- 1Department of Silviculture and Agroforestry, Dr YSP University of Horticulture and Forestry, Solan, India
- 2Division of Temperate Animal Husbandry, IVRI, Mukteshwar, India
- 3Department of Basic Sciences, Dr YSP University of Horticulture and Forestry, Solan, India
Sustainable forest management is the key to biodiversity conservation, flow of resources and climate change mitigation. We assessed the impact of various forest management regimes (FMRs): legal felling series [(reserve forest (RF), demarcated protected forest (DPF), un-demarcated protected forest (UPF), co-operative society forest (CSF) and un-classed forest (UF)] on biodiversity conservations and carbon storage in Acacia catechu Willd. Dominated northern tropical dry deciduous forest ecosystems in Nurpur Forest Division of north-western Himalaya, India. The study revealed significant variations in floristic composition, biodiversity indices, population structure and C storage potential among different forest management regimes. The RF and DPF were found to be rich in species diversity and richness whereas the Simpson dominance index for trees and shrubs was maximum in UF and UPF, respectively. The diversity of understory herbs were higher in CSF and UF. The maximum density of seedlings, saplings and poles were recorded in RF followed by DPF and UPF, whereas the minimum density was found in CSF. The tree C density (69.15 Mg C ha−1) was maximum in UF closely followed by RF; whereas the minimum was recorded in CSF (33.27 Mg C ha−1). The soil C density was maximum in RF (115.49 Mg C ha−1) and minimum in CSF (90.28 Mg C ha−1). Similarly, the maximum total ecosystem C density was recorded in RF (183.52 Mg C ha−1) followed by DPF (166.61 Mg C ha−1) and minimum in CSF (126.05 Mg C ha−1). Overall, UF management regimes were shown to have a greater capacity for C storage in vegetation, whereas strict FMRs, such as RF and DPF, were found to be more diverse and have a higher soil and ecosystem carbon density. The study established that in the midst of climate and biodiversity emergencies, it is urgent to maintain, protect and strengthen the network of RF and DPF FMRs for biodiversity conservation, climate change adaptation and mitigation.
Introduction
Tropical forests once covered over half of the total area of the tropics (Janzen, 1988), but have dwindled to a great extent during the last decennia (Sagar and Singh, 2005). On the global scale, out of the total coverage of forests, 52% are tropical forests (Singh and Singh, 1988). In India, forests cover nearly 21.71% of the country’s total geographical area (FSI, 2021) and tropical forests account for 86% of the total forest land (Singh and Singh, 1988). Tropical forests also harbor maximum diversity of plant species found on the earth (WCMC, 1992). Among the different forest types, tropical forests contribute to 25% of the total terrestrial carbon stock and are major sink (Bonan, 2008), playing major roles in regulating climate dynamics on earth (Lewis et al., 2009; Zhou et al., 2013). Thus, tropical forests play a critical role in the global carbon (C) balance, biodiversity conservation and offer several other valuable environmental services. In addition to this, these ecosystems are a stockpile of resources and sustain the lives of millions of people across the globe. Tropical dry deciduous forests (TDFs) are particularly indispensable for sustaining vulnerable households during hardships (Blackie et al., 2014). These forests are rich in medicinal and economically important plants. But TDFs are dwindling at an alarming rate (Blackie et al., 2014) due to excessive exploitation and land-use change, biological invasion (Kumar et al., 2021) and climate change. Thus, a study on species composition and diversity, carbon sink potential, population structure and soil health status of northern TDFs is ecologically significant besides being useful in forest management.
Terrestrial ecosystems, particularly TDFs with their abundant biodiversity and C storage capacity (Kothandaraman, et al., 2020) are major C sinks playing a dynamic role in the mitigation of global warming. In a forest ecosystem, C is stored in vegetation carbon, soil carbon, and detritus pools (Lee et al., 2014; Herault and Piponiot, 2018; Lafleur et al., 2018). Therefore, the estimation of forest ecosystem C storage is of utmost importance to understand global carbon cycle and for framing strategies for mitigating the likely impacts of climate change (Sun and Liu, 2020). The global forests hold 662 Gt C stock of which 300 Gt is in soil organic matter, 295 Gt in living biomass and 68.0 Gt in detritus, which accounts for the sequestration of 2.4 pentagrams of C every year (FAO, 2020). As a result, preserving or restoring standing forest biomass is a relatively low-hanging fruit in terms of reducing human GHG emissions (Seddon et al., 2020). Forest management is thus required which aims at the long-term storage of biomass and soil. Defining the C storage/sequestration capacity of forest ecosystems always remains a major strategy for reducing CO2 concentrations in the atmosphere (Forster et al., 2021). Particularly, protected forests (PF) are one of the major C sinks in the forest ecosystems, although their efficiency varies with continuance (Collins and Mitchard, 2017).
The type of forest management regimes (FMRs) have a key role in maintaining structural diversity of forests and also helps in protection of forests (Gao et al., 2014; Chazdon et al., 2017; Dieler et al., 2017; Mancosu et al., 2018; Reise et al., 2019). Stand diversity is recognized as a significant aspect of forest ecosystem functioning such as primary production (Paquette and Messier 2010; Liang et al., 2016), stability of wood production (Jucker et al., 2014), resistance to environmental disturbances (Pretzsch et al., 2013; Jactel et al., 2017) and nutrient cycling (Richards et al., 2010; Handa et al., 2014). A forest stand with greater diversity is more efficient in providing a wide range of provisioning services (Gamfeldt et al., 2013; Forrester and Bauhus, 2016), more resistant to various disturbances, holds sufficient soil nutrients and has a considerably greater prediction value for tree C (Liang et al., 2016; Van et al., 2017; Zhang et al., 2017; Liu et al., 2018) than the stand with low species diversity (Wardle, 2001; Lefcheck et al., 2015; Jactel et al., 2017).
The intrinsic role of forests in C management and biodiversity management is an additional service that sustainable forest management can offer leading to earning financial incentives through REDD+ in developing countries. Being a signatory to the UNFCCC, India participates in REDD+ through the Forest Carbon Partnership Facility program. In India, forests cover 21.71% of the land area (FSI 2021), are legally managed under the Indian Forest Act of 1927 and are classified as reserve forest (RF), PF and un-classed forest (UF) based on degree of protection provided for the conservation or restoration. In RF, utilization is generally strictly prohibited, while the PF have a limited degree of protection. The UF is an area recorded as a forest but not included in RF or PF category (IFA, 2016). In India, more than half (57.05%) of the total recorded forest area falls under RF, whereas less than one-third (27.38%) of the forests are designated as PF and 15.57% as UF (FSI, 2021). The PF are further divided into demarcated protected forest (DPF) and un-demarcated protected forest (UPF) based on boundary demarcation. Further, in Himachal Pradesh, a small hill state situated in north-western Himalayas, some other category of forest exists, such as co-operative society forest (CSF) under Kangra Forest Co-operative Scheme, 1938, where local people are involved in management and utilization of certain forest. The recorded forest area of Himachal Pradesh is 37,948 sq km, of which the majority of the forest area (76.12%) is under PF (DPF-33.87% + 42.25% UPF), 18.05% is under UF, 4% under RF, and 0.05% is managed under CSF (FSI, 2021). In Himachal Pradesh, the tropical dry deciduous forest occurs up to an altitude of 1,200 m in the lower hills and accounts for about 14.54% of the total forest cover of state (FSI, 2019).
Acacia catechu, commonly known as Khair, is a multipurpose moderate-sized deciduous tree primarily occurring in the tropical moist deciduous forests, dry tropical forests and tropical thorn forests (Champion and Seth, 1968). In Himachal Pradesh, Khair is widely distributed in Mandi, Hamirpur, Kangra (Nurpur), Solan, Sirmour, Una and Bilaspur districts. However, it is most abundantly found in the Nurpur Forest Division which is maintained under Khair Working Circle because of favorable climatic and edaphic condition. It is an economically significant plant that is commercially exploited for katha (catechin) and cutch (Catechu tannic acid) (Lakshmi and Kumar, 2011). Besides its commercial importance, the rural communities depend on this tree for fulfilling their daily needs of fuel, fodder, building material, etc. Owing to their high commercial and social value, Khair forests are susceptible to a high degree of anthropogenic pressure, especially illicit logging. These disturbances, tend to change/influence the species composition, stand structure, C cycling of the forest ecosystem (Chauhan, 1999; Yohannes et al., 2015) along with biomass loss (Gautam and Mandal, 2016), and a decline in the plant diversity and other associated vegetation (Sapkota et al., 2010; Gautam and Mandal, 2016). Even a minor perturbation in these forest ecosystems could have a substantial impact on biodiversity, species composition and forests C-storage capacity. Studies carried out in various parts of the world have revealed that the stand diversity and C stock of forest ecosystems are influenced by several factors, of which the intensity and frequency of anthropogenic disturbances have been reported to play a significant role in regulating the regeneration dynamics, structure and floristic composition of forest ecosystems (Upadhaya et al., 2008; Mir and Upadhaya, 2017). Hence, the Khair forests in the foothills of Shiwalik of the Nurpur forest division are legally managed under different FMRs i.e., RF, DPF, UPF, CSF, and UF based on the management objectives and location of forests with respect to distance from human habitat (Working Plan, 2013). The level of disturbances in these forest categories varied with their protection status and rights to utilize resources. These FMRs provide varied degree of protection from encroachment to Khair Forest and play a crucial role in the maintenance of species diversity and C stock. Consequently, the occurrence of Khair dominated forests in Nurpur Forest Division makes the region more appropriate for knowing the influence of different FMR’s and disturbances which ultimately help to determine the conservation implication (Working Plan, 2013). Further, management and cultural operations in a forest are the most important factors that influence the species diversity, C content, stand characteristics, and regeneration process. However, the lack of monitoring and evaluation of various management regimes is a great hindrance to understanding the effectiveness and impact of FMRs on these ecosystem services. As these forests are continuously subjected to exploitation and anthropogenic pressure, therefore it has become imminent to have an in-depth understanding of the impact of management practices on biodiversity and C storage capacity. Despite their vast ecological and socio-economic significance so far, there has not been any comprehensive study that examines the biodiversity and C stock in relation to various management regimes, thus creating a hindrance to set conservation implications for C management, biodiversity conservation and sustainable utilization that will eventually enhance the ecological health of the forests and increase the flow of ecosystem services (Pearce, 2001; Malhi et al., 2008; Paudel and Sah, 2015). Therefore, this study was carried out with the objectives to 1) quantify the differences in the C stock of the woody biomass and soil, 2) estimate biodiversity conservation and 3) study population distribution and stand structure, under different FMRs in the Nurpur Forest Division of Himachal Pradesh in India.
