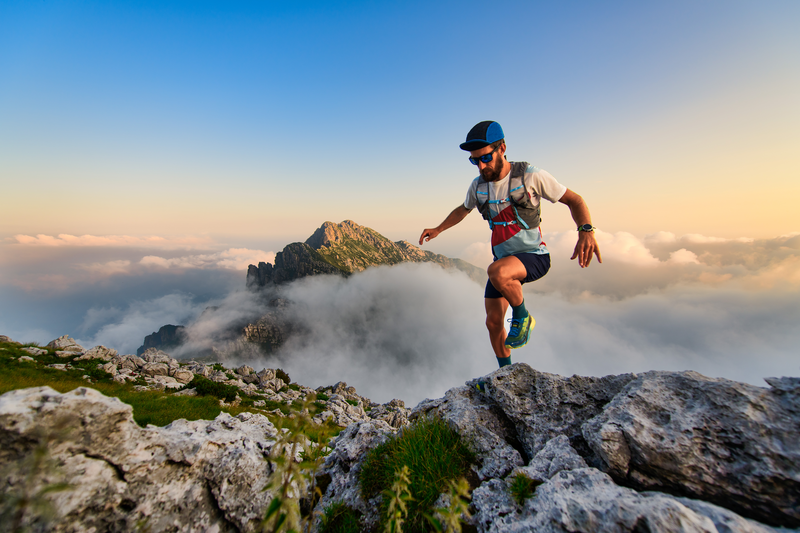
94% of researchers rate our articles as excellent or good
Learn more about the work of our research integrity team to safeguard the quality of each article we publish.
Find out more
ORIGINAL RESEARCH article
Front. Environ. Sci. , 19 September 2022
Sec. Toxicology, Pollution and the Environment
Volume 10 - 2022 | https://doi.org/10.3389/fenvs.2022.978850
This article is part of the Research Topic Environmental Contamination Solutions for Complex Heterogeneous Systems View all 10 articles
In this study, we investigated the importance of plant cover for secondary succession and soil fungal community development in remediated substrates after EDTA washing of metal-contaminated soils. The abundance of the total fungal community, determined by ITS fungal marker genes (Internal Transcribed Spacer region), and root colonisation by arbuscular mycorrhizal (AM) fungi were monitored in two types of soil material (calcareous and acidic) sown with perennial ryegrass (Lolium perenne L.) and without plant cover (bulk soil). Four months after the start of the experiment, the abundance of ITS genes in the soil clearly showed that the presence of plants was the main factor affecting the total fungal community, which increased in the rhizosphere soil in most treatments, while it remained at a low level in the bulk soil (without plants). Interestingly, the addition of environmental inoculum, i.e., rhizosphere soil from a semi-natural meadow, did not have a positive effect on the abundance of the total fungal community. While fungal ITS genes were detected in soils at the end of the first growing season, arbuscular mycorrhizal (AM) structures were scarce in Lolium roots in all treatments throughout the first season. However, in the second season, more than a year after the start of the experiment, AM fungal colonisation was detected in Lolium roots in virtually all treatments, with the frequency of colonised root length ranging from 30% to >75% in some treatments, the latter also in remediated soil. This study demonstrates the importance of plants and rhizosphere in the development and secondary succession of fungal communities in soil, which has important implications for the revitalisation of remediated soils and regenerative agriculture.
Fertile soil is a valuable and finite resource. The formation of soil is a slow process, taking millennia to form a few cm of soil. Soils contaminated with potentially toxic metals (also called heavy metals) are of particular concern because toxic metals are not degradable and remain in the environment for long periods (Amundson et al., 2015). In most cases, such soils are lost to their intended uses, or their continued use poses a serious threat to humans and other living organisms in the contaminated area as toxic metals enter food webs and ecological networks (Hartley and Lepp, 2008). Soil contamination with toxic metals is common even in heavily populated urban and rural areas (Li and Huang, 2007). Despite contamination, people often have vegetable gardens and grow their food on contaminated soil, even in polluted areas. Children are particularly vulnerable to metal contamination, which can trigger serious health problems (Jez and Lestan, 2015). Slovenia and many other countries have areas of arable and urban land (garden soils) contaminated with heavy metals due to industrial activities in the 20th century (EEA 2020, The EU Environment, State and Outlook). In this study, we used Pb- and Zn-contaminated soils from two sites, calcareous soils with neutral pH from Mežica, Slovenia (SI) and acidic soils from Arnoldstein, Austria (AT), to investigate the effects of remediation procedures specifically designed for different soil types on soil microbiota, especially on soil fungi.
Soil biodiversity is essential for soil health and regulation of soil functions (Griffiths and Philippot, 2013; Delgado-Baquerizo et al., 2016). Microbes represent the most diverse group of soil organisms, and understanding the mechanisms of soil microbial community development is urgently needed to predict the ecological impacts of environmental change (Maček et al., 2019). In particular, soil fungi are susceptible to mechanical and chemical disturbances, as many are filamentous organisms. Their hyphae are easily damaged during mechanical tillage of the soil, which can also affect their communities. For example, some fungi disappear from intensively managed agricultural soils with high levels of mechanical soil disturbance (Helgason et al., 1998; Oehl et al., 2010). Among soil fungi, the ancient and ubiquitous group of arbuscular mycorrhizal (AM) fungi (Glomeromycotina/Glomeromycota) (Spatafora et al., 2016; Tedersoo et al., 2018) is of particular interest because they are known to colonise plant roots and live in mutualistic symbiosis with more than 2/3rd of all terrestrial plants (Fitter and Moyersoen, 1996), including crops, vegetables, and fruit trees. Highlighting the importance of AM fungi in agroecosystems goes hand in hand with the Sustainable Development Goals. Awareness of the importance of sustainable food production and self-sufficiency is increasing as many people tend to grow their own food and try to do so in a sustainable and ecological way, with minimal disturbance to the soil and reduced use of mineral fertilisers and pesticides. Rising prices in the food market and producing healthy, homegrown food will become even more critical in the future. This way of growing vegetables at home also promotes the regeneration of soil biodiversity, including indigenous AM fungal communities in the soil, and can be an important factor in regenerative agriculture.
