- 1College of Desert Control Science and Engineering, Inner Mongolia Agricultural University, Hohhot, China
- 2Inner Mongolia Academy of Agriculture and Animal Husbandry Sciences, Hohhot, China
- 3Inner Mongolia Autonomous Region Meteorological Bureau, Hohhot, China
Boundaries may have important effects on landscape patterns, landscape change mechanisms, and dynamic processes. However, little is known about the dynamic mechanism of patch boundary changes at a fine scale. To elucidate the characteristics of grassland patches at fine scales and to provide a reference for the mechanism of change and development direction of patchy landscapes. In this paper, the patch of different grassland types in Xilingol League was studied by NMDS, RDA, and SEM methods, to analyze the vegetation community and soil characteristics of surface soil and the relationship between them: The changes in soil vegetation community and soil characteristics were completely different among the three grassland types, and the abrupt changes of vegetation index and soil properties were different. Vegetation index mostly ranged from −1 m to 0 m, and soil index mostly ranged from −0.5 m to 1 m. Fine-scale vegetation and soil boundaries are well defined, vegetation boundaries are mostly between −1 and 0 m and soil boundaries are mostly between −0.5–1 m, and soil properties have a clear influence on plant characteristics. The difference in organic matter, nitrogen, and phosphorus content is an important factor affecting the change of patch boundary, the distribution of the RDA results showed that the organic matter, nitrogen and phosphorus contents in all three grasslands explained >70% of the environmental factors. The emergence of annual vegetation involves a process of succession, specifically, the nature of the underlying soil determines the type of plants at the boundary. The dynamic characteristics of the soil-plant mutual-feed mechanism determine the location and variation of patch boundaries to adapt to disturbance states. The results of this study provide insight into how boundaries respond to changes in environmental conditions and drive dynamic changes at the landscape level.
Introduction
In arid and semi-arid regions, the patchy distribution pattern of grassland is the self-regulation of plant adaptation to water conditions (Li and Zhang, 2018). In patches of grassland, there will be more or less obvious boundaries between plant patches, and more obvious boundaries between plant patches and bare patches, the properties at the boundary are different from the properties inside the patch on either side, and this is called the “edge effect” (Liding et al., 2004; Burst et al., 2020). As an important ecological process, the “edge effect” has an important relationship with species diversity, ecological change process, community evolution, and ecological restoration. Studies on edge effect are also divided into large scale, medium scale, and micro-scale. In previous studies, the large-scale scale was at the global scale, in which vegetation was divided based on climate (Ni, 2001), and vegetation corresponding to each climate zone showed the zonal distribution. The mesoscale edge effect usually refers to the interlacing zone of two ecosystems (Ting and Shaolin, 2008), which is usually the region with the most abundant biodiversity, and the mesoscale edge effect is also the region with the most studies at present. There have been extensive studies on forest grassland and grass-thicket edge effects (Bai et al., 2015; Cao et al., 2019). It was found that species diversity usually increases in areas where different ecosystems intersect, while other indicators fall between the two ecosystems (Erdős et al., 2019). Marginal intersections are considered as local biodiversity research hotspots (Bátori et al., 2018). Raphael K’s (Didham and Lawton, 1999) study even proposed that the edge structure of patches inside the forest would determine the scope and degree of edge effect and also affect the microclimate in this region. Other research shows that the scholars’ conclusions are the same edge effect, therefore, the edge effect more focused on different community studies on the mesoscale, and the study of different ecological edge effect, there are few studies on patch boundaries of the same site conditions and environmental characteristics, for example, in the grass, in the same ecological system is less. By studying more detailed data on soils and vegetation in different patches, it is possible to reveal their soil properties and vegetation patterns and the relationship between them, as well as to have a clearer role in the influence of fine-scale changes in edge effects on larger-scale processes of landscape dynamics.
Changes in vegetation and soil are usually consistent and interact at different patch boundaries (Zhu et al., 2012). In general, the soil has a great influence on the growth of plants, and in the same way, the growth of plants affects the properties of the soil (Liu et al., 2003; Zhongming, et al., 2010; Chandra, et al., 2016). Therefore, the formation and dynamic change of vegetation patterns are limited by soil properties, and in the same way, the dynamic feedback of vegetation also causes the change in soil properties (Fenu et al., 2013; Dovčiak and Brown, 2014). The study by Mason (Mason et al., 2016) et al. showed that in the transition from forest to grassland in the Midwest of the United States, soil characteristics suitable for grassland growth are found under the forest deciduous layer, while in the transition from grassland to forest, the soil parent layer also retains soil characteristics that should be forest, indicating that the succession of vegetation is influenced by soil properties. Laura M’s (Ladwig et al., 2021) study also showed that in the interspersed areas of grassland and scrub, soil properties influenced the fungal populations in the growing areas of the plant communities and that the fungal populations determined the growth condition as well as the species of the vegetation. His research also showed that in the grassland-shrub crisscross area, the flora of the shrub growth area included not only the flora of the grassland but also the flora of the grassland. Shrub species are more abundant than grassland species while retaining some of them.