Materials and methods
Study area
The present study was carried out in the foothills of Shiwalik ranges of Himachal Pradesh that lies between the latitudes 75.77°–76.02° N and longitudes 32.23°–32.40° E with elevation ranges from 500 to 700 m above mean sea level (asl). The area has a hot dry summer from April to June and monsoon season from July to September, followed by a cool and relatively dry winter. The texture of the soil is sandy loam, with sand stones rock type. The scientific management of Khair forests in Himachal Pradesh was initiated in 1879 and was traditionally managed through selection felling, i.e., harvesting of an individual tree above certain diameter and leaving a few mother trees for regeneration. The interval and diameter for harvest varied according to species with no tree less than 10 cm diameter harvested. However, green felling has been banned vide orders of the Hon’ble Supreme Court of India dated 12th December 1996 and February 2000 and reinitiated on experimental basis in the selected forest compartments vide the Hon’ble Supreme court order of 2018 through which it has been proposed that 80% of trees of A. catechu of 25 cm diameter and above be felled and the remaining 20% (>20 cm) retained as mother trees. Therefore, five differently managed FMRs viz., Reserve Forest (RF), Demarcated Protected Forest (DPF), Un-demarcated Protected Forest (UPF), Co-operative Society Forest (CSF) and Un-class Forest (UF) in Khair Working Circle of Nurpur Forest Division of Himachal Pradesh, India were selected (Figure 1) so as to represent the entire range of canopy conditions and management regimes based on the satellite and field observations. The detailed description of each FMRs is tabulated in Table 1.
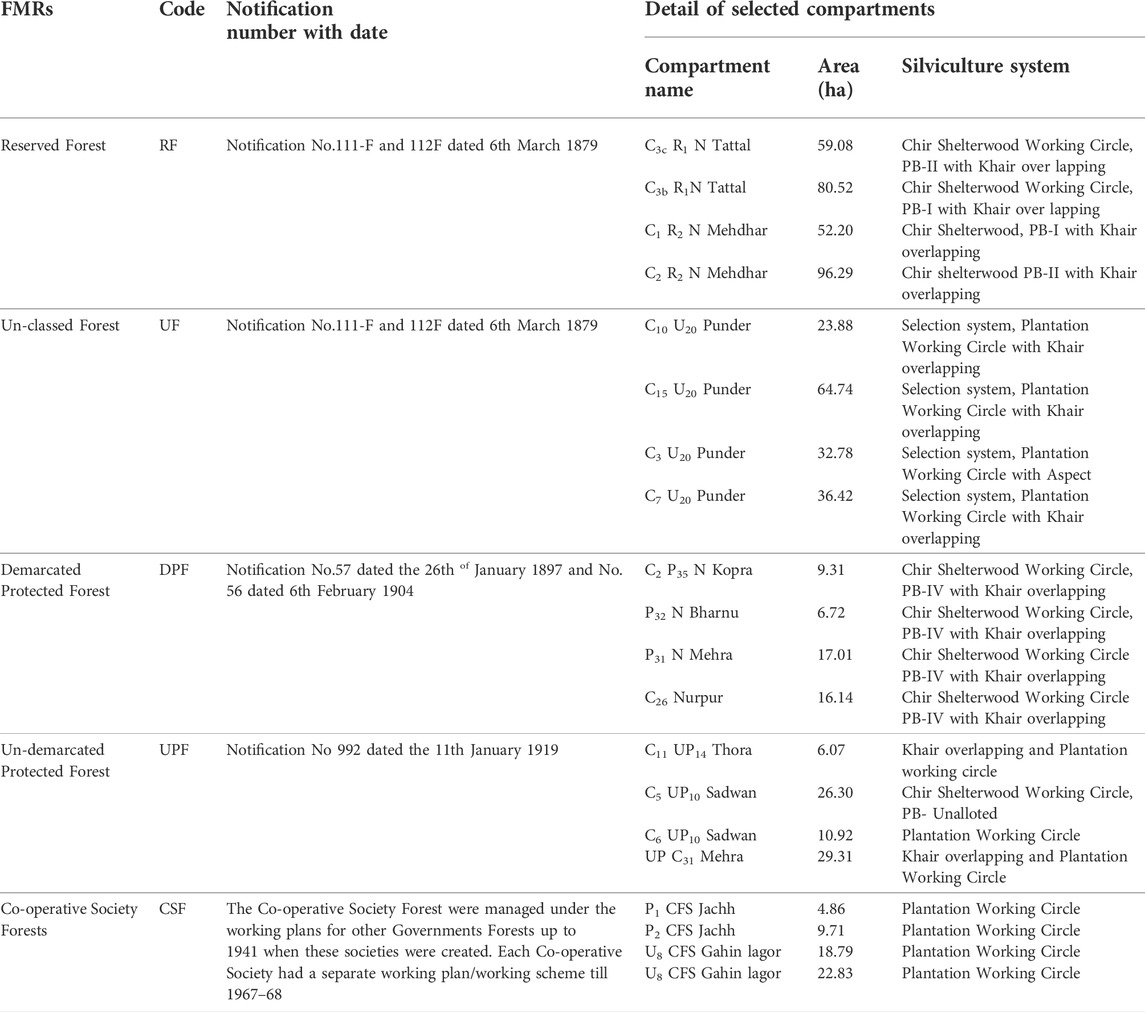
TABLE 1. Detailed description of each FMRs of Khair Working Circle in Nurpur Forest Division, Himachal Pradesh, India (Source: Working Plan, 2013).
Species diversity and stand structure
In each FMRs, four separate compartments were selected for executing the present study. Under each compartment, a plot of 6.25 ha (250 m × 250 m) was demarcated for a detailed study. Thus, there were 20 stands (4 compartments × 5 Forest regimes). In each forest stand, four sample plots of 0.1 ha (31.62 m × 31.62 m) were laid out in the main plot for trees. In each 0.1 ha of sample plot, two subplots of size 5 m × 5 m were laid out. Further, in each shrub plot, two subplots of size 1 m × 1 m were laid out to study the herb-related traits. Standard measurement procedures were followed for taking primary observations, such as tree height and diameter at breast height (DBH). Tree height was measured with a Ravi altimeter (Blue Leiss Hypsometer). The diameter was measured by taking two measurements of stems (major and minor axis) at breast height (1.37 m) with tree callipers, and their mean was calculated as the DBH of a tree. To analyze the tree structure, the individuals were categorized into six diameter classes viz., 10–15, 15–20, 20–25, 25–30, 30–35, >35 cm. A regeneration survey was also carried out in a 2 m × 2 m plot by recording the number of seedlings (<0.5 m) and saplings (0.5–2 m) (Schwab et al., 2022). Trees, saplings and seedlings within each plot were counted to determine their density. The specimens were prepared and identified at Department of Forest Products, Dr. YSP University of Horticulture and Forestry, Nauni, Solan, Himachal Pradesh.
Vegetation indices
Community diversity was assessed using non-parametric measures such as diversity indices (Magurran, 1988). Simpson diversity index (D′) (Simpson, 1949), Margalef index of species richness (MI) (Margalef, 1958), Shannon–Weaver diversity index (H′) (Shannon and Weaver, 1963) and Pielou equitability (Ep) (Pielou, 1966) were calculated using the formulae mentioned below.
Shannon-Weaver Index of diversity (H′) = -∑pi In pi.
Simpson’s diversity index (D′) = 1-∑pi2
Margalef’s Index of richness (MI) =(S-1)/lnN.
Pielou equitability (Ep) =
pi = ni/n (ni = IVI value of ith species, n = Total IVI value).
S = total number of species.
N = total density per ha
ln = log natural.
Estimation of tree C density
To determine the tree C stock, the above-ground tree biomass (AGB) and the volume (m3 ha−1) of each species were first estimated using volumetric equations (Table 2) (FSI, 1996). The estimated growing stock volume of the tree was then converted to AGB (Mg ha−1) by multiplying the growing stock volume with specific wood density (g cm−3) of the respective species (Rajput et al., 1996). Global wood density database was used for wood specific gravity of each species (Chave et al., 2009; Zanne et al., 2009). The below-ground tree biomass (BGB) (fine and coarse roots) was estimated using regression equation (Cairns et al., 1997):
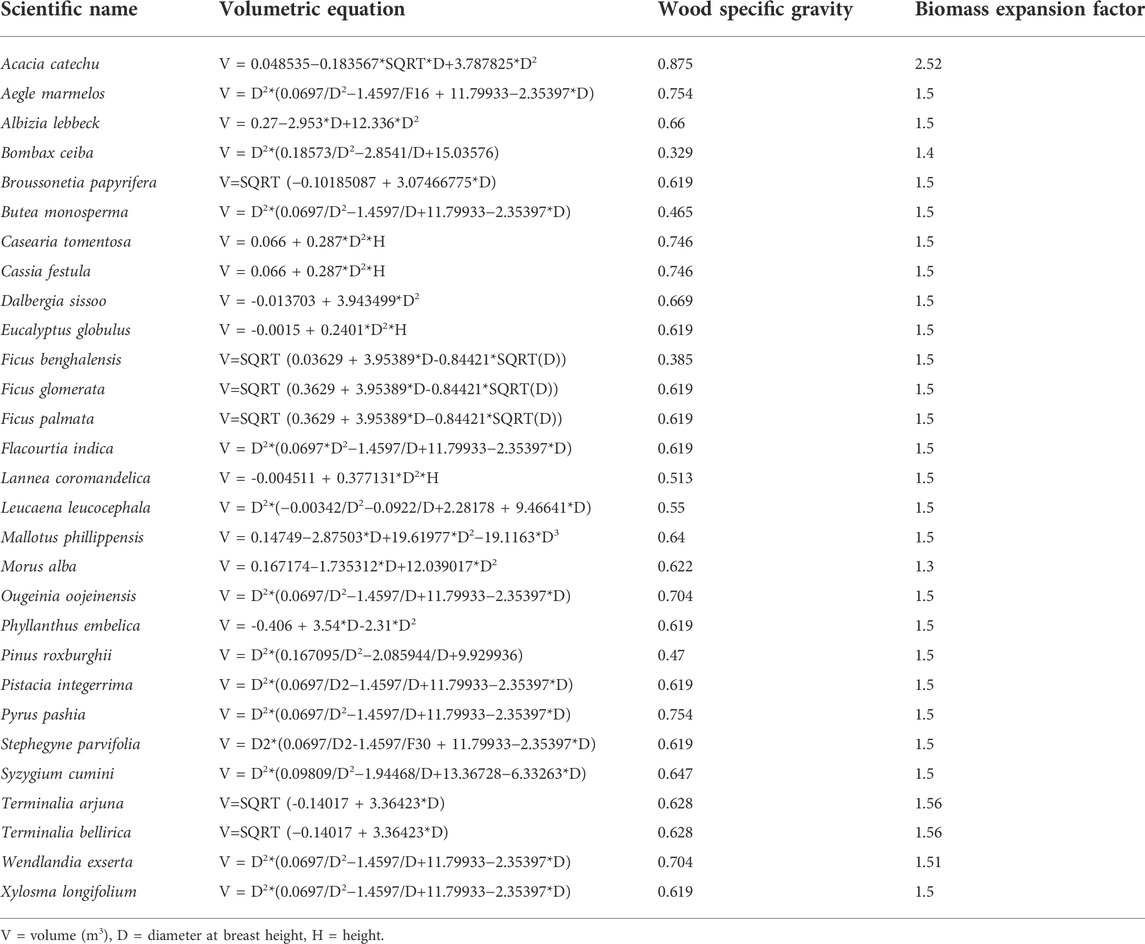
TABLE 2. List of volumetric equations and species-specific gravity, biomass expansion factor used in present study (Source: FSI, 1996).
