- 1College of Agronomy, Northwest A and F University, Xianyang, Shaanxi, China
- 2National Agriculture Research Center, Islamabad, Pakistan
Microplastics (MPs) in soil cause severe damage to the ecological environment and organisms, and research on their health risks has received extensive attention, but there is no comprehensive review of this research. From the perspective of bibliometrics, this paper systematically and comprehensively describes the progress, trends, and hotspots of health risks of MPs in soil based on the Web of Science Core Collection, Pubmed, and Scopus databases. Since 2016, people’s research on the health risks of MPs in soil has increased yearly; MPs in soil mainly come from Plastic mulch, Plastic waste, Sludge and sewage, and Organic fertilizer. China has the most publications on the health risks of MPs on soil, and more than half of the top 10 institutions with active publications in this field are from China. This paper systematically expounds on the health risks of MPs to organisms (plants, humans, and microorganisms) and control measures. Cooperation between different countries/institutions and fields/disciplines on the health risk analysis of MPs in soil and research on more efficient, green, and environmentally friendly methods and technologies for reducing soil MPs content will become the frontier trends of future development which provide valuable reference and help for future researchers.
1 Introduction
Since 2014, due to the convenience, durability, and low cost of plastics, people’s large-scale use of plastics has led to the global annual output of plastics exceeding 300 million tons in 2015, an increase of nearly 84 million tons compared with 2004, but only 6%–26% of them is recycled, which means that 74%–94% will end up in landfills or discharged into the ecological environment through various human activities (Barnes et al., 2009; Dayaratne and Gunawardana, 2015; Pittmann and Steinmetz, 2016). Once plastic enters the ecological environment, it will threaten the safety of the ecosystem, such as the release of toxic and endocrine substances, such as bisphenol A (Sajiki and Yonekubo, 2003), and as an adsorbent for other toxic pollutants (heavy metals and polychlorinated biphenyls) (Ashton et al., 2010; Velzeboer et al., 2014). As the COVID-19 outbreak in 2019 gained global attention, it led to a sudden and dramatic increase in the number of people hospitalized with multi-organ disease pneumonia worldwide. On 11 March 2020, COVID-19 was declared a pandemic by the World Health Organization (WHO), posing a massive threat to global public health due to the absence of specific treatments and vaccines for disease control (Wiersinga et al., 2020; Yan-Chao Li et al., 2020). Then people began to pay more attention to health risks, such as healthy food, clean water, healthy soil, and a healthy ecological environment.
Plastic fragments can shatter at sea and on land, with large plastic fragments breaking down to form microplastics (defined here as >25 mm), mesoplastics (5–25 mm), microplastics (0.1–5 mm), and nano plastics (<100 nm) (Claessens et al., 2011; Jahnke et al., 2017). According to the different sources of MPs, they can be divided into primary plastics and secondary plastics (Cole et al., 2011). Primary MPs are mainly derived from plastic particles from personal care products (exfoliants, facial cleansers) and factory effluents (Browne et al., 2011; Prata, 2018b). Secondary plastics are the result of hydrolysis, biodegradation (agricultural mulch), photodegradation, and mechanical wear (automotive tires) of larger plastics in the environment (Mattsson et al., 2015; McDevitt et al., 2017). Soil is a fragile, non-renewable resource whose health and sustainable development are affected by human activities and natural processes (X. Guo et al., 2022; Keesstra et al., 2016; K. Liu et al., 2022). MPs smaller than 1 mm are easily ingested by organisms in the soil. These MPs act as a medium for different organisms in the soil to communicate with their surrounding environment, thereby causing adverse effects on the survival and adaptability of organisms and further harming the health of organisms (Lusher et al., 2014; Auta et al., 2017; Amy L). MPs particles can also enter the soil microstructure through agglomeration, thereby directly changing soil porosity, water-holding capacity, bulk density, and soil structural integrity (Machado et al., 2018; Wan et al., 2019).
MPs pollution is considered one of the most important influencing factors for destroying biodiversity on a global scale. In recent years, the scientific community’s attention to MPs has increased significantly, and there is abundant evidence that MPs may have adverse effects on soil (Huerta Lwanga et al., 2016) and coastal and marine biota (A. L. Lusher et al., 2017; Sutherland et al., 2010). In addition, MPs also pose a significant challenge to human health, and the intake of MPs usually does not cause direct fatal injury but may have chronic adverse effects (Wright et al., 2013; Van Cauwenberghe and Janssen, 2014).
Bibliometrics is the quantitative analysis of patterns in scientific literature to review a field of research and analyze its emerging trends (Carve et al., 2021; C. Chen et al., 2012). Compared with traditional reviews, scientometric reviews are more systematic, comprehensive, and objective. To date, scientometric analysis has been applied in many research fields, such as urban metabolism (Xinjing Wang et al., 2021), environment and energy (Niu et al., 2021), ecosystem services (Bojie Wang et al., 2021), polycyclic aromatic hydrocarbon biology (Xian Chen et al., 2021), and capacitive deionization (Pang and Shen, 2022). However, since the 21st century, MPs have emerged pollutants in soil systems. Despite increasing attention to their dynamics in recent years and increasing research results in this field, the health risk analysis of MPs in soils still lacks a systematic scientometric view. Mapping and visualizing the structure and dynamics of this research area enable the rapid organization of a large number of published articles, and subsequent analysis can identify major research themes and fronts (X. Chen and Liu, 2020). Here, we use CiteSpace software to visualize, scientometric analysis, and map the field of MPs’ health risk research in soil. In this paper, we performed a scientometric analysis of the health risks of MPs in soil. The main objectives are to 1) elucidate an overview of research on health risks of MPs in the soil in terms of annual yields and primary sources; 2) analyze the most influential research forces (national, institutional, and journals); 3) Determine the health risks of MPs to plants, people, and soil microorganisms and what measures should be taken to mitigate the emerging environmental threats of MPs in soil; 4) Predict emerging trends, possible opportunities, and challenges in the health risks of MPs in soil. We hope this article can provide readers with an unbiased and comprehensive picture of the current status and trends of MPs’ health risks in soil.
2 Methodology and data
2.1 Data source and retrieval
Articles related to the health risks of MPs in soil were retrieved from the three databases of Web of Science Core Collection (Science Citation Index Expanded (SCIE), Social Sciences Citation Index (SSCI)), Pubmed, and Scopus on 15 May 2022. The specific retrieval strategy is shown in Figure 1. In order to avoid bias due to the daily update of the database, the articles required for the retrieval were conducted within 15 May 2022, and the retrieval time range was set in the 21st century (1 January 2000-31 December 2021), and articles published after 1 January 2022, were excluded, as any collection for that year from this period onwards would include incomplete bibliometric data for that year. After reading the full text through manual screening, excluding articles not related to the subject content, 435 articles were retrieved.
2.2 Statistical methods
CiteSpace software is based on Java applications for data analysis and visualization, focusing on finding critical points in a field, especially turning points. In the development of a field, transforming some of the traditional labor burdens of content analysis into computer algorithms and interactive visualizations facilitates a comprehensive analysis of developments in this field (C. M. Chen, 2006). Content analyzed by CiteSpace includes countries, institutions, and keywords. The time-slicing was set to 2000–2021 with 1 year per slice; the source terms selected were title, abstract, author, keywords, and keywords plus; and the threshold settings were the top 50 (Top N) and 10% (Top N%), respectively. A visual atlas can represent the results of health risk analysis of MPs in the soil, and in the knowledge domain mapping, each node represents an item, such as a country or an institution, between them. The size of the circle represents the frequency of keyword occurrence. The larger the purple circle, the more critical the node’s status; the color of the line represents different times, and the number of lines represents the coupling strength between the index nodes, and the thicker the line, the stronger the cooperative relationship. The analysis results can reflect the development speed and progress of research on the health risk of MPs in the soil to a certain extent and reflect the concentration and fluctuation of research in this field. Centrality refers to the strength of the correlation between the index node and other nodes in a particular measurement analysis content. The higher the centrality, the stronger the correlation and the greater the importance of the indicator.
In Eq. 1, ρjk represents the number of shortest paths between node j and node k, a n d ρjk 1) is the number of those paths that pass-through node i.In CiteSpace, nodes whose betweenness centrality exceeds 0.1 are called key nodes. At the documental level, the importance of each document in a co-citing network can be partially evaluated by the indicator between-ness centrality (C. M. Chen, 2006; Ghisi et al., 2020).
3 Results
3.1 Characteristics of publication outputs
After literature identification and screening, 435 pieces of literature about the health risk of MPs in soil were extracted (Figure 2). It can be seen that since the 21st century, the first article on the health risk field of MPs in soil was published in 2009, which has attracted widespread attention since then. There were few articles in this field in the subsequent time, and people mainly focused on the research of marine MPs (Andrady, 2011; Cole et al., 2011; Lebreton et al., 2012). Since 2016, with the strengthening of the concept of global sustainable development and low-carbon environmental protection, especially in recent years with the global COVID-19 (Guan et al., 2020; Huang et al., 2020; Wu and McGoogan, 2020), people have paid more and more attention to the concept of health. Many studies have been carried out in the field of MPs’ health risk, and the number of publications has been increasing, reaching a peak of 213 in 2021. It can be seen that this field has become a research hotspot and will become a future research trend.