Others have also argued that changes in communities and plant species are sometimes more influenced by abiotic factors, such as sudden changes in the landscape within a region (Yuan et al., 2019), or changes in moisture (Kim et al., 2017), all of them can affect the change of vegetation pattern and even the change of edge effect. In addition, the interaction between biological factors can also influence the formation and expansion of boundaries, and, in some cases, the intensity and frequency of human disturbance (grazing or fire) (Teague et al., 2011; Veldman and Putz, 2011), It can also affect the composition of plant community inside the boundary and thus affect the edge effect. Therefore, based on the summary of previous studies, it can be concluded that in the study of boundary effects at various scales, the large scale determinants are often determined by regional climate, topography, or regional hydrological conditions (Parks and Mulligan, 2010). However, the causes of mesoscale boundaries are more complex, and in addition to the interaction of soils, topography, and growing vegetation, they may also be caused by human interference (Botha et al., 2020). There are many factors involved, so most studies are not sure how much factors affect the relationship between soil and vegetation (Müller et al., 2012). Under the same topographic and water conditions, the relationship between soil and vegetation can be revealed by investigating their coupling characteristics and their relationship with the edge effect. All the above studies indicate that it is necessary to study edge effects at a finer scale. Within a defined area, changes in boundary effects can lead to changes in many other properties within this area, for example, Y.H. Cao et al. (Cao et al., 2019) scholars have studied annual and perennial patches within saline lands in the Northeast. The results showed that the changes in species richness and coverage of vegetation patches were obviously influenced by soil changes, but the changes were not consistent with all soil changes. Soil properties limit the formation and change of plant patterns. The authors explain that this is because the process of plant succession in the study area superimposed soil conditions, resulting in different patterns in the staggering area. Therefore, it is important to study the edge effects of different types of grassland patches under fine-scale conditions to understand the relationship between soil and vegetation, and even its change direction, and to clarify the vegetation succession direction of this area in advance. The Xilinguole League grasslands have a more comprehensive range of grassland types in China, and it is also an important place for the implementation of ecological and environmental projects, so the study area was set in Xilinguole League.
The grassland in Xilingol League is one of the four grasslands in the north of Inner Mongolia. From the northeast to southwest of Xilingol League, the grassland type also changes from meadow grassland to typical steppe to desert steppe (Guo, 2016). In recent decades, due to human grazing and unrestrained mowing, patch vegetation of different sizes has appeared in different grassland types in Xilingol League, and the grassland landscape is seriously fragmented, and the edge effect is also very obvious in visually abrupt boundaries. These patches of vegetation provide a good environment for the study of micro-scale edge effects, all biotic and abiotic factors, and the small dynamics of their relationship with the environment are highly relevant, this relationship can greatly affect the vegetated landscape in the study area, resulting in a landscape pattern that now varies in patch size. (Ferro and Morrone, 2014; Oliveras and Malhi, 2016; Cao et al., 2019). And this association will have a great impact on the vegetation landscape in the study area, resulting in the formation of the current patch size of the landscape pattern. The dynamic change of landscape pattern is mainly due to the change of patch and its boundary, and will also affect the vegetation coverage in this region.
This study will take typical perennial-bare patch boundaries in different grassland types in Xilingol League as the research object, mainly using plant community indexes and related soil indexes, to reveal the following issues:1. The coupling relationship between vegetation and soil was studied by quantifying the fine-scale of patch boundary; 2. Studied the mechanism of boundary location determination and change; 3. By its direction of change, the differences in soil and plant communities at the boundaries of different grassland types are clarified. To provide more practical suggestions for improving the grassland ecological environment.
Methods
Study area and patch characteristics
Samples were collected from the typical steppe, desert steppe, and typical steppe - desert steppe transition zone in Xilingol League. The sampling area of the typical steppe is located in East Uzhumqin Banner, the sampling area of the desert steppe is located in Suniteyou Banner, and the sampling area of the typical steppe and desert steppe transition zone is mainly located in Abaga Banner near Sunitezuo Banner. XilinGol League is located 115°13′-117°06′E, 43°02′-44°52′N, The climate is temperate continental, with cold and dry winters and an average annual temperature of 0–3°C, average annual precipitation 380–135 mm, decreasing from southeast to northwest. Precipitation is mostly concentrated in July, August, and September, with high annual evapotranspiration, the average is between 1500 and 2700 mm, about 7–10 times the annual precipitation. The main soil types in the study area are chernozem, chestnut, and calcareous brown soil (Lv, 2010; Bao, 2018).
Sampling was conducted at the junction of bare ground and vegetated scrub where there was a clear. The main vegetation type of the plot is the perennial herbaceous community patch, and the patch area is about dozens of square meters. Vegetation patches are in a stable stage.
Sampling design and measurement methods
In late August 2021, soil and plant sampling was conducted simultaneously at three sample plots, and no precipitation events occurred before or during sampling. A total of nine representative patches were randomly selected in each type of grassland. Patches are about 2 m in diameter, vegetation patches, and bare ground patches are included, and the shrub patches included Gramineae, annual herbs, and perennial herbs, with regular shapes, the vegetation patches are all herbaceous perennials in the interior and annuals at the boundaries, indicating that they were gradually formed. The grasslands in the sampling area were all composed of staggered perennial patches and bare patches, and the landscapes presented were also staggered distribution of bare and perennial. The patches were all about 2 m in diameter, and generally there were 1-2 shrubs. A sample strip of about 4 m perpendicular to the boundary was set up for each patch, including both bare ground and vegetation patches. Each sample strip is composed of 13–15 adjacent rectangular samples, each rectangular sample is 50 × 50 cm in size. Among them, 7-9 quadrats were located in the community patch, 5-7 quadrats were located in the bare patch, and the middle quadrat was located at the boundary between the bare patch and vegetation patch (Figure 1E). For recording and subsequent convenience, the transect was defined as an axis, and the midpoint of the middle quadrat was 0, the bare patch was negative, the community patch was positive, and the midpoint of the bare patch adjacent to the middle quadrat was defined as -1, the community patch adjacent to the middle patch was defined as 1, and so on. In several sets of replicated sample strips, samples at the same location are independent replicates, and each indicator is averaged using the sampled sample strips.
