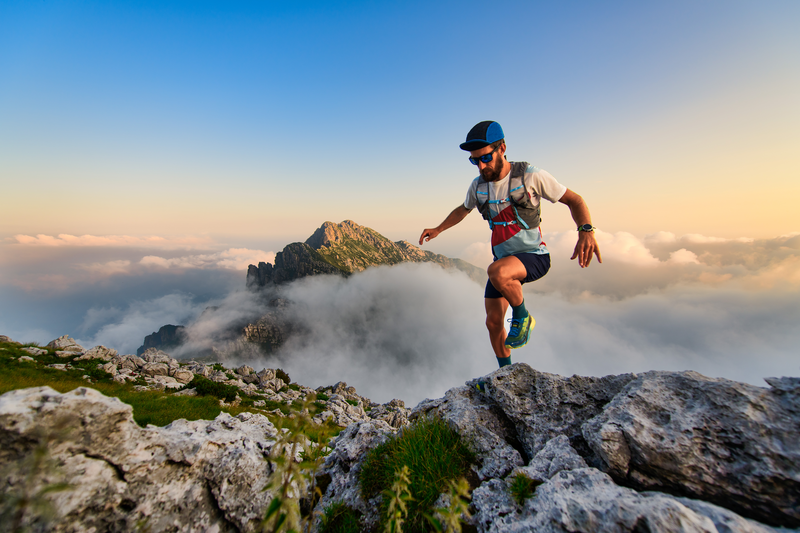
94% of researchers rate our articles as excellent or good
Learn more about the work of our research integrity team to safeguard the quality of each article we publish.
Find out more
ORIGINAL RESEARCH article
Front. Environ. Sci. , 24 November 2022
Sec. Freshwater Science
Volume 10 - 2022 | https://doi.org/10.3389/fenvs.2022.985672
This article is part of the Research Topic Transport and Fate of Contaminants in Waters View all 4 articles
In aquatic ecosystems, the dynamics of ecological parameters play an important role in community organizations. To understand the seasonal variation in the phytoplankton community in Lake Wuchang, Anhui Province, water samples were collected in three different hydrological periods (dry season, normal season, and wet season) from December 2019 to August 2020. The dominance value (Y), niche width (Bi), niche overlap (Oij), variance ratio method (VR), chi-squared test (χ2), and association coefficients were used to analyze the niche and interspecific association with respect to the dominant phytoplankton species in different hydrological periods, while the redundancy analysis method (RDA) was used to distinguish the key driving environmental factors for the dominant phytoplankton species. The results showed that 308 species belonging to 8 phyla were identified, of which 13 species from 4 phyla were the dominant species. From the dry season to wet season, the dominant species changed from Bacillariophyta to Cyanophyta, while Synedra acus was the dominant species throughout the hydrological periods. The niche width of the dominant species varied from 1.77 to 6.65, and the niche width of Dinobryon was the lowest, while that of Synedra acus was the highest. The niche overlap showed temporal and spatial differences, of which 30 dominant species pairs were greater than 0.600, indicating that they occupied the environmental resources in a highly similar way. The interspecific association results showed that there was a significant positive correlation between the dominant species, especially in normal and wet seasons. Only 10 of the 48 dominant species pairs had significant associations based on the χ2 test, and two pairs had negative associations in the wet season, which might be related to the hydrological condition in the wet season. According to redundancy analysis, NO3−-N, NH4+-N, PO43--P, water depth (WD), and water temperature (WT) were the key environmental factors affecting the phytoplankton community variation in Lake Wuchang. This paper provides a new perspective for the study of the early warnings regarding the cyanobacteria bloom outbreak, and the management and protection of the water ecological environment in shallow lakes in the middle and lower reaches of the Yangtze River.
The niche is allegedly the conceptual bedrock underpinning the most prominent, and some would say most important, theories in ecology (Wakil and Justus, 2022), since the term “niche” was first used to investigate species distribution in the 1920s. As one of the basic mechanisms of species’ coexistence, the niche theory plays an important role in predicting species distribution along an environmental gradient (Chase, 2011), which has become a mainstream ecological concept to study the competition between different species for resources and to compare the adaptability of different species to the environment (Bates et al., 2020; Sales et al., 2021). The niche theory mainly focuses on expressing the competitiveness of species in a specific environment through the niche width and overlap with other species to explain their richness and distribution (Ahmad et al., 2021). The niche width can be used to measure the ability of a species to use environmental resources in a given habitat and plays an important role in predicting the changes of underground microbial community diversity in a coastal pull-out gradient (Feng et al., 2020). Populations with broad ecological niches are usually called generalized species, which have a strong ability to use different resources, reproduce in different habitats, have strong ecological adaptability, and are in a strong position in inter-species resource competition (Chaparro-Pedraza and de Roos, 2020). It is also considered to be the ability of two different species or populations with similar environmental adaptations to use the same limited resources (Tilman, 2004). Interspecific relationships, especially competition and predation, are important driving factors in community structure changes and have significant effects on the formation of the dominant species community structure (Arroyo et al., 2015; Tarjuelo et al., 2017). The study of interspecific association among different species is helpful to understand the basic ecological characteristics of the whole community, such as structure and function, and community succession and evolution (Prati et al., 2021). The niche of species in a community is closely related to interspecific linkages, and the interspecific interactions will lead to the formation of unique environmental niches among species (Ciancio et al., 2021).
Recent studies on the niche have been widely used in the field of aquatic ecosystems such as fish (Barroeta et al., 2022), crab (Zhang et al., 2020), zooplankton (Park et al., 2021; Gao et al., 2022; Pantel et al., 2022), and phytoplankton (da Silva et al., 2021; Ma Y et al., 2022). Phytoplankton plays a crucial role in maintaining the ecological balance of water in the process of energy flow, material circulation, and information transfer in the ecosystem (Várbíró et al., 2020) as the basis components of the food chain in the aquatic environment (Nagy-László et al., 2020). Therefore, the combination of community stability, interspecific association, and niche theory can reflect the basic structural and developmental state of the phytoplankton community comprehensively and effectively, which is helpful to understand the ecological habits and the relationships of the dominant species in resource utilization, and has guiding significance for the management and restoration of the lake ecosystem (Chen et al., 2018). The dominant species has a significant impact on the formation of the community structure and community environment, and its temporal changes are mainly used to manifest various community structures (Ma C et al., 2022). The reliability of the phytoplankton niche model in algal bloom prediction has been verified by analyzing the niche width and niche overlap of the dominant phytoplankton species in lake and reservoir ecosystems (Shan et al., 2019; Wang et al., 2020; Cervantes-Urieta et al., 2021; Hofmann et al., 2021; Peng et al., 2021; Tao et al., 2021; Kléparski et al., 2022; Ma Y et al., 2022; Machado et al., 2022). In addition, the knowledge of the ecological requirements and relationships of dominant species regarding resource utilization is important for lake ecosystem management and restoration.