3.2 Major sources of health risks from soil microplastics
In order to find a reasonable way to manage MPs in soil and conduct a comprehensive and comprehensive health risk analysis of MPs in soil, a significant part is to explore its primary sources. Figure 3 shows the ten principal sources of MPs in soil.
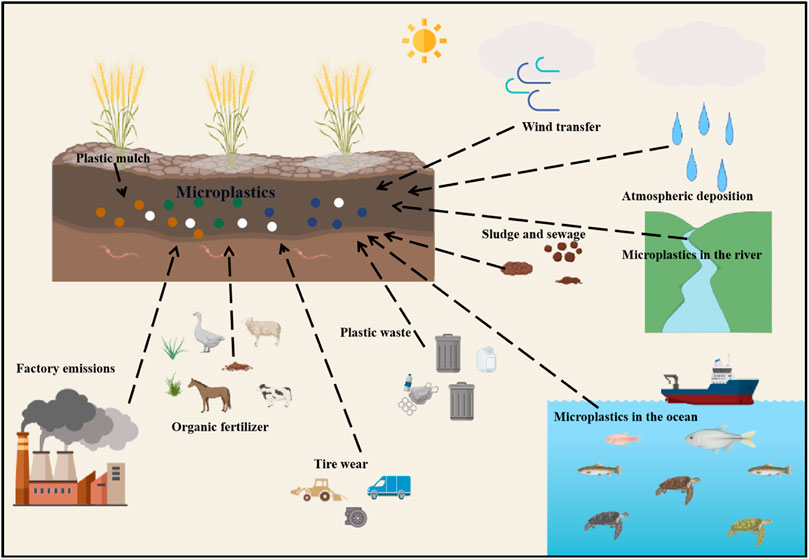
FIGURE 3. Sources of health risks from soil microplastics.(MPs in soil mainly come from agricultural mulch film, plastic waste, sludge, and sewage discharged from factories, organic fertilizers applied on farmland, atmospheric deposition, fine particles from road tire wear, and the migration of plastic debris in oceans and lakes).
3.2.1 Plastic mulch
Plastic mulch was first noticed in 1950 to increase soil temperature (M. Liu et al., 2018). The number of plastic mulch films widely used in agriculture is increasing year by year, and its introduction has wholly changed the commercial production mode of traditional crops and brought substantial economic benefits to human beings (Steinmetz et al., 2016). Plastic film mulching will increase soil carbon and nitrogen metabolism, deplete organic matter storage, help maintain soil moisture and temperature, and promote nutrient cycling (Zhang et al., 2015). However, as one of the primary sources of MPs, plastic mulch is not biodegradable and has specific toxicity. When the plastic mulch is embedded in the soil, it will undergo multiple processes such as fragmentation, aging, and biodegradation, and finally be converted into MPs, causing severe pollution to soil health and toxicity to various soil microorganisms and plants (Blaesing and Amelung, 2018). Therefore, it is imperative to find a material to replace the plastic mulch.
3.2.2 Plastic waste
Some plastic products (plastic bottles, shopping bags, packaging bags) have been used in large quantities, and plastic products have become increasingly dominant in the consumer market (Jambeck et al., 2015). According to statistics, in 2008 alone, the International Coastal Conservation Association removed 1,377,141 plastic bags (Roman et al., 2021). The widespread use of plastics has caused severe “white pollution” in many areas (Horton et al., 2017). The severe pollution of MPs in the soil is inseparable from the massive reuse of single-use plastic products. When plastic products are improperly used and discarded by humans, much plastic debris accumulates in the environment. During the degradation of these plastics, the additives and toxic substances in the plastics will be released, breaking the balance of the ecological environment, causing severe pollution, and it is easy to accumulate in the soil to produce poison. The development of alternatives to plastic products and policies to restrict plastics in some countries will become the future trend.
3.2.3 Sludge and sewage
As a biological residue, sewage sludge is often used in agriculture as an alternative disposal technology for waste. Therefore, an essential source of direct input of MPs to soil is sewage sludge containing plastic sediments (Horton et al., 2017). Since a sewage treatment plant can efficiently remove most MPs particles in wastewater, many of the removed particles will remain in the sludge (Iqbal et al., 2020). Studies have shown that through the index detection of sewage sludge produced by sewage discharge plants, it is found that the MPs in it are as high as 15,385 items/kg (Athey and Erdle, 2022). In addition, the European Union has also researched sewage sludge and found that about 5 million tons of dry weight sewage sludge are used for arable land every year, causing severe MPs pollution. (Willen et al., 2017). Although there are regulations for hazardous substances in sludge applied to land, these do not consider MPs (Nizzetto et al., 2016). Improper treatment methods can increase soil MPs contamination to alarming levels and pose a significant risk to human health. Therefore, using new technologies and methods is of great significance in improving sludge treatment, disposal efficiency, and utilization (Corradini et al., 2019).
3.2.4 Organic fertilizer
Organic fertilizers provide vital crop nutrients for agricultural, forestry, and horticultural production, which can increase the activity of microorganisms in the soil, improve soil quality, and stabilize the physical and chemical properties of soil. (Karimi et al., 2018). Composting organic waste is essential for producing organic fertilizers, reducing the need for chemical fertilizers. However, extensive application of organic fertilizers may lead to MPs contamination in the soil, resulting in residual toxic substances (T. Guo et al., 2018). Studies have shown that organic fertilizers are one of the significant sources of neglected MPs in the environment (Weithmann et al., 2018). Scientists find plastic content of up to 1,200 mg kg−1 in organic compost in Slovenia (Lagace et al., 2017). Therefore, there is an urgent need to assess the impact of organic fertilizer application on the accumulation of MPs in agricultural soil and balance the pros and cons of using biological waste for fertilizer production.
3.2.5 Others
In addition, the MPs in soil include sewage discharged from various chemical plants (Kim et al., 2006); fine particles (containing rubber, mainly synthetic polymers) from road tire wear (Wik and Dave, 2009); atmospheric deposition, and wind-driven plastic debris (Dris et al., 2015); and the migration of plastic debris in oceans and lakes (Gregory, 2009; Horton et al., 2017). MPs appear almost everywhere and have caused severe health risks to the ecological environment, and some measures and technologies are urgently needed to improve.
3.3 Analysis of research countries (regions), institutions and journals
In order to further explore the cooperation between countries, the top 10 countries in the field of published papers (Table 1), the geographic distribution of the top 10 countries (Figure 4), and the top 20 countries through the Cite Space software were compiled. They were drawing the map (Figure 5). China has published the most significant number of papers in this field (the largest node), with 174 papers, accounting for 40.00% of the global total, with a centrality of 0.18. The first paper was published in 2012, and the cooperation with countries such as Australia and England started in 2012 Start; followed by the United States (76%, 17.47%, 0.29); the first article was also published in 2012; it is worth noting that the centrality of the United States is the largest (the outer ring has the deepest purple), indicating that there are many high-quality and high-quality articles. China, the United States, and Australia have the most representative and authoritative research in this field, and the number of published papers exceeds 50% of the total number of papers in the world, occupying a dominant position in the field of MPs health risk research in soil. Among the top ten countries in terms of the number of published papers, only China and India are developing countries, and the remaining 8 are developed countries. It can be seen that developed countries attach more importance to environmental health and have more authoritative scholars and more financial and technical support in this field.
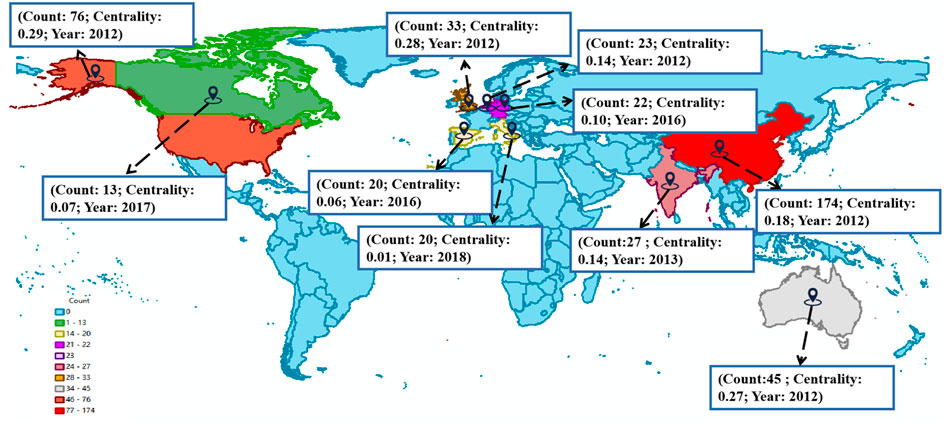
FIGURE 4. Geographical distribution of the top ten countries.Count: Number of publications by country. Year: The year the publications were originally published in a country. Centrality: The importance of each article in the co-citation network.(The color of the top ten countries in the figure represents the number of their posts.The China region in the picture only includes the mainland region).