The total tree biomass (Mg ha−1) is the sum of AGB and BGB.
The C density of the tree species was then determined as:
where C is the carbon concentration of the corresponding vegetation. A universal coefficient of 0.475 was used for tree C estimation (Raghubanshi, 1991; Singh and Chand, 2012), indicating approximately 47.5% of C in dry plant biomass (Westlake, 1963).
Understory C density
The understory biomass, i.e., shrubs (woody species other than trees with less than 1 m height) and herbs, was estimated by randomly laying 5 m × 5 m and 1 m × 1 m quadrat for shrubs and herbs, respectively. The shrub biomass was estimated by harvesting method, where 10% of each species of shrub was harvested and the fresh weight of the harvested sample was measured immediately with an electronic balance in the field. For the herbaceous biomass, all the herbaceous vegetation falling in 1 m × 1 m quadrat was harvested and the fresh weight was measured immediately in the field. The representative samples of both herbs and shrubs were taken to the laboratory, where they were oven-dried at 65°C for 48 h. The dry weight of the sample was then extrapolated to find out the entire biomass of shrub and herb samples. The C stock in the understory (shrubs and herbs) was considered to be 50% of dry weight (Dar and Sundarapandian, 2015). Therefore, the understory C stock was determined by multiplying the dry weight with a coefficient of 0.50.
Soil C density
Soil carbon density (SCD) was then calculated based on the bulk density and soil organic carbon concentration using the following equation:
SCD = [Soil bulk density (g cm−3) × Soil depth (0–40 cm) × soil organic carbon] × 100 (Nelson and Sommers, 1996).Where, the soil organic carbon was calculated using Walkley and Black (1934). For bulk density, undisturbed soil samples were collected with a soil corer of known volume (31.4 cm3) and oven-dried at 105°C for 72 h to determine the dry weight.
Total vegetation and ecosystem C density (Mg ha−1)
The total vegetation C density (VCD) was taken as the sum of C content in trees, shrubs and herbs whereas the total ecosystem C density (ECD) was taken as the sum of VCD and soil pool i.e., SCD.
Statistical analysis
Species diversity matrices were calculated using the software Past 3.1 program (version 3.1; Oyvind Hammer, Natural History Museum, University of Oslo) (Hammer et al., 2001). SPSS Software package (ver. 20.0; SPSS, Chicago, IL) was used for statistical analysis and the analysis of variance (ANOVA) was performed using Duncan’s Multiple Range Test to test the significance of C density of different FMRs.
Results
Biodiversity of Khair dominated forests
The diversity indices of different FMRs of Khair Working Circle is depicted in Table 3. The maximum number of tree species representations was recorded in the RF (17) while the minimum number was found in UPF and UF. Under the shrub category, the maximum number of shrub species (9.0) was recorded in RF; however, its variation with the other forest regime was very less. In the herb category, the maximum number of herbs (6) was recorded in UF while the minimum number of herb species was found in the DPF (3). Similarly, the maximum tree density (399.17 N ha−1) and herb density (43,700.00 N ha−1) among the different FMRs were observed in RF. However, the shrub forms were having the maximum density (3,725.00 N ha−1) under the DPF management regimes. A similar pattern for basal area (m2 for trees and cm2 for understory vegetation) was observed under herb, shrub and tree layers among different FMRs.
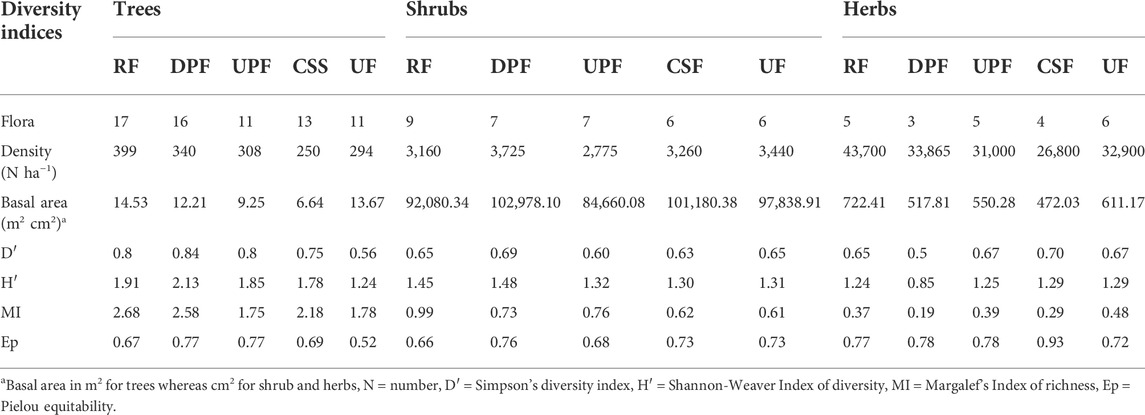
TABLE 3. Values of various diversity indices in forests under different FMRs in Khair Working Circle of Nurpur Forest Division, Himachal Pradesh, India.
A cursory look at the tree component (Table 3) of the Khair Working Circle revealed that the different biodiversity indices viz., Simpson’s diversity index (D′), Shannon-Weaver Index of diversity (H′), Pielou equitability (Ep), and Margalef’s Index of richness (MI) were having their highest values in DPF/RF categories as compared to UPF, CSF and UF. However, D′ (0.56), H′ (1.24) and Ep (0.52) exhibited their minimum values under UF. Ep demonstrated that the tree species are distributed equitably in DPF and UPF categories. For the shrub component (Table 3), not much variation was found for D′ under different FMRs. However, the H′ revealed the highest values under RF (1.45) and DPF (1.48) than under UPF (1.32), CSF (1.30) and UF (1.31). Similarly, MI (0.99) exhibited the maximum species richness under RF management regime. Shrub species in the Khair Working Circle demonstrated maximum value of Ep (0.76) under DPF. For herbs, both D′ and H′ indicated the highest value under CSF and UF. MI also showed its highest value under UF (0.48) and Ep was maximum under CSF (0.93) whereas the other FMRs revealed little or no variability for Ep.
Stand and population structure (N ha−1)
Figure 2 depicts the stand structure under the different FMRs. The distribution of trees under different FMRs barring UF exhibited the reverse J-shaped pattern (a characteristic feature of De liocourt’s law) from 15–20 cm diameter class onward. Among all the FMRs, the RF had more similarity to De liocourt’s law in terms of stem progression from 15–20 cm to >35 cm diameter class. The distribution of stems in the diameter classes viz., 10–15, 20–25, 25–30, and 30–35 cm was the greatest in RF whereas the distribution of stems in the diameter class 15–20 cm was the highest in DPF FMRs. Further, the maximum stem density in the diameter class >35 cm and the minimum in diameter class 10–15 cm were found in UF. CSF had the lowest stem distribution among all diameter classes, except for 10–15 cm diameter class.
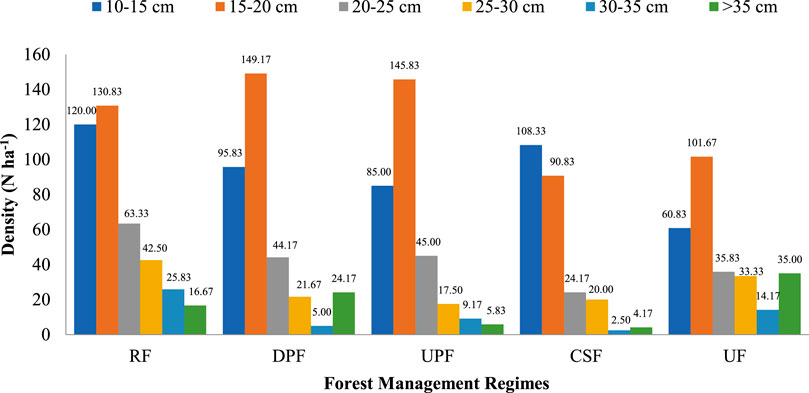
FIGURE 2. Stand structure of Khair Working Circle under different FMRs in Nurpur Forest Division, Himachal Pradesh, India.
The distribution of seedlings, saplings and poles under different FMRs is depicted in Figure 3. A reverse J shape curve can be clearly noticed under RF, DPF and UPF. The maximum number of seedlings, saplings and poles was recorded in RF followed by DPF, UPF, UF, and CSF, respectively in the descending order. Additionally, the proportion of Khair individuals alone in comparison to other species at pole stage was higher in UF than in the other FMRs of Khair Working Circle.