The method of soil washing with the chelating agent EDTA (ethylenediamine tetraacetic acid) and extraction of the contaminants have been shown to be highly efficient in the removal of metals (Pb, Zn, Cd) (e.g., Finžgar and Leštan, 2007; Pociecha and Lestan, 2012; Voglar and Lestan, 2013). The method has been modified for large-scale remediation of heavy metal contaminated soils and tested in demonstrational vegetable garden experiments (e.g., Gluhar et al., 2021a; Gluhar et al., 2021b; Kaurin et al., 2021). While the removal of various forms of toxic metals reduces hazards to humans and the environment, the process also has significant effect on microbial activity in the soil (Jelusic and Lestan, 2014; Maček et al., 2016; Kaurin et al., 2021). Particularly strong effects have been reported on fungal communities in the soil, reflected as lower mycorrhizal potential in the soil (Maček et al., 2016; Kaurin et al., 2021). In a demonstrational garden experiment with vegetable beds, the function of EDTA-washed soil was evaluated using enzyme and microbial indicators, including root colonisation by AM fungi (Kaurin et al., 2021). Conventional gardening largely restored the biological functions of the remediated soil; however, the most significant and long-lasting negative impact was confirmed on symbiotic AM fungi. Their colonisation recovered to some degree after several years of outdoor gardening, as indicated by root fungal colonisation recovery. However, there are currently no data on the composition and diversity of AM fungal communities in remediated systems. Based on the abundance of the total fungal community (qPCR targeting ITS genes) and root colonisation with AM fungi, this study is the first step in demonstrating the potential of recovery of fungal communities in remediated soils in outdoor settings and showing the importance of plants in this process.
In this study, we investigated the extent to which plants (Lolium perenne L.) that create a uniform plant cover and rhizosphere can contribute to faster and more intense establishment of fungal communities in remediated soils after EDTA washing treatment. We tested the following hypotheses: 1) EDTA washing procedure reduces fungal potential in soil and reduces the abundance of the total fungal community shown as ITS gene copy number 2). The potential for plant root colonisation by root endophytes, including arbuscular mycorrhizal fungi, is significantly reduced after EDTA treatment but rebuilds over time. 3) Plant cover and rhizosphere are the most important factors for the development and secondary succession of fungal communities in remediated soils.
Soils used for the mesocosm experiment were collected from the top 30 cm layer from a vegetable garden in Mežica, Slovenia (SI) and farmland in Arnoldstein, Austria (AT). The initial content of Pb, Cd and Zn before remediation was higher in SI soil with 1,029.7 mg Pb kg−1, 699 mg Cd kg−1 and 7.3 mg Zn kg−1 soil, while AT soil had 799.8 mg Pb kg−1, 437 mg Cd kg−1 and 5.4 mg Zn kg−1 soil. As pH is the most influential parameter for metal availability in soil, pH-neutral SI soil and acidic AT were selected (pH 6.9 vs. pH 5.0, respectively). Non-contaminated soil from grassland site in Ljubljana—Savlje, Slovenia, pedogenetically similar to Mežica soil, e.g. calcareous with pH 7.4, was used as control (CONT) with low levels of metals: 29.9 mg Pb kg−1, 64 mg Cd kg−1 and 0.4 mg Zn kg−1 soil, and pH 7.4.
Half of the soils from all three sites were remediated by washing using EDTA based (the ReSoil®) technology as described by Lestan (2017), Gluhar et al. (2019), and Gluhar et al. (2021a) using 60 mM Ca-EDTA kg−1 for the AT soil and 100 mM Ca-EDTA kg−1 for SI and CONT soils (remediated soil), and the other half was left untreated (original soil). The original and remediated soils from all three sites were placed in 35-L mesocosms (80 mesocosms; 24 cm diameter, 42 cm height) on 23 June 2016, filled with a 5-cm layer of quartz sand (1–3 mm) at the bottom, and covered with a plastic mesh (0.2 mm).
The contaminated SI and AT soils were examined in the experiment according to three factors: the first factor was remediation with two levels: no remediation (ORIG) and remediation (REM). The second factor was the presence of plants in the mesocosm with two levels: no plant (BULK) and plant (RHIZO). The third factor was inoculum (uncontaminated semi-natural grassland rhizosphere soil with plant roots): absence (no inoculum) or presence of inoculum in the soil (inoculum). In the case of the CONT soil, treatments were determined by only two factors: remediation (ORIG, REM) and plant (BULK, RHIZO). No inoculum was added at CONT. Thus, treatments with all combinations of the four factors (2 × 2 × 2 × 2 + 2*2 = 20 treatments) were applied in the experiment. Each treatment was replicated in four mesocosms (n = 4).
Half of the treatments were planted (40 mesocosms, RHIZO) with 70 ml of sterilised (2 min in 10% bleach) plant seeds (Lolium perenne L.) on 11 July 2016, and half of the treatments were left unplanted (bare soil) (40 mesocosms, BULK). We added inoculum (rhizosphere soil and roots) to the mesocosms as treatments (32 mesocosms) to determine its effect on soil microbial communities (100 ml inoculum in each mesocosms of the treatment). The rhizosphere inoculum was taken from uncontaminated semi-natural grassland in Ljubljana and mixed only into the top 5 cm of the soil layer in the mesocosms.
