- 1Bauhaus-Institute for Infrastructure Solutions (b.is), Chair of Urban Water Management and Sanitation, Bauhaus-Universität Weimar, Weimar, Germany
- 2Bauhaus-Institute for Infrastructure Solutions (b.is), Chair of Urban Bioengineering for Resource Recovery, Bauhaus-Universität Weimar, Weimar, Germany
This work presents a modular Vertical Green System (VGS) for gray water treatment, developed at the Bauhaus-Universität Weimar. The concept was transformed into a field study with four modules built and tested with synthetic gray water. Each module set contains a small and larger module with the same treatment substrate and was fed hourly. A combination of lightweight structural material and biochar of agricultural residues and wood chips was used as the treatment substrate. In this article, we present the first 18 weeks of operation. Regarding the treatment efficiency, the parameters chemical oxygen demand (COD), total phosphorous (TP), ortho-phosphate (ortho-P), total bound nitrogen (TNb), ammonium nitrogen (NH4-N), and nitrate nitrogen (NO3-N) were analyzed and are presented in this work. The results of the modules with agricultural residues are promising. Up to 92% COD reduction is stated in the data. The phosphate and nitrogen fractions are reduced significantly in these modules. By contrast, the modules with wood chips reduce only 67% of the incoming COD and respectively less regarding phosphates and the nitrogen fraction.
Introduction
Water is vital not only for all living creatures and plants, but it balances in many ways the climate and the urban climate. This is mostly relevant when nature regulates the microclimate in human-built surroundings. Thus, the global climatic changes and densified cities challenge the water balance in urban areas on a micro- and meso-level. Lacking water for a short period of time, these climatic regulations cannot take full responsibility. Additionally, climate change is resulting in longer periods of unbalanced weather conditions for most regions. Water scarcity for longer periods threatens the survival of greenery in the city and unfolds a not-green-influenced climate which amplifies the Urban Heat Island (UHI) effect in multiple ways.
Previously, water-rich areas were subject to increasingly frequent and prolonged periods of drought, which also has a negative impact on urban greenery. Replacing natural precipitation with tap water as irrigation for urban greenery, as is carried out during heatwaves in many Central European cities, is costly and puts additional strain on water sources for human needs. The use of rainwater for the irrigation of a vertical green system (VGS) often does not cover the demand for the entire season. To bridge these precipitation-free phases, large amounts of water must be temporarily stored (Pearlmutter et al., 2021). However, municipal wastewater is available as a constant water stream in any urban settlement and could be readily used for irrigation and evapotranspiration after treatment. A disadvantage is the centralized sewage system, which is predominant in the Western world. Wastewater is collected and treated in gravity pipes at the lowest point and, in order to be reused in cities, would have to have another network of pipes similar to the drinking water network that distributes treated wastewater.
In contrast, decentralized wastewater treatment plants such as constructed wetlands or small-scale SBR treatment plants in houses can provide a reliable source of treated wastewater for irrigation and cooling through evapotranspiration in most parts of a city for multiple uses, without the need to install a centralized distribution system. In addition, wastewater treatment plants known as nature-based solutions (NBS) (Langergraber et al., 2021) can provide various benefits (eco-services) to the city (Pearlmutter et al., 2020;Perez Urrestarazu et al., 2015). VGSs for the treatment of a wastewater substream—gray water—are special in the NBS catalog because they require a minimum of horizontal space and use surfaces that are usually defined by aesthetic reasons rather than services, namely the surface of the building envelope.
Vertical greening in its simplest form as climbers is well-known and widely used all over the world (Pearlmutter et al., 2020). Regarding its technical state as VGS, Stanley White presented the first system as a patent in the 1940s (Hart, 1938). With VGSs becoming popular and widely known through the work of Patrick Blanc in the 1980s, the green covering of building envelopes started to spread as a design aspect and entered the research field for its benefits within the city structure. Regarding wall-based VGSs, two systems were developed for the market. Firstly, the growing substrate is parallel to the building envelope in modular panels or as a continuous area with a supporting structure (wall-based). In these systems, the plants root horizontally in the growing substrate. Secondly, the plants grow in containers in a supporting structure at the building’s envelope and root vertically (pot-based) (Pearlmutter et al., 2021).