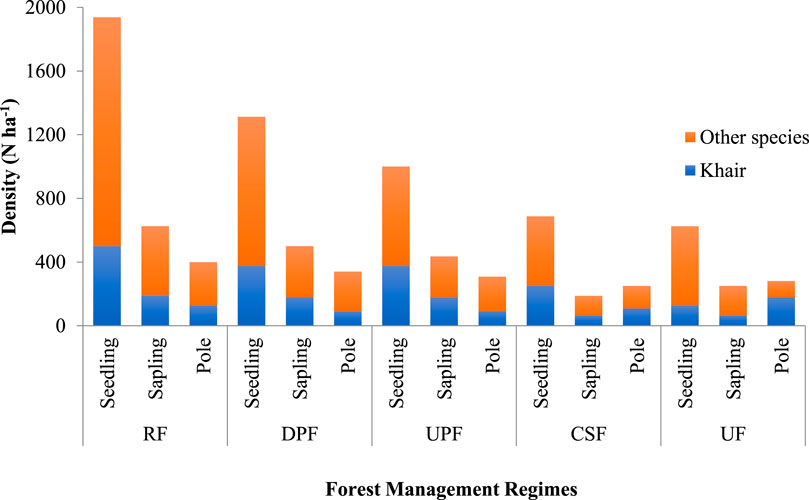
FIGURE 3. Population structure of Khair Working Circle under different FMRs in Nurpur Forest Division, Himachal Pradesh, India.
Carbon density (Mg ha−1) of Khair dominated forests
Among different FMRs, UF had the highest AG tree C density (46.03 Mg C ha−1), whereas the lowest AG C density (21.51 Mg C ha−1) was recorded in CSF (Table 4). Similarly, the BG and the total tree C density were highest in UF (23.12 and 69.15 Mg C ha−1). While the lowest value was recorded in CSF (11.76, 33.27 Mg C ha−1). However, the different FMRs i.e., DPF and UPF; UPF and CSF on the other hand remained statistically alike. The C density also varied significantly in shrub component of different FMRs. DPF and CSF displayed maximum AG shrub C density (1.62 Mg C ha−1). The BG shrub C density was maximum in DPF (0.83 Mg C ha−1) and the lowest in UPF (0.52 Mg C ha−1). The minimum value of total shrub C density was recorded in UPF (1.67 Mg C ha−1). The maximum C density for herb components (AG, BG and TC) was found in RF (0.52 Mg C ha−1). Overall, VCD under different FMRs remained highest in UF (71.81 Mg C ha−1). The SCD under different FMRs varied from 90.28–115.49 Mg C ha−1 with a mean value of 102.57 Mg C ha−1. The maximum SCD was recorded in RF (115.49 Mg C ha−1) and the minimum in CSF (90.28 Mg C ha−1). Additionally, the variation between UPF and DPF, CSF and UF remained statistically identical. The ECD was estimated to be significantly higher in RF (183.52 Mg C ha−1) than in the other FMRs, whereas CSF (126.05 Mg C ha−1) had the lowest ECD.
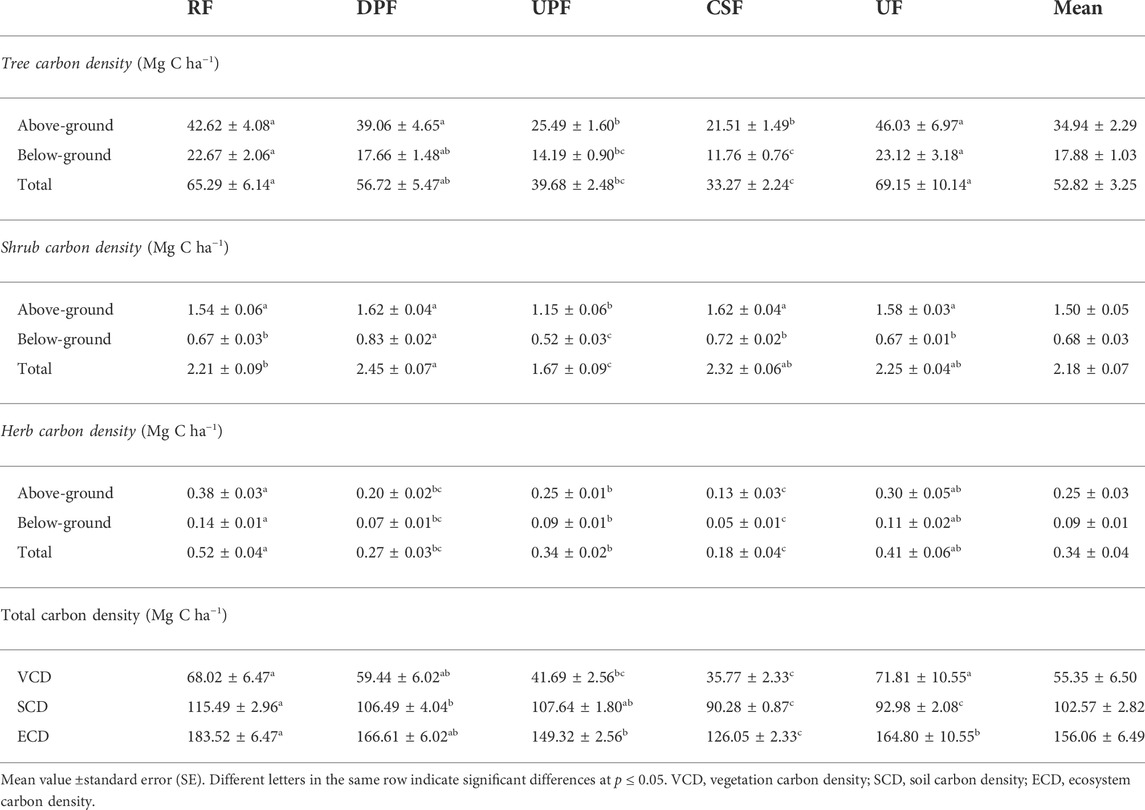
TABLE 4. Carbon density (Mg C ha−1) of trees, shrubs and herbs under different FMRs in Khair Working Circle of Nurpur Forest Division, Himachal Pradesh, India.
Species-wise contribution to total biomass
The species-wise contribution to the total tree biomass in each FMR is presented in Table 5. Acacia catechu contributed 40.59% to the total tree biomass in RF (137.45 Mg ha−1), followed by Pinus roxburghii (14.56%), Lannea coromandelica (12.93%) and Cassia fistula (11.83%). However, in DPF, P. roxburghii contributed the most (31.17%) followed by A. catechu (29.56%). The overall tree biomass in UPF was 83.53 Mg ha−1of which A. catechu accounted for 49.19% of the total biomass, followed by L. coromandelica (17.87%) and Albizia lebbeck (10.71%), respectively. In CSF, the total tree biomass was estimated to be 70.04 Mg ha−1 of which A. catechu contributed 57.02% followed by L. coromandelica (12.31%). In UF, out of the total tree biomass of 145.58 Mg ha−1, A. catechu contributed the most biomass (66.07%) followed by P. roxburghii (14.54%). The other associated tree species under different FMRs accounted for a low proportion (less than 10%) of the total tree biomass.
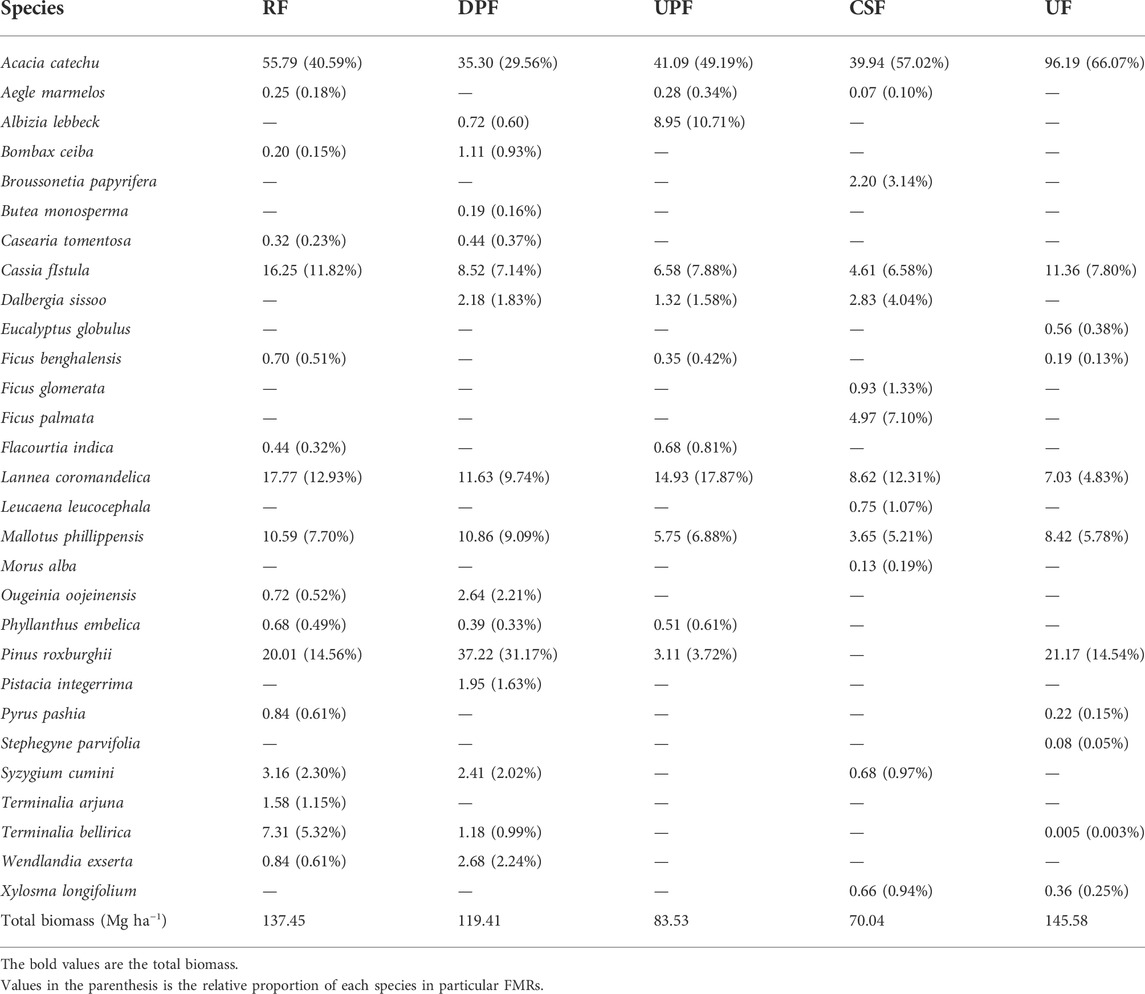
TABLE 5. Contribution of each species in total biomass (Mg ha−1) under different FMRs in Khair Working Circle of Nurpur Forest Division, Himachal Pradesh, India.