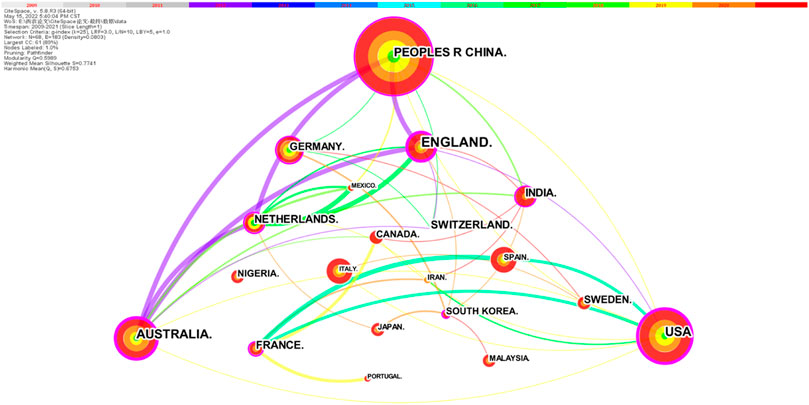
FIGURE 5. Country/Region cooperation knowledge graph. (Nodes represent country/region. The size of a node is proportional to the literature number of the country/region. The links represent the co-occurring relationship between different subject categories. The color of the rings and links corresponds to the year. The purple rings indicate high centrality).
In the same research field, the cooperation between institutions can best reflect the relationship between organizations, professional information, and institutions. The top ten institutions in this field by the number of published papers are counted (Table 2), and the top 20 research institutions are conducted through Cite Space software Mapping the inter-agency partnership map (Figure 6). The Chinese Academy of Sciences in China is the most productive institution. It has published 30 articles on the health risk research of MPs in soil, accounting for 6.90% of the global total. The centrality is the largest at 0.09. Agency, whose first article was published in 2018. Among the top 10 institutions in terms of publication volume, eight are from China, which shows that China has contributed the most. On the other hand, it can be seen that most institutions cooperate among institutions in the same country, and there is less cooperation between multinational institutions. Observing the cooperation between existing institutions will help future researchers to find potential partner institutions faster and more accurately and help more institutions to carry out cross-border cooperation.
Among the top ten journals in terms of publication volume (Table 3), the publication volume, TCa value, and h-index value (81; 5,414; 32) of the journal Science of the Total Environment are the highest, indicating that the journals published in the journal have The overall quality of the articles on MPs health risk analysis in the soil is high, and more authoritative scholars have contributed to this journal. On the other hand, the journal has a significant influence. Among the top ten journals in terms of publication volume, except for “Environmental Science and Pollution Research” and “International Journal of Environmental Research and Public Health,” the remaining eight journals (80%) have IF more remarkable than five and JCI greater than 1, with Significant international influence.
3.4 Health risks of MPs to different organisms
3.4.1 Plants
The entry of MPs into the soil can cause serious environmental problems, especially related to some characteristics of plants (H. Liu et al., 2017). As the starting point of biosphere cycle accumulation, plants are inseparable from human life (Li Chen et al., 2021). Residual MPs in the soil can be quickly absorbed by plants and accumulate in large quantities in plants, which may cause toxic effects (Jiang et al., 2019), like inhibiting plant nutrients, affecting plant growth and development, hindering photosynthesis in plants, and making lipid membrane oxidation and genotoxic effects, changes in enzyme activity, etc., lead to plant degradation or death (Dong et al., 2020; Maity et al., 2020). Some studies have shown that MPs in the soil can accumulate in large quantities in plant roots, affecting plant root growth and traits (root length, root rooting ability, root activity, etc.) (Machado et al., 2019; Zhenxia Li et al., 2020; Meng et al., 2021). Wu et al. (2021) explored the effects of polystyrene MPs of different sizes (0.1 and 1 μm) on rice. They found that the length of primary roots of rice was reduced, nutrient absorption was inhibited, and lateral roots were stimulated. 1 μm MP exhibited stronger phytotoxicity than 100 nm MP; Hu et al. (2020) explored the effect of MPs on maize and found that MPs had adverse effects on root and stem growth in several growth stages of maize crops, significantly when it exceeded 300 kg ha−1. In addition, some studies have also shown that MPs can promote root growth. MPs in the soil can increase the biomass of plant roots, mainly by expanding the root system and increasing the root/cap of plants to meet water and nutrient requirements (Maskova and Herben, 2018). The absorption and transfer of nutrients by plant tissues is a complex biological process, and MPs in soil may be toxic to plants by disrupting the balance of plant hormones, nutrient metabolism, and nutrient absorption (X. Wu et al., 2020). Studies have shown (Li et al., 2021) that MPs with a concentration of 2 g ml−1 and a size of 5.64 ± 0.07 µm disrupt redox homeostasis, carbohydrate metabolism, and phytohormone regulatory networks in barley. Zhou et al. (2021) found that the complex effect of MPs and Cd at 10 mg L−1 could change leaves through energy metabolism and reduce the Zn concentration in wheat tissues. Table 4 shows the research on the response of 10 different plants to MPs, and the effects produced by different plants are also different. Overall, most studies on plant responses to MPs in soil are short-term pot experiments using soils containing MPs, which can only provide rapid stimulation of plants by MPs but not chronic responses.
3.4.2 Humans
The presence of MPs poses a considerable risk to human health as massive plastic waste increases globally (Cole et al., 2011). A study has shown that because MPs in soil are widely present in the food chain of nature, MPs can enter the human food chain and become toxic to humans in various ways (Vethaak and Leslie, 2016; Ohlwein et al., 2019). Such as some animals (cattle, sheep, chicken, duck) that people eat in their lives, these animals are rich in MPs by eating MPs-containing plants in the natural environment (Santillo et al., 2017); and food processing and production processes MPs produced in contamination or MPs leached from plastic packaging of some food and beverages (Karami et al., 2017; Mason et al., 2018). MPs inhaled into the nasal cavity are mainly derived from urban dust in the air, including synthetic textiles and rubber tires, and atmospheric deposition (Prata, 2018a). Although the skin membrane is too thin for some daily cosmetics containing MPs, shampoos, etc., to pass directly, they may enter the human body through wounds, sweat glands, or hair follicles (Schneider et al., 2009). Since the maximum functional size of the associated pores at tight junction channels in humans is around 1.5 nm, MPs are unlikely to penetrate at the paracellular level (Farrar and Schreiber, 1993). Nevertheless, MPs are likely to enter lymphoid tissue with larger pores, and they may enter via phagocytosis or endocytosis and infiltrate the microfold (M) cells in the Peyer’s patches (Nelms et al., 2017).Particles of MPs may also penetrate deep into human lungs and then accumulates on the surface of the alveoli, potentially causing severe damage to the lungs (Porter et al., 2010). Wang et al. (2020) studied the intake of polystyrene plastics with five different particle sizes (0.3, 0.5, 1, 3, and 6 μm) by colon cancer Caco-2 cells and found that different particle sizes of Polystyrene MPs were toxic to colon cancer Caco-2 cells, and the toxicity mainly depended on particle size and concentration. Forte et al. (2016) found that unaltered or carboxylated polystyrene nanoparticles significantly upregulated IL-6 and IL-8 genes in human gastric adenocarcinoma, leukemia, and histiocytic lymphoma cells. In addition to this, some effects, such as changes in lipid metabolism, were observed in the offspring of mice by oral administration of a mixture of MPs(Luo et al., 2019). There are three critical pathways for MPs to enter the human body (Figure 7): 1) Microplastics enter the human body through the nasal cavity in the form of airborne particles; 2) Microplastics enter the human body through the mouth in the form of food; and 3) Plastic products and cosmetics containing microplastics enter the human body through contact with the skin. There are few studies on the risk analysis of MPs in soil on human health, and further research is needed to study the effect of MPs on the human body in the future: potential toxicity mechanisms and treatment and preventive measures.
3.4.3 Soil microorganisms
As the leading life form of the soil biological cycle, soil microorganisms are the prominent participants in the soil nutrient cycle (Crawford et al., 2005; Lowery and Ursell, 2019). Different soil microorganisms can hinder or inhibit plants (Bever, 1994). Soil microbial communities can lay the foundation for plant growth and development (Classen et al., 2015; Smith-Ramesh and Reynolds, 2017). Soil microbes can also indirectly affect plants, and through mediated methods, they interact with different plants, giving plants species-specific advantages (Hartnett and Wilson, 1999; Smith et al., 1999). However, the presence of MPs in soil has several adverse effects on microorganisms, such as decreased growth rate, reduced microbial biomass, slower energy regeneration (metabolic rate), and blocked synthesis of new macromolecules for cytoprotective purposes (Abbasi et al., 2020; Bhatt et al., 2021), MPs can also attach to the secretions of microorganisms, thereby affecting enzyme activity and carbon (C) cycling in soil (Rillig et al., 2017). After MPs pollutes the soil, they will produce different microbial environments, change the composition of the microbial community in the soil, enrich the community members involved in the degradation of MPs in the soil, and affect the function of the microbial community (Yu et al., 2021), and also make The diversity of microbial activities in bacterial communities decreased (Fei et al., 2020).Liu et al. (2017) conducted soil incubation experiments in a climate-controlled room and found that microplastic addition stimulated luciferin diacetate hydrolase (FDAse) s soil activity and that higher levels of microplastic addition significantly increased the nutrient composition of organic matter solutions. Machado et al. (2018), in a 5-weeks garden experiment, found that the correlation of MPs with microbial activity may be related to the inadequate response of the whole process. In future studies, we should consider the effects of different factors affecting soil microbes (climate, rainfall, sunshine, and land type) on MPs and further investigate the effects of MPs on microbial activity and function in soil.