Both systems have advantages in different ways for a conventional VGS. The containers for the pot-based system can root in any kind of growth substrate (e.g., mineral material of fine particle size) which works for plants at a higher level. However, in wall-based systems, the growth substrate itself connects the plant roots to the supporting structure vertically. This specification limits the materials to mostly meshed inorganic fibers or textile-supported structures.
For irrigation, most VGSs use tap water or solid-free rainwater and additional liquid fertilizer due to the clogging problems of the irrigation system and the growth substrate. Using gray water as the source for irrigation started with some research pilot projects in the late 1980s (Thomas Peter, 1987).
With regard to irrigation exceeding the plants’ needs, which is the same in gray water treatment, the pot-based system was more promising because it avoided leakages and spilling in the modules. Gray water treatment in a VGS uses the same treatment mechanism as that used in constructed wetlands (Pucher et al., 2022). However, the pot-based system offers more opportunities material-wise for the treatment process with gray water. Material mixes that fit both specifications, for example, trickling or biofilter processes and root substrate advantages, can be used. Therefore, most actual projects researching gray water use or treatment opt for the pot-based system (Gattringer et al., 2016; Masi et al., 2016;Pucher et al., 2020; Pucher et al., 2022).
Mainly, the disadvantages of this approach are problems with clogging of the growth/treatment substrate due to microorganisms growing in between the fine particles, and the low height of the container which limits the effectiveness of the process (Pucher et al., 2022).
Wall-based VGSs for gray water treatment do have the abovementioned disadvantages of spilling, and the meshed material which could form anaerobic wet areas and disrupt the aerobic treatment process and affect the root growth negatively. But they can use lighter fiber materials and, thus, a lighter supporting structure. Wall-based VGSs combined with gray water treatment are mostly found in research work (Galvao et al., 2022) and are yet not represented in the market.
The VertiKKA-module uses a wall-based system with some adjustments to avert the disadvantages of spilling from the front, clogging the growth or treatment substrate, and enlarging the height for better retention time and therefore better treatment efficiency.
Materials and methods
In terms of study design, the modules presented in this article need to be seen in a broader context for a fully functional treatment process. However, additional pre-treatment and optional post-treatment for different reuse options are necessary but may vary depending on the availability of financial resources, space, or specific reuse needs.
System integration
The VertiKKA-module design combines the mechanism of a trickling filter and bio filter and uses gravity-driven water flow without additional energy, despite lifting the inlet to the highest point to the upper module. The full working design for the gray water treatment covers three different sections for treatment: pre-treatment, the main module as the biological stage, and further treatment for distinct purposes.
Additionally, a buffer tank after the pre-treatment equals the incoming gray water during the day and eases the pumping.
Gray water for the inlet into the VertiKKA-module should be free from sand or other inorganic material and other larger solids to avoid clogging. Suspended solids in some quantity fit the purpose of the process of VertiKKA. In simple form, a settlement tank is the method of choice, but any other filter technique can be installed. The treatment after the VertiKKA-module must fit the purpose of the reuse quality standards for the regional or state legislature, but a small settling basin is strongly recommended to avoid fine biochar particles or biofilm leaving the overall system.
VertiKKA-module
The design of the module is defined by some findings of the architectural demands for built city facades and demand for the treatment process. A 1.6 m height for all modules works for the latter, and the length of a multiple of 40 cm (40, 80, 120 cm) fits most of the spaces between two windows in both old and modern facades. The width of the module is 17 cm for the treatment part, and an additional 5 cm for the greening part. Optionally installed photovoltaic panels, which are attached to the front of the module, extend the total width up to 42 cm. The growing green, which is not part of this calculation, can vary extremely, even for the same species.
The substrate for treatment is a combination of lightweight structural material (expanded glass granulate ∅ 8–16 mm) and biochar from agricultural and woodworking residues, respectively, barley husks (BH), and wood chips (WC) as illustrated in (see Figure 1).