Soil samples were collected twice, at the beginning of the mesocosm experiment, just before the soils were placed into the mesocosms (summer 2016), and 4 months after plants seeds and inoculum were added to mesocosms (2 November 2016). These soil samples were dried at 40°C for 24 h and sieved through a 2 mm mesh sieve to remove roots and stones, and used for soil chemo-physical analyses and heavy metals content measurements. For the second soil sampling (2 November 2016), soil samples were collected from mesocosms at a depth of 0–5 cm, homogenised, the roots and stones were removed, and each soil sample was divided into three parts. One part of the sample was oven dried at 40°C for 24 h and used for chemical analysis. The second part was placed on dry ice and stored at -20°C for molecular analysis. Plant roots were sampled for AM fungal colonisation on 3 August 2016, 2 November 2016, and 17 November 2017. Roots were collected in a 3 × 15 cm soil core from each mesocosm, washed with tap water, and stored in 70% ethanol until root colonisation was measured.
Analyses of soil material were performed separately for each of the three locations, two contaminated (AT, SI) and one non-contaminated (CONT) soil. For the soil analyses, samples were air-dried and sieved to 2 mm (ISO11464, 2006). Total metal contents (Pb, Cd and Zn) were measured using inductively coupled plasma mass spectrometry (ICP-ES/MS) after digestion in aqua regia (Bureau Veritas Mineral laboratories, Canada). Soil organic carbon (SOC) and total nitrogen (TN) were determined by dry combustion (ISO 10694 1996; ISO 13878 1987) using an elemental analyser (Elementar vario MAX instrument, Germany). Carbonates were determined manometrically after a soil reaction with HCl (ISO 10693, 1995) and soil texture by the pipette method (ISO 11277, 2009). Soil pH was measured in a 1/2.5 (w/v) ratio of soil and 0.01 M CaCl2 suspension (ISO 10390 2005).
DNA was extracted from 0.5 g of fresh soil using the PowerSoil DNA Isolation Kit (MoBIO Laboratories, Carlsbad, CA, United States) following the recommended manufacturers protocol and stored at -20°C until further use. The quality and concentration of DNA extracts were determined spectrophotometrically (NanoDrop 2000 UV–vis Spectrometer; Thermo Fisher Scientific, Waltham, Massachusetts, United States).
Quantification of target ITS genes was done with a standard curve using SYBR green chemistry on the 7,500 Fast Real-Time PCR System (Applied Biosystems, Carlsbad, CA, United States). Reactions were performed in a 15 µl reaction volume containing 2 µl of DNA template (1 ng μl−1), 7.5 µl ABsolute Blue QPCR SYBR Green Rox mix (Thermo Fischer Scientific, Waltham, Massachusetts, United States), and 1.5 µl of 10 µM of ITS primers (ITS 3F, ITS 4R) (White et al., 1990). Two independent quantitative PCR assays were performed. The qPCR conditions were 95°C for 15min, followed by 35 cycles of 15 s at 95°C, 30 s at 55°C, 30 s at 72°C and a final step of 30 s at 80°C at which fluorescence was acquired. Standard curves were obtained using serial dilutions of the plasmid standard of the ITS gene ranging from 107 to 102 copies per reaction.
We used plasmid standards obtained from transformed Escherichia coli cells (strain JM109) with inserted plasmid PGEM-T (Promega, Madison, WI, United States). We greatly acknowledge Dr Laurent Philippot (INRAE, Dijon, France) for providing the ITS standard. No PCR inhibition was detected as tested by spiking samples with a known amount of external pGEM-T plasmid (Promega, Madison, Wisconsin, United States) and comparing its Cq values with positive control containing only plasmid. Melting curves were analysed for all runs to ensure PCR specificity. We calculated the copy numbers of gene per gram of dry soil to determine absolute gene abundance.
The roots were cleaned with hot 10% KOH (90°C) and acidified with 1 N HCl. The fungal tissue inside the roots was stained with 0.05% trypan blue in lactoglycerol. Several parameters of AM fungal colonisation of roots (F - frequency of mycorrhiza in the root system, M—intensity of the mycorrhizal colonisation in the root system, m—intensity of the mycorrhizal colonisation in the root fragments, A—abundance of arbuscules in the root system, a—arbuscular density in colonised root fragments, and S/V—spore/vesicle abundance in the root system) were determined by detailed estimation of AM fungal structures in roots according to Trouvelot et al. (1986) using an Olympus Provis AX70 microscope (n = 30 mixed root segments of 1 cm length for each pooled root sample—separate pot).
The results of the four-factor experiment for the frequency of the fungal ITS genes were evaluated using a linear model. In Table ANOVA for the four-factor experiment, the four-factor interaction was statistically significant, indicating that the influence of remediation, plant, or inoculum is not the same for all soil types. Contrast analysis was performed to evaluate the differences between the means of ITS gene abundance between treatments. Data were log transformed to meet the assumption of homogeneity of variance between treatments. In the case of AM fungal colonisation data (F, M, and m), only three factors were examined because all measurements were made only in the pots with plants. All three variables were transformed using the asin [sqrt()] transformation. A generalised linear model was used for the variable m to account for the different variance modelling in the different treatments. To evaluate the colonisation of plant roots by arbuscular mycorrhizal fungi, we divided the contrasts into three groups. First, we examined the difference in colonisation between heavy metal contaminated (AT and SI) and control sites; second, we focused on the effect of the remediation procedure on colonisation; and third, the effect of inoculum addition on colonisation. All statistical analyses were performed in an R environment (R Core Team, 2021).