Lake Wuchang is a typical shallow lake (30° 14′–30° 20′ N and 116° 36′–116° 53′ E) located in Anhui Province, southeast China. As an important part of the lakes along the Yangtze River, it plays an important ecological role in fish breeding, runoff regulation, climate improvement, and species protection. According to the investigation report on China’s lakes, the water level of Lake Wuchang has typical seasonal variation characteristics due to the influence of climate, rainfall, and the water level of the Yangtze River. In recent years, due to the impact of climate change, industrial and agricultural production, and other human activities, there were great changes in the ecosystem of this lake (Zhao et al., 2021). During the dry season in winter, with the water level dropped and the lake bed exposed, a large number of wild rice grasses wither and deposit, which will accelerate the decomposition of plants and release nitrogen, phosphorus, and other biogenic elements into the water body and increase water temperature. In case of sustained high temperature, it will further increase the eutrophication of the lake, promote the rapid propagation and growth of some species of cyanobacteria such as Microcystis, induce algal bloom or lake flooding, and threaten the service function of the lake ecosystem (Kang et al., 2020).
To learn more about the change characteristics of the dominant phytoplankton species and their key driving environmental factors, we studied the dominant species based on the niche theory and our main questions are 1) what is the difference in the niche breadth, niche overlap, and the interspecific relationship of the dominant phytoplankton species across the three different hydrological seasons? 2) What physicochemical factors significantly affect the abundance of the dominant species, and what is the relative contribution of these factors? And 3) can the niche theory method often used in higher plants and large fish be applied to phytoplankton to predict the possibility of cyanobacteria bloom in the shallow lakes of the middle and lower reaches of the Yangtze River?
Lake Wuchang is a typical shallow lake in the middle and lower reaches of the Yangtze River (Figure 1). It belongs to the subtropical monsoon climate zone and has typical transitional zone climate characteristics. It has an area of 87.5 km2 corresponding to a volume of 4.23 × 108 m3. It is 30.0 km long and 9.5 km wide, with an average depth of 2.52 m and a maximum depth of 5.0 m. The average annual temperature, rainfall, and water level are 16.6°C, 1299.6 mm, and 11.99 m, respectively. Lake Wuchang is divided into two lake regions by the Anqing–Jiujiang highway, named Wuchang and Qingcao lake regions, respectively. A total of eight sites were set according to the lake’s morphological characteristics. Among those sites, WC1 and WC3 sites in the Wuchang lake region are close to the lake inlets, and the LHK site in the Qingcao lake region located at the intersection of the Xingfu River and Xinzhang River is the lake outlet, which connects Lake Wuchang and the Yangtze River. Samples were collected in December 2019 (dry season), June 2020 (normal season), and August 2020 (wet season), respectively, except for the WC2 site, which was not collected because of the bad weather in the wet season.
The samples were collected according to the freshwater biological resource survey specifications (China, 2009). Quantitative samples of phytoplankton were collected from the surface water (0.5 m) using the 5-L water collector thrice and mixed thoroughly. After that, 1 L water was scooped into a plastic container and immediately added to 10 ml of 1% Lugol’s iodine solution for fixation. All samples were taken back to the laboratory and precipitated in the dark and cooled for 48 h. After siphoning off the supernatant and concentrating it to 30 ml, 0.1 ml of the 30 ml supernatant was taken and observed under a microscope for the algae species identification and calculation of abundance and biomass. For the algae identification, we referred to the classification and ecology of freshwater algae in China by Hu and Wei (2006).
Water quality parameters, including water temperature (WT), pH, dissolved oxygen (DO), and conductivity (EC), were measured using a Hach HQ40d (Danaher, CO, United States) portable multi-parameter water quality detector on site; Secchi disk and SM-5 bathometers were used to measure the transparency (SD) and water depth (WD), respectively. Total nitrogen (TN), ammonia nitrogen (NH4+-N), nitrate nitrogen (NO3−-N), nitrite nitrogen (NO2−-N), total phosphorus (TP), phosphate (PO43--P), and total suspended solids (TSS) were determined following the Chinese national standard analysis method (GB3838-2002) using a Hach DRB 200 digester (Danaher, CO, United States) and a DR1900 portable spectrophotometer (Danaher, CO, United States).
The dominant phytoplankton species were determined by the value of dominance (Y), which was calculated by the formula according to McNaughton (1967):
where Y is the value of dominance, ni is the individual number of the ith phytoplankton species, n is the total number of the phytoplankton species, and fi is the frequency of the ith species. The species with Y ≥ 0.02 represents the dominant species, and Y > 0.1 represents the absolute dominant species (Wu et al., 2021).
The niche width (Bi) and niche overlap (Oik) were calculated with indexes of Levins (2020) and Gu et al. (2017), respectively.
where Bi represents the niche width of the ith species, Pij = nij/Ni represents the proportion of the number of individuals of species i in the jth sampling site to the number of all individuals of the species, factor nij is the number of the sampling site j used by species i, Ni is the total number of species i, N is the total number of sampling sites, Oik represents the niche overlap value of the dominant species i and k in the jth sampling site, Pkj represents the proportion of the number of individuals of species k to the number of all individuals of the species in the jth sampling site.
Using the variance ratio (VR) method to test the overall association between species, the chi-squared (χ2) test was used to study the interspecific association after being calculated by Yates’ continuity correction formula (Ma Y et al., 2022). The formulas are as follows:
where δT2 represents the variance of species in all the sampling sites, S is the total number of all the dominant phytoplankton species, Pi = mi/N, N represents the total number of sampling sites, and mi represents the number of sampling sites where species i appears. ST2 represents the variance of occurrence frequency of total species, Tj is the dominant species number of the jth sampling site, t is the average number of species of sampling sites, and VR represents the overall correlation form among the species. χ2 represents the chi-value, a is the number of sampling sites in which species A and B occurred together, b is the number of sampling sites where species A but not B occurred, and c is the number of sampling sites where species B but not A occurred, and d is the number sampling points where neither species A nor B occurred. The statistical quantity W was used to test the significance of the deviation of the VR value from 1.