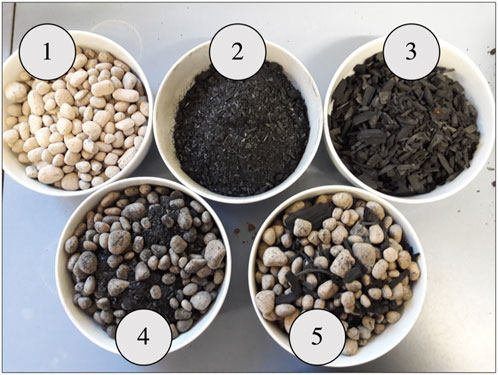
FIGURE 1. Filling substrate for the treatment section: expanded glass granulate (1), biochar made of barley husks (2), biochar made of wood chips (3), the implemented mixture of expanded glass granulate and biochar of barley husks (4), and the implemented mixture of expanded glass granulate and biochar of wood chips (5).
The rooting substrate, which is specially designed for this purpose by a company highly experienced in vertical greening, is attached to the front by panels with the textile which holds one row of plants.
The upper part of the module of approximately 5 cm contains the inner distribution system for the settled gray water. The part on the bottom end of approximately 15 cm carries the treated gray water to the outlet point.
An earlier study at Bauhaus-Univeristät Weimar on this topic found that both parts—the treatment of gray water and plant cultivation—work better when the substrate does not have to serve both purposes. From this finding, the treatment section and the plant section are separated (see Figure 2). The sections are separated with watertight panels, which are the bearing structure for the substrate bags of the greening. Each waterproof panel (20 cm high) is placed at a slight angle and overlaps the top panel by a few cm. The gray water is introduced only in the rear part and trickles through the treatment body, where the biofilm biologically treats the water, and the structural material or biochar absorbs some components.
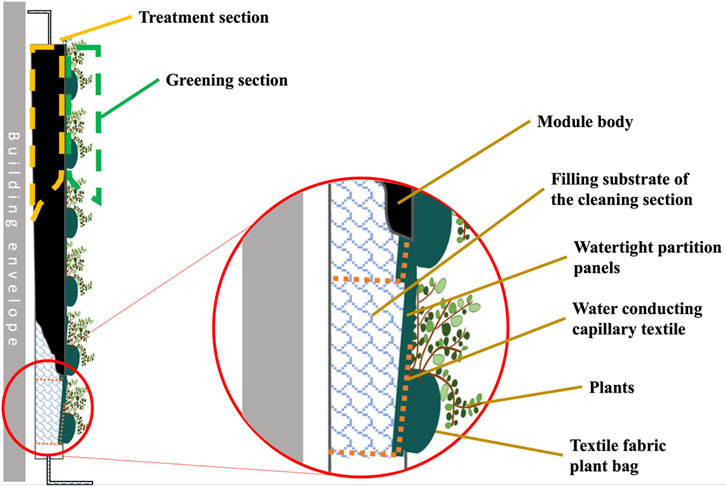
FIGURE 2. The VertiKKA concept with the separate utilization units (treatment and plant section) and the water-bearing connection between these two.
A water-conduction capillary textile, which is attached to the back of the treatment section and lies between the treatment substrate, directs water to the front of the watertight panels. Recesses in the textile ensure that the gray water can always run through the module unhindered. Due to the capillary effect, only the volume needed for the plant requirements, evapotranspiration, or evaporation is transported to the greening section. The unused gray water volume by the plant section is treated by the treatment section and can be used for other purposes.
Plant growth, the precise selection of plant species, the compatibility of gray water as irrigation water, and the nutrients available in gray water, as well as the effect of photovoltaic panels on heat transfer through the module and plant growth, were investigated during the field trial. However, the presentation of these results is beyond the format of this article and could not be presented. These results will be published in separate works.
The modules tested in the field trial are the small (S) and the medium (L) lengths, 40 and 80 cm, respectively, with two modules of each length. A peristaltic pump with adjustable speed was used to feed the modules for 5 min in a constant manner hourly from the same source.
The amount of gray water each hour is (S) 1.75 L and (L) 3.5 L, which is (S) 42 L/d and (L) 84 L/d. After trickling through the filter, the treated gray water was discharged into the local sewer system.