3.5 Countermeasures for soil microplastics
Based on the mass production of plastics around the world and the improper disposal of plastic waste by humans (Wright et al., 2013; Van Cauwenberghe and Janssen, 2014), and our study of annual changes in the number of publications on the health risks of MPs in soil, MPs’ output will continue to increase in the future. However, MPs’ massive emission and accumulation may lead to varying degrees of destruction of soil-plant systems, soil-human systems, and soil-microbe systems. Therefore, there is an urgent need to reduce the contamination of MPs in the soil and mitigate its risk. The main measures to reduce MPs pollution include substituting new materials, policies, sustainable methods, and emerging technologies.
3.5.1 Substitution of novel materials
The growing global focus on issues such as sustainability means that these non-biodegradable materials will eventually be phased out. Natural biopolymers have attracted much attention among the various polymers currently produced due to their easy availability, low cost, biocompatibility, unique properties, and wide range of uses (Koh et al., 2015; Huber et al., 2017), and a large number of related products have been Instead of plastic products, such as brackets (Arango et al., 2021), food packaging bags (Rhim and Ng, 2007) and films, etc.(Kasirajan and Ngouajio, 2012). In addition, biodegradable materials are also an important measure to replace plastic products, which can be produced from renewable resources, fossil fuels, or a mixture.(Vroman and Tighzert, 2009). Its new definition refers to materials that microorganisms can degrade with no residues or new biogenesis (Orgiles-Calpena et al., 2014; Siwek et al., 2019). Among them, the most common is the replacement of traditional plastic mulch with biodegradable mulch in agriculture.
3.5.2 Policies and sustainable methods
Many MPs’ pollution in soils is mainly due to inadequate planning and policy interventions for plastic waste management. With the emphasis on health, the development/implementation of solid policies and sustainable approaches must begin to ameliorate pollution from MPs and reduce their adverse effects on the environment and human health. The scientific community has made recommendations to governments and the public to encourage the process of reforming existing plastic waste management models (Kulkarni and Anantharama, 2020; Parashar and Hait, 2021). In addition to replacing plastic materials with novel materials, implementing public health conservation strategies can also help reduce waste generation. For example, the World Health Organization recommends minimizing the need for protective equipment by maintaining social distancing in the outside world (Leddin et al., 2020).
Governments can levy landfill taxes to local municipalities based on landfill plastic waste (Kulkarni and Anantharama, 2020), provide consumers with recycling benefits (e.g., buy-back programs for plastic bags and plastic bottles), and use intelligent sorting bins for waste sorting, the recycling of plastic products. Taking China as an example, treatment equipment such as Sterilwave SW440 is applied in hospitals (sterilized by microwave at 110°C, with a processing capacity of up to 80 kg/h). After sterilization, biomedical plastic waste no longer poses a threat to public health and can follow conventional waste streams to appropriately end the useful life of these materials (Ilyas et al., 2020). The central authority in Taiwan Province of China, the Environmental Protection Agency (EPA), has also promulgated laws on reducing and recycling plastic waste, intending to increase the total amount of recycled plastic containers to 194,133 tons in 2019 (Tsai, 2021).
3.5.3 Emerging technologies
Some emerging technologies are also necessary means of dealing with MPs, such as: Implementing a biorefinery at (or near) landfills, provided that plastic waste is separated from mixed waste, will help reduce on-site plastic waste and indirectly reduce waste Converted to the cost of an energy plant (Madadian et al., 2020). There is also a strategy to reduce plastic in landfills by using plastic compactors, which melt plastic waste into a disc, reducing the amount of water available for biodegradation, pollutant adsorption, and monomers and additives Leached surface area (Inghelbrecht and Remon, 1998). In addition, some microorganisms in the soil environment decompose MPs through their activities (Ball, 2017), and studies have shown that some polyethylene-degrading bacteria can be isolated from the Waxworm body, and polystyrene-degrading bacteria can be isolated from the Tenebrio molitor (Yang et al., 2015). Bioreactor technology can transform and stabilize MPs within 5–10 years through enhanced microbial processes, and with the help of crucial microorganisms, this technology can even improve plastic degradation, significantly increasing the decomposition, conversion rate, and processing efficiency of MPs(Kumar et al., 2011). Metabolomics technology can understand exogenous disturbances in biological systems, understand soil metabolites and metabolic pathways under the influence of exogenous pollutants, and interact with microorganisms in the soil to obtain additional potential degradation biomarkers (Jones et al., 2014; Matich et al., 2019). Munir et al. (2021), by using different dosages of MPs to modify biochar, found that the modification of biochar by MPs can repair the toxicity of Cr and Pb in soil, providing safe disposal for MPs waste and an alternative convenience strategy.
3.6 Hotspots and trend directions of MPs research
Outbreak detection can be used to find keywords of particular interest to the scientific community at a specific time so that we can determine the development of keywords and potential research topics (Keesstra et al., 2016); the appearance time, end time, and keyword strength of keywords can be seen, Predict future research trends in hot areas. Therefore, outbreak keywords can be used as indicators to investigate research fronts and predict research trends. This paper uses CiteSpace software to perform outbreak detection based on the keywords appearing in the article (Table 5).
The keywords “litter,” “marine debris,” and “accumulation” appeared the earliest and appeared for the first time in 2009. The burst intensities of these three keywords were 4.25, 2.92, and 2.37, respectively, which shows that they are significant for the research of MPs. Mainly from Gregory, MR (Gregory, 2009), “Environmental implications of plastic debris in marine settings-entanglement, ingestion, smothering, hangers-on, hitch-hiking and alien invasions” on land-derived marine debris (“plastic and other synthetic non-biodegradable materials”) have caused severe pollution to the sea and various waters, thus causing significant damage to natural health and species habitat environment. In the next few years, people’s research on MPs mainly Focused on the ocean; less research has been done on MPs on land and soil. The keyword “plastic debris” first appeared in 2017 but had the highest burst intensity with a value of 4.49. It continued to burst into 2021 because the fragmentation of plastic fragments produces MPs or as pre-production pellets or components of consumer industrial products are released into the environment.
In addition, there are 9 keywords that will continue to break out until 2021 and will become future research hotspots and directions, namely “polymer,” “lake,” “polystyrene microplastics,” “chemical,” “china,” “phthalate ester,” “carbon,” “extracting microplastics” and “environmental risk”.
Among them, the appearance of the keyword “China” is because China is currently in a leading position in the field of research on the health risks of MPs in soil, and the number of published papers ranks first in the world, far surpassing the second-ranked United States, which shows that China is in the field of this field. It has made significant contributions and has excellent international influence and status.
The emergence of “lake,” “carbon,” “extracting microplastics,” and “environmental risk” is because the currently emerging MPs pose severe risks to the environment, destroying the ecological balance and biodiversity. MPs from lakes, rivers, land, and other pathway inputs through which plastic concentrations in soils may reach the per-unit range of soil organic carbon (Xu et al., 2021). Most of these plastics are likely to remain in the soil for decades or more, with immeasurable consequences, so there is an urgent need for a way to address this dilemma. Huber et al., 2017 demonstrated the suitability of Fenton’s reagent in conjunction with density separation to extract MPs by experimentally analyzing the removal of organic material during MPs in complex solid matrices. While Koh et al., 2015 provided an improved and optimized process for the extraction of MPs particles by improving the flotation technology and the flotation solution, using a mixture of NaCl as the flotation solution.
“polymer,” “polystyrene microplastics,” “chemical,” and “phthalate ester” appear because, as the main chemical constituents of MPs, once they enter the environment such as soil, they will be toxic to organisms, and the persistence of adsorption and Toxic desorption elements such as organic pollutants and heavy metals accumulate in the tissues of organisms and may biomagnify along the food chain (Arango et al., 2021). Therefore, further studies on the prevalence and fate of this synthetic polymer in soil are urgently needed.
4 Discuss
(1) The health risks to MPs were mainly concentrated in marine ecosystems at the beginning (A. L. Lusher et al., 2017; Sutherland et al., 2010) and gradually began to turn to MPs research in soil ecosystems. The effect of MPs on soil pH, trace elements, water, and nutrients needs to be further studied by scientists in the future.
(2) The current research on the health risks of MPs in soil shows an increasing trend yearly. The most representative force in China is less cooperation between countries/institutions. Cooperation between different countries/institutions will become a development trend in the future.
(3) The current research on the health risks of MPs in the soil is mainly concentrated in the field of environmental science, and the health risks of plants, microorganisms, and humans are almost all acute adverse reactions caused by short-term experiments, while there are almost no chronic reactions in long-term experiments. Long-term experiments on the health risks of MPs and research on cross-disciplinary cooperation in various fields such as ecology and medicine are yet to be carried out.
(4) In the current research on the health risks of MPs in soil, almost only one pollutant is targeted at MPs, and the health risks are caused by the typical reaction of MPs with other harmful pollutants (heavy metals, organic pollutants, etc.) in the soil environment are lacking.
(5) At present, the control methods for MPs are still few and in their infancy, mainly suppressing at the root (reducing the use of plastic products) and using bioreactor technology (Kumar et al., 2011). One of the primary sources of MPs in the soil is organic fertilizer; the existence of MPs has been integrated into the cycle of the entire ecosystem. In the future, scholars need to carry out more work to study more efficient, green, and environmentally friendly ways to reduce MPs in soil—content methods and techniques.
5 Conclusion
We systematically reviewed the publication characteristics, representative forces, research status, hotspots, and prospects of MPs’ health risks in soil. At present, research on the health risks of MPs in the soil is still in its infancy, and in this study, readers can obtain exciting information from the rich bibliometric data, which has particular value and reference for future research.