First, detrended correspondence analysis (DCA) was performed to get the gradient length of the first axis (3.025), which means redundancy analysis (RDA) was suitable for further analysis (Yang et al., 2022). Redundancy analysis (RDA) is a linear model which has been widely used to investigate the relationships between environmental factors and phytoplankton compositions (Tian et al., 2021; Xu et al., 2021). Prior to that, environmental factors, except pH, were converted by lg (x+1) to meet normality and homoscedasticity conducting a Monte Carlo permutation test (p < 0.05), and the abundance data of the dominant species were converted by Hellinger (Yan et al., 2020; Sánchez et al., 2021), Subsequently, the variance inflation factor (VIF) was calculated with the transformed explanatory variables to reduce the collinearity effects, which were eliminated with the VIF over 10 (Wu et al., 2018). After that, the forward selection method was used to filter the most important explanatory variables for the community structure (Peng et al., 2021). The variance decomposition function “varpart” was used to test the contribution of different environmental explanatory variables to the community structure, and the significance of the difference was verified after 9,999 times replacement (Chen et al., 2022). In addition, Excel 2016 was used to calculate the dominance (Y), niche width (Bi), niche overlap (Oik), and interspecific association, and the χ2 test was performed with the “spaa” and “vegan” packages by R v4.1.1 and ArcGis10.5, and the R “ggplot2” package was used for scientific mapping.
During the survey, we collected and identified a total species of 308 belonging to 8 phyla (Table 1). Among them, there were 145 species of Chlorophyta, accounting for 47.1%, followed by Bacillariophyta, Euglenophyta, and Cyanophyta, with 71 species, 35 species, and 32 species, accounting for 23.1%, 11.4%, and 10.4%, respectively. The number of phytoplankton species in the wet season and the normal season was significantly higher than that in dry season.
There was a total of 13 dominant species from 4 phyla during the survey period (Table 2). There were notable differences in the composition of the dominant species in different hydrological periods. The Synedra aacus of Bacillariophyta was the common dominant species that occurred in all the three hydrological seasons, and Pseudoanabaena of cyanobacteria was the common dominant species in dry and wet seasons, while Microcystis and Anabaena of cyanobacteria were the common dominant species in normal and wet seasons, and Aulacoseira was the absolute dominant species whose dominance value (Y) was 0.363, which was significantly higher than 0.1.
The spatial-temporal variation in the relative abundance and the biomass of the dominant species in different hydrological seasons is shown in Figure 2 and Figure 3. The relative abundance of the dominant species of cyanobacteria exceeded 80% at all sampling sites except for dry season, where Bacillariophyta accounted for 91.4% and 66.8% at LHK and QC2 sites, respectively. The dominant species’ relative biomass of Cyanophyta and Bacillariophyta was higher than others at different sampling sites across the whole hydrological period, and their relative biomass was mainly composed of Cyanophyta and Bacillariophyta except for the QC3 site of the dry season and WC3 site of the normal season, where the proportion of Chrysophyta and Euglenophyta was over 75% and 60.0%, respectively.
FIGURE 2. Spatial-temporal variation in the relative abundance of the dominant phytoplankton species in different hydrological seasons.
FIGURE 3. Spatial-temporal variation in the relative biomass of the dominant phytoplankton species in different hydrological seasons.
The niche width of the dominant species ranged from 1.77 to 6.65, with the lowest value for Dinobryon and the highest value for Synedra acus. The niche width range in normal and wet seasons was 1.99–4.59 and 3.41–4.87, respectively. Microcystis and Anabaena of cyanobacteria showed the lowest and highest value in the normal season, respectively, while Raphidiopsis of cyanobacteria showed the highest value in the wet season. The niche breadth of the common dominant species in the three periods was greater than 3.0, indicating they had a stronger ability to utilize environmental resources (Table 3). The niche overlap ranges of dry, normal, and wet seasons were 0.210–0.972, 0.025–0.973, and 0.273–0.918, respectively. The maximum and minimum niche overlap values occurred in the normal season, in which the minimum niche overlap value of Leptolyngbya and Microcystis was 0.025, while the maximum niche overlap value of Leptolyngbya and Aulacoseira granulata was 0.973. There were 30 dominant species pairs with a value of the niche overlap over 0.600, and 5, 18, and 7 pairs in dry, normal, and wet seasons, accounting for 50.0%, 70.0%, and 64.3% of the number of dominant species, respectively (Figure 4).
TABLE 3. Variations in the niche width (Bi) of the dominant phytoplankton species in different hydrological seasons.
FIGURE 4. Variations in the niche overlap (Oik) of the dominant phytoplankton species in different hydrological seasons. The dominant species number and their corresponding relationships in the figure were consistent with those in Table 2.
As shown in Table 4, the variance ratio (VR) value was over 1 in the three hydrological periods, and the W value in the dry season is lower than the upper limit of χ2, while the value in normal and wet periods was higher, indicating that the overall interspecific association between phytoplankton communities was not significant in the dry season (p > 0.05), but significant in the normal and wet season (p < 0.05). The interspecific association analysis among the dominant species is shown in Figure 5. Among 48 dominant species pairs, 10 pairs had significant interspecific association (χ2 > 3.84, p < 0.05) including five pairs with positive (χ2 > 3.84, p < 0.05, and ad > bc) and one pair with an extremely significant positive interspecific association in the normal season; similarly, in the wet season, the interspecific association with four pairs was extremely significant (χ2 > 6.65, p < 0.05, and ad > bc).
TABLE 4. Overall association of the dominant phytoplankton species in different hydrological seasons.
FIGURE 5. Half matrix of association coefficients χ2 test among the dominant phytoplankton species in different hydrological seasons. The dominant species number and their corresponding relationships in the figure were consistent with those in Table 2.