The field trial started in June 2021 at the facilities of the Bauhaus-Universität Weimar at the local treatment plant (see Figure 3). The modules were placed at the walls of the laboratory container at the site. The fed gray water was synthetic based on a formula designed at the Bauhaus-Universität Weimar (Giese and Londong, 2015). After mixing the synthetic gray water in 600 or 800 L batches, it was stored without additional cooling, and constantly stirred and pumped into the module intermediate bulk container (IBC). The formula, which contains no larger particles, for example, hair or textile fibers, is like settled gray water particle- and load-wise.
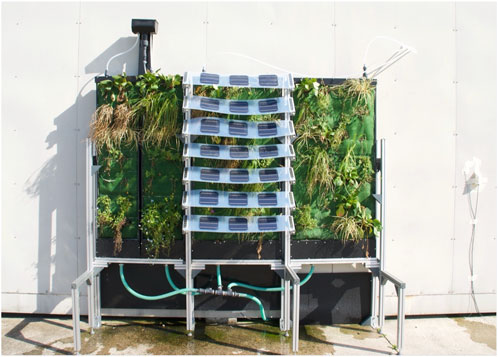
FIGURE 3. The field trial setup with four VertiKKA-modules shortly after installation. On the left, the compartment with HB (S) and WC (S); the compartment in the middle with BH (L) and attached photovoltaic; and on the right, the compartment with WC (L).
Analysis
Each week a correspondent sample of the influent (one sample of synthetic gray water in the IBC) and the outlet of each module were taken. Analyzing the samples at the laboratories of the Bauhaus-Univeristät Weimar took place within 24 h. The parameters presented in this work are chemical oxygen demand (COD), total phosphorous (TP), ortho-phosphate (ortho-P), total bound nitrogen (TNb), ammonium nitrogen (NH4-N), and nitrate nitrogen (NO3-N). The analytics were conducted with Hach LCK cell tests and a DR3900 photometer (Hach). Filtering of the samples was conducted with 0.1 µm filters.
Results
In the following, the modules will be identified by the biochar material and the specification of size. Barley husks (BH) and wood chips (WC) and additionally the specification small (S) or large (L) identify the modules, for example, BH (L) for the large module filled with expanded granulate and barley husks. The efficiency of the process is determined by the equation: (Inflow − Outflow)/Inflow. Detailed results and evaluations of all modules and parameters concerning the trial period presented in this article are in the Supplementary Material. If the concentration in the sample is below the threshold value of the cuvette test, the threshold value of the cuvette test is used as the measured value and not the possible absence of the substance of this parameter. This prevents a supposed better cleaning efficiency by the calculation methods in the average and median values, indicating the minimum efficiency of the cleaning performance of the modules. A slightly higher cleaning efficiency is possible and probable, but not provable. Samples that could not be collected or analyzed for any reason are not included in the calculation. In total, there were 23 sampling rounds in this field test with a duration of 20 weeks.
Chemical oxygen demand
Regarding the reduction of COD in the outlet, the modules show close similarities material-wise. The inlet was highly loaded with COD, represented in a median value of 928 mg⋅O2/l over the presented period. Nonetheless, all modules showed a reduction in COD from the first day.
In total, the reduction resulted in 67% for WC (S) and 65% WC (L), respectively, and 92% for BH (S) and 90% for BH (L). With regard to the COD values for the effluent in Figure 4, only the module filled with barley husks could treat the synthetic gray water to meet the legal requirements consistently.
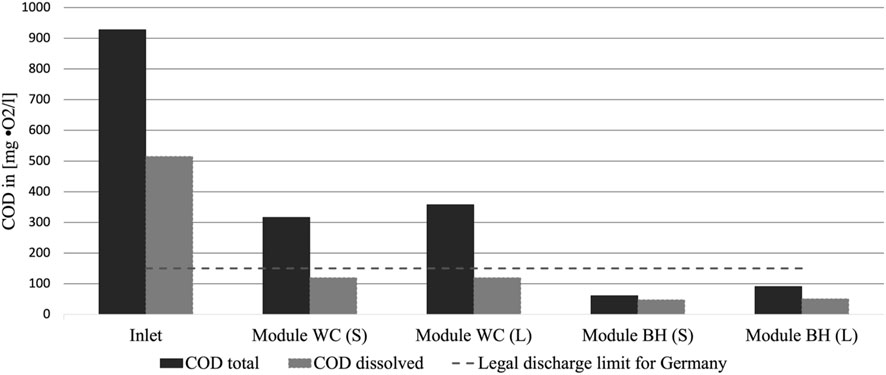
FIGURE 4. Median COD values for inlet and outlets. The legal discharge limit refers to the legal limit for discharge for small-scale treatment plants in Germany.
