). Disaggregating the environmental effects of renewable and non-renewable energy consumption in South Africa: Fresh evidence from the novel dynamic ARDL simulations approach. Econ. Change Restruct. 55, 1767–1814. doi:10.1007/s10644-021-09368-y
Udeagha, M. C., and Ngepah, N. (2022b). Does trade openness mitigate the environmental degradation in South Africa? Environ. Sci. Pollut. Res. 29 (13), 19352–19377. doi:10.1007/s11356-021-17193-z
Udeagha, M. C., and Ngepah, N. (2022c). Dynamic ARDL simulations effects of fiscal decentralization, green technological innovation, trade openness, and institutional quality on environmental sustainability: Evidence from South Africa. Sustainability 14, 10268. doi:10.3390/su141610268
Udeagha, M. C., and Ngepah, N. (2019). Revisiting trade and environment nexus in South Africa: Fresh evidence from new measure. Environ. Sci. Pollut. Res. 26, 29283–29306. doi:10.1007/s11356-019-05944-y
Udeagha, M. C., and Ngepah, N. (2022d). Striving towards environmental sustainability in the BRICS economies: The combined influence of fiscal decentralization and environmental innovation. Int. J. Sustain. Dev. World Ecol. 1, 15. doi:10.1080/13504509.2022.2123411
Udeagha, M. C., and Ngepah, N. (2021a). The asymmetric effect of trade openness on economic growth in South Africa: A nonlinear ARDL approach. Econ. Change Restruct. 54 (2), 491–540. doi:10.1007/s10644-020-09285-6
Udeagha, M. C., and Ngepah, N. (2020). Trade liberalization and the geography of industries in South Africa: Fresh evidence from a new measure. Int. J. Urban Sci. 24 (3), 354–396. doi:10.1080/12265934.2019.1695652
Udeagha, M. C., and Ngepah, N. N. (2021b). A step towards environmental mitigation in South Africa: Does trade liberalisation really matter? Fresh evidence from A novel dynamic ARDL simulations approach. Res. Square. doi:10.21203/rs.3.rs-419113/v1
Usman, M., and Hammar, N. (2021). Dynamic relationship between technological innovations, financial development, renewable energy, and ecological footprint: Fresh insights based on the STIRPAT model for Asia pacific economic cooperation countries. Environ. Sci. Pollut. Res. 28 (12), 15519–15536. doi:10.1007/s11356-020-11640-z
Villanthenkodath, M. A., and Mahalik, M. K. (2022). Technological innovation and environmental quality nexus in India: Does inwardremittance matter? J. Public Aff. 22 (1), e2291. doi:10.1002/pa.2291
Vitenu-Sackey, P. A., and Acheampong, T. (2022). Impact of economic policy uncertainty, energy intensity, technological innovation and R&D on CO2 emissions: Evidence from a panel of 18 developed economies. Environ. Sci. Pollut. Res. Int. 1, 20. doi:10.1007/s11356-022-21729-2
Wang, L., Wang, Z., and Ma, Y. (2022b). Does environmental regulation promote the high-quality development of manufacturing? A quasi-natural experiment based on China's carbon emission trading pilot scheme. Socio-Economic Plan. Sci. 81, 101216. doi:10.1016/j.seps.2021.101216
Wang, X., Zhang, T., Nathwani, J., Yang, F., and Shao, Q. (2022a). Environmental regulation, technology innovation, and low carbon development: Revisiting the EKC Hypothesis, Porter Hypothesis, and Jevons’ Paradox in China's iron & steel industry. Technol. Forecast. Soc. Change 176, 121471. doi:10.1016/j.techfore.2022.121471
Wang, Z., Gao, L., Wei, Z., Majeed, A., and Alam, I. (2022c). How FDI and technology innovation mitigate CO2 emissions in high-tech industries: Evidence from province-level data of China. Environ. Sci. Pollut. Res. 29 (3), 4641–4653. doi:10.1007/s11356-021-15946-4
Xia, Y., and Zhong, M. C. (2016). Relationship between EKC hypothesis and the decoupling of environmental pollution from economic development: Based on China prefecture-level cities’ decoupling partition. China Popul. Resour. Environ. 26 (10), 8–16.