Soil remediation efficiently removed Pb, Zn, and Cd in both contaminated soils. In the SI soil, Pb was reduced from 1,029.7 mg kg−1 to 451.9 mg kg−1, Cd from 699 mg kg−1 to 493 mg kg−1 and Zn from 7.3 mg kg−1 to 3.7 mg kg−1. An efficient reduction of toxic metals after remediation was also observed in AT soils, with a reduction of Pb from 799.8 mg kg−1 to 216.8 mg kg−1, Cd from 437.0 mg kg−1 to 243.0 mg kg−1, and Zn from 5.4 mg kg−1 to 1.3 mg kg−1. It is important to note that after remediation, the Pb, Cd and Zn contents in both soils, SI and AT, are below the critical values according to Slovenian legislation (Ur, 1996). In addition to efficient metal removal, EDTA remediation increased soil pH in both soils, e.g., by 0.4 pH units in SI soil and 1.2 pH units in AT soil, to a pH of 7.3 and 6.2 in SI and AT soil, respectively. In addition, soil organic carbon content (SOC) was decreased in both soils: from 6.6% to 4.1% in SI and from 3.0% to 2.8% in AT. Changes in soil texture were also observed after remediation, with heavier soils after remediation. Clay content increased from 5.8% to 7.3% and from 11.4% to 14.9% for soils SI and AT, respectively. Silt content increased from 36.7% to 52.6% and from 44.9% to 50.6% for soil SI and AT, respectively. No significant changes in soil chemical properties after remediation, including Pb, Zn and Cd contents, were observed in CONT soil, while remediation changed soil texture, similarly to soils SI and AT soils. In CONT soil, clay content increased from 9.2% to 11.9%, and silt increased from 44.7% to 50%.
The abundance of fungal ITS genes was significantly affected by the interaction between all factors (remediation, plant, inoculum and location) (p < 0.027). Among the three soils, fungal abundance was significantly lower in CONT soil, compared to AT and SI soils, but this effect varied depending on remediation, plant and inoculum (Table 1; Figure 1). A significant difference in fungal abundance between AT and SI soils was evident only in ORIG BULK soils with inoculum (Table 1), where the fungal abundance was significantly higher in SI soil than in AT soil. On an average, the fungal ITS gene abundance in AT ORIG BULK soil with inoculum was 23% (6 %–96%) of that in SI ORIG BULK soil with inoculum.
TABLE 1. Estimated ratios of means of fungal ITS abundance [location (AT, SI, CONT), remediation (ORIG, REM), plant (RHIZO, BULK), inoculum (I, NoI)] in contrast analysis with 95% confidence intervals. Only statistically significant contrasts are shown.
FIGURE 1. Fungal ITS abundance in Austrian (AT), Slovenian (SI) and control (CONT) soil, before (ORIG) and after remediation (REM). Soil from mesocosms was sampled 4 months after the addition of plants seeds and indigenous inoculum (rhizosphere soil and roots from a grassland) (2 November 2016). Mean ± SE of four replicates is shown.
It also showed that the influence of plant and inoculum on fungal abundance was different in different soils (AT, SI, CONT), while remediation had no effect. The variability of values within treatments differed among treatments and was greater at higher values, especially in treatments with plant presence (Figure 1). When plants were present, fungal abundance was higher on average than in treatments without plants. The highest fungal abundance was found in SI REM and ORIG RHIZO soil (8.33 × 108 and 1.60 × 108 copies/g soil, respectively), compared to that without plants. On average, fungal abundance in SI REM BULK and SI ORIG BULK soil was 16% (4%–67%) and 23% (5%–108%) of that in SI REM and ORIG RHIZO soil, respectively. In addition, fungal abundance was significantly higher in AT ORIG soil with inoculum, SI REM soil with inoculum and CONT ORIG soil due to plant presence (Table 1; Figure 1). The opposite pattern was observed in the treatments with inoculum compared to the treatments without. Due to inoculum addition, we observed lower fungal abundance in SI REM BULK and RHIZO, AT ORIG BULK and AT REM RHIZO soils (Table 1; Figure 1).
AM fungal colonisation of Lolium perenne roots was detected only in two mesocosms during the first growing season, 1 month after the start of the experiment, both from original AT soil (sampled on 3 August 2016, 1 month after L. perenne seeding). The addition of inoculum to the original AT soil increased the frequency of mycorrhiza (F), the intensity of root cortex colonisation (M), and the intensity of colonisation within individual mycorrhizal roots (m) (13.3%, 2.1% and 15.5%, respectively) compared to the mesocosms with the original AT soil without the addition of inoculum (3.3%, 0.03% and 1.0%, respectively). In addition, the frequency of vesicles and spores in the mesocosms with the original AT soil with added inoculum was 13.3% (F), 2.1% (M), 15.5% (m), 29.0% (a) and 0.6% (A), respectively. In all other mesocosms, AM colonisation was not yet established.
One year and 5 months after the start of the experiment (sampled on 17 November 2017), AM fungal colonisation (Table 2) was established in all sampled mesocosms. No significant difference in AM fungal colonisation (F—Figure 2, M—Figure 3 and m—Figure 4) was observed between the contaminated ORIG (AT and SI) and CONT soils (p > 0.99). The remediation process had a significant effect on frequency of plant roots colonised with arbuscular mycorrhizal fungi (F) in SI soils (p = 0.0103), where we have observed significantly higher F in ORIG SI soil (58.0 ± 13.5%) compared to REM SI soils (32.4 ± 12.8%).