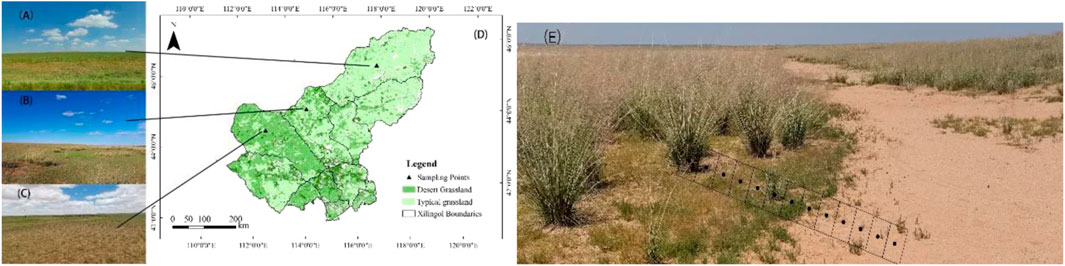
FIGURE 1. Sampling points and field sampling photos of three different types of grasslands: typical grassland (A), transition zone (B) and desert grassland (C). Sampling scene photos, rectangles indicate the 13–15 plant sampling locations along the sampling zone, these points illustrate the locations sampled in each sample square, sample location relative to the boundary is indicated by positive sample distances within perennial patches and negative sample distances outside bare patches (E). Study area map (D).
All plant species within each quadrat were identified, all plant species within each quadrat were identified, their coverage, number, height were recorded and then averaged as species richness at the plot level, above-ground biomass was measured by clipping the above-ground portion, and soil samples were collected from the 0–20 cm soil layer in the center of each sample plot. When sampling, the vegetation in the sample was first identified and counted for individual plant species. After that, all the plants in the quadrat were collected in the aboveground part and brought back to the laboratory for weighing to obtain fresh weight, then bake in the oven at 70°C to constant weight and continue weighing to obtain dry weight. Soil samples were taken by soil auger at a depth of <10 cm and stored in plastic bags to be taken back to the laboratory, and remove stones and some plant roots were by hand before analysis. The soil samples were dried naturally, and Soil organic matter and soil physical and chemical properties were measured by a 2 mm soil sieve.
The data analysis
In this study, the Shannon-Wiener index, species richness index, evenness, and biomass were used to describe vegetation community characteristics. Shannon-Wiener index can be calculated by:
Where Pi is the proportion of the number of individuals of species i to the total number of individuals of all species in the community. Species richness refers to the number of species in a community and is represented by S. The calculation formula of the Pielou index is:
where H is the Shannon-Wiener index and is the species richness.
Determination of soil organic matter by potassium dichromate oxidation - external heating method, determination of soil fast-acting nitrogen by alkaline diffusion method, determination of soil available phosphorus by 0.5 M sodium bicarbonate method, determination of soil available potassium by NH4OAc leaching, flame photometer, determination of total nitrogen by H2SO4-H2O2 elimination cooking method, determination of total phosphorus by vanadium-molybdenum yellow absorbance spectrophotometry, determination of total potassium by flame photometry.
According to previous studies, the number of species will be affected by the factors of vegetation community distribution pattern, and there is a certain correlation between the factors of the distribution pattern. In this study, three methods: Non-metric multidimensional scaling (NMDS)、Canonical Correlation Analysis (RDA)、Structural Equation Modeling (SEM), were used to study the correlation between plaque distribution patterns and their influencing factors.
RDA (Chen et al., 2021)is the combination of correspondence analysis and multiple regression analysis. The important values of the factors affecting vegetation community patterns were sorted to find out the most important factors. . The main purpose of NMDS is to identify and explain the distribution pattern of each factor of the quadrat, reflect the sequential relationship between them, and find gradient information that demonstrates the sources of variation (Li et al., 2019). The RDA and NMDS were conducted with the canoco 5 software (Microcomputer Power, United States). SEM is the simplification of multiple study data of multiple vegetation and soil multiple indicators of the sampled area communities into a low-dimensional space for analysis and categorization. But at the same time, the original relationship between the indexes is retained, which can reflect the relationship between community and soil indexes in the patch distribution. The SEMs were conducted with the Amos 22.0 software package (SmallWaters Corporation, United States). All of drawing was completed with GraphPad Prism 8 (GraphPad Software, United States).
Therefore, the present study is based on the situation that there is no need to study the dynamic change characteristics of vegetation, RDA/NMDS/SEM were used to identify the differences between the two patch boundaries, and the change of the patch boundary between vegetation and bare land was analyzed by combining the growth gradient and distance of flora. At the same time, the potential correlations among the factors are then analyzed in conjunction with the individual factors of change to understand the micro-scale characteristics of the boundary effects of different grassland types of patches.