These results are also reflected in the dissolved fraction of COD—see Supplementary Material. While the solids fraction of the influent sample was 42% for the median, solids accounted for 55% (S) and 60% (L) for the WC material, and 20% (S) and 40% (L) for the BH material. For the latter, the values that form the basis for the calculation were generally correspondingly low.
A reduction clearly related to biodegradation can hardly be observed. A continuously increasing degradation up to the plateau phase, which typically characterizes biologically operated treatment processes in the initial phase, is not evident in the data.
Phosphorus
The results for total phosphorus (TP) show a 34% reduction for the WC (S) module and 28% for WC (L) as the median. For the BH (S) and (L) modules, it is 52% and 35%, respectively.
In the first weeks of the experiment, the BH (L) module showed a higher value than the input (see Supplementary Material), which could be explained by the leaching of fines from the biochar. However, the second module with barley husks (BH (S)) shows a different result, with higher concentrations than the modules with biochar from wood chips in the effluent, but not higher than those in the influent.
After the first 4 weeks, the biochar made from barley husks reached a constant level of 50–60% reduction rate.
Results for orthophosphates in the synthetic gray water are low for unknown reasons (see Supplementary Material). While the target concentration for TP was almost achieved, the target concentration of orthophosphates should be about 2.1 mg/L just by the KH2PO4 added to the synthetic gray water. The most likely explanation is the rapid reaction of the orthophosphate with other components of the synthetic gray water formulation, but this assumption requires further investigation.
Dissolution of the orthophosphate while running through the modules was partially reflected in the results for effluent from all modules during the first weeks of operation. The dissolution of bound phosphate was remarkably high, almost to the target concentration.
Nitrogen fraction
As for the nitrogen fraction, total fixed nitrogen, ammonium, and nitrate are presented as TNb, NH4-N, and NO3-N in this article.
TNb decreased between 50 and 59% in all modules. The reduction is most likely due to the retention of solid particles, as shown by the COD results. The highest reduction is 79%. In the last period of the considered experimental phase, the release of higher amounts of TNb (and also TP and ortho-phosphate) is clearly visible in the data (see Supplementary Material).
For nitrification, the NH4-N parameter and the NO3-N in the effluent were presented. The intermediate NO2-N was also considered, but the values never exceeded 0.45 mg/L and were below the detection limit for most samples.
Ammonium shows constant retention or biological transformation of 80% and 73% for WC (S) and WC (L). The modules BH (S) and BH (L) converted the ammonia concentration to the level of 90% and 93% as the median.
As for biological conversion to nitrate, BH (S) and BH (L) modules were observed about 3 weeks after the start of the experiment (see Supplementary Material). After the initial phase, 52% BH (S) and 27% BH (L) as the median of the incoming ammonium were converted to nitrate. The modules WC (S) and WC (L) showed no significant conversion of incoming ammonium.
Discussion
The field test has shown that the VertiKKA system, with the right choice of treatment substrate, can treat gray water to a level that easily meets the legal requirements in Germany. However, the efficiency is mainly influenced by the particle size of the biochar. If the particle size is too large, as is the case here with pyrolyzed wood chips, the gray water passes through the modules too quickly, and the microbiological community does not have the time to absorb and oxidize the contaminants from the gray water. Also, the particle surface area is much smaller for the large particle sizes than for the small particle sizes. Therefore, the adsorption capacity is much lower than that of the fine material of pyrolyzed barley husks.