Author contributions
YS: investigation, methodology, writing—original draft, Writing—review and editing CY: investigation, methodology HL: methodology SZ: Writing—review and editing RZ: methodology YD: writing—review and editing ST: methodology JH: conceptualization, project administration, funding acquisition, Writing—review and editing.
Funding
This study was supported by the Ecological Security and Bioremediation Mechanism of Saline-alkali Soil Improvement in the Middle Yellow River (No. DL2021172002L).
Conflicts of interest
The authors declare that the research was conducted in the absence of any commercial or financial relationships that could be construed as a potential conflict of interest.
Publisher’s note
All claims expressed in this article are solely those of the authors and do not necessarily represent those of their affiliated organizations, or those of the publisher, the editors and the reviewers. Any product that may be evaluated in this article, or claim that may be made by its manufacturer, is not guaranteed or endorsed by the publisher.
References
Abbasi, S., Moore, F., Keshavarzi, B., Hopke, P. K., Naidu, R., Rahman, M. M., et al. (2020). PET-microplastics as a vector for heavy metals in a simulated plant rhizosphere zone. Sci. Total Environ. 744, 140984. doi:10.1016/j.scitotenv.2020.140984
Andrady, A. L. (2011). Microplastics in the marine environment. Mar. Pollut. Bull. 62 (8), 1596–1605. doi:10.1016/j.marpolbul.2011.05.030
Arango, M. C., Montoya, Y., Peresin, M. S., Bustamante, J., and alvarez-Lopez, C. (2021). Silk sericin as a biomaterial for tissue engineering: A review. Int. J. Polym. Mater. Polym. Biomaterials 70 (16), 1115–1129. doi:10.1080/00914037.2020.1785454
Ashton, K., Holmes, L., and Turner, A. (2010). Association of metals with plastic production pellets in the marine environment. Mar. Pollut. Bull. 60 (11), 2050–2055. doi:10.1016/j.marpolbul.2010.07.014
Athey, S. N., and Erdle, L. M. (2022). Are we underestimating anthropogenic microfiber pollution? A critical review of occurrence, methods, and reporting. Environ. Toxicol. Chem. 41 (4), 822–837. doi:10.1002/etc.5173
Auta, H. S., Emenike, C. U., and Fauziah, S. H. (2017). Distribution and importance of microplastics in the marine environment: A review of the sources, fate, effects, and potential solutions. Environ. Int. 102, 165–176. doi:10.1016/j.envint.2017.02.013
Barnes, D. K. A., Galgani, F., Thompson, R. C., and Barlaz, M. (2009). Accumulation and fragmentation of plastic debris in global environments. Phil. Trans. R. Soc. B 364 (1526), 1985–1998. doi:10.1098/rstb.2008.0205
Bever, J. D. (1994). Feeback between plants and their soil communities in an old field community. Ecology 75 (7), 1965–1977. doi:10.2307/1941601
Bhatt, P., Pathak, V. M., Bagheri, A. R., and Bilal, M. (2021). Microplastic contaminants in the aqueous environment, fate, toxicity consequences, and remediation strategies. Environ. Res. 200, 111762. doi:10.1016/j.envres.2021.111762
Blaesing, M., and Amelung, W. (2018). Plastics in soil: Analytical methods and possible sources. Sci. Total Environ. 612, 422–435. doi:10.1016/j.scitotenv.2017.08.086
Bojie Wang, B., Zhang, Q., and Cui, F. (2021). Scientific research on ecosystem services and human well-being: A bibliometric analysis. Ecol. Indic. 125, 107449. doi:10.1016/j.ecolind.2021.107449
Browne, M. A., Crump, P., Niven, S. J., Teuten, E., Tonkin, A., Galloway, T., et al. (2011). Accumulation of microplastic on shorelines woldwide: Sources and sinks. Environ. Sci. Technol. 45 (21), 9175–9179. doi:10.1021/es201811s
Carve, M., Allinson, G., Nugegoda, D., and Shimeta, J. (2021). Trends in environmental and toxicity research on organic ultraviolet filters: A scientometric review. Sci. Total Environ. 773, 145628. doi:10.1016/j.scitotenv.2021.145628
Chen, C., Hu, Z., Liu, S., and Tseng, H. (2012). Emerging trends in regenerative medicine: A scientometric analysis in CiteSpace. Expert Opin. Biol. Ther. 12 (5), 593–608. doi:10.1517/14712598.2012.674507
Chen, C. M. (2006). CiteSpace II: Detecting and visualizing emerging trends and transient patterns in scientific literature. J. Am. Soc. Inf. Sci. Technol. 57 (3), 359–377. doi:10.1002/asi.20317
Chen, X., and Liu, Y. (2020). Visualization analysis of high-speed railway research based on CiteSpace. Transp. Policy 85, 1–17. doi:10.1016/j.tranpol.2019.10.004
Claessens, M., De Meester, S., Van Landuyt, L., De Clerck, K., and Janssen, C. R. (2011). Occurrence and distribution of microplastics in marine sediments along the Belgian coast. Mar. Pollut. Bull. 62 (10), 2199–2204. doi:10.1016/j.marpolbul.2011.06.030
Classen, A. T., Sundqvist, M. K., Henning, J. A., Newman, G. S., Moore, J. A. M., Cregger, M. A., et al. (2015). Direct and indirect effects of climate change on soil microbial and soil microbial-plant interactions: What lies ahead? Ecosphere 6 (8), art130. doi:10.1890/es15-00217.1
Cole, M., Lindeque, P., Halsband, C., and Galloway, T. S. (2011). Microplastics as contaminants in the marine environment: A review. Mar. Pollut. Bull. 62 (12), 2588–2597. doi:10.1016/j.marpolbul.2011.09.025
Colzi, I., Renna, L., Bianchi, E., Castellani, M. B., Coppi, A., Pignattelli, S., et al. (2022). Impact of microplastics on growth, photosynthesis and essential elements in Cucurbita pepo L. J. Hazard. Mater. 423, 127238. doi:10.1016/j.jhazmat.2021.127238
Corradini, F., Meza, P., Eguiluz, R., Casado, F., Huerta-Lwanga, E., and Geissen, V. (2019). Evidence of microplastic accumulation in agricultural soils from sewage sludge disposal. Sci. Total Environ. 671, 411–420. doi:10.1016/j.scitotenv.2019.03.368
Crawford, J. W., Harris, J. A., Ritz, K., and Young, I. M. (2005). Towards an evolutionary ecology of life in soil. Trends Ecol. Evol. 20 (2), 81–87. doi:10.1016/j.tree.2004.11.014
Dayaratne, S. P., and Gunawardana, K. D. (2015). Carbon footprint reduction: A critical study of rubber production in small and medium scale enterprises in Sri Lanka. J. Clean. Prod. 103, 87–103. doi:10.1016/j.jclepro.2014.09.101
Dong, Y., Gao, M., Song, Z., and Qiu, W. (2020). Microplastic particles increase arsenic toxicity to rice seedlings. Environ. Pollut. 259, 113892. doi:10.1016/j.envpol.2019.113892
Dris, R., Gasperi, J., Rocher, V., Saad, M., Renault, N., and Tassin, B. (2015). Microplastic contamination in an urban area: A case study in greater paris. Environ. Chem. 12 (5), 592–599. doi:10.1071/en14167
Enyoh, C. E., Verla, A. W., Verla, E. N., and Enyoh, E. C. (2020). Effect of macro- and micro-plastics in soil on quantitative phytochemicals in different part of juvenile lime tree (citrus aurantium). Int. J. Environ. Res. 14 (6), 705–726. doi:10.1007/s41742-020-00292-z
Farrar, M. A., and Schreiber, R. D. (1993). The molecular cell biology of interferon-gamma and its receptor. Annu. Rev. Immunol. 11, 571–611. doi:10.1146/annurev.iy.11.040193.003035
Fei, Y., Huang, S., Zhang, H., Tong, Y., Wen, D., Xia, X., et al. (2020). Response of soil enzyme activities and bacterial communities to the accumulation of microplastics in an acid cropped soil. Sci. Total Environ. 707, 135634. doi:10.1016/j.scitotenv.2019.135634
Forte, M., Iachetta, G., Tussellino, M., Carotenuto, R., Prisco, M., De Falco, M., et al. (2016). Polystyrene nanoparticles internalization in human gastric adenocarcinoma cells. Toxicol. Vitro 31, 126–136. doi:10.1016/j.tiv.2015.11.006
Ghisi, N. d. C., Zuanazzi, N. R., Carrenho Fabrin, T. M., and Oliveira, E. C. (2020). Glyphosate and its toxicology: A scientometric review. Sci. Total Environ. 733, 139359. doi:10.1016/j.scitotenv.2020.139359
Gregory, M. R. (2009). Environmental implications of plastic debris in marine settings-entanglement, ingestion, smothering, hangers-on, hitch-hiking and alien invasions. Phil. Trans. R. Soc. B 364 (1526), 2013–2025. doi:10.1098/rstb.2008.0265