Result
Spatial pattern of plant variables
This study investigated the community characteristics of plants, including richness (Figure 2A), Shannon–Wiener index, (Figure 2B), plant above-ground biomass (Figure 2C), and Pielou index (Figure 2D). As can be seen from the figure, species richness and plant Shannon–Wiener index in the desert steppe, typical steppe, and transition zone are the largest at the junction of the two types of patches, All the changes were different from the Pielou index and biomass, but the evenness was the largest at the patch edge boundary of the typical steppe. Within 0.5–3.5 m from the edge of the patch, the Pielou index decreases with the increase of the distance from the edge of the patch (Figure 2D1). The Pielou index of vegetation patches in desert steppe and transition zone increased. Within 0.5–3.5 m from the edge of the patch, the Pielou index of the transition zone between the two grasslands was continuously increasing (Figure 2D2). However, the increasing trend of desert grasslands is becoming more and more stable (Figure 2D3).
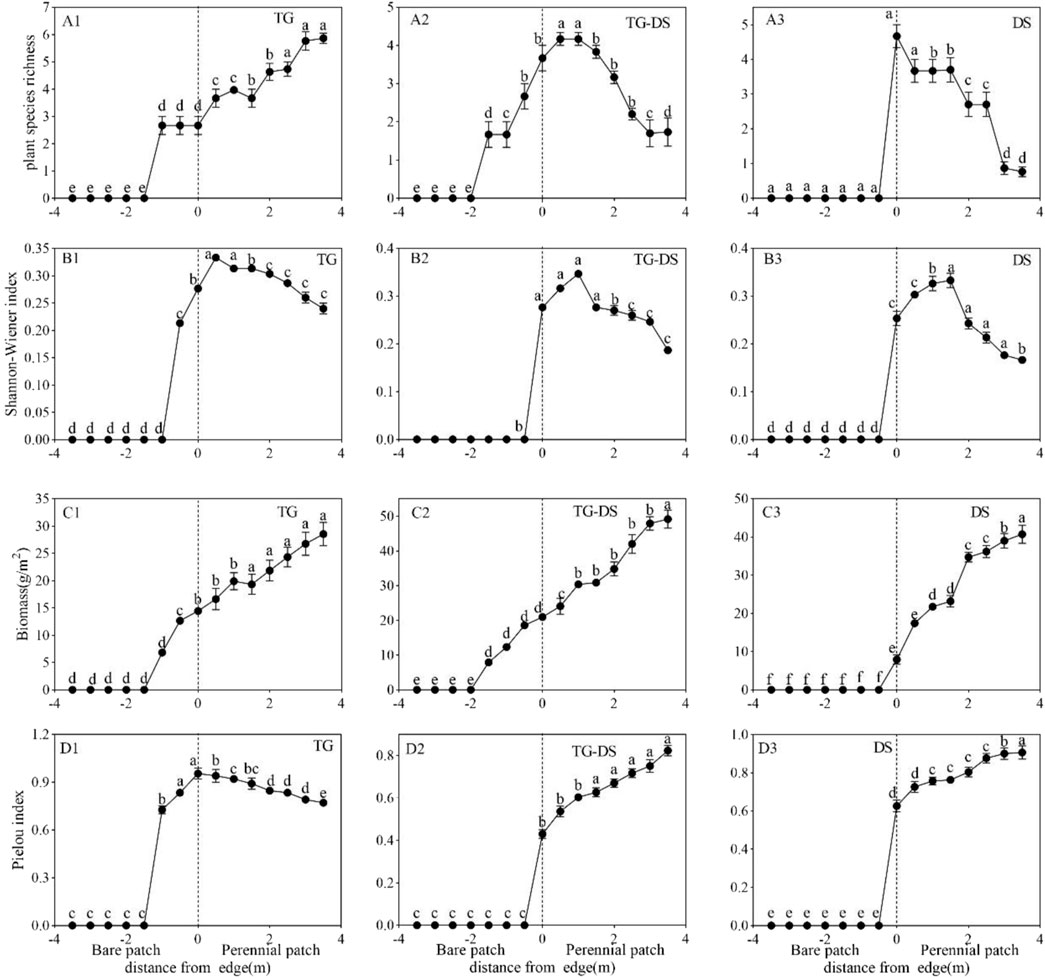
FIGURE 2. Species richness (A1,A2,A3), Shannon-Wiener index (B1,B2,B3), plant above-ground biomass (C1,C2,C3), and Pielou index (D1,D2,D3). The graphs with the same letter indicate no significant difference between each other, and with different letters, there is a significant difference.
The biomass of the three types of steppe increased gradually, but the biomass of the transition zone of the two steppes was the largest, which was 52.14 g/m2 (Figure 2C2), 36.54 g/m2 for typical grassland (Figure 2C1), and 43.87 g/m2 for desert grassland (Figure 2C3), but the overall difference between the three is not much.
The Shannon-Wiener index of the typical steppe was the lowest, with a maximum of 0.32 (Figure 2B1). There was little difference between the transition region and the desert steppe, with a maximum of >0.35 (Figures 2B2,B3). However, the Shannon-Wiener index at the patch boundary of the desert steppe was 2.2 times that at 3.5 m, and the Shannon-Wiener index at the transition zone was 2 times that at 3.5 m.
The species richness of the typical grassland is the largest among the three (Figure 2A1), while the richness of the typical grassland increases further away from the patch boundary and only starts to decrease at 3 m from the boundary. In contrast, the trends of desert grassland and transition zone are different from those of typical grassland, both of them have the highest species richness near the boundary and then gradually decline (Figures 2A2,A3), and the richness indices at the boundary of both (0 m) are two and five times higher than those at 3.5 m, respectively.