TABLE 2. Linear model estimates of mean ± SE AM fungal root colonisation (sampled on 17 November 2017) calculated as frequency of mycorrhization (F), intensity of root cortex colonisation (M) and intensity of colonisation within individual mycorrhizal roots (m).
FIGURE 2. Mean relative frequency (F) of plant roots colonised with arbuscular mycorrhizal fungi (F) in Lolium perenne L. in Austrian (AT), Slovenian (SI) and control soil (CONT), before (ORIG) and after remediation (REM). Root sampling on 17 November 2017. The addition of indigenous inoculum (rhizosphere soil and roots from a grassland) is indicated with yes/no. Presented are means ± SE of measured values.
FIGURE 3. Mean intensity (M) of mycorrhizal colonisation in the root system (M) in Lolium perenne L. in Austrian (AT), Slovenian (SI) and control soil (CONT), before (ORIG) and after remediation (REM). Root sampling on 17 November 2017. The addition of indigenous inoculum (rhizosphere soil and roots from a grassland) is marked yes/no. Shown are the measured values of mean and SE.
FIGURE 4. Mean intensity of mycorrhizal colonisation in root fragments (m) in Lolium perenne L. in Austrian (AT), Slovenian (SI) and control (CONT) soil, before (ORIG) and after remediation (REM). Root sampling on 17 November 2017. The addition of indigenous inoculum (rhizosphere soil and roots from a grassland) is marked yes/no. Shown are the measured values of mean and SE.
The significant effect of inoculum addition on F was observed only in REM AT soils (p = 0.0451). REM AT soil without added inoculum (83.9 ± 10.1%) had significantly higher F compared to REM AT soil with added inoculum (28.7 ± 12.4%).
The intensity of mycorrhizal colonisation in the root system (M) was significantly different in both ORIG soils (SI and AT) before and after remediation, showing a clear effect of the remediation process on AM colonisation. In AT soils, the remediation process significantly (p = 0.047) increased M from 15.3 ± 5.3% in ORIG soil to 43.2 ± 7.3% in REM soil, whereas in SI soil, M was significantly (p = 0.077) decreased from 15.1 ± 5.3 in ORIG to 3.9 ± 2.8% in REM soil. Addition of inoculum had a significant effect on M only in REM AT soils (p = 0.0028) and showed a decrease in M when inoculum was added to the soil (43.2 ± 7.3 to 8.0 ± 4.0%).
The remediation process significantly affected the intensity of mycorrhizal colonisation in root fragments (m) in SI soils (p = 0.0111), reducing m from 28.2 ± 5.7% in ORIG SI soil to 13.7 ± 6.9% in REM SI soil. There was no significant effect of the addition of inoculum on m (p > 0.23).
Arbuscular abundance in the mycorrhizal parts of the root fragments (a) was still very low 1 year and 5 months after the start of the field experiment. A large proportion of the roots did not have arbuscules, so only the measured average values with standard error are reported. The highest percentage of arbuscules in the mycorrhizal parts of the root fragments (12.2 ± 11.6%) was found in ORIG SI soil, followed by REM CONT soil (9.6 ± 4.6%). The percentage was very low In ORIG AT soil and REM soil with and without added inoculum (< 1%).
The highest abundance of arbuscules in the root system (A) was observed in CONT REM soils (2.0 ± 0.9%), followed by ORIG SI soil (1.0 ± 0.9%), while in AT soils, the average abundance in root system was below 0.3%.
Another parameter indicating the presence of AM fungi in the roots was vesicles and spores, which were very variable in the different treatments. The highest abundance (40.4%) of spores and vesicles in the root system (S/V%) was found in Lolium grown in remediated AT soil (Table 3).
TABLE 3. Spores and vesicles abundance in the root system (S + V), sampled on 17 November 2017. Presented is measured value of one replicate per treatment.
This study reveals the time-scale of the revitalisation of remediated soil by fungi and highlights the importance of plant cover for secondary succession of soil fungal communities in remediated soils. While root colonisation by symbiotic AM fungi showed a slower response, soil fungal ITS molecular markers indicate that fungal communities begin to develop relatively fast after remediation and within a few months after the initiation of the outdoor experiment with remediated soil. Furthermore, neither the fungal ITS markers nor AM fungal root colonisation showed a positive response to the addition of environmental inoculum regardless of soil characteristics (SI or AT soil). Because microbes, including fungi, play essential functional roles in soils (Griffiths and Philippot, 2013; Delgado-Baquerizo et al., 2016), higher abundance of the total fungal community may reflect ecologically relevant functional traits (e.g., mycelium growth, phosphate, nitrogen and carbon metabolism, and other functional traits) associated to fungal taxa identity (e.g., Maček et al., 2019; Põlme et al., 2020).
EDTA remediation has been shown to successfully remove toxic metals from the soil, and the remediated soil has been tested and confirmed to be well suited as a plant substrate for vegetable production (e.g., Gluhar et al., 2021b; Kaurin et al., 2021). Maintaining plant cover has been shown to be an important factor in soil health and is also part of the practice of sustainable and regenerative agriculture (Sherwood and Uphoff, 2000; Cappelli et al., 2022). When plants, including their roots, are removed from the soil, an important source of carbon is lost as plants are known to support the rhizosphere by transferring approximately 30% of photosynthetically sequestered carbon to their roots (Bago et al., 2000). Therefore, the post-remediation soil revitalisation procedure must consider the importance of continuous plant presence and a plant rhizosphere for the development of a functional fungal community the soils. This contributes to the restoration of soil biodiversity after intensive remediation treatment with EDTA washing and mechanical disturbance in addition to other already tested practices (e.g., Kaurin et al., 2021).