Guan, W., Ni, Z., Hu, Y., Liang, W., Ou, C., He, J., et al. (2020). Clinical characteristics of coronavirus disease 2019 in China. N. Engl. J. Med. Overseas. Ed. 382 (18), 1708–1720. doi:10.1056/nejmoa2002032
Guo, T., Lou, C., Zhai, W., Tang, X., Hashmi, M. Z., Murtaza, R., et al. (2018). Increased occurrence of heavy metals, antibiotics and resistance genes in surface soil after long-term application of manure. Sci. Total Environ. 635, 995–1003. doi:10.1016/j.scitotenv.2018.04.194
Guo, X., Wang, H., Yu, Q., Ahmad, N., Li, J., Wang, R., et al. (2022). Subsoiling and plowing rotation increase soil C and N storage and crop yield on a semiarid Loess Plateau. Soil Tillage Res. 221, 105413. doi:10.1016/j.still.2022.105413
Hartnett, D. C., and Wilson, G. W. T. (1999). Mycorrhizae influence plant community structure and diversity in tallgrass prairie. Ecology 80 (4), 1187–1195. doi:10.1890/0012-9658(1999)080[1187:mipcsa]2.0.co;2
Horton, A. A., Walton, A., Spurgeon, D. J., Lahive, E., and Svendsen, C. (2017). Microplastics in freshwater and terrestrial environments: Evaluating the current understanding to identify the knowledge gaps and future research priorities. Sci. Total Environ. 586, 127–141. doi:10.1016/j.scitotenv.2017.01.190
Hu, Q., Li, X., Goncalves, J. M., Shi, H., Tian, T., and Chen, N. (2020). Effects of residual plastic-film mulch on field corn growth and productivity. Sci. Total Environ. 729, 138901. doi:10.1016/j.scitotenv.2020.138901
Huang, C., Wang, Y., Li, X., Ren, L., Zhao, J., Hu, Y., et al. (2020). Clinical features of patients infected with 2019 novel coronavirus in Wuhan, China. Lancet 395 (10223), 497–506. doi:10.1016/s0140-6736(20)30183-5
Huber, D., Tegl, G., Baumann, M., Sommer, E., Gorji, E. G., Borth, N., et al. (2017). Chitosan hydrogel formation using laccase activated phenolics as cross-linkers. Carbohydr. Polym. 157, 814–822. doi:10.1016/j.carbpol.2016.10.012
Huerta Lwanga, E., Gertsen, H., Gooren, H., Peters, P., Salanki, T., van der Ploeg, M., et al. (2016). Microplastics in the terrestrial ecosystem: Implications for lumbricus terrestris (Oligochaeta, lumbricidae). Environ. Sci. Technol. 50 (5), 2685–2691. doi:10.1021/acs.est.5b05478
Ilyas, S., Srivastava, R. R., and Kim, H. (2020). Disinfection technology and strategies for COVID-19 hospital and bio-medical waste management. Sci. Total Environ. 749, 141652. doi:10.1016/j.scitotenv.2020.141652
Inghelbrecht, S., and Remon, J. P. (1998). Roller compaction and tableting of microcrystalline cellulose drug mixtures. Int. J. Pharm. 161 (2), 215–224. doi:10.1016/s0378-5173(97)00356-6
Iqbal, S., Xu, J. C., Allen, S. D., Khan, S., Nadir, S., Arif, M. S., et al. (2020). Unraveling consequences of soil micro- and nano-plastic pollution on soil-plant system: Implications for nitrogen (N) cycling and soil microbial activity. Chemosphere 260, 127578. doi:10.1016/j.chemosphere.2020.127578
Jahnke, A., Arp, H. P. H., Escher, B. I., Gewert, B., Gorokhova, E., Kuehnel, D., et al. (2017). Reducing uncertainty and confronting ignorance about the possible impacts of weathering plastic in the marine environment. Environ. Sci. Technol. Lett. 4 (3), 85–90. doi:10.1021/acs.estlett.7b00008
Jambeck, J. R., Geyer, R., Wilcox, C., Siegler, T. R., Perryman, M., Andrady, A., et al. (2015). Plastic waste inputs from land into the ocean. Science 347 (6223), 768–771. doi:10.1126/science.1260352
Jiang, X., Chen, H., Liao, Y., Ye, Z., Li, M., and Klobucar, G. (2019). Ecotoxicity and genotoxicity of polystyrene microplastics on higher plant Vicia faba. Environ. Pollut. 250, 831–838. doi:10.1016/j.envpol.2019.04.055
Jones, O. A. H., Sdepanian, S., Lofts, S., Svendsen, C., Spurgeon, D. J., Maguire, M. L., et al. (2014). Metabolomic analysis of soil communities can be used for pollution assessment. Environ. Toxicol. Chem. 33 (1), 61–64. doi:10.1002/etc.2418
Karami, A., Golieskardi, A., Choo, C. K., Larat, V., Galloway, T. S., and Salamatinia, B. (2017). The presence of microplastics in commercial salts from different countries. Sci. Rep. 7, 46173. doi:10.1038/srep46173
Karimi, R., Akinremi, W., and Flaten, D. (2018). Nitrogen- or phosphorus-based pig manure application rates affect soil test phosphorus and phosphorus loss risk. Nutr. Cycl. Agroecosyst. 111 (2-3), 217–230. doi:10.1007/s10705-018-9924-8
Kasirajan, S., and Ngouajio, M. (2012). Polyethylene and biodegradable mulches for agricultural applications: A review. Agron. Sustain. Dev. 32 (2), 501–529. doi:10.1007/s13593-011-0068-3
Keesstra, S. D., Bouma, J., Wallinga, J., Tittonell, P., Smith, P., Cerda, A., et al. (2016). The significance of soils and soil science towards realization of the United Nations Sustainable Development Goals. Soil 2 (2), 111–128. doi:10.5194/soil-2-111-2016
Kim, L. H., Kang, J., Kayhanian, M., Gil, K. I., Stenstrom, M. K., and Zoh, K. D. (2006). Characteristics of litter waste in highway storm runoff. Water Sci. Technol. 53 (2), 225–234. doi:10.2166/wst.2006.056
Koh, L.-D., Cheng, Y., Teng, C.-P., Khin, Y.-W., Loh, X.-J., Tee, S.-Y., et al. (2015). Structures, mechanical properties and applications of silk fibroin materials. Prog. Polym. Sci. 46, 86–110. doi:10.1016/j.progpolymsci.2015.02.001
Kulkarni, B. N., and Anantharama, V. (2020). Repercussions of COVID-19 pandemic on municipal solid waste management: Challenges and opportunities. Sci. Total Environ. 743, 140693. doi:10.1016/j.scitotenv.2020.140693
Kumar, S., Chiemchaisri, C., and Mudhoo, A. (2011). Bioreactor landfill technology in municipal solid waste treatment: An overview. Crit. Rev. Biotechnol. 31 (1), 77–97. doi:10.3109/07388551.2010.492206
Lagace, L., Charron, C., and Sadiki, M. (2017). Analysis of plastic residues in maple sap and syrup collected from tubing systems sanitized with isopropyl alcohol. Heliyon 3 (5), e00306. doi:10.1016/j.heliyon.2017.e00306
Lebreton, L. C. M., Greer, S. D., and Borrero, J. C. (2012). Numerical modelling of floating debris in the world's oceans. Mar. Pollut. Bull. 64 (3), 653–661. doi:10.1016/j.marpolbul.2011.10.027
Leddin, D., Armstrong, D., Ali, R. A. R., Barkun, A., Butt, A. S., Chen, Y., et al. (2020). Personal protective equipment for endoscopy in low-resource settings during the COVID-19 pandemic guidance from the world gastroenterology organisation. J. Clin. Gastroenterology 54 (10), 833–840. doi:10.1097/mcg.0000000000001411
Li Chen, L., Liu, J.-r., Hu, W.-f., Gao, J., and Yang, J.-y. (2021). Vanadium in soil-plant system: Source, fate, toxicity, and bioremediation. J. Hazard. Mater. 405, 124200. doi:10.1016/j.jhazmat.2020.124200
Li, S., Wang, T., Guo, J., Dong, Y., Wang, Z., Gong, L., et al. (2021). Polystyrene microplastics disturb the redox homeostasis, carbohydrate metabolism and phytohormone regulatory network in barley. J. Hazard. Mater. 415, 125614. doi:10.1016/j.jhazmat.2021.125614
Lin Wang, L., Liu, Y., Kaur, M., Yao, Z. S., Chen, T. Z., and Xu, M. (2021). Phytotoxic effects of polyethylene microplastics on the growth of food crops soybean (Glycine max) and mung bean (vigna radiata). Int. J. Environ. Res. Public Health 18 (20), 10629. doi:10.3390/ijerph182010629
Liu, H., Yang, X., Liu, G., Liang, C., Xue, S., Chen, H., et al. (2017). Response of soil dissolved organic matter to microplastic addition in Chinese loess soil. Chemosphere 185, 907–917. doi:10.1016/j.chemosphere.2017.07.064
Liu, M., Lu, S., Song, Y., Lei, L., Hu, J., Lv, W., et al. (2018). Microplastic and mesoplastic pollution in farmland soils in suburbs of Shanghai, China. Environ. Pollut. 242, 855–862. doi:10.1016/j.envpol.2018.07.051
Liu, Y., Huang, Q., Hu, W., Qin, J. M., Zheng, Y. R., Wang, J. F., et al. (2021). Effects of plastic mulch film residues on soil-microbe-plant systems under different soil pH conditions. Chemosphere 267, 128901. doi:10.1016/j.chemosphere.2020.128901