The Pielou index of the typical grassland is the largest at the boundary, and then decreases steadily and tends to stabilize (Figure 2D1); while the Pielou index of the desert grassland and the transition zone both increase with the distance from the boundary, the transition zone shows a trend of increasing all the time, while the desert grassland starts to increase at 2.5 m from the boundary with a slower increase (Figures 2D2,D3).
Distribution patterns of plant types
In this study, the species richness of all sample areas showed a sudden and large jump at the patch boundary. The species richness of typical grasslands began to change gradually at -1.5 m but tended to be the same at −1–0 m, 0.5–1.5 m, 2–2.5 m, and 3–3.5 m. The dominant species in the typical steppe transect are Allium ramosum, Achnatherum splendens, and Leymus chinensis. Meanwhile, the richness of typical grassland was the highest in this survey, with six species, and the sample variability of all vegetation patches was not significant (p < 0.001). The richness of the desert steppe and transition zone increased to the highest at first, and the richness of 3.5 m farthest from the patch boundary was the lowest. The dominant species in the transition zone was Leymus chinensis, and the patch richness showed a gradual decline with no significant difference (p < 0.001).
In all survey plots, Poaceae showed dominance, with a greater proportion of perennial Poaceae and a peak of absolute dominance at the boundary. The average proportion of Poaceae in typical grasslands was 82.86%, and the percentage of miscellaneous grasses was 17.14%; the average percentage of Poaceae in desert grasslands was 58.82%, and the percentage of miscellaneous grasses was 41.18%; the average percentage of Poaceae in transition areas was 62.50%, and the percentage of miscellaneous grasses was 37.50%.
Spatial patterns of soil properties
In general, there was no pattern in the content of soil properties in this study. The organic matter content of the typical grassland is the largest among the three grassland types, with a maximum value of 27.98 g/kg (Figure 3D1), while the desert grassland and the transition zone are only just ≥10 g/kg (Figures 3D2,D3). Among them, the organic matter content of typical grasslands has been increasing from bare patches to vegetation patches, while the organic matter content of transition zones and desert grasslands shows a trend of first increasing, maximizing at the junction of patches, and then decreasing. The trend of fast-acting phosphorus was also basically the same, both showed a trend of increasing and then decreasing, and the maximum values were at the junction of the patches. The size of the quick-acting phosphorus content did not vary much among the three, all varying between 10 and 16 g/kg (Figure 3B). The trends of soil nitrogen were different again: the nitrogen content of the three was desert grassland > typical grassland > transition zone. The highest content of nitrogen was 110.95 g/kg in the desert steppe (Figure 3A3), which was 7–10 times that in the desert steppe and transition