The internal transcribed spacer regions (ITS) are used as molecular markers for fungi (Schoch et al., 2012) and are not specific to arbuscular mycorrhizal fungi. Therefore, markers of other fungal groups, including non-symbiotrophs are also amplified using ITS primers. In our study, the abundance of ITS genes was consistently higher in treatments with Lolium perenne plants that formed a functional rhizosphere, regardless of the remediation treatments (Figure 1). The presence of plants and their rhizosphere supports several microbial (fungal) groups in the soil, which is also indicated in our results (Figure 1), but it is crucial and obligatory in the case of arbuscular mycorrhiza, as AM fungi are mutualistic biotrophs and receive all their carbon from partner plants, in our case Lolium.
Amendments in the form of compost and other substrates (Gluhar et al., 2021b; Kaurin et al., 2021) to the remediated substrate can work well as a source of local soil microbiota, but care should be taken to ensure that these substrates do not contain additional toxic substances, especially if they were also produced in the contaminated area. Interestingly, original (contaminated) soil that is left untreated and usually deposited in a loose state in a given location until a remediation treatment is applied often loses a significant amount of its vitality, which translates into reduced mycorrhizal potential in terms of the potential of the fungal inocula present in the soil to initiate mycorrhiza with newly seeded plants, as indicated in our study (Figures 2–4; Table 2). This has been observed in several studies where soils from the ORIG treatment also had relatively low mycorrhizal potential at the beginning of the experiment, even though the soil was not treated with chemicals and mechanical treatments as part of the remediation process (e.g., Maček et al., 2016; Kaurin et al., 2021).
In our experiment, mycorrhizal structures were detected in roots in some of the treatments already in the first growing season, one and 4 months after the start of our experiment, but in a very small proportion of roots. In the second growing season of 2017, F reached >30% of root parts colonised with mycorrhizal fungi in all experimental soil treatments (Figure 2). This result implies that soil processing during the remediation procedure, in addition to mechanical disturbance (mixing and sieving of soil), negatively affects the potential of AM fungi to form a functional symbiosis even in the non-remediated soil treatment, but mycorrhiza establishes with time in an outdoor setting (Figures 2–4; Table 2).
Other important mycorrhizal parameters (a—arbuscule abundance in the mycorrhizal parts of the root fragments and calculated A—abundance of arbuscules in the root system) and the presence of spores and vesicles indicate the mycorrhizal potential of AM fungi and compatibility with the plant partner, showed that mycorrhizal symbiosis was well established in the second season. In particular, a relatively high number of vesicles and spores in the roots of Lolium, which serve as reproductive and reserve organs for AM fungi to store lipids, indicated that a significant amount of carbon photosynthetically fixed by Lolium was transferred to the rhizosphere and stored in fungal tissues. In particular, the arbuscules are indicative of a functional symbiosis and nutrient exchange between plants and AM fungi. However, their low density in our experiment could be a consequence of the relatively late sampling time in November 2017. The timing of root sampling, which was conducted relatively late in the season in November, was chosen to minimize disruption to the experimental system, as root sampling is always invasive and can interfere with rhizosphere and root processes.
In a vegetable garden study using the same type of remediated soil (SI carbonate soil) and a range of vegetable plant species, the full extent of mycorrhizal colonisation was established, with abundance densities approaching 50% in all soil treatments, but vary depending on the identity of the plant host (Kaurin et al., 2021). The trend of arbuscule formation has been shown to follow a similar pattern to the frequency of the roots mycorrhiza (F), but with some time lag (Kaurin et al., 2021).
Data on colonisation of roots by AM fungi do not provide information on the diversity of fungi in the root system. Therefore, to gain better insight into the ecology and diversity of the AM fungal community in the experiment, additional molecular sequencing of marker genes for fungal community analysis should be performed as a next step. Previous studies on revitalisation of remediated soils with AM fungi (Maček et al., 2016) showed that the most abundant taxa in the newly established AM fungal communities belonged to fungi known for their ruderal (opportunistic) strategy and tolerating anthropogenic disturbances. Among them, Funneliformis mosseae, which is also described as a disturbance-resistant fungus in many studies, and other fungal taxa from the Funneliformis and Rhizophagus clades were also common in a small-scale pot experiment with SI remediated soil (Maček et al., 2016). These AM fungal taxa may also be responsible for the majority of plant root colonisation in this study, but this could only be verified using molecular methods and sequencing. The identity of AM fungal taxa is important because they are functionally distinct and different taxa provide different benefits to host plants, so changes in symbiotic AM fungal populations have the potential to resonate throughout their associated plant communities (Maček et al., 2019). This can lead to changes in above-ground competition dynamics and ecosystem productivity also in systems that develop on remediated soils. Therefore, soil microbes, including soil fungi, and their changes in population density in response to remediation procedures can have significant impact on terrestrial plant communities that develop on these soils and also on their productivity, which is particularly important in agroecosystems.
The results of our experiments clearly show that fungal communities and functional arbuscular mycorrhiza can be established in remediated soils with or without the addition of inoculum when the soils are outdoors and surrounded by semi-natural grassland that allows spontaneous inoculation with fungal spores by wind, water, and animals. Care should be taken to ensure that the remediated substrate is always covered by plants to encourage the development of symbiotic root endophytic fungi that are completely dependent on their host plant as a carbon source.
The raw data supporting the conclusions of this article will be made available by the authors, without undue reservation.