Discussion
An enhanced level of protection plays a pivotal role in conserving biodiversity (Hoffmann et al., 2018; Birben, 2019); however, its effectiveness depends upon governance and management (Cazalis et al., 2020). The forests in India are governed by various agencies viz., Government Forest officials, right holders/stakeholders, private individuals and organizations, indigenous people, etc. Each governance type has differentiated strategies depending upon the key actors involved in decision-making and level of their powers resulting in variation in the conservation outcomes (Macura et al., 2015). Strict FMRs are vital for biodiversity conservation and the minimization of loss (Hoffmann et al., 2018; Birben, 2019; Geldmann et al., 2019). However, the efficiency of any FMRs in conserving biodiversity is contingent on the governance or management of a specific forest (Cazalis et al., 2020).
Biodiversity of Khair dominated forests
In the present investigation, the maximum representation of trees and shrubs as recorded in terms of their number, density and basal area was observed in RF and DPF, which can be owed to high level of protection accorded to them. The maximum number of herb species, their density as well as basal area were found in UF, which could be due to the lesser tree density under this management regime, thus offering enough openings for the emergence of herbs on the ground floor. Additionally, the basal area and stem density recorded in response to FMRs can represent the stand structure and is also a useful indicator of human impact on a forest stand (Ingram et al., 2005) and may be ascribed to the species composition, age structure and degree of disturbance (Sundarapandian and Swamy, 1997). For Indian forests, the H′ for tree components varied from 0.83 to 4.1 (Devi and Yadava, 2006), 1.85–5.68 for different tropical forests of the Eastern Ghats of India (Chittibabu and Parthadarathy, 2000; Reddy et al., 2011; Panda et al., 2013; Naidu and Kumar, 2016). The studies undertaken in various parts of the north-western Himalayas in India indicates wide range of tree species diversity. Pokhriyal et al. (2012) recorded a range of 2.42–2.44 H′ in Phakot, Tehri Garhwal at 600–1900 m asl elevation, whereas, Sharma et al. (2010) discovered 1.14 H′ at Fatehpur, Deogadd at 750–1,250 m elevation in the foothills of Garhwal Himalaya. On addition, Sharma and Kala, (2022) reported a range of 2.01–2.11 tree H′ in Dhauladhar mountain of north-western Himalayas. In Kashmir, the average Ep, D′ and H′ was reported to be 1.42, 3.13, and 0.92, respectively (Shaheen et al., 2011). The DPF had the maximum tree H′, followed by RF, UPF, UF and CSF, respectively, which could be attributed to the degree of legal protection accorded to each FMRs. The degree of protection adopted in these FMRs followed the order; RF > DPF > UPF > UF > CSF, respectively. Anthropogenic pressure in UF and CSF FMRs may have led to disturbances, resulting in decreased species diversity and composition. This shows that the forest managed under varied regimes had a substantial effect on the diversity and heterogeneity among different FMRs. Amongst FMRs of Khair Working Circle, A. catechu was identified as a highly dispersed tree species followed by M. phillippensis, C. fistula, L. coromandelica and P. roxburghii; while, the remaining species exhibited accidental population occurrence. The findings of Biswas and Mukhopadhyay (2016) on phyto-sociology in developing conservation and management strategies for forests reveal that the degree of protection and anthropogenic activities considerably influence the vegetation structure in terms of species dominance and richness. They reported the maximum value for Simpson dominance in the area with the maximum disturbance. However, the richness was higher in the area with the least disturbance. The phyto-sociological attributes and diversity indices of differentially managed forests are comparable to the community-managed forests and government-managed forests of Bangladesh (Shinwari and Khan, 2000). They observed greater floral diversity, species richness and mature trees in the reserve forest as compared to those in the unreserved forest.
Since the anthropogenic disturbances occur in UF and CS, this might be the reason for the low species diversity under these FMRs observed in our study. However, the greater tree diversity in DPF than that of RF may be attributed to complete protection accorded to RF thus allowing the ecological succession to proceed smoothly, which may have resulted in the disappearance of species. However, in UF and CSF, trees of human interest are cultivated which ultimately increases the species dominance in the area. Similarly, Aime et al. (2015) found that PF (5.78) and natural RF (5.06) had a higher H′ than the community forests (4.64). However, Ep did not differ much between the protected (0.81) and community forests (0.83) but it was considerably different in natural voluntary reserve (0.71). In our study, the maximum Ep for trees was reported in DPF and UPF (0.77), whereas the minimum was in UF (0.52). The apparent similarity in Ep distribution in DPF and UPF was most likely due to the spatial patterns of stands that enable them to efficiently utilize the resources, such as sunlight, soil fertility, and species coexistence from the same functional group. Contrary to our findings, Pradhan et al. (2019) reported that the sacred groove forest managed by the local community had greater species richness, density and diversity than the wildlife sanctuary. This may be due to more awareness, religious beliefs and involvement of local people in forest management and conservation activities.
Stand and population structure
The stand structure of different FMRs viz., RF, DPF, UPF and CSF followed a reverse J-shaped curve from 15–20 cm diameter class onward. However, in reserve forests, the distribution of trees in various diameter classes was more balanced than in the other FMRs. A reverse J-shaped curve suggests exploitation in higher diameter classes (Rao et al., 1990) and is a good indicator for sustainable management of forest stands and steady growth of forest. In all FMRs, the lesser number of trees in diameter class 10–15 cm compared to 15–20 cm diameter class indicates concerns related to natural regeneration in the area. According to Philip and Gentry (1993), a reverse “J” shape is common for natural forests with active regeneration and recruitment. In general, trees with larger diameters contribute more basal area, than those with smaller ones (Hailemariam and Temam, 2018). Additionally, the lesser number of trees in bigger diameter classes in CSF compared to other FMRs is attributable to anthropogenic disturbances such as logging, fuel wood extraction and encroachments.
The seedlings and saplings of diverse species can help in forecasting future changes, regeneration, and status of flora biodiversity in any forest (Malik and Bhatt, 2016). The regeneration behavior of species depends on the internal community processes and exogenic disturbances (Barker and Patrik, 1994). In the present study, the lowest seedling and sapling density was found in UF and CSF which can be owed to variations in their management approach and the accessibility of these forests to local communities. According to Saxena and Singh (1984), grazing and trampling by animals affect the seedlings, regenerations, establishment and soil structure by compacting it, and leads to lower moisture content and permeability. This may alter the habitat and render it less suitable for the germination and establishment of seedlings. The enhanced canopy cover in CSF and UF categories had a direct effect on seed production, amount of light reaching the understory vegetation, soil properties and ultimately regenerative capacity of the stand (Vetaas, 2000). The reason for greater numbers of seedlings and saplings in RF and DPF as compared to other FMRs may be due to their higher degree of protection resulting in reduced disturbance and favorable conditions for regeneration to take place (Nagamastu et al., 2002). Traore et al. (2008) studied the regeneration success of Acacia spp. In eastern Burkina under two land-use regimes and reported good regeneration rate of Acacia spp. In protected areas as compared to the areas with high human impact. Also, Fayiah et al. (2018) recorded a fair regeneration for two forest reserves inventoried in southern Sierra Leone.
Carbon density of Khair dominated forests
Biomass and C stocks under different components-above, below and total (above + below) of trees, shrubs and herbs varied significantly in response to variation in FMRs. Forest C is considered a function of tree size and tree density (Lecina-Diaz et al., 2018; Van De Perreet al., 2018; Yuan et al., 2018; Ali et al., 2019), canopy cover, age, altitude and stand structure, composition, architectural attributes and ecological processes (Panzou et al., 2018) and forest management practices (Poudyal et al., 2019). Thus, this provides critical insights for sustainable forest management, enhancing forest conservation and ecosystem services. We found that RF and DPF have more ECD than the other FMRs. The maximum VCD was recorded in UF, which was mainly due to single species dominance (A. catechu) with a large basal area, each containing a disproportionately large C stock (Lung and Espira, 2015; Srinivas and Sundarapandian, 2019; Damptey et al., 2020) than many small trees as these forests are of plantation origin. Further, the high value of specific gravity (0.874 g cm−3) of Khair trees and biomass expansion factor also play a key role in C stock accumulation. Trees of bigger diameter classes are the main drivers of biomass and C stock variation across the tropics (Slik et al., 2013) and the heterogeneous distribution of large trees is influenced by basal area (Panzou et al., 2018). Our study confirms that RF and protected forest (DPF and UPF) have a favorable impact on biomass and C storage. Structurally, diverse forests were reported to have higher ecosystem productivity than simpler forests (Ali, 2019). In contrast, the smaller trees in CSF accounted for lower values of tree C density. Anthropogenic pressure near settlements altered the forest structure and had negative consequences on vegetation carbon (Vaidyanathan et al., 2010; Sapkota et al., 2018). Similarly, Gogoi et al. (2017) also reported that the total plant biomass and diversity decreased from the least disturbed to the most disturbed site. The difference in C stock values among these FMRs can also be attributed to species variability, tree structure, basal area, heterogeneity in diameter class, soil characteristics, study site conditions as well as anthropogenic factors (Lal, 2008; Becknell et al., 2012; Lewis et al., 2013; Slik et al., 2013; Sundarapandian et al., 2013; Dar and Sundarapandian 2015). The lower values in the less protected FMRs could also be due to unchecked extraction of timber, fuel wood and fodder from these forests, because of their proximity to human settlements (Sagar et al., 2003; Pande, 2005).
Conclusion
Ecosystem-based forest management practices are critical for the maintenance of species biodiversity, C density and forest soil health. In the present study, it was found that under different FMRs of Khair Working Circle, a representative forest of northern tropical deciduous forest, degree of protection and variation in their management approaches considerably influenced the biodiversity and soil C storage. UF management regime was found to be better at storing carbon in vegetation, while RF and DPF management regimes were more diverse and had more carbon in the soil and at ecosystem level. So, our study concluded that the legal framework is a crucial factor explaining much higher biodiversity, SCD and balanced distribution of tree biomass among different diameter classes inside RF and DPF than in UPF, UF and CSF. Although, the value for C density was higher in UF, yet diversity, as well as regeneration also have an important role in bio-resource conservation and sustainable management. Understanding the impact of different FMRs on forest composition, diversity and C storage capacity may offer evidence to support management decisions that will eventually improve the ecological health of the forests, increase the flow of ecosystem services, and support livelihoods in rural societies that are dependent on forest resources. In the present-day scenario, local communities also play a decisive role in conservation and maintenance of forest resources. However, in the present study, the CSF exhibited reduced biodiversity and C storage status. This calls for better coordination between local community and forest department officials in the management of Khair Working Circle forests to ensure their C mitigation potential and biodiversity conservation. Thus, it was clearly demonstrated that legal binding on forest protection would increase the amount of protected habitat for biodiversity conservation and C storage to maintain ecological functions for climate change adaptation. Therefore, in order to ensure biodiversity conservation and maintain large C sinks, it is crucial to fully protect RF and DPF and bring the unprotected FMRs viz., UPF, UF, and CSFs FMRs under REDD + initiative.