zone. The nitrogen content in desert grassland was increasing all the time, and there was a great difference between bare patches and vegetation patches. Typical grasslands first show an increasing trend and then start to decrease around 3–3.5 m (Figure 3A1). The transition zone showed an increasing-then decreasing trend, and the alkaline nitrogen content was greatest at the junction of the patches (Figure 3A2). The difference in soil fast-acting potassium content between desert grassland and typical grassland is small, but the difference between the transition zone and both is large, and both are 10 times larger than the transition zone. The overall change trend of the three grasslands is also different, and the typical grassland shows a steady increase trend from bare patch to vegetation patch (Figure 3C1); the transition zone showed a trend of first growth and then decrease, and the maximum value was near the junction, and the maximum value was only 0.038 g/kg (Figure 3C2). In contrast, the desert grassland showed a continuous decreasing trend from bare ground patches to vegetated patches (Figure 3C3).
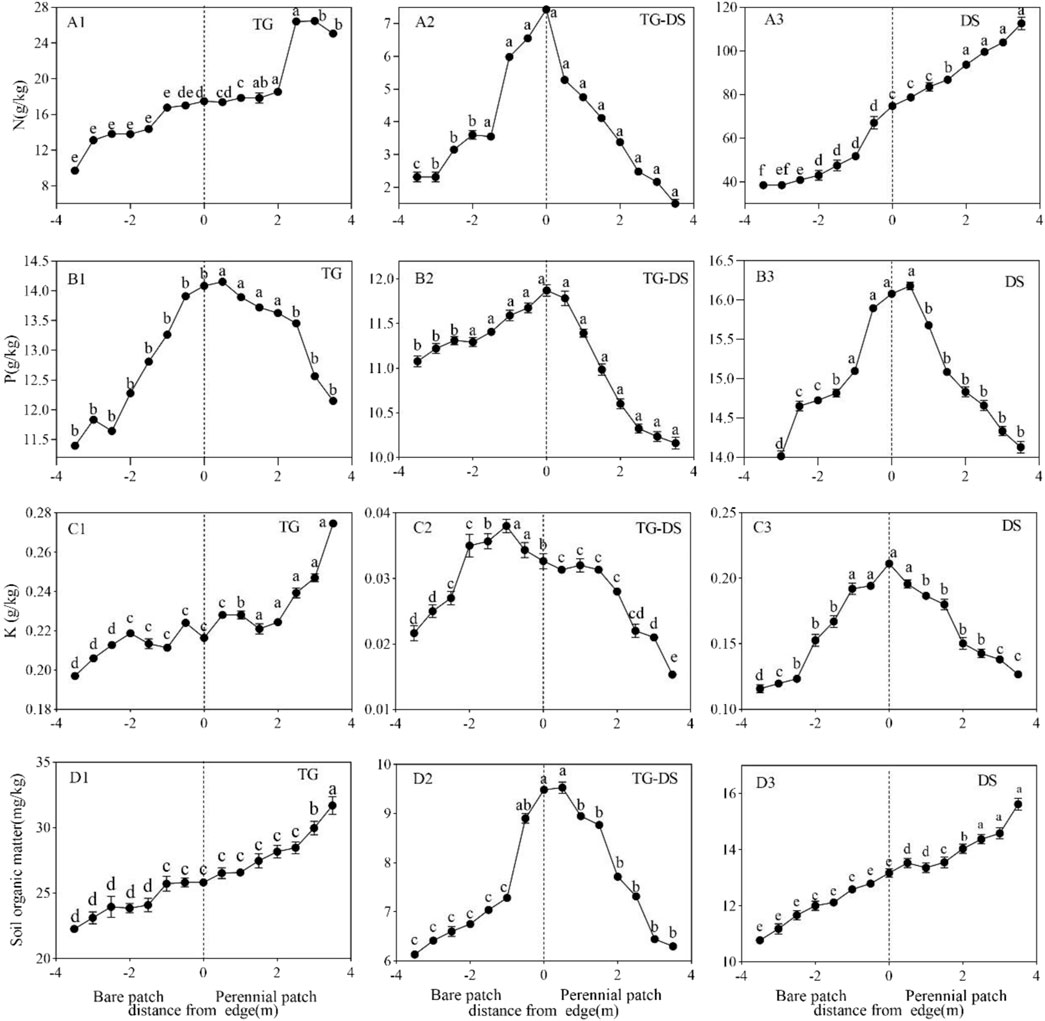
FIGURE 3. Soil nitrogen (A1,A2,A3), soil available phosphorus (B1,B2,B3), soil available potassium (C1,C2,C3), and Soil organic matter (D1,D2,D3). The same letters in the figure indicate that there are no significant differences between them, while different letters indicate significant differences.
Relationship between plant and environmental characteristics
NMDS was used to analyze the change characteristics of communities and soils with the change of patch boundaries, combined with the RDA method to analyze the potential changes and correlations of vegetation community and soil characteristics. The axis extracted by RDA represents the change directly related to environmental variable factors.
Among the changes in typical grassland, the first two axes of the RDA method accounted for 89.6% of the seven environmental factors. The final results of variation allocation showed that the independent contribution value of organic matter was 76.8%, followed by soil total phosphorus at 11.9%. The independent contribution values of soil nitrogen and total nitrogen were 2.7% and 1.6%, respectively, and the independent contribution values of other environmental factors were all <1% (Figure 4A2). Soil organic matter, alkali-hydrolyzable nitrogen, and available potassium contents were most positively correlated with patch distance and biomass, combined with NMDS analysis (Figure 4A1), with the increase of the distance from the bare patch, the distance from the vegetation patch decreased, biomass and Pielou index of high organic matter, high nitrogen, and high available potassium content increased, while richness decreased.
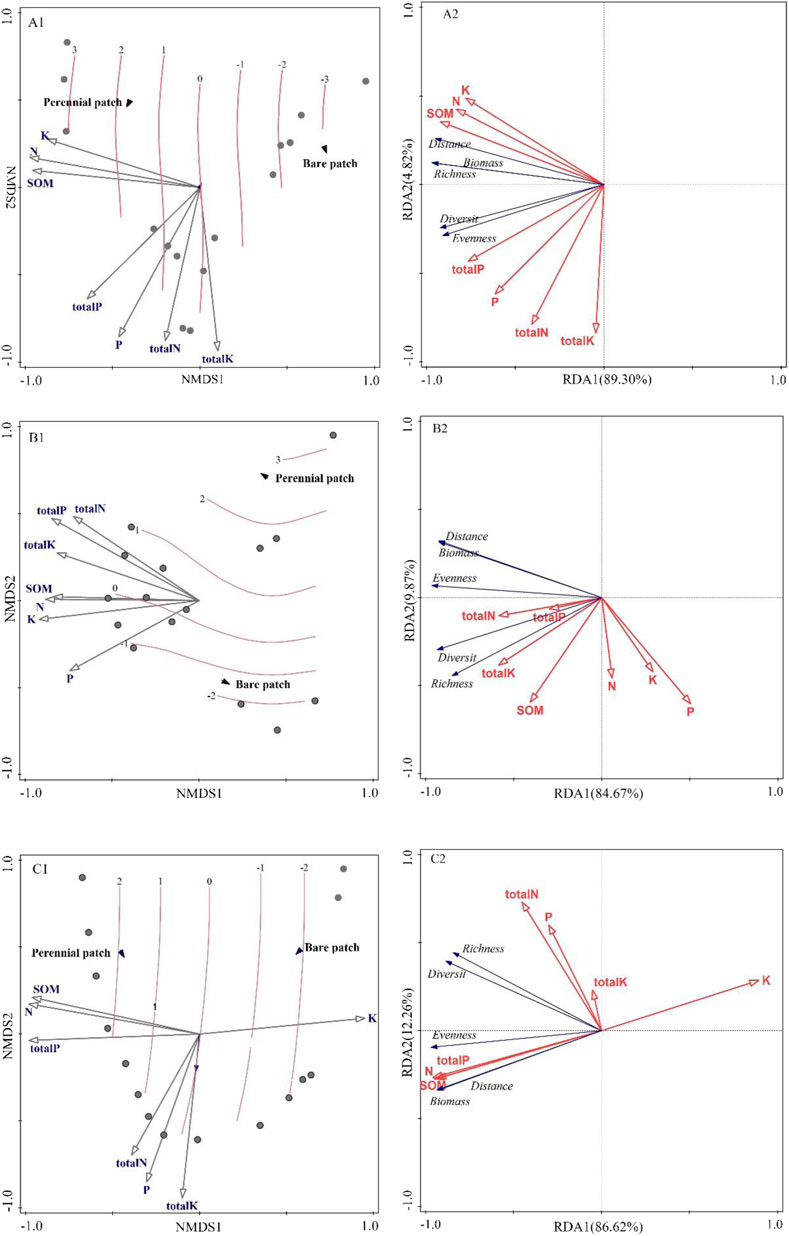