IM and MS contributed to the conception and design of the study. DL was responsible for the EDTA remediation of soils. SP, NS, and TR carried out pot experiment and laboratory analyses. DK performed the statistical analysis. IM wrote the first draft of the manuscript. SP, NS, DK and MS wrote sections of the manuscript. All authors contributed to manuscript revision, read, and approved the submitted version.
The authors acknowledge the financial support from the Slovenian Research Agency (projects J4-7052 and J4-3098 and Research Core Funding No. P4-0085 and P4-0107).
We also thank Simon Gluhar for technical assistance and Natalija Dovč and Rok Damjanić for the help with data analysis.
The authors declare that the research was conducted in the absence of any commercial or financial relationships that could be construed as a potential conflict of interest.
All claims expressed in this article are solely those of the authors and do not necessarily represent those of their affiliated organizations, or those of the publisher, the editors and the reviewers. Any product that may be evaluated in this article, or claim that may be made by its manufacturer, is not guaranteed or endorsed by the publisher.
Amundson, R., Berhe, A. A., Hopmans, J. W., Olson, C., Sztein, A. E., and Sparks, D. L. (2015). Soil science. Soil and human security in the 21st century. Science 80, 1261071. doi:10.1126/science.1261071
Bago, B., Pfeffer, P., and Shachar-hill, Y. (2000). Carbon metabolism and transport in arbuscular mycorrhizas. Plant Physiol. 124, 949–958. doi:10.1104/pp.124.3.949
Cappelli, S. L., Domeignoz-Horta, L. A., Loaiza, V., and Laine, A. L. (2022). Plant biodiversity promotes sustainable agriculture directly and via belowground effects. Trends Plant Sci. 27, 674–687. doi:10.1016/j.tplants.2022.02.003
Delgado-Baquerizo, M., Maestre, F. T., Reich, P. B., Jeffries, T. C., Gaitan, J. J., Encinar, D., et al. (2016). Microbial diversity drives multifunctionality in terrestrial ecosystems. Nat. Commun. 7, 10541–10548. doi:10.1038/ncomms10541
EEA (2020). The EU environment, state & outlook. Available at https://www.eea.europa.eu/publications/soer-2020.
Finžgar, N., and Leštan, D. (2007). Multi-step leaching of Pb and Zn contaminated soils with EDTA. Chemosphere 66, 824–832. doi:10.1016/j.chemosphere.2006.06.029
Fitter, A. A. H., and Moyersoen, B. (1996). Evolutionary trends in root-microbe symbioses evolutionary trends in root-microbe symbioses. Philosophical Trans. R. Soc. B Biol. Sci. 351, 1367–1375.
Gluhar, S., Jez, E., and Lestan, D. (2019). The use of zero-valent Fe for curbing toxic emissions after EDTA-based washing of Pb, Zn and Cd contaminated calcareous and acidic soil. Chemosphere 215, 482–489. doi:10.1016/j.chemosphere.2018.10.074
Gluhar, S., Kaurin, A., Finžgar, N., Gerl, M., Kastelec, D., and Lestan, D. (2021a). Demonstrational gardens with EDTA-washed soil. Part I: Remediation efficiency, effect on soil properties and toxicity hazards. Sci. Total Environ. 792, 149060. doi:10.1016/j.scitotenv.2021.149060
Gluhar, S., Kaurin, A., Vodnik, D., Kastelec, D., Zupanc, V., and Lestan, D. (2021b). Demonstration gardens with EDTA-washed soil. Part III: Plant growth, soil physical properties and production of safe vegetables. Sci. Total Environ. 792, 148521. doi:10.1016/j.scitotenv.2021.148521
Griffiths, B. S., and Philippot, L. (2013). Insights into the resistance and resilience of the soil microbial community. FEMS Microbiol. Rev. 37, 112–129. doi:10.1111/j.1574-6976.2012.00343.x
Hartley, W., and Lepp, N. W. (2008). Remediation of arsenic contaminated soils by iron-oxide application, evaluated in terms of plant productivity, arsenic and phytotoxic metal uptake. Sci. Total Environ. 390, 35–44. doi:10.1016/j.scitotenv.2007.09.021
Helgason, T., Daniell, T., Husband, R., Fitter, A., and Young, J. (1998). Ploughing up the wood-wide web? Nature 394, 431. doi:10.1038/28764
Jelusic, M., and Lestan, D. (2014). Effect of EDTA washing of metal polluted garden soils. Part I: Toxicity hazards and impact on soil properties. Sci. Total Environ. 475, 132–141. doi:10.1016/j.scitotenv.2013.11.049
Jez, E., and Lestan, D. (2015). Prediction of blood lead levels in children before and after remediation of soil samples in the upper Meza Valley, Slovenia. J. Hazard. Mat. 296, 138–146. doi:10.1016/j.jhazmat.2015.04.049
Kaurin, A., Gluhar, S., Maček, I., Kastelec, D., and Lestan, D. (2021). Demonstrational gardens with EDTA-washed soil. Part II: Soil quality assessment using biological indicators. Sci. Total Environ. 792, 148522–148529. doi:10.1016/j.scitotenv.2021.148522
Lestan, D. (2017). Novel chelant-based washing method for soil contaminated with Pb and other metals: A pilot-scale study. Land Degrad. Dev. 28, 2585–2595. doi:10.1002/ldr.2818
Li, X., and Huang, C. (2007). Environment impact of heavy metals on urban soil in the vicinity of industrial area of Baoji city, P.R. China. Environ. Geol. 52, 1631–1637. doi:10.1007/s00254-006-0608-3
Maček, I., Clark, D. R., Šibanc, N., Moser, G., Vodnik, D., Müller, C., et al. (2019). Impacts of long-term elevated atmospheric CO2 concentrations on communities of arbuscular mycorrhizal fungi. Mol. Ecol. 28, 3445–3458. doi:10.1111/mec.15160
Maček, I., Šibanc, N., Kavšček, M., and Lestan, D. (2016). Diversity of arbuscular mycorrhizal fungi in metal polluted and EDTA washed garden soils before and after soil revitalization with commercial and indigenous fungal inoculum. Ecol. Eng. 95, 330–339. doi:10.1016/j.ecoleng.2016.06.026
Oehl, F., Laczko, E., Bogenrieder, A., Stahr, K., Bösch, R., van der Heijden, M., et al. (2010). Soil type and land use intensity determine the composition of arbuscular mycorrhizal fungal communities. Soil Biol. Biochem. 42, 724–738. doi:10.1016/j.soilbio.2010.01.006
Pociecha, M., and Lestan, D. (2012). Washing of metal contaminated soil with EDTA and process water recycling. J. Hazard. Mat. 235–236, 384–387. doi:10.1016/j.jhazmat.2012.08.001
Põlme, S., Abarenkov, K., Henrik Nilsson, R., Lindahl, B. D., Clemmensen, K. E., Kauserud, H., et al. (2020). FungalTraits: A user-friendly traits database of fungi and fungus-like stramenopiles. Fungal Divers. 105, 1–16. doi:10.1007/s13225-020-00466-2
R Core Team (2021). R: A language and environment for statistical computing. Vienna, Austria: R Foundation for Statistical Computing. Available at: https://www.R-project.org/.