In general, the purification performance in this study is defined only on the (inflow − outflow)/inflow relationship. However, three or four different processes took place in the module:
The filtering effect of the cleaning substrate. It can be shown in the collected data that the ratio of the proportions of solids in the gray water, or purified gray water, decreased significantly with a smaller particle size. Therefore, retention of the fine suspended solids within the BH (S) and BH (L) modules can be concluded. This retention is further supported by the second process, adsorption capacity.
During the first week of the field test, no pronounced microbiological activity was expected. In this phase, the contaminants were retained only by the filtering effect and the adsorption capacity. Therefore, it can be concluded from the reduced dissolved COD in the WC (S) and WC (L) modules that the cleaning substrate retained a proportion of the substances by adsorption. These were then available again to the growing biofilm.
The biological activity of a biofilm can be concluded by the conversion of the ammonium to nitrate in the BH (S) and BH (L) modules. The formation of a biofilm that also respires carbon is assumed but cannot be proven from the values alone. The proven carbon reduction could theoretically have been achieved only by the mechanical filter and adsorption mechanisms. However, this is not very likely.
The fourth purification mechanism—the intake of nutrients by plants—refers only to the nutrients contained in the gray water. These were carried with the gray water through the capillary flow to the root substrate, where they were taken up by the roots of the plants and used for the cellular creation of new plant parts.
All three provide a reduction of COD and additionally the latter to the reduction of relevant nutrients in the modules; however, the proportion to which each mechanism was responsible for the reduction cannot be stated with conclusive certainty at this time.
Several events become apparent in the analyzed values (see Supplementary Material) that reveal a need for further research. First, the concentration found for TP and orthophosphate in some samples was higher in the effluent than in the influent. This can only be explained to a limited extent by prior adsorption and then occurring desorption. Also, the amount of orthophosphate found in the influent was too low. The recipe for synthetic gray water was aimed at a higher concentration, to achieve which KH2PO4 is added. However, the target concentration was not achieved. Reactions with other added substances could have led to the binding of orthophosphate. However, this would need to be investigated further.
Two unintentional interruptions of the influent (pump failure 09/14/2021 and emptying of the receiver tank 09/28/2021) for more than 24 h resulted in an increase of the measured COD, TNb, and TP in the respective effluents. This may have been caused by drying and detachment of the formed biofilm. This is also supported by the increased solids content visible to the naked eye.
Another finding from the field test is the washing out of fine biochar, which was repeatedly found in the effluent samples. This behavior was expected for the initial phase since, despite screening and washing of the biochar, fines still adhered to the material. However, this should theoretically have subsided after a few weeks, due to the growth of biomass and the washing out of these residual components. The biochar can thus be included in the COD measurements as inorganic carbon without having any real relevance in biological oxygen consumption. In addition, the orthophosphate potentially adsorbed on the biochar, which has a relevant biological environmental effect when desorbed, could also be found in the effluent values. This requires further investigation.
Regarding the treatment process, the general design works. Adjustments for the feeding volume, resulting in more pumping or more module surface, still need to be considered. But the overall results for plants and heat reduction, which will be presented separately, are very promising.
Data availability statement
The original contributions presented in the study are included in the article/Supplementary Material. Further inquiries can be directed to the corresponding author.
Author contributions
AA developed the presented concept. AA and MB carried out the experiment. AA wrote the manuscript with support from MB. SB and JL supervised the project.
Acknowledgments
We would like to thank the Federal Ministry of Education and Research of Germany (BMBF, funding code 033W108G), which supported this research.
Conflict of interest
The authors declare that the research was conducted in the absence of any commercial or financial relationships that could be construed as a potential conflict of interest.
Publisher’s note
All claims expressed in this article are solely those of the authors and do not necessarily represent those of their affiliated organizations, or those of the publisher, the editors, and the reviewers. Any product that may be evaluated in this article, or claim that may be made by its manufacturer, is not guaranteed or endorsed by the publisher.