Liu, K., Liu, Z., Zhou, N., Shi, X., Lock, T. R., Kallenbach, R. L., et al. (2022). Diversity-stability relationships in temperate grasslands as a function of soil pH. Land Degrad. Dev. 33, 1704–1717. doi:10.1002/ldr.4259
Lowery, N. V., and Ursell, T. (2019). Structured environments fundamentally alter dynamics and stability of ecological communities. Proc. Natl. Acad. Sci. U. S. A. 116 (2), 379–388. doi:10.1073/pnas.1811887116
Luo, T., Wang, C., Pan, Z., Jin, C., Fu, Z., and Jin, Y. (2019). Maternal polystyrene microplastic exposure during gestation and lactation altered metabolic homeostasis in the dams and their F1 and F2 offspring. Environ. Sci. Technol. 53 (18), 10978–10992. doi:10.1021/acs.est.9b03191
Lusher, A. L., Burke, A., O'Connor, I., and Officer, R. (2014). Microplastic pollution in the northeast atlantic ocean: Validated and opportunistic sampling. Mar. Pollut. Bull. 88 (1-2), 325–333. doi:10.1016/j.marpolbul.2014.08.023
Lusher, A. L., Welden, N. A., Sobral, P., and Cole, M. (2017). Sampling, isolating and identifying microplastics ingested by fish and invertebrates. Anal. Methods 9 (9), 1346–1360. doi:10.1039/c6ay02415g
Machado, A. A. d. S., Lau, C. W., Till, J., Kloas, W., Lehmann, A., Becker, R., et al. (2018). Impacts of microplastics on the soil biophysical environment. Environ. Sci. Technol. 52 (17), 9656–9665. doi:10.1021/acs.est.8b02212
Machado, A. A. d. S., Lau, C. W., Kloas, W., Bergmann, J., Bacheher, J. B., Faltin, E., et al. (2019). Microplastics can change soil properties and affect plant performance. Environ. Sci. Technol. 53 (10), 6044–6052. doi:10.1021/acs.est.9b01339
Madadian, E., Haelssig, J. B., and Pegg, M. (2020). A comparison of thermal processing strategies for landfill reclamation: Methods, products, and a promising path forward. Resour. Conservation Recycl. 160, 104876. doi:10.1016/j.resconrec.2020.104876
Maity, S., Chatterjee, A., Guchhait, R., De, S., and Pramanick, K. (2020). Cytogenotoxic potential of a hazardous material, polystyrene microparticles on Allium cepa L. J. Hazard. Mater. 385, 121560. doi:10.1016/j.jhazmat.2019.121560
Maskova, T., and Herben, T. (2018). Root:shoot ratio in developing seedlings: How seedlings change their allocation in response to seed mass and ambient nutrient supply. Ecol. Evol. 8 (14), 7143–7150. doi:10.1002/ece3.4238
Mason, S. A., Welch, V. G., and Neratko, J. (2018). Synthetic polymer contamination in bottled water. Front. Chem. 6, 407. doi:10.3389/fchem.2018.00407
Matich, E. K., Soria, N. G. C., Aga, D. S., and Atilla-Gokcumen, G. E. (2019). Applications of metabolomics in assessing ecological effects of emerging contaminants and pollutants on plants. J. Hazard. Mater. 373, 527–535. doi:10.1016/j.jhazmat.2019.02.084
Mattsson, K., Hansson, L. A., and Cedervall, T. (2015). Nano-plastics in the aquatic environment. Environ. Sci. Process. Impacts 17 (10), 1712–1721. doi:10.1039/c5em00227c
McDevitt, J. P., Criddle, C. S., Morse, M., Hale, R. C., Bott, C. B., and Rochman, C. M. (2017). Addressing the issue of microplastics in the wake of the microbead-free waters act-A new standard can facilitate improved policy. Environ. Sci. Technol. 51 (12), 6611–6617. doi:10.1021/acs.est.6b05812
Meng, F., Yang, X., Riksen, M., Xu, M., and Geissen, V. (2021). Response of common bean (Phaseolus vulgaris L.) growth to soil contaminated with microplastics. Sci. Total Environ. 755, 142516. doi:10.1016/j.scitotenv.2020.142516
Munir, M. A. M., Yousaf, B., Ali, M. U., Dan, C., Abbas, Q., Arif, M., et al. (2021). In situ synthesis of micro-plastics embedded sewage-sludge co-pyrolyzed biochar: Implications for the remediation of Cr and Pb availability and enzymatic activities from the contaminated soil. J. Clean. Prod. 302, 127005. doi:10.1016/j.jclepro.2021.127005
Nelms, S. E., Coombes, C., Foster, L. C., Galloway, T. S., Godley, B. J., Lindeque, P. K., et al. (2017). Marine anthropogenic litter on British beaches: A 10-year nationwide assessment using citizen science data. Sci. Total Environ. 579, 1399–1409. doi:10.1016/j.scitotenv.2016.11.137
Niu, L., Zhao, X., Wu, F., Tang, Z., Lv, H., Wang, J., et al. (2021). Hotpots and trends of covalent organic frameworks (COFs) in the environmental and energy field: Bibliometric analysis. Sci. Total Environ. 783, 146838. doi:10.1016/j.scitotenv.2021.146838
Nizzetto, L., Futter, M., and Langaas, S. (2016). Are agricultural soils dumps for microplastics of urban origin? Environ. Sci. Technol. 50 (20), 10777–10779. doi:10.1021/acs.est.6b04140
Ohlwein, S., Kappeler, R., Joss, M. K., Kunzli, N., and Hoffmann, B. (2019). Health effects of ultrafine particles: A systematic literature review update of epidemiological evidence. Int. J. Public Health 64 (4), 547–559. doi:10.1007/s00038-019-01202-7
Orgiles-Calpena, E., Aran-Ais, F., Torro-Palau, A. M., and Orgiles-Barcelo, C. (2014). Biodegradable polyurethane adhesives based on polyols derived from renewable resources. Proc. Institution Mech. Eng. Part L J. Mater. Des. Appl. 228 (2), 125–136. doi:10.1177/1464420713517674
Pang, T., and Shen, J. (2022). Visualizing the landscape and evolution of capacitive deionization by scientometric analysis. Desalination 527, 115562. doi:10.1016/j.desal.2022.115562
Parashar, N., and Hait, S. (2021). Plastics in the time of COVID-19 pandemic: Protector or polluter? Sci. Total Environ. 759, 144274. doi:10.1016/j.scitotenv.2020.144274
Pignattelli, S., Broccoli, A., and Renzi, M. (2020). Physiological responses of garden cress (L. sativum) to different types of microplastics. Sci. Total Environ. 727, 138609. doi:10.1016/j.scitotenv.2020.138609
Pittmann, T., and Steinmetz, H. (2016). Potential for polyhydroxyalkanoate production on German or European municipal waste water treatment plants. Bioresour. Technol. 214, 9–15. doi:10.1016/j.biortech.2016.04.074
Porter, D. W., Hubbs, A. F., Mercer, R. R., Wu, N., Wolfarth, M. G., Sriram, K., et al. (2010). Mouse pulmonary dose- and time course-responses induced by exposure to multi-walled carbon nanotubes. Toxicology 269 (2-3), 136–147. doi:10.1016/j.tox.2009.10.017
Prata, J. C. (2018a). Airborne microplastics: Consequences to human health? Environ. Pollut. 234, 115–126. doi:10.1016/j.envpol.2017.11.043
Prata, J. C. (2018b). Microplastics in wastewater: State of the knowledge on sources, fate and solutions. Mar. Pollut. Bull. 129 (1), 262–265. doi:10.1016/j.marpolbul.2018.02.046
Qi, Y. L., Yang, X. M., Pelaez, A. M., Lwanga, E. H., Beriot, N., Gertsen, H., et al. (2018). Macro- and micro- plastics in soil-plant system: Effects of plastic mulch film residues on wheat (Triticum aestivum) growth. Sci. Total Environ. 645, 1048–1056. doi:10.1016/j.scitotenv.2018.07.229
Ren, X., Tang, J., Wang, L., and Liu, Q. (2021). Microplastics in soil-plant system: Effects of nano/microplastics on plant photosynthesis, rhizosphere microbes and soil properties in soil with different residues. Plant Soil 462 (1-2), 561–576. doi:10.1007/s11104-021-04869-1
Rhim, J.-W., and Ng, P. K. W. (2007). Natural biopolymer-based nanocomposite films for packaging applications. Crit. Rev. Food Sci. Nutr. 47 (4), 411–433. doi:10.1080/10408390600846366
Rillig, M. C., Ingraffia, R., and Machado, A. A. d. S. (2017). Microplastic incorporation into soil in agroecosystems. Front. Plant Sci. 8, 1805. doi:10.3389/fpls.2017.01805
Roman, L., Schuyler, Q., Wilcox, C., and Hardesty, B. D. (2021). Plastic pollution is killing marine megafauna, but how do we prioritize policies to reduce mortality? Conserv. Lett. 14 (2), 12781. doi:10.1111/conl.12781
Sajiki, J., and Yonekubo, J. (2003). Leaching of bisphenol A (BPA) to seawater from polycarbonate plastic and its degradation by reactive oxygen species. Chemosphere 51 (1), 55–62. doi:10.1016/s0045-6535(02)00789-0
Santillo, D., Miller, K., and Johnston, P. (2017). Microplastics as contaminants in commercially important seafood species. Integr. Environ. Assess. Manag. 13 (3), 516–521. doi:10.1002/ieam.1909