According to the VIF value of the explanatory variables and the forward selection, nine environmental factors (Table 5; Figure 6) were selected in total to explain 79.97% of the variance, of which the first axis interpretation rate was 54.25% (F = 34.83, p = 0.001) and the second axis interpretation rate was 9.14% (F = 16.48, p = 0.001). Among the environmental factors (Table 5), except for the DO (F = 2.193, p = 0.089) and SD (F = 2.531, p = 0.099), whose effects were not significant (p > 0.05), the effects of other environmental factors were significant (p < 0.05). Remarkably, the effects of WD (F = 15.821 and p = 0.001) and WT (F = 31.110 and p = 0.001) were extremely significant (p < 0.01).
During the survey period, the dominant species exhibited the succession patterns from diatom to Cyanophyta with the sampling time changed from the dry season to wet season in Lake Wuchang (Figure 2), which was consistent with the study on the phytoplankton community structure of Lake Honghu, a large-scale flood lake in the middle and lower reaches of the Yangtze River (Wu et al., 2019). Structural equation modeling analysis suggested that water temperature, dissolved oxygen, and nutrition were the dominant factors affecting the phytoplankton structure (Zhang et al., 2020). We can verify the internal relationship between species and environmental factors with the detruded correspondence analysis, which can also intuitively reveal the ecological differentiation of different species. Results show that DO, pH, and NO3−-N were the key factors driving the succession of dominant species in the dry season (Figure 5). It has been demonstrated that lower water temperature and higher dissolved oxygen are more beneficial to the growth of diatoms (Behrenfeld et al., 2021), which lead to Cyclotella, Aulacoseira, and Synedra acus becoming the dominant species in the dry season, especially for Synedra acus with the dominance of 0.363. From the dry season to the normal season, as a result of the temperature and rainfall gradually increasing, the nitrogen and phosphorus nutrients of the lake area continuously increased with water runoff (Zhao et al., 2021). In addition, the increase in temperature promoted the growth and reproduction of some algae of cyanobacteria that need higher water temperature (Li et al., 2018), which led to Aphanizomenon and Leptolyngbya becoming the dominant species in the normal season. This is why NH4+-N, NO3−-N, and PO43--P were the key factors driving the changes in the dominant species in the normal season (Figure 5). Compared with other hydrological periods, they were generally characterized by high temperature and rainfall in summer, resulting in significantly higher water temperature and water depth (Table 6), which gradually became the key environmental factors affecting the phytoplankton community during the wet season. WD was closely related to the change in the water level, which has been proven to be a key environmental factor affecting the phytoplankton functional groups in Lake Poyang (Liu et al., 2019). In addition, climate (Yang et al., 2020), human activities (Antell et al., 2021), competition and predation among different biological communities, and other factors that affect the phytoplankton community structure and function should not be ignored (Forster et al., 2021).
The niche width represents the ability of aquatic life to use and adapt to the environmental resources of the aquatic ecosystem (Lörz et al., 2022). Therefore, these species with significant differences in the niche breadth are usually defined as widespread or generalized specie, and conversely as specialized species. The two aforementioned groups of phytoplankton (widespread and specialized species) are important indicator species of the community structure and water environment quality in the aquatic ecosystem (Kong et al., 2019). In this study, it was found that the niche width of Dinobryon was the lowest at 1.77, indicating that it was the specialized species during our survey of Lake Wuchang, which was further confirmed by the analysis results of dominance and occurrence frequency (Table 2). The species was dominant only in the dry season with a dominance of 0.046 and distributed in the three sampling sites of the lower lake with the least occurrence frequency of 37.5%. In a complex ecological environment, the competitive relationship between species fluctuates with environmental changes, resulting in the niche changes in the phytoplankton community in different periods (Britten and Sibert, 2020). The significantly higher dissolved oxygen (10.47 ± 1.22 mg/L) and lower water temperature (9.23 ± 0.62 °C) provided convenience for the growth of Dinobryon sp, which was better adapted to high dissolved oxygen and low water temperature (Logares et al., 2018); that is the reason why Dinobryon sp. proved to be the dominant species only in the dry season. Compared with the other dominant species, the niche width of Synedra acus varied from 3.55 to 6.65 (Table 2), indicating that Synedra acus had a stronger ability to compete for environmental resources and could be considered a widespread species in Lake Wuchang. In the wet season, an unbalanced nutrient supply arose with the increasing water temperature, which caused phytoplankton species to exhibit specific survival strategies, such as investment in growth, adaptation, tolerance of species to environmental stresses, solidification, and resource specialization (Breton et al., 2022), which led to cyanobacteria becoming the most common dominant species in the wet season. Moreover, Cyclotella and Aulacoseira were the dominant species only in the dry season, although both of them had higher niche widths (6.48 and 3.44, respectively), which might be due to their better adaptability to winter environmental conditions and less common resource use, so they were more likely to become the dominant species in a single habitat. Similar to the study on the niche of the dominant phytoplankton species in Lake Wuliangsuhai, Inner Mongolia, the dominant species from diatoms had a higher relative abundance and occupied a larger number of sampling sites and more resources in winter (Li et al., 2017).
When the niche overlap value of two dominant species is greater than 0.6, it indicates that they have significant similarities in resource use (Wathne et al., 2000). During the survey in Lake Wuchang, there were 30 dominant species pairs whose niche overlap value was greater than 0.6 (Table 4), indicating that these dominant species possessed or used environmental resources (such as dissolved oxygen, nutrients, and habitat space) with significant similarities (Wei et al., 2020). In the communities, the complex ecological relationship caused the niche of each species to share the basic niches of other species (Xiao et al., 2019). It was generally believed that the species with more similar classification status or living habits and having more similar ways of using environmental resources would face competition in the same habitat, which leads to a significant niche overlap in phytoplankton communities (Wen et al., 2021). However, we found that despite Leptolyngbya and Microcystis belonging to the same phylum meaning the relatively close classification status, their niche overlap value was only 0.025. Meanwhile, the niche width of Leptolyngbya (3.96) was much larger than that of another (1.99). We hypothesize that this was the result of the former’s stronger competitiveness for environmental resources, which limited the latter’s access to resources, especially when the environmental resources they jointly need were limited. Interestingly, Microcystis and Aphanizomenon were similar in the niche width value (1.99 and 2.13, respectively), indicating that they shared similar habitats (Bates et al., 2020); their niche overlap was as high as 0.955, which should be related to the fact that the way they used resources was complementary rather than complete; and they tended to coexist stably even though they shared similar habitat resources (Qiu et al., 2018). In addition, the niche overlap value of the dominant species pair may vary greatly under different habitats or resource states, even if they are composed of the same dominant species. For example, the dominant species pair consisted of Microcystis and Anabaena whose niche overlap values were significantly different in the normal season (0.280) and the wet season (0.706). Similar phenomena were found in the study of the dominant phytoplankton species niche in Lake Changhu, a shallow lake located in the middle and lower reaches of the Yangtze River, the same as Lake Wuchang. There were significant differences in the niche overlap of the same dominant species pair of Chlorella vulgaris and Chlorocoecum infusionum in autumn and winter, where the former was in an advantageous competitive position in summer, while the latter was on the contrary in winter (Wei et al., 2020).