Data availability statement
The raw data supporting the conclusions of this article will be made available by the authors, without undue reservation.
Author contributions
Conceptualization, methodology, validation, formal analysis, investigation, DK, DB, and CT; data curation, writing—original draft, DK, DB, and CT; writing—review and editing, software visualization, NSh, PS, and NSa. All authors have read and agreed to the published version of the manuscript.
Acknowledgments
We duly acknowledge the technical guidance and contribution of Sh. V.P. Mohan, Chairman of the committee constituted for monitoring Silviculture Green Felling in Himachal Pradesh by Hon’ble Supreme Court of India, Basu Kaushal (DFO, Nurpur Forest Division), Sh. Sandeep Kohli (ACF, Nurpur Division), Sh. Arun Sharma (ACF, HP State Forest Department) and field staff of the Nurpur Forest Division. The authors are also grateful to the Head of the Department of Silviculture and Agroforestry, Y.S. Parmar, University of Horticulture and Forestry, Solan (HP), India, for providing the necessary facilities and technical support during the study. We are also thankful to two reviewers and Jinniu Wang from the Chinese Academy of Sciences, China for his valuable suggestions and comments on the overall improvement of the manuscript.
Conflict of interest
The authors declare that the research was conducted in the absence of any commercial or financial relationships that could be construed as a potential conflict of interest.
Publisher’s note
All claims expressed in this article are solely those of the authors and do not necessarily represent those of their affiliated organizations, or those of the publisher, the editors and the reviewers. Any product that may be evaluated in this article, or claim that may be made by its manufacturer, is not guaranteed or endorsed by the publisher.
References
Aime, C. D. V. B. T., Yves, A. Y. C., Djaha, K., Bruno, K. K., Bertin, G. B. Z., and Edouard, N. K. (2015). Trees species diversity and above ground biomass in three tropical forest types in Azaguie area. Glob. Adv. Res. J. Plant Sci. 1, 30–38.
Ali, A. (2019). Forest stand structure and functioning: Current knowledge and future challenges. Ecol. Indic. 98, 665–677. doi:10.1016/j.ecolind.2018.11.017
Ali, A., Lin, S. L., He, J. K., Kong, F. M., Yu, J. H., and Jiang, H. S. (2019). Big-sized trees overrule remaining trees’ attributes and species richness as determinants of aboveground biomass in tropical forests. Glob. Change Biol. 25, 2810–2824. doi:10.1111/gcb.14707
Barker, P. C. J., and Patrik, L. B. K. (1994). Phyllocladus aspleniifolius: Variability in the population structure, the regeneration niche and dispersion patterns in tasmanian forests. Aust. J. Bot. 42, 163–190. doi:10.1071/bt9940163
Becknell, J. M., Kucek, L. K., and Powers, J. S. (2012). Aboveground biomass in mature and secondary seasonally dry tropical forests: A literature review and global synthesis. For. Ecol. Manag. 276, 88–95. doi:10.1016/j.foreco.2012.03.033
Birben, U. (2019). The effectiveness of protected areas in biodiversity conservation: The case of Turkey. Cerne 25, 424–438. doi:10.1590/01047760201925042644
Biswas, S., and Mukhopadhyay, S. C. (2016). Plant community structure and carbon stock assessment through phytosociological approach at dry tropics of Banka Forest Division, Bihar. Indian J. Plant Sci. 5, 72–82.
Blackie, R., Baldauf, C., Gautier, D., Gumbo, D., Kassa, H., Parthasarathy, N., et al. (2014). Tropical dry forests: The state of global knowledge and recommendations for future research. Bogor: CIFOR. Discussion Paper.
Bonan, G. B. (2008). Forests and climate change: Forcings, feedbacks, and the climate benefits of forests. Science 320, 1444–1449. doi:10.1126/science.1155121
Cairns, M. A., Brown, S., Helmer, E. H., and Baumgardner, G. A. (1997). Root biomass allocation in the world’s upland forests. Oecologia 111, 1–11. doi:10.1007/s004420050201
Cazalis, V., Prince, K., Mihoub, J. B., Kelly, J., Butchart, S. H., and Rodrigues, A. S. (2020). Effectiveness of protected areas in conserving tropical forest birds. Nat. Commun. 11, 4461–4468. doi:10.1038/s41467-020-18230-0
Champion, H. G., and Seth, S. K. (1968). A revised survey of the forest types of India. Manager of Publications, Delhi, India. 404p.
Chauhan, N. S. (1999). Medicinal and aromatic plants of Himachal Pradesh. Indus Publishing Company. New Delhi, India. 632p.
Chave, J., Coomes, D. A., Jansen, S., Lewis, S. L., Swenson, N. G., and Zanne, A. E. (2009). Towards a worldwide wood economics spectrum. Ecol. Lett. 12, 351–366. doi:10.1111/j.1461-0248.2009.01285.x
Chazdon, R. L., Brancalion, P. H. S., Lamb, D., Laestadius, L., Calmon, M., and Kumar, C. (2017). A policy-driven knowledge agenda for global forest and landscape restoration. Conserv. Lett. 10, 125–132. doi:10.1111/conl.12220
Chittibabu, C. V., and Parthasarathy, N. (2000). Attenuated tree species diversity in human-impacted tropical evergreen forest sites at Kolli hills, Eastern Ghats, India. Biodivers. Conserv. 9, 1493–1519. doi:10.1023/a:1008971015545
Collins, M. B., and Mitchard, E. T. A. (2017). A small subset of protected areas are a highly significant source of carbon emissions. Sci. Rep. 7, 41902. doi:10.1038/srep41902
Damptey, F. G., Birkhofer, K., Nsiah, P. K., and De la Riva, E. G. (2020). Soil properties and biomass attributes in a former gravel mine area after two decades of forest restoration. Land 9, 209. doi:10.3390/land9060209
Dar, J. A., and Sundarapandian, S. M. (2015). Variation of biomass and carbon pools with forest type in temperate forests of Kashmir Himalaya, India. Environ. Monit. Assess. 187, 55–61. doi:10.1007/s10661-015-4299-7
Devi, L. S., and Yadava, P. S. (2006). Floristic diversity assessment and vegetation analysis of tropical semievergreen forest of Manipur, north east India. Trop. Ecol. 47, 89–98.
Dieler, J., Uhl, E., Biber, P., Muller, J., Rotzer, T., and Pretzsch, H. (2017). Effect of forest stand management on species composition, structural diversity, and productivity in the temperate zone of Europe. Eur. J. For. Res. 136, 739–766. doi:10.1007/s10342-017-1056-1
Fayiah, M., Swarray, M. K., Otesile, A., and Chen, B. (2018). “Comparative study of the regeneration potential of kasewe and taia riverine forests, moyamba district, Sierra Leone,” in presented at the 40th Annual Conference of Forestry Association of Nigeria (FAN) (New York: Conscientia Beam).
Forrester, D. I., and Bauhus, J. (2016). A review of processes behind diversity—Productivity relationships in forests. Curr. For. Rep. 2, 45–61. doi:10.1007/s40725-016-0031-2
Forster, E. J., Healey, J. R., Dymond, C., and Styles, D. (2021). Commercial afforestation can deliver effective climate change mitigation under multiple decarbonisation pathways. Nat. Commun. 12, 3831. doi:10.1038/s41467-021-24084-x
FSI (2019). India state of forest report 2019. Dehradun, India: Ministry of Environment, Forest and Climate Change.
FSI (2021). India state of forest report 2021. Dehradun, India: Ministry of Environment, Forest and Climate Change.
FSI (1996). Volume equations for forests of India, Nepal and Bhutan. India: Forest Survey of India, Ministry of Environment and Forests, Government of India.
Gamfeldt, L., Sneall, T., Bagchi, R., Jonsson, M., Gustafsson, L., Kjellander, P., et al. (2013). Higher levels of multiple ecosystem services are found in forests with more tree species. Nat. Commun. 4, 1340–1348. doi:10.1038/ncomms2328
Gao, T., Hedblom, M., Emilsson, T., and Nielsen, A. B. (2014). The role of forest stand structure as biodiversity indicator. For. Ecol. Manage. 330, 82–93. doi:10.1016/j.foreco.2014.07.007
Gautam, T. P., and Mandal, T. N. (2016). Effect of disturbance on biomass, production and carbon dynamics in moist tropical forest of eastern Nepal. For. Ecosyst. 3, 11–10. doi:10.1186/s40663-016-0070-y
Geldmann, J., Manica, A., Burgess, N. D., Coad, L., and Balmford, A. (2019). A global-level assessment of the effectiveness of protected areas at resisting anthropogenic pressures. Proc. Natl. Acad. Sci. U. S. A. 116, 23209–23215. doi:10.1073/pnas.1908221116
Gogoi, A., Sahoo, U. K., and Singh, S. L. (2017). Assessment of biomass and total carbon stock in a tropical wet evergreen rainforest of Eastern Himalaya along a disturbance gradient. J. Plant. Nutr. Soil Sci. 4, 1–8.
Hailemariam, M. B., and Temam, T. D. (2018). The vegetation composition, structure and regeneration status of Gole natural forest, West Arsi Zone, Oromia regional state. Ethiop. J. Agric. Sci. 2, 10–21. doi:10.35841/2591-7897.2.2.10-21
Hammer, O., Harper, D. A. T., and Ryan, P. D. (2001). Past: Paleontological statistics software package for education and data analysis. Palaeont. Electron. 4, 4–9.