Xiao, Z. (2009). Quantile cointegrating regression. J. Econ. 150 (2), 248–260. doi:10.1016/j.jeconom.2008.12.005
Xie, L., Zhou, Z., and Hui, S. (2022). Does environmental regulation improve the structure of power generation technology? Evidence from China's pilot policy on the carbon emissions trading market (CETM). Technol. Forecast. Soc. Change 176, 121428. doi:10.1016/j.techfore.2021.121428
Xu, B., and Xu, R. (2022). Assessing the role of environmental regulations in improving energy efficiency and reducing CO2 emissions: Evidence from the logistics industry. Environ. Impact Assess. Rev. 96, 106831. doi:10.1016/j.eiar.2022.106831
Yang, B., Jahanger, A., and Ali, M. (2021). Remittance inflows affect the ecological footprint in BICS countries: Do technological innovation and financial development matter? Environ. Sci. Pollut. Res. 28 (18), 23482–23500. doi:10.1007/s11356-021-12400-3
Yang, Y., and Meng, G. F. (2019). The decoupling effect and driving factors of carbon footprint in megacities: The case study of xi’an in western China. Sustain. Cities Soc. 44, 783–792. doi:10.1016/j.scs.2018.11.012
Yii, K. J., and Geetha, C. (2017). The nexus between technology innovation and CO2 emissions in Malaysia: Evidence from granger causality test. Energy Procedia 105, 3118–3124. doi:10.1016/j.egypro.2017.03.654
Yin, K., Liu, L., and Gu, H. (2022). Green paradox or forced emission reduction—the dual effects of environmental regulation on carbon emissions. Int. J. Environ. Res. Public Health 19 (17), 11058. doi:10.3390/ijerph191711058
Yirong, Q. (2022). Does environmental policy stringency reduce CO2 emissions? Evidence from high-polluted economies. J. Clean. Prod. 341, 130648. doi:10.1016/j.jclepro.2022.130648
Zahonogo, P. (2017). Trade and economic growth in developing countries: Evidence from sub-saharan Africa. J. Afr. Trade 3 (1-2), 41–56. doi:10.1016/j.joat.2017.02.001
Zameer, H., Shahbaz, M., and Vo, X. V. (2020). Reinforcing poverty alleviation efficiency through technological innovation, globalization, and financial development. Technol. Forecast. Soc. Change 161, 120326. doi:10.1016/j.techfore.2020.120326
Zhang, Q., Zhang, S., Ding, Z., and Hao, Y. (2017a). Does government expenditure affect environmental quality? Empirical evidence using Chinese city-level data. J. Clean. Prod. 161, 143–152. doi:10.1016/j.jclepro.2017.05.096
Zhang, Y. J., Peng, Y. L., Ma, C. Q., and Shen, B. (2017b). Can environmental innovation facilitate carbon emissions reduction? Evidence from China. Energy Policy 100, 18–28. doi:10.1016/j.enpol.2016.10.005
Keywords: CO2 emissions, QARDL, energy consumption, EKC, economic growth, industrial value-added, South Africa, trade openness
Citation: Udeagha MC and Ngepah N (2022) The asymmetric effect of technological innovation on CO2 emissions in South Africa: New evidence from the QARDL approach. Front. Environ. Sci. 10:985719. doi: 10.3389/fenvs.2022.985719
Received: 04 July 2022; Accepted: 25 October 2022;
Published: 23 November 2022.
Edited by:
Yingcheng Li, Southeast University, ChinaReviewed by:
Umer Shahzad, Anhui University of Finance and Economics, ChinaJose Balsa-Barreiro, Massachusetts Institute of Technology, United States
Copyright © 2022 Udeagha and Ngepah. This is an open-access article distributed under the terms of the Creative Commons Attribution License (CC BY). The use, distribution or reproduction in other forums is permitted, provided the original author(s) and the copyright owner(s) are credited and that the original publication in this journal is cited, in accordance with accepted academic practice. No use, distribution or reproduction is permitted which does not comply with these terms.
*Correspondence: Maxwell Chukwudi Udeagha, bWF4d2VsbHVjQHlhaG9vLmNvbQ==
‡These authors have contributed equally to this work
†ORCID: Maxwell Chukwudi Udeagha, orcid.org/0000-0001-6143-8362Nicholas Ngepah, orcid.org/0000-0002-1947-0008