FIGURE 4. Two-dimensional sequence diagrams of soil and vegetation communities analyzed by RDA and NMDS. (A1–C1) Based on the Bray–Curtis distance. Method, the soil characteristics and vegetation community characteristics closely related to patch boundaries were arranged by NMDS. The pink contour is a smooth response surface arranged based on the gradient of boundary distance. The influence of soil characteristic parameters on vegetation community is represented by the length of the arrow, and the triangle represents the two-dimensional location of the patch. (A2–C2) RDA ranking of vegetation community characteristics and soil parameter characteristics in boundary effects. Red represents soil characteristic parameters and blue represents vegetation community characteristic parameters.
In the transition zone between typical grassland and desert grassland, the first two axes of RDA accounted for 91.2% of the seven environmental factors. The results of variation allocation showed that available potassium accounted for 51.1% of the total nitrogen, followed by total phosphorus, available potassium, and total potassium, 7.9%, 3.0%, 1.9%, and 1.1%, respectively, and nitrogen <1% (Figure 4B2). Combined with NMDS analysis patch biomass was positively correlated with total nitrogen, total phosphorus, and total potassium with the increase of forwarding distance from the patch boundary, the correlation between total nitrogen and total nitrogen is smaller than the first two. There was no correlation with organic matter, but a high negative correlation with nitrogen, available potassium, and available phosphorus (Figure 4B1).
In the desert grassland data, the first two axes explained 93.10% of these seven environmental factors. The results of variation allocation showed that the independent contribution value of organic matter was the highest at 77.6%, followed by total nitrogen at 13.9% and total phosphorus at 4.2%, and the rest of the environmental factors had independent contribution values < 1%. The contents of organic matter, nitrogen, and total phosphorus were positively correlated with patch distance and biomass. Total nitrogen, available phosphorus, and total potassium had little correlation with patch distance and biomass, while available potassium had a negative correlation with patch distance and biomass (Figure 4C2). Combined with NMDS plot analysis, it is clear that with decreasing distance from vegetation patches, high organic matter, high alkaline soluble nitrogen and high total phosphorus content and low effective potassium content, biomass and Pielou index increased (Figure 4C1).
Boundary description
The discontinuous positions of the peaks and their variations are derived from the Euclidean distance calculations applied to plant characteristics and soil properties. The results of the study showed that mutations occurred in different locations of each indicator (Figure 5). However, the location of mutations in different types of grasslands in this study showed differently.
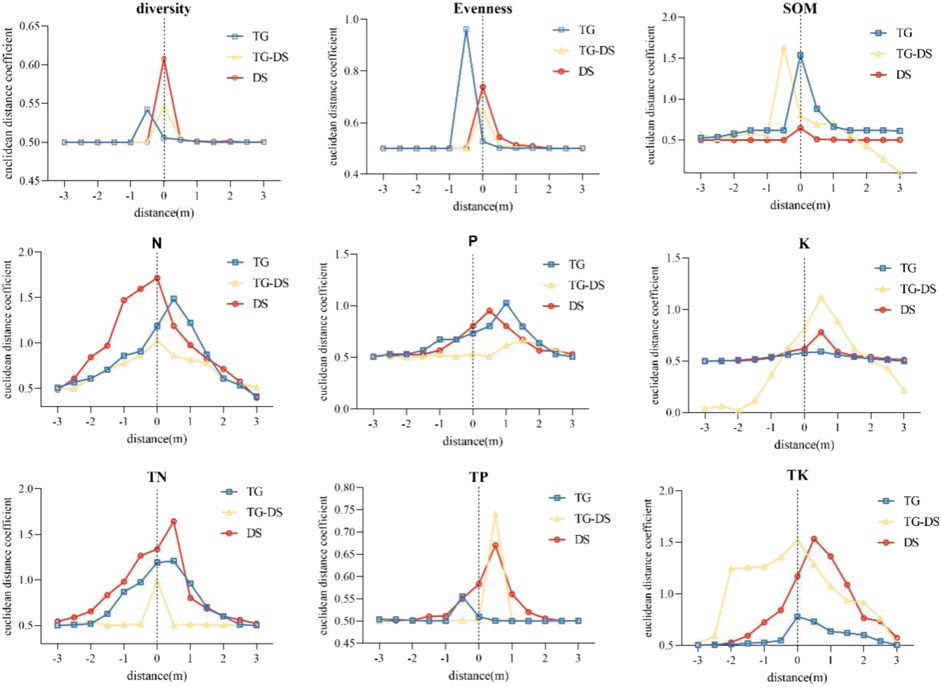
FIGURE 5. Euclidean distance coefficients of plant species richness and Shannon-Wiener index indices and soil properties along with vegetation patches and bare patches. The peak value of the curve is the place where the index changes the most, which is also the statistical identification boundary of this study.