Handa, I. T., Aerts, R., Berendse, F., Berg, M. P., Bruder, A., Butenschoen, O., et al. (2014). Consequences of biodiversity loss for litter decomposition across biomes. Nature 509, 218–221. doi:10.1038/nature13247
Herault, B., and Piponiot, C. (2018). Key drivers of ecosystem recovery after disturbance in a neotropical forest. For. Ecosyst. 5, 2. doi:10.1186/s40663-017-0126-7
Hoffmann, S., Beierkuhnlein, C., Field, R., Provenzale, A., and Chiarucci, A. (2018). Uniqueness of protected areas for conservation strategies in the European Union. Sci. Rep. 8, 6445. doi:10.1038/s41598-018-24390-3
Ingram, J. C., Whittaker, R. J., and Dawson, T. P. (2005). Tree structure and diversity in human-impacted littoral forests, Madagascar. Environ. Manage. 35, 779–798. doi:10.1007/s00267-004-0079-9
Jactel, H., Bauhus, J., Boberg, J., Bonal, D., Castagneyrol, B., Gardiner, B., et al. (2017). Tree diversity drives forest stand resistance to natural disturbances. Curr. For. Rep. 3, 223–243. doi:10.1007/s40725-017-0064-1
Janzen, D. H. (1988). Management of habitat fragments in a tropical dry forest: Growth. Ann. Mo. Botanical Gard. 75, 105–116. doi:10.2307/2399468
Jucker, T., Bouriaud, O., Avacaritei, D., Danila, I., Duduman, G., Valladares, F., et al. (2014). Competition for light and water play contrasting roles in driving diversity-productivity relationships in Iberian forests. J. Ecol. 102, 1202–1213. doi:10.1111/1365-2745.12276
Kumar, A., Pinto, M. C., Candeias, C., and Dinis, P. A. (2021). Baseline Maps of Potentially Toxic Elements in the Soils of Garhwal Himalayas, India: Assessment of Their Eco-environmental and Human Health Risks. Land Degrad. Dev. 32, 3856–3869. doi:10.1002/ldr.3984
Kothandaraman, S., Dar, J. A., Sundarapandian, S., Dayanandan, S., and Khan, M. L. (2020). Ecosystem-level carbon storage and its links to diversity, structural and environmental drivers in tropical forests of Western Ghats, India. Sci. Rep. 10, 1–15. doi:10.1038/s41598-020-70313-6
Lafleur, B., Fenton, N. J., Simard, M., Leduc, A., Pare, D., Valeria, O., et al. (2018). Ecosystem management in paludified boreal forests: Enhancing wood production, biodiversity, and carbon sequestration at the landscape level. For. Ecosyst. 5, 27. doi:10.1186/s40663-018-0145-z
Lakshmi, T., and Kumar, A. (2011). Preliminary phytochemical analysis & invitro antibacterial activity of Acacia catechu Willd Bark against Streptococcus mitis, Streptococcus sanguis & Lactobacillus acidophilus. Int. J. Phytomedicine 3, 579.
Lal, R. (2008). Sequestration of atmospheric CO2 in global carbon pools. Energy Environ. Sci. 1, 86–100. doi:10.1039/b80-9492-f
Lecina-Diaz, J., Alvarez, A., Regos, A., Drapeau, P., Paquette, A., Messier, C., et al. (2018). The positive carbon stocks-biodiversity relationship in forests: Co-occurrence and drivers across five subclimates. Ecol. Appl. 28, 1481–1493. doi:10.1002/eap.1749
Lee, J., Yoon, T. K., Han, S., Kim, S., Yi, M. J., Park, G. S., et al. (2014). Estimating the carbon dynamics of South Korean forests from 1954 to 2012. Biogeosciences 11, 4637–4650. doi:10.5194/bg-11-4637-2014
Lefcheck, J. S., Byrnes, J. E., Isbell, F., Gamfeldt, L., Griffin, J. N., Eisenhauer, N., et al. (2015). Biodiversity enhances ecosystem multifunctionality across trophic levels and habitats. Nat. Commun. 6, 6936. doi:10.1038/ncomms7936
Lewis, S. L., Lopez-Gonzalez, G., Sonke, B., Affum-Baffoe, K., Baker, T. R., Ojo, L. O., et al. (2009). Increasing carbon ´ storage in intact African tropical forests. Nature 457, 1003–1006. doi:10.1038/nature07771
Lewis, S. L., Sonke, B., Sunderland, T., Begne, S. K., Lopez-Gonzalez, G., van der Heijden, G. M. F., et al. (2013). Above-ground biomass and structure of 260 African tropical forests. Philos. Trans. R. Soc. Lond. B Biol. Sci. 368, 20120295. doi:10.1098/rstb.2012.0295
Liang, J., Crowther, T. W., Picard, N., Wiser, S., Reich, P. B., Alberti, G., et al. (2016). Positive biodiversity-productivity relationship predominant in global forests. Science 354, 8957. doi:10.1126/science.aaf8957
Liu, B., Liu, Q., Daryanto, S., Guo, S., Huang, Z., Wang, Z., et al. (2018). Responses of Chinese fir and Schima superba seedlings to light gradients: Implications for the restoration of mixed broadleaf-conifer forests from Chinese fir monocultures. For. Ecol. Manage. 41, 51–57. doi:10.1016/j.foreco.2018.03.033
Lung, M., and Espira, A. (2015). The influence of stand variables and human use on biomass and carbon stocks of a transitional african forest: Implications for forest carbon projects. For. Ecol. Manage. 351, 36–46. doi:10.1016/j.foreco.2015.04.032
Macura, B., Secco, L., and Pullin, A. S. (2015). What evidence exists on the impact of governance type on the conservation effectiveness of forest protected areas? Knowledge base and evidence gaps. Environ. Evid. 4, 24–29. doi:10.1186/s13750-015-0051-6
Magurran, A. E. (1988). Ecological diversity and its measurement. Princeton, New Jersey: Princeton University Press.
Malhi, Y., Roberts, J. T., Betts, R. A., Killeen, T. J., Li, W., and Nobre, C. A. (2008). Climate change, deforestation and the fate of the Amazon. Science 319, 169–172. doi:10.1126/science.1146961
Malik, Z. A., and Bhatt, A. B. (2016). Regeneration status of tree species and survival of their seedlings in Kedarnath Wildlife Sanctuary and its adjoining areas in Western Himalaya, India. Trop. Ecol. 57, 677–690.
Mancosu, E., Marín, A. I., Malak, D. A., Trombetti, M., and Bastrup-Birk, A. (2018). “Setting priorities for the management of primary forest areas: The importance of a harmonized inventory for supporting regional conservation and restoration efforts,” in 5th Forum Carpaticum.
Mir, A. H., and Upadhaya, K. (2017). Effect of traditional management practices on woody species composition and structure in montane subtropical forests of Meghalaya, Northeast India. J. Mt. Sci. 14, 1500–1512. doi:10.1007/s11629-016-4145-6
Nagamastu, D., Seiwa, K., and Sakai, A. (2002). Seedling establishment of deciduous trees in various topographic positions. J. Veg. Sci. 13, 35–44. doi:10.1111/j.1654-1103.2002.tb02021.x
Naidu, M. T., and Kumar, O. A. (2016). Tree diversity, stand structure, and community composition of tropical forests in Eastern Ghats of Andhra Pradesh, India. J. Asia. Pac. Biodivers. 9, 328–334. doi:10.1016/j.japb.2016.03.019
Nelson, D. W., and Sommers, L. E. (1996). Total carbon, organic matter. In: Method of soil analysis. Part 3. Chemical methods (D. L. Sparks eds). Soil Science Society of America, Madison, Wisconsin. 961–1010pp.
Panda, P. C., Mahapatra, A. K., Acharya, P. K., and Debata, A. K. (2013). Plant diversity in tropical deciduous forests of eastern Ghats, India: A landscape level assessment. Int. J. Biodivers. Conserv. 5, 625–639. doi:10.5897/IJBC2013.0581x
Pande, P. K. (2005). Biomass and productivity in some disturbed tropical dry deciduous teak forests of Satpura plateau. Madhya Pradesh. Trop. Ecol. 46, 229–240.
Panzou, G. J. L., Fayolle, A., Feldpausch, T. R., Ligot, G., Doucet, J. L., Forni, E., et al. (2018). What controls local-scale aboveground biomass variation in central africa? Testing structural, composition and architectural attributes. For. Ecol. Manage. 429, 570–578. doi:10.1016/j.foreco.2018.07.056
Paquette, A., and Messier, C. (2010). The role of plantations in managing the world’s forests in the Anthropocene. Front. Ecol. Environ. 8, 27–34. doi:10.1890/080116
Paudel, S., and Sah, P. J. (2015). Effects of different management practices on stand composition and species diversity in subtropical forests in Nepal: Implications of community participation in biodiversity conservation. J. Sustain. For. 34, 738–760. doi:10.1080/10549811.2015.1036298
Pearce, D. W. (2001). The economic value of forest ecosystems. Ecosyst. Health 7, 284–296. doi:10.1046/j.1526-0992.2001.01037.x
Philip, O., and Gentry, A. H. (1993). The useful plants of tambopata, Peru: Statistical hypothesis tests with a new quantitative technique. Econ. Bot. 47, 15–32.
Pielou, E. C. (1966). The measurement of diversity in different types of biological collections. J. Theor. Biol. 13, 131–144. doi:10.1016/0022-5193(66)90013-0
Pokhriyal, P., Chauhan, D. S., and Todaria, N. P. (2012). Effect of altitude and disturbance on structure and species diversity of forest vegetation in a watershed of central Himalaya. Trop. Ecol. 53, 307–315.
Poudyal, B. H., Maraseni, T., and Cockfield, G. (2019). Impacts of forest management on tree species richness and composition: Assessment of forest management regimes in Tarai landscape Nepal. Appl. Geogr. 111, 102078. doi:10.1016/j.apgeog.2019.102078
Pradhan, A., Ormsby, A. A., and Behera, N. (2019). A comparative assessment of tree diversity, biomass and biomass carbon stock between a protected area and a sacred forest of Western Odisha, India. Ecoscience 26, 195–204. doi:10.1080/11956860.2019.1586118
Pretzsch, H., Schutze, G., and Uhl, E. (2013). Resistance of European tree € species to drought stress in mixed versus pure forests: Evidence of stress release by inter-specific facilitation. Plant Biol. (Stuttg). 15, 483–495. doi:10.1111/j.1438-8677.2012.00670.x
Raghubanshi, A. S. (1991). Dynamics of soil biomass C, N, and P in a dry tropical forest in India. Biol. Fertil. Soils 12, 55–59. doi:10.1007/bf00369388
Rajput, S. S., Shukla, N. K., Gupta, V. K., and Jain, J. D. (1996). Timber mechanics: Strength classification and grading of timber. New Forest, Dehradun: Indian Council of Forestry Research and Education, 189.