The magnitude of the variance ratio value (VR) reflects the association between different species, and the difference between the test statistic W and the critical value of χ2 reflects the significance of correlation (Nagy-László et al., 2020). The VR value gradually changed from 1.49 to 2.27 across the dry to wet season, indicating that the ecosystem structure was relatively mature, with a positive succession trend (Ma Y et al., 2022). A total of 79.2% of 48 dominant species pairs were not significantly associated (p > 0.05), which may be related to the suitable growing season of the dominant species and the community structure difference in the hydrological season (Wu et al., 2019). In this study, the dominant phytoplankton species had a low probability of sympatric occurrence in the same season, and the interspecific association was independent of each other. In the normal season, six dominant species pairs showed a significant association, and most of them were the dominant species pairs of Leptolyngbya, which was similar to the results of the Huaxi Reservoir of the Guizhou Plateau (Ma et al., 2021). It was demonstrated that the structure of the phytoplankton community was relatively stable and formed a dynamic balance pattern in which the dominant species of Leptolyngbya shared the same food, nutrient composition, and space with others. There were two pairs of dominant species pairs with negatively significant associations in the wet season, indicating that the phytoplankton community structure changed significantly and its stability decreased from the normal to wet season, which might be related to the hydrological conditions, especially the fluctuation in water level in the lake, and heavy rainfall in summer causing drastic changes in the water environment, which may lead to poor stability of the phytoplankton community and easy fluctuation (Tao et al., 2021). In general, the dominant species with a high niche overlap in lake ecosystems have a higher proportion of positive associations in contrast to river systems (Li and Ma, 2022); however, we found that there was no close correlation between the interspecific association niche overlap of the two dominant species pairs of Synedra acus with Cyclotella and Pseudoanabaena with Dinobryon, whose niche overlap reached a significant level with values 0.941 and 0.972, respectively, but were insignificantly associated (Oik > 0.6) in the wet season. At the same time, we also found that the interspecific association between Anabaena, Microcystis, and Raphidiopsis were significant, while the values of their niche overlap were 0.273 and 0.363, respectively, which were lower than 0.6 (p < 0.05). We speculated that this might be related to the great seasonal variation in the phytoplankton community, which also reflected the limitation of the niche theory in explaining the interspecific association among the dominant phytoplankton species (Wu et al., 2019).
By observing the niche breadth and niche overlap of the dominant phytoplankton species and the habitat differences reflected by the community structure in Lake Wuchang across the different hydrological seasons, it was found that the phytoplankton community structure was relatively stable and had a trend of positive succession in Lake Wuchang during the whole hydrological period based on the interspecific association analysis. NH4+-N, NO3−-N, and PO43--P contents were the key factors driving the changes in the plankton community structure in Lake Wuchang, More importantly, the continuous high temperature or rainfall in summer would lead to the rise in water temperature and the increase in nutrients due to the surface runoff into the lake, which will accelerate the growth and reproduction of cyanobacteria, and may induce intermittent cyanobacterial blooms dominated by Microcystis, Pseudoanabaena, and Anabaena in the lake area. Therefore, we suggest implementing the green planting project of emergent plants in the lakeside zone and the entrance of the lake to form a green ecological barrier that will reduce and transform the pollutants. Moreover, it is possible to optimize the community structure of the aquatic life by planting plants, transplanting benthos, and proliferating filter-feeding fish such as the silver carp and bighead carp, so as to improve the water quality and substrate the environment of the lake. However, for dominant species with significant interspecific association reducing and the stability of the phytoplankton community decreasing from the normal season to the wet season, which might be related to the hydrological condition, especially the large fluctuation in the water level in the wet season, further relevant studies need to be conducted.
The original contributions presented in the study are included in the article/Supplementary Material; further inquiries can be directed to the corresponding authors.
ZM and DY conceived and designed the experiments; KC, LL, and FH performed the sample collection; ZM, YZ, and XL analyzed the data; and ZM and XL wrote the manuscript.
This study was supported by the National Key R&D Program of China (2019YFD0900603), the China Agriculture Research System of MOF and MARA (CARS-46), and the Central Public-Interest Scientific Institution Basal Research Fund, CAFS (2020XT1302 and 2020TD57).
The authors declare that the research was conducted in the absence of any commercial or financial relationships that could be construed as a potential conflict of interest.
All claims expressed in this article are solely those of the authors and do not necessarily represent those of their affiliated organizations, or those of the publisher, the editors, and the reviewers. Any product that may be evaluated in this article, or claim that may be made by its manufacturer, is not guaranteed or endorsed by the publisher.