Schoch, C. L., Seifert, K. A., Huhndorf, S., Robert, V., Spouge, J. L., Levesque, C. A., et al. (2012). Nuclear ribosomal internal transcribed spacer (ITS) region as a universal DNA barcode marker for Fungi. Proc. Natl. Acad. Sci. U. S. A. 109, 6241–6246. doi:10.1073/pnas.1117018109
Sherwood, S., and Uphoff, N. (2000). Soil health: Research, practice and policy for a more regenerative agriculture. Appl. Soil Ecol. 15, 85–97. doi:10.1016/S0929-1393(00)00074-3
Spatafora, J. W., Chang, Y., Benny, G. L., Lazarus, K., Smith, M. E., Berbee, M. L., et al. (2016). A phylum level phylogenetic classification of zygomycete fungi based on genome scale data. Mycologia 108, 1028–1046. doi:10.3852/16-042
Tedersoo, L., Sánchez-Ramírez, S., Kõljalg, U., Bahram, M., Döring, M., Schigel, D., et al. (2018). High-level classification of the Fungi and a tool for evolutionary ecological analyses. Fungal Divers. 90, 135–159. doi:10.1007/s13225-018-0401-0
Trouvelot, A., Kough, J. L., and Gianinazzi-Pearson, V. (1986). “»Mesure du taux de mycorhization VA d’un systeme radiculaire. Recherche de methods d’estimation ayant une signification fonctionnelle,” in « in physiological and genetical aspects of mycorrhizae. Editors V. Gianinazzi-Pearson, and S. Gianinazzi (Paris): INRA), 217–221.
Voglar, D., and Lestan, D. (2013). Pilot-scale washing of Pb, Zn and Cd contaminated soil using EDTA and process water recycling. Chemosphere 91, 76–82. doi:10.1016/j.chemosphere.2012.12.016
White, T. J., Burns, T., Lee, S., and Taylor, J. (1990). “Amplification and direct sequencing of fungal ribosomal RNA genes for phylogenetics,” in PCR Protocols: A Guide to Methods and Applications. Editors M. A. Innis, D. H. Gelfand, J. J. Snisky, and T. J. White (San Diego: Academic Press), 315–322. doi:10.1016/b978-0-12-372180-8.50042-1
Keywords: heavy metals, arbuscular mycorrhiza, remediation, revitalisation, secondary succession, biodiversity, qPCR, toxic metals pollution
Citation: Maček I, Pintarič S, Šibanc N, Rajniš T, Kastelec D, Leštan D and Suhadolc M (2022) Plants play a crucial role in the development of soil fungal communities in the remediated substrate after EDTA washing of metal-contaminated soils. Front. Environ. Sci. 10:978850. doi: 10.3389/fenvs.2022.978850
Received: 11 July 2022; Accepted: 31 August 2022;
Published: 19 September 2022.
Edited by:
Ravi Naidu, University of Newcastle, AustraliaReviewed by:
Shobit Thapa, National Bureau of Agriculturally Important Microorganisms (ICAR), IndiaCopyright © 2022 Maček, Pintarič, Šibanc, Rajniš, Kastelec, Leštan and Suhadolc. This is an open-access article distributed under the terms of the Creative Commons Attribution License (CC BY). The use, distribution or reproduction in other forums is permitted, provided the original author(s) and the copyright owner(s) are credited and that the original publication in this journal is cited, in accordance with accepted academic practice. No use, distribution or reproduction is permitted which does not comply with these terms.
*Correspondence: Marjetka Suhadolc, bWFyamV0a2Euc3VoYWRvbGNAYmYudW5pLWxqLnNp
Disclaimer: All claims expressed in this article are solely those of the authors and do not necessarily represent those of their affiliated organizations, or those of the publisher, the editors and the reviewers. Any product that may be evaluated in this article or claim that may be made by its manufacturer is not guaranteed or endorsed by the publisher.
Research integrity at Frontiers
Learn more about the work of our research integrity team to safeguard the quality of each article we publish.