The mutation point of organic matter in typical steppe and desert steppe is located at 0m, but the transition zone is located at −0.5 m. Except for organic matter, the mutation locations of all soil indicators were within the vegetation patches. Soil nitrogen is located at 0 m in both desert grassland and transition zone, but at 0.5 m in typical grassland; soil available phosphorus is located at 1 m in typical grassland, 1.5 m in the transition zone, and 0.5 m in desert grassland. All three types of grassland available phosphorus mutations are located at 0.5m, but among them, the typical grassland mutation is not obvious. The total nitrogen in typical grassland and desert grassland is then located at 0.5 m, and the transition zone is at 0 m. Total potassium was located at 0.5 m in desert grassland and transition zone and was -0.5 m in the typical grassland, available potassium, which is typically located at 0 m for grasslands and transition zones and 0.5 m for desert grasslands.
In general, mutation locations mostly occurred at −0.5–1 m, with 0.5 m being the majority, so the peak Euclidean distance indicates a different boundary between plant and soil variables.
Discussion
Many previous studies have explored and demonstrated the causes of boundary structure formation at large and mesoscale, as well as the interaction between biological and abiotic factors, but the direction of interaction has not been shown. By analyzing the spatial variation of plant distribution, community characteristics, and soil characteristic parameters at a fine scale, we know that the community characteristic parameters are affected by the change of soil characteristic parameters, but they are not completely consistent with the change of soil characteristic parameters. Therefore, it can be concluded from the study that, This change can be explained by superimposed plant-soil feedback mechanisms that maintain soil conditions.
Spatial pattern of plant variables and soil characteristics
The results of this study showed that the change of boundary was closely related to the change of soil indexes, and usually, the mutations in boundaries were at the locations where the changes in soil indicators occurred. And these mutations hint at the underlying relationship between soil and plants. According to the results of this study, the contents of organic matter, total phosphorus, and nitrogen were the biggest indexes affecting boundary changes in all types of grassland.
These results are similar to those found in previous studies of soil and vegetation indicators at the boundary (Cao et al., 2019, Maas et al, 2016). Meanwhile, the results of this study found that the changes in vegetation richness were most influenced by organic matter, and the changes in vegetation richness and organic matter were completely consistent in all types of grasslands (Figure 2A, Figure 3D). According to previous studies, this research result is also limited by the accumulation of plant biomass (Bai et al., 2015; Ford et al., 2016), compared with this study, the conclusion is similar because the change of biomass affects the mutation location of richness and thus the mutation location of organic matter during the transition from bare patch to vegetation patch. Specifically, places with high richness also have more growth of perennial herbaceous plants (Most of the articles are from Poaceae). Perennials are usually more adaptable to the heterogeneity of soil and adapt to the habitat by changing their morphological structure and growth habits (Oom et al., 2010; Moore, 2015). They usually give priority to areas that are relatively favorable to their growing environment, which explains why vegetation richness is higher at the boundary between bare patches and vegetation patches than inside vegetation patches (Zhang et al., 2014; Mason et al., 2016).
RDA analysis of three grassland types showed that soil nitrogen and phosphorus content was the most important predictor of plant richness and biomass change. This result is consistent with the results of several current studies (Güsewell, 2004; Mariotte et al., 2020). The results of the study by DeMalach, N confirmed that different nitrogen and phosphorus additions are the main factors influencing species richness, among which the “nitrogen to phosphorus ratio hypothesis” is considered to be more objective (DeMalach, 2018). This hypothesis proposes that nitrogen and phosphorus contents above 10–20% of plant tissues relative to biomass represent the “optimum balance” for the coexistence of nitrogen and phosphorus, but higher or lower values than this limit it, suggesting that a more balanced nitrogen and phosphorus content is better than the highest abundance.
It is also worth noting that several studies have shown that soil pH is a key factor that directly affects soil organic matter and thus vegetation conditions (Moreno-de las Heras et al., 2016; Osman et al., 2019). However, during the preliminary experiments of this study, the pH did not vary much between samples, and the variation between samples was <1, which was not the same as the trend of organic matter, nor was it related to nitrogen and phosphorus content, so the pH was not used as one of the main research indicators in the subsequent experiments. At the same time, a large amount of literature was reviewed and it was found that the soil salinity-alkalinity in the study area with a high pH influence had a great change, while the changing trend of pH in this paper was too small to be taken as one of the indicators. Therefore, nitrogen and phosphorus were considered the most important influencing factors in this study area. To test this idea, this study used SEM to verify the relationship between some soil indicators and community indicators.
In this paper, only one SEM diagram was presented for the three grassland types, including nitrogen, phosphorus, potassium, total nitrogen, total phosphorus, total potassium, soil organic matter, and three vegetation community indexes (biomass, richness, and Pielou index). Different soil indicators and community indicators were selected to construct the equations, and finally, the best equation effects were found for nitrogen, phosphorus