Supplementary material
The Supplementary Material for this article can be found online at: https://www.frontiersin.org/articles/10.3389/fenvs.2022.976005/full#supplementary-material
References
Galvão, A., Martins, D., Rodrigues, A., Manso, M., Ferreira, J., and Silva, C. (2022). Green walls with recycled filling media to treat greywater. Sci. Total Environ. 23, 156748. doi:10.1016/j.scitotenv.2022.156748
Gattringer, H., Claret, A., Radtke, M., Kisser, J., Sarashgi, A., Rodríguez-Roda, I., et al. (2016). Novel vertical ecosystem for sustainable water treatment and reuse in tourist resorts. Int. J. SDP. 11, 263–274. doi:10.2495/SDP-V11-N3-263-274
Giese, T., and Londong, J. (2015). “Kopplung von regenerativer Energiegewinnung mit innovativer Stadtentwässerung. Synthesebericht zum Forschungsprojekt KREIS (Connecting regenerative energy production with new alternativew sanitation systems),” in Synthesis report on the KREIS research project) Schriftenreihe des Bauhaus-Instituts für zukunftsweisende Infrastruktursysteme (Berlin: RHOMBOS-Verlag).
Langergraber, G., Castellar, J., Pucher, B., Baganz, G., Milosevic, D., Andreucci, M., et al. (2021). A framework for addressing circularity challenges in cities with nature-based solutions. Water 13, 2355. doi:10.3390/w13172355
Masi, F., Bresciani, R., Rizzo, A., Edathoot, A., Patwardhan, N., Panse, D., et al. (2016). Green walls for greywater treatment and recycling in dense urban areas: A case-study in pune. J. Water Sanit. Hyg. Dev. 6, 342–347. doi:10.2166/washdev.2016.019
Pearlmutter, D., Pucher, B., Calheiros, C., Hoffmann, K., Aicher, A., Pinho, P., et al. (2021). Closing water cycles in the built environment through nature-based solutions: The contribution of vertical greening systems and green roofs. Water 13 (16), 2165. doi:10.3390/w13162165
Pearlmutter, D., Theochari, D., Nehls, T., Pinho, P., Piro, P., Korolova, A., et al. (2020). Enhancing the circular economy with nature-based solutions in the built urban environment: Green building materials, systems and sites. Blue-Green Syst. 2 (1), 46–72. doi:10.2166/bgs.2019.928
Perez Urrestarazu, L., Fernández-Cañero, R., Franco-Salas, A., and Egea, G. (2015). Vertical greening systems and sustainable cities. J. Urban Technol. 22, 65–85. doi:10.1080/10630732.2015.1073900
Pucher, B., Zluwa, I., Spörl, P., Pitha, U., and Langergraber, G. (2022). Evaluation of the multifunctionality of a vertical greening system using different irrigation strategies on cooling, plant development and greywater use. Sci. Total Environ. 849, 157842. doi:10.1016/j.scitotenv.2022.157842
Pucher, B., Riberio, G., Langergraber, G., Zluwa, I., Spörl, P., and Pitha, U. (2020). Entwicklung eines multifunktionalen Living-Wall-Systems zur Reinigung und Nutzung von Grauwasser. Wasser Abfall 22, 37–40. doi:10.1007/s35152-020-0241-6
Keywords: vertical green system (VGS), gray water treatment, urban heat island effect, living wall system, nature-based solution system, blue-green infrastructure
Citation: Aicher A, Boermel M, Londong J and Beier S (2022) Vertical green system for gray water treatment: Analysis of the VertiKKA-module in a field test. Front. Environ. Sci. 10:976005. doi: 10.3389/fenvs.2022.976005
Received: 22 June 2022; Accepted: 27 October 2022;
Published: 30 November 2022.
Edited by:
Björn Vinnerås, Swedish University of Agricultural Sciences, SwedenReviewed by:
Zhengyi Hu, University of Chinese Academy of Sciences, ChinaRadin Maya Saphira, Universiti Tun Hussein Onn Malaysia, Malaysia
Copyright © 2022 Aicher, Boermel, Londong and Beier. This is an open-access article distributed under the terms of the Creative Commons Attribution License (CC BY). The use, distribution or reproduction in other forums is permitted, provided the original author(s) and the copyright owner(s) are credited and that the original publication in this journal is cited, in accordance with accepted academic practice. No use, distribution or reproduction is permitted which does not comply with these terms.
*Correspondence: Andreas Aicher, andreas.aicher@uni-weimar.de