Ahmad, M., Sharma, P., Rathee, S., Singh, H. P., Batish, D. R., Lone, G. R., et al. (2021). Niche width analyses facilitate identification of high-risk endemic species at high altitudes in Western Himalayas. Ecol. Indic. 126, 107653. doi:10.1016/j.ecolind.2021.107653
Antell, G. S., Fenton, I. S., Valdes, P. J., and Saupe, E. E. (2021). Thermal niches of planktonic foraminifera are static throughout glacial-interglacial climate change. Proc. Natl. Acad. Sci. U. S. A. 118 (18), e2017105118. doi:10.1073/pnas.2017105118
Arroyo, A. I., Pueyo, Y., Saiz, H., and Alados, C. L. (2015). Plant-plant interactions as a mechanism structuring plant diversity in a Mediterranean semi-arid ecosystem. Ecol. Evol. 5 (22), 5305–5317. doi:10.1002/ece3.1770
Barroeta, Z., Garcia, T., Uriarte, I., Iriarte, A., and Villate, F. (2022). Response of native and non-indigenous zooplankton to inherent system features and management in two Basque estuaries: A niche decomposition approach. Estuar. Coast. Shelf Sci. 272, 107878. doi:10.1016/j.ecss.2022.107878
Bates, O. K., Ollier, S., and Bertelsmeier, C. (2020). Smaller climatic niche shifts in invasive than non-invasive alien ant species. Nat. Commun. 11 (1), 5213. doi:10.1038/s41467-020-19031-1
Behrenfeld, M. J., Halsey, K. H., Boss, E., Karp-Boss, L., Milligan, A. J., and Peers, G. (2021). Thoughts on the evolution and ecological niche of diatoms. Ecol. Monogr. 91 (3), e01457. doi:10.1002/ecm.1457
Breton, E., Goberville, E., Sautour, B., Ouadi, A., Skouroliakou, D.-I., Seuront, L., et al. (2022). Multiple phytoplankton community responses to environmental change in a temperate coastal system: A trait-based approach. Front. Mar. Sci. 9, 914475. doi:10.3389/fmars.2022.914475
Britten, G. L., and Sibert, E. C. (2020). Enhanced fish production during a period of extreme global warmth. Nat. Commun. 11 (1), 5636–6. doi:10.1038/s41467-020-19462-w
Cervantes-Urieta, V. A., Trujillo-Tapia, M. N., Violante-González, J., Moreno-Díaz, G., Rojas-Herrera, A. A., and Rosas-Guerrero, V. (2021). Temporal dynamics of the phytoplankton community associated with environmental factors and harmful algal blooms in Acapulco Bay, Mexico. Lat. Am. J. Aquat. Res. 49 (1), 110–124. doi:10.3856/vol49-issue1-fulltext-2525
Chaparro-Pedraza, P. C., and de Roos, A. M. (2020). Ecological changes with minor effect initiate evolution to delayed regime shifts. Nat. Ecol. Evol. 4 (3), 412–418. doi:10.1038/s41559-020-1110-0
Chen, K., Meng, Z., Li, X., Zhu, T., Hu, F., Liu, L., et al. (2022). Phytoplankton community strycture and divering mechanism of its construction process in antumn in Zhelin Reservoir, Lake Poyang Basin. J. Lake. Sci. 34 (2), 433–444. doi:10.18307/2022.0206
Chen, Y., Yuan, Z., Bi, S., Wang, X., Ye, Y., and Svenning, J. C. (2018). Macrofungal species distributions depend on habitat partitioning of topography, light, and vegetation in a temperate mountain forest. Sci. Rep. 8 (1), 13589. doi:10.1038/s41598-018-31795-7
Ciancio, J. E., Yorio, P., Buratti, C., Colombo, G. Á., and Frere, E. (2021). Isotopic niche plasticity in a marine top predator as indicator of a large marine ecosystem food web status. Ecol. Indic. 126, 107687. doi:10.1016/j.ecolind.2021.107687
da Silva, M. N., Granzotti, R. V., de Carvalho, P., Rodrigues, L. C., and Bini, L. M. (2021). Niche measures and growth rate do not predict interspecific variation in spatial synchrony of phytoplankton. Limnology 22 (1), 121–127. doi:10.1007/s10201-020-00640-0
Feng, K., Wang, S., Wei, Z., Wang, Z., Zhang, Z., Wu, Y., et al. (2020). Niche width of above- and below-ground organisms varied in predicting biodiversity profiling along a latitudinal gradient. Mol. Ecol. 29 (10), 1890–1902. doi:10.1111/mec.15441
Forster, D., Qu, Z., Pitsch, G., Bruni, E. P., Kammerlander, B., Proschold, T., et al. (2021). lake ecosystem robustness and resilience inferred from a climate-stressed protistan plankton network. Microorganisms 9 (3), 549. doi:10.3390/microorganisms9030549
Gao, X., Wang, W., Ndayishimiye, J. C., Govaert, L., Chen, H., Jeppesen, E., et al. (2022). Invasive and toxic cyanobacteria regulate allochthonous resource use and community niche width of reservoir zooplankton. Freshw. Biol. 67 (8), 1344–1356. doi:10.1111/fwb.13921
Gu, L., Gong, Z.-w., and Li, W.-z. (2017). Niches and interspecific associations of dominant populations in three changed stages of natural secondary forests on Loess Plateau, PR China. Sci. Rep. 7 (1), 6604–6612. doi:10.1038/s41598-017-06689-9
Hofmann, P., Clark, A., Hoffmann, P., Chatzinotas, A., Harpole, W. S., and Dunker, S. (2021). Beyond nitrogen: phosphorus – estimating the minimum niche dimensionality for resource competition between phytoplankton. Ecol. Lett. 24 (4), 761–771. doi:10.1111/ele.13695
Hu, H. J., and Wei, Y. X. (2006). Freshwater algae in China—system, classification and ecology. Beijing: Science Press.
Kang, L. J., Xu, H., Zou, W., Zhu, G. W., Zhu, M. Y., Ji, B. F., et al. (2020). Influence of Potamogeton crispus on lake water environment and phytoplankton community structure. Huan Jing Ke Xue 41 (9), 4053–4061. doi:10.13227/j.hjkx.202002006
Kléparski, L., Beaugrand, G., Edwards, M., Schmitt, F. G., Kirby, R. R., Breton, E., et al. (2022). Morphological traits, niche-environment interaction and temporal changes in diatoms. Prog. Oceanogr. 201, 102747. doi:10.1016/j.pocean.2022.102747
Kong, J., Wang, Y., Warren, A., Huang, B., and Sun, P. (2019). Diversity distribution and assembly mechanisms of planktonic and benthic microeukaryote communities in intertidal zones of southeast fujian, China. Front. Microbiol. 10, 2640. doi:10.3389/fmicb.2019.02640
Levins, R. (2020). “Evolution in changing environments,” in Evolution in changing environments (Princeton: Princeton University Press).