Schneider, M., Stracke, F., Hansen, S., and Schaefer, U. F. (2009). Nanoparticles and their interactions with the dermal barrier. Dermato-endocrinology 1 (4), 197–206. doi:10.4161/derm.1.4.9501
Siwek, P., Domagala-Swiatkiewicz, I., Bucki, P., and Puchalski, M. (2019). Biodegradable agroplastics in 21st century horticulture. Polimery 64 (7-8), 480–486. doi:10.14314/polimery.2019.7.2
Smith, M. D., Hartnett, D. C., and Wilson, G. W. T. (1999). Interacting influence of mycorrhizal symbiosis and competition on plant diversity in tallgrass prairie. Oecologia 121 (4), 574–582. doi:10.1007/s004420050964
Smith-Ramesh, L. M., and Reynolds, H. L. (2017). The next frontier of plant-soil feedback research: Unraveling context dependence across biotic and abiotic gradients. J. Veg. Sci. 28 (3), 484–494. doi:10.1111/jvs.12519
Steinmetz, Z., Wollmann, C., Schaefer, M., Buchmann, C., David, J., Troeger, J., et al. (2016). Plastic mulching in agriculture. Trading short-term agronomic benefits for long-term soil degradation? Sci. Total Environ. 550, 690–705. doi:10.1016/j.scitotenv.2016.01.153
Sutherland, W. J., Clout, M., Cote, I. M., Daszak, P., Depledge, M. H., Fellman, L., et al. (2010). A horizon scan of global conservation issues for 2010. Trends Ecol. Evol. 25 (1), 1–7. doi:10.1016/j.tree.2009.10.003
Suyadi, and Manullang, C. Y. (2020). Distribution of plastic debris pollution and it is implications on mangrove vegetation. Mar. Pollut. Bull. 160, 111642. doi:10.1016/j.marpolbul.2020.111642
Tsai, W.-T. (2021). Analysis of plastic waste reduction and recycling in Taiwan. Waste Manag. Res. 39 (5), 713–719. doi:10.1177/0734242x21996821
Van Cauwenberghe, L., and Janssen, C. R. (2014). Microplastics in bivalves cultured for human consumption. Environ. Pollut. 193, 65–70. doi:10.1016/j.envpol.2014.06.010
Velzeboer, I., Kwadijk, C. J. A. F., and Koelmans, A. A. (2014). Strong sorption of PCBs to nanoplastics, microplastics, carbon nanotubes, and fullerenes. Environ. Sci. Technol. 48 (9), 4869–4876. doi:10.1021/es405721v
Vethaak, A. D., and Leslie, H. A. (2016). Plastic debris is a human health issue. Environ. Sci. Technol. 50 (13), 6825–6826. doi:10.1021/acs.est.6b02569
Vroman, I., and Tighzert, L. (2009). Biodegradable polymers. Materials 2 (2), 307–344. doi:10.3390/ma2020307
Wan, Y., Wu, C., Xue, Q., and Hui, X. (2019). Effects of plastic contamination on water evaporation and desiccation cracking in soil. Sci. Total Environ. 654, 576–582. doi:10.1016/j.scitotenv.2018.11.123
Wang, Q., Bai, J., Ning, B., Fan, L., Sun, T., Fang, Y., et al. (2020). Effects of bisphenol A and nanoscale and microscale polystyrene plastic exposure on particle uptake and toxicity in human Caco-2 cells. Chemosphere 254, 126788. doi:10.1016/j.chemosphere.2020.126788
Weithmann, N., Moeller, J. N., Loeder, M. G. J., Piehl, S., Laforsch, C., and Freitag, R. (2018). Organic fertilizer as a vehicle for the entry of microplastic into the environment. Sci. Adv. 4 (4), eaap8060. doi:10.1126/sciadv.aap8060
Wiersinga, W. J., Rhodes, A., Cheng, A. C., Peacock, S. J., and Prescott, H. C. (2020). Pathophysiology, transmission, diagnosis, and treatment of coronavirus disease 2019 (COVID-19) A review. Jama-Journal Am. Med. Assoc. 324 (8), 782–793. doi:10.1001/jama.2020.12839
Wik, A., and Dave, G. (2009). Occurrence and effects of tire wear particles in the environment - a critical review and an initial risk assessment. Environ. Pollut. 157 (1), 1–11. doi:10.1016/j.envpol.2008.09.028
Willen, A., Junestedt, C., Rodhe, L., Pell, M., and Jonsson, H. (2017). Sewage sludge as fertiliser - environmental assessment of storage and land application options. Water Sci. Technol. 75 (5), 1034–1050. doi:10.2166/wst.2016.584
Wright, S. L., Rowe, D., Thompson, R. C., and Galloway, T. S. (2013). Microplastic ingestion decreases energy reserves in marine worms. Curr. Biol. 23 (23), R1031–R1033. doi:10.1016/j.cub.2013.10.068
Wu, J., Liu, W., Zeb, A., Lian, J., Sun, Y., and Sun, H. (2021). Polystyrene microplastic interaction with oryza sativa: Toxicity and metabolic mechanism. Environ. Sci. Nano 8 (12), 3699–3710. doi:10.1039/d1en00636c
Wu, X., Liu, Y., Yin, S., Xiao, K., Xiong, Q., Bian, S., et al. (2020). Metabolomics revealing the response of rice (Oryza sativa L.) exposed to polystyrene microplastics. Environ. Pollut. 266, 115159. doi:10.1016/j.envpol.2020.115159
Wu, Z., and McGoogan, J. M. (2020). Characteristics of and important lessons from the coronavirus disease 2019 (COVID-19) outbreak in China. Jama-Journal Am. Med. Assoc. 323 (13), 1239–1242. doi:10.1001/jama.2020.2648
Xian Chen, X., Cheng, X., Meng, H., Selvaraj, K. K., Li, H., He, H., et al. (2021). Past, present, and future perspectives on the assessment of bioavailability/bioaccessibility of polycyclic aromatic hydrocarbons: A 20-year systemic review based on scientific econometrics. Sci. Total Environ. 774, 145585. doi:10.1016/j.scitotenv.2021.145585
Xinjing Wang, X., Zhang, Y., Zhang, J., Fu, C., and Zhang, X. (2021). Progress in urban metabolism research and hotspot analysis based on CiteSpace analysis. J. Clean. Prod. 281, 125224. doi:10.1016/j.jclepro.2020.125224
Xu, B., Huang, D., Liu, F., Alfaro, D., Lu, Z., Tang, C., et al. (2021). Contrasting effects of microplastics on sorption of diazepam and phenanthrene in soil. J. Hazard. Mater. 406, 124312. doi:10.1016/j.jhazmat.2020.124312
Yan-Chao Li, Y.-C., Bai, W.-Z., and Hashikawa, T. (2020). The neuroinvasive potential of SARS-CoV2 may play a role in the respiratory failure of COVID-19 patients. J. Med. Virol. 92 (6), 552–555. doi:10.1002/jmv.25728
Yang, Y., Yang, J., Wu, W.-M., Zhao, J., Song, Y., Gao, L., et al. (2015). Biodegradation and mineralization of polystyrene by plastic-eating mealworms: Part 1. Chemical and physical characterization and isotopic tests. Environ. Sci. Technol. 49 (20), 12080–12086. doi:10.1021/acs.est.5b02661
Yu, H., Zhang, Y., and Tan, W. (2021). The "neighbor avoidance effect" of microplastics on bacterial and fungal diversity and communities in different soil horizons. Environ. Sci. Ecotechnology 8, 100121. doi:10.1016/j.ese.2021.100121
Zhang, G. S., Hu, X. B., Zhang, X. X., and Li, J. (2015). Effects of plastic mulch and crop rotation on soil physical properties in rain-fed vegetable production in the mid-Yunnan plateau, China. Soil Tillage Res. 145, 111–117. doi:10.1016/j.still.2014.09.010
Zhenxia Li, Z., Li, Q., Li, R., Zhao, Y., Geng, J., and Wang, G. (2020). Physiological responses of lettuce (Lactuca sativa L.) to microplastic pollution. Environ. Sci. Pollut. Res. 27 (24), 30306–30314. doi:10.1007/s11356-020-09349-0
Keywords: microplastics, soil, health, risk, bibliometrics, citespace
Citation: Sun Y, Yang C, Liang H, Zhang S, Zhang R, Dong Y, Tanveer SK and Hai J (2022) Health risk analysis of microplastics in soil in the 21st century: A scientometrics review. Front. Environ. Sci. 10:976237. doi: 10.3389/fenvs.2022.976237
Received: 24 June 2022; Accepted: 08 August 2022;
Published: 26 August 2022.
Edited by:
Mallavarapu Megharaj, The University of Newcastle, AustraliaReviewed by:
Jie Wang, China Agricultural University, ChinaHao Zheng, Ocean University of China, China
Copyright © 2022 Sun, Yang, Liang, Zhang, Zhang, Dong, Tanveer and Hai. This is an open-access article distributed under the terms of the Creative Commons Attribution License (CC BY). The use, distribution or reproduction in other forums is permitted, provided the original author(s) and the copyright owner(s) are credited and that the original publication in this journal is cited, in accordance with accepted academic practice. No use, distribution or reproduction is permitted which does not comply with these terms.
*Correspondence: Jiangbo Hai, aGFpamlhbmdib0AxMjYuY29t