Rao, P., Barik, S. K., Pandey, H. N., and Tripathi, R. S. (1990). Community composition and tree population structure in a sub-tropical broad-leaved forest along a disturbance gradient. Vegetatio 88, 151–162. doi:10.1007/bf00044832
Reddy, C. S., Babar, S., Amarnath, G., and Pattanaik, C. (2011). Structure and floristic composition of tree stand in tropical forest in the Eastern Ghats of northern Andhra Pradesh. Indian J. For. 22, 491. doi:10.1007/s11676-011-0193-5
Reise, J., Kukulka, F., Flade, M., and Winter, S. (2019). Characterising the richness and diversity of forest bird species using National Forest Inventory data in Germany. For. Ecol. Manage. 432, 799–811. doi:10.1016/j.foreco.2018.10.012
Richards, A. E., Forrester, D. I., Bauhus, J., and Scherer-Lorenzen, M. (2010). The influence of mixed tree plantations on the nutrition of individual species: A review. Tree Physiol. 30, 1192–1208. doi:10.1093/treephys/tpq035
Sagar, R., Raghubanshi, A. S., and Singh, J. S. (2003). Tree species composition, dispersion and diversity along a disturbance gradient in a dry tropical forest region of India. For. Ecol. Manage. 186, 61–71. doi:10.1016/s0378-1127(03)00235-4
Sagar, R., and Singh, J. S. (2005). Structure, diversity, and regeneration of tropical dry deciduous forest of northern India. Biodivers. Conserv. 14, 935–959. doi:10.1007/s10531-004-0671-6
Sapkota, I. P., Tigabu, M., and Oden, P. C. (2010). Changes in tree species diversity and dominance across a disturbance gradient in Nepalese Sal (Shorea robusta Gaertn. f.) forests. J. For. Res. 21, 25–32. doi:10.1007/s11676-010-0004-4
Sapkota, R. P., Stahl, P. D., Hengaju, K., and Rijal, K. (2018). Changes in the ecological parameters of mixed forests of sal (Shorea robusta gaertn.) are a function of distance from the human settlements. Int. J. Ecol. 2018, 1–29. doi:10.1155/2018/1394814
Saxena, A. K., and Singh, J. S. (1984). Tree population structure of certain Himalayan Forest associations and implications concerning their future composition. Vegetatio 58, 61–69. doi:10.1007/bf00044928
Schwab, N., Burzle, B., Bobrowski, M., Bohner, J., Chaudhary, R. P., Scholten, T., et al. (2022). Predictors of the success of natural regeneration in a himalayan treeline ecotone. Forests 13, 454. doi:10.3390/f13030454
Seddon, N., Chausson, A., Berry, P., Girardin, C. A. J., Smith, A., and Turner, B. (2020). Understanding the value and limits of nature-based solutions to climate change and other global challenges. Phil. Trans. R. Soc. B 375, 20190120. doi:10.1098/rstb.2019.0120
Shaheen, H., Khan, S. M., Harper, D. M., Ullah, Z., and Qureshi, R. A. (2011). Species diversity, community structure, and distribution patterns in Western Himalayan alpine pastures of Kashmir, Pakistan. Mt. Res. Dev. 31, 153–159. doi:10.1659/mrd-journal-d-10-00091.1
Shannon, C. E., and Weaver, W. (1963). The mathematical theory of communication. Urbana, USA: University Illinois Press, 117p.
Sharma, C. M., Baduni, N. P., Gairola, S., Ghildiyal, S. K., and Suyal, S. (2010). Tree diversity and carbon stocks of some major forest types of Garhwal Himalaya. India. For. Ecol. Manage. 260, 2170–2179. doi:10.1016/j.foreco.2010.09.014
Sharma, N., and Kala, C. P. (2022). Patterns in plant species diversity along the altitudinal gradient in Dhauladhar mountain range of the North-West Himalaya in India. Trees, For. People 1, 100196. doi:10.1016/j.tfp.2022.100196
Shinwari, M. I., and Khan, M. A. (2000). Folk use of medicinal herbs of margalla hills national park, islamabad. J. Ethnopharmacol. 69, 45–56. doi:10.1016/s0378-8741(99)00135-x
Singh, K., and Chand, P. (2012). Above-ground tree outside forest (TOF) phytomass and carbon estimation in the semi-arid region of southern Haryana: A synthesis approach of remote sensing and field data. J. Earth Syst. Sci. 121, 1469–1482. doi:10.1007/s12040-012-0237-z
Singh, K. P., and Singh, J. S. (1988). Certain structural and functional aspects of dry tropical forests and savanna. Int. J. Ecol. Environ. Sci. 14, 31–45.
Slik, J. W. F., Paoli, G., McGuire, K., Amaral, I., Barroso, J., Bastian, M., et al. (2013). Large trees drive forest aboveground biomass variation in moist lowland forests across the tropics. Glob. Ecol. Biogeogr. 22, 1261–1271. doi:10.1111/geb.12092
Srinivas, K., and Sundarapandian, S. (2019). Biomass and carbon stocks of trees in tropical dry forest of East Godavari region, Andhra Pradesh, India. Geol. Ecol. Landscapes 3, 114–122. doi:10.1080/24749508.2018.1522837
Sun, W., and Liu, X. (2020). Review on carbon storage estimation of forest ecosystem and applications in China. For. Ecosyst. 7, 4. doi:10.1186/s40663-019-0210-2
Sundarapandian, S. M., and Swamy, P. S. (1997). Plant biodiversity at low-elevation evergreen and moist deciduous forests at Kodayar (W. Ghats, India). Int. J. Ecol. Environ. Sci. 23, 363–379.
Sundarapandian, S. M., Dar, J. A., and Gandhi, D. S. (2013). Estimation of biomass and carbon stocks in tropical dry forests in Sivagangai district, Tamil Nadu, India. Int. J. Environ. Sci. Eng. Res. 4, 66–76.
Traore, S., Kabore, O., Rasolodimby, J. M., Thiombiano, L., and Guinko, S. (2008). Impact of protected areas and land use on regeneration of Acacia woodlands in eastern Burkina Faso. Flora Veg. Sudano-Sambesica 11, 17–24. doi:10.21248/fvss.11.3
Upadhaya, K., Barik, S. K., and Pandey, H. N. (2008). Response of woody species to anthropogenic disturbances in sacred forests of northeast India. Inter. J. Ecol. Environ. Sci. 34, 245–257.
Vaidyanathan, S., Krishnaswamy, J., Samba, K. N., Dhanwatey, H., Dhanwatey, P., and Karanth, K. U. (2010). Patterns of tropical forest dynamics and human impacts: Views from above and below the canopy. Biol. Conserv. 143, 2881–2890. doi:10.1016/j.biocon.2010.04.027
Van, P. F., Ratcliffe, S., Ruiz-Benito, P., Allan, E., Verheyen, K., Wirth, C., et al. (2017). Continental mapping of forest ecosystem functions reveals a high but unrealised potential for forest multifunctionality. Ecol. Lett. 21, 31–42. doi:10.1111/ele.12868
Van De Perre, F., Willig, M. R., Presley, S. J., Andemwana, F. B., Beeckman, H., Boeckx, P., et al. (2018). Reconciling biodiversity and carbon stock conservation in tropical forest landscape. Sci. Adv. 4, 1–9. doi:10.1126/sciadv.aar6603
Vetaas, O. R. (2000). The effect of environmental factors on the regeneration of Quercus semecarpifolia Sm. in Central Himalaya, Nepal. Plant Ecol. 146, 137–144. doi:10.1023/a:1009860227886
Walkley, A., and Black, J. A. (1934). Estimation of soil organic carbon by chromic acid filtration method. Soil Sci. 37, 38–39.
Wardle, D. A. (2001). Experimental demonstration that plant diversity reduces invasibility–evidence of a biological mechanism or a consequence of sampling effect? Oikos 95, 161–170. doi:10.1034/j.1600-0706.2001.950119.x
Westlake, D. F. (1963). Comparison of plant productivity. Biol. Rev. 38, 385–425. doi:10.1111/j.1469-185x.1963.tb00788.x
Working Plan (2013). Nurpur forest division - working plan 2012-13. Himachal Pradesh, India: Himachal Pradesh State Forest Department.
Yohannes, H., Soromessa, T., and Argaw, M. (2015). Carbon stock analysis along forest disturbance gradient in gedo forest: Implications of managing forest for climate change mitigation. J. Ecosyst. Ecogr. 5, 1–5. doi:10.4172/2157-7625.1000170
Yuan, Z., Wang, S., Ali, A., Gazol, A., Ruiz-Benito, P., Wang, X., et al. (2018). Aboveground carbon storage is driven by functional trait composition and stand structural attributes rather than biodiversity in temperate mixed forests recovering from disturbances. Ann. For. Sci. 75, 67–71. doi:10.1007/s13595-018-0745-3
Zanne, A. E., Lopez Gonzalez, G., Comes, D. A., Ilic, J., Janson, S., Lewis, S. L., et al. (2009). Global wood density database. Dryad digital repository. Available at: http://hdl.handle.net/10255/dryad.235.
Zhang, Z. Z., Zhou, J., Zhao, X. H., Zhao, P., Zhu, L. W., Ouyang, L., et al. (2017). Maximised photosynthetic capacity and decreased hydraulic failure risk during aging in the clump bamboo, Bambusa chungii. Funct. Plant Biol. 44, 785–794. doi:10.1071/FP16381
Keywords: carbon density, reserve forests, protected forest, unclassed forest, community society forest, stand characteristics, natural regeneration, biodiversity conservation
Citation: Kumar D, Thakur CL, Bhardwaj DR, Sharma N, Sharma P and Sankhyan N (2022) Biodiversity conservation and carbon storage of Acacia catechu willd. Dominated northern tropical dry deciduous forest ecosystems in north-western Himalaya: Implications of different forest management regimes. Front. Environ. Sci. 10:981608. doi: 10.3389/fenvs.2022.981608
Received: 29 June 2022; Accepted: 19 August 2022;
Published: 20 September 2022.
Edited by:
Thiru Selvan, Tripura University, IndiaReviewed by:
Amit Chawla, Institute of Himalayan Bioresource Technology (CSIR), IndiaPankaj Panwar, Indian Institute of Soil and Water Conservation (ICAR), India
Copyright © 2022 Kumar, Thakur, Bhardwaj, Sharma, Sharma and Sankhyan. This is an open-access article distributed under the terms of the Creative Commons Attribution License (CC BY). The use, distribution or reproduction in other forums is permitted, provided the original author(s) and the copyright owner(s) are credited and that the original publication in this journal is cited, in accordance with accepted academic practice. No use, distribution or reproduction is permitted which does not comply with these terms.
*Correspondence: D. R. Bhardwaj, bhardwajdr_uhf@rediffmail.com