Li, N., Li, J. Q., Li, G. W., Li, Y., Xi, B. D., Wu, Y. W., et al. (2018). Eutrophication status and regional difference analysis of typical lakes in China. Acta Hydrobiol. Sin. 42 (4), 854–864. doi:10.7541/2018.105
Li, Q., and Ma, Y. (2022). Analysis on niche and interspecific association of dominant species of phytoplankton in Tongzi River. J. Guizhou Normal Univ. Nat. Sci. 40 (2), 11–18. doi:10.16614/j.gunuj.zrb.2022.02.002
Li, X., Li, J. R., and Li, C. Y. (2017). Ecological niche analysis of dominant phytoplankton species in Wusuhai Lake, Inner Mongolia. J. Hydrol. 38 (6), 40–47. doi:10.15928/j.1674-3075.2017.06.006
Liu, J., Chen, Y., Li, M., Liu, B., Liu, X., Wu, Z., et al. (2019). Water-level fluctuations are key for phytoplankton taxonomic communities and functional groups in Poyang Lake. Ecol. Indic. 104, 470–478. doi:10.1016/j.ecolind.2019.05.021
Logares, R., Tesson, S. V., Canbäck, B., Pontarp, M., Hedlund, K., and Rengefors, K. (2018). Contrasting prevalence of selection and drift in the community structuring of bacteria and microbial eukaryotes. Environ. Microbiol. 20 (6), 2231–2240. doi:10.1111/1462-2920.14265
Lörz, A. N., Oldeland, J., and Kaiser, S., (2022). Niche breadth and biodiversity change derived from marine Amphipoda species off Iceland. Ecol. Evol. 12 (4), e8802. doi:10.1002/ece3.8802
Ma, C., Li, Z., Mwagona, P. C., Rabbany, A., and Bhadha, J. H. (2022). Spatial and seasonal dynamics of phytoplankton groups and its relationship with environmental variables in Lake Okeechobee, USA. J. Freshw. Ecol. 37 (1), 173–187. doi:10.1080/02705060.2022.2032852
Ma, Y., Li, Q., Pan, S., Kuang, P., and Jin, S. (2021). Dynamic analysis of niche and interspecific association of dominant phytoplankyon species in Huaxi Reservoir of Guizhou Plateau. J. Lake. Sci. 33 (3), 785–796. doi:10.18307/2021.0314
Ma, Y., Li, Q., Pan, S., Liu, C., Han, M., and Brancelj, A. (2022). Niche and interspecific associations of Pseudoanabaena limnetica – exploring the influencing factors of its succession stage. Ecol. Indic. 138, 108806–108811. doi:10.1016/j.ecolind.2022.108806
Machado, K. B., Bini, L. M., Melo, A. S., Andrade, A. T. d., Almeida, M. F. d., Carvalho, P., et al. (2022). Functional and taxonomic diversities are better early indicators of eutrophication than composition of freshwater phytoplankton. Hydrobiologia, 1–19. doi:10.1007/s10750-022-04954-1
McNaughton, S. J. N. (1967). Relationships among functional properties of Californian grassland. Nature 216 (5111), 168–169. doi:10.1038/216168b0
Nagy-László, Z., Padisák, J., Borics, G., Abonyi, A., B-Béres, V., and Várbíró, G. (2020). Analysis of niche characteristics of phytoplankton functional groups in fluvial ecosystems. J. Plankton Res. 42 (3), 355–367. doi:10.1093/plankt/fbaa020
Pantel, J. H., Engelen, J. M. T., and De Meester, L. (2022). Niche use and co-occurrence patterns of zooplankton along a strong urbanization gradient. Ecography 2022, 1–14. doi:10.1111/ecog.05513
Park, H. J., Park, T. H., Kang, H. Y., Lee, K.-S., Kim, Y. K., and Kang, C.-K. (2021). Assessment of restoration success in a transplanted seagrass bed based on isotopic niche metrics. Ecol. Eng. 166, 106239. doi:10.1016/j.ecoleng.2021.106239
Peng, X., Zhang, L., Li, Y., Lin, Q., He, C., Huang, S., et al. (2021). The changing characteristics of phytoplankton community and biomass in subtropical shallow lakes: Coupling effects of land use patterns and lake morphology. Water Res. 200, 117235. doi:10.1016/j.watres.2021.117235
Prati, S., Henriksen, E. H., Smalås, A., Knudsen, R., Klemetsen, A., Sánchez-Hernández, J., et al. (2021). The effect of inter- and intraspecific competition on individual and population niche widths: a four-decade study on two interacting salmonids. Oikos 130 (10), 1679–1691. doi:10.1111/oik.08375
Qiu, J. J., Wang, Y. X., Li, T. J., Sun, P., Zhang, M. M., Yu, Z. L., et al. (2018). Study on the niche and differentiation of major nekton species in the Zhoushan Changbai sea area. Acta eco. Sin. 38 (18), 6759–6767. doi:10.5846/stxb201803300680
Sales, L. P., Hayward, M. W., and Loyola, R. (2021). What do you mean by “niche”? Modern ecological theories are not coherent on rhetoric about the niche concept. Acta oecol. (Montrouge). 110, 103701. doi:10.1016/j.actao.2020.103701
Sánchez, M. L., Schiaffino, M. R., Graziano, M., Huber, P., Lagomarsino, L., Minotti, P., et al. (2021). Effect of land use on the phytoplankton community of Pampean shallow lakes of the Salado River basin (Buenos Aires Province, Argentina). Aquat. Ecol. 55 (2), 417–435. doi:10.1007/s10452-021-09835-8
Shan, K., Song, L., Chen, W., Li, L., Liu, L., Wu, Y., et al. (2019). Analysis of environmental drivers influencing interspecific variations and associations among bloom-forming cyanobacteria in large, shallow eutrophic lakes. Harmful Algae 84, 84–94. doi:10.1016/j.hal.2019.02.002
Tao, M., Yue, X. J., Yue, S., Dai, L. N., Han, W. W., Wang, Y. M., et al. (2021). Phytoplankton community structure and cyanobacteria bloom risk of reservoirs in hilly regions of Sichuan Province based on dominant species and interspecific association. Sheng Tai Xue Bao 41 (23), 9457–9469. doi:10.5846/stxb202008182155
Tarjuelo, R., Morales, M. B., Arroyo, B., Mañosa, S., Bota, G., Casas, F., et al. (2017). Intraspecific and interspecific competition induces density-dependent habitat niche shifts in an endangered steppe bird. Ecol. Evol. 7 (22), 9720–9730. doi:10.1002/ece3.3444
Tian, Y., Jiang, Y., Liu, Q., Xu, D., Liu, Y., and Song, J. (2021). The impacts of local and regional factors on the phytoplankton community dynamics in a temperate river, northern China. Ecol. Indic. 123, 107352. doi:10.1016/j.ecolind.2021.107352
Tilman, D. (2004). Niche tradeoffs, neutrality, and community structure: a stochastic theory of resource competition, invasion, and community assembly. Proc. Natl. Acad. Sci. U. S. A. 101 (30), 10854–10861. doi:10.1073/pnas.0403458101
Várbíró, G., B-Béres, V., Abonyi, A., Borics, G., Padisák, J., and Nagy-László, Z. (2020). Analysis of niche characteristics of phytoplankton functional groups in fluvial ecosystems. J. Plankton Res. 42 (3), 355–367. doi:10.1093/plankt/fbaa020
Wakil, S., and Justus, J. (2022). The ‘niche’ in niche-based theorizing: much ado about nothing. Biol. Philos. 37 (2), 10–21. doi:10.1007/s10539-022-09839-0
Wang, K., Razzano, M., and Mou, X. (2020). Cyanobacterial blooms alter the relative importance of neutral and selective processes in assembling freshwater bacterioplankton community. Sci. Total Environ. 706, 135724. doi:10.1016/j.scitotenv.2019.135724
Wathne, J. A., Haug, T., and Lydersen, C. (2000). Prey preference and niche overlap of ringed seals Phoca hispida and harp seals P. groenlandica in the Barents Sea. Mar. Ecol. Prog. Ser. 194, 233–239. doi:10.3354/meps194233
Wei, Z., Chai, Y., Luo, J., Guo, K., Tan, F., Yang, D., et al. (2020). Seasonal succession and ecological niche analysis of the dominant species of phytoplankton in Changhu Lake. Acta Hydrobiol. Sin. 44 (3), 612–621. doi:10.7541/2020.075
Wen, X., Yang, K., Piñero, J. C., and Wen, J. (2021). Contrasting behavioral and electrophysiological responses of eucryptorrhynchus scrobiculatus and E. brandti (Coleoptera: Curculionidae) to volatiles emitted from the tree of heaven, ailanthus altissima. Insects 12 (1), 68. doi:10.3390/insects12010068
Wu, J., Xu, N., Zhang, W., Xu, L., Hu, J., and Zhu, X. (2019). Seasonal analysis of the niche and interspecific association of dominant species of phytoplankton in the Dinghai Moat, Zhoushan City. J. Lake. Sci. 31 (2), 429–439. doi:10.18307/2019.0212
Wu, N., Qu, Y., Guse, B., Makarevičiūtė, K., To, S., Riis, T., et al. (2018). Hydrological and environmental variables outperform spatial factors in structuring species, trait composition, and beta diversity of pelagic algae. Ecol. Evol. 1 (00), 2947–2961. doi:10.1002/ece3.3903
Wu, Y., Guo, P., Su, H., Zhang, Y., Deng, J., Wang, M., et al. (2021). Seasonal and spatial variations in the phytoplankton community and their correlation with environmental factors in the Jinjiang River Estuary in Quanzhou, China. Environ. Monit. Assess. 194 (1), 44. doi:10.1007/s10661-021-09697-5
Xiao, W., Laws, E. A., Xie, Y., Wang, L., Liu, X., Chen, J., et al. (2019). Responses of marine phytoplankton communities to environmental changes: New insights from a niche classification scheme. Water Res. 166, 115070. doi:10.1016/j.watres.2019.115070
Xu, D., Wang, H., Han, D., Chen, A., and Niu, Y. (2021). Phytoplankton community structural reshaping as response to the thermal effect of cooling water discharged from power plant. Environ. Pollut. 285, 117517. doi:10.1016/j.envpol.2021.117517
Yan, M., Chen, S., Huang, T., Li, B., Li, N., Liu, K., et al. (2020). Community compositions of phytoplankton and eukaryotes during the mixing periods of a drinking water reservoir: Dynamics and interactions. Int. J. Environ. Res. Public Health 17 (4), 1128. doi:10.3390/ijerph17041128
Yang, X., Tan, Y., Li, K., Zhang, H., Liu, J., and Xiang, C. (2020). Long-term changes in summer phytoplankton communities and their influencing factors in Daya Bay, China (1991-2017). Mar. Pollut. Bull. 161, 111694. doi:10.1016/j.marpolbul.2020.111694
Yang, Y., Chen, H., Abdullah Al, M., Ndayishimiye, J. C., Yang, J. R., Isabwe, A., et al. (2022). Urbanization reduces resource use efficiency of phytoplankton community by altering the environment and decreasing biodiversity. J. Environ. Sci. 112, 140–151. doi:10.1016/j.jes.2021.05.001
Zhang, Z., Mammola, S., McLay, C. L., Capinha, C., and Yokota, M. (2020). To invade or not to invade? Exploring the niche-based processes underlying the failure of a biological invasion using the invasive Chinese mitten crab. Sci. Total Environ. 728, 138815. doi:10.1016/j.scitotenv.2020.138815
Keywords: phytoplankton, niche, interspecific association, dominant species, Lake Wuchang
Citation: Meng Z, Chen K, Liu L, Hu F, Zhu Y, Li X and Yang D (2022) Niche and interspecific association with respect to the dominant phytoplankton species in different hydrological periods of Lake Wuchang, China. Front. Environ. Sci. 10:985672. doi: 10.3389/fenvs.2022.985672
Received: 04 July 2022; Accepted: 08 November 2022;
Published: 24 November 2022.
Edited by:
Li Zeng, China Institute of Water Resources and Hydropower Research, ChinaReviewed by:
Tan Lu, Institute of Hydrobiology (CAS), ChinaCopyright © 2022 Meng, Chen, Liu, Hu, Zhu, Li and Yang. This is an open-access article distributed under the terms of the Creative Commons Attribution License (CC BY). The use, distribution or reproduction in other forums is permitted, provided the original author(s) and the copyright owner(s) are credited and that the original publication in this journal is cited, in accordance with accepted academic practice. No use, distribution or reproduction is permitted which does not comply with these terms.
*Correspondence: Xuemei Li, eG1saUB5ZmkuYWMuY24=; Deguo Yang, eWFuZ2RnQHlmaS5hYy5jbg==
Disclaimer: All claims expressed in this article are solely those of the authors and do not necessarily represent those of their affiliated organizations, or those of the publisher, the editors and the reviewers. Any product that may be evaluated in this article or claim that may be made by its manufacturer is not guaranteed or endorsed by the publisher.
Research integrity at Frontiers
Learn more about the work of our research integrity team to safeguard the quality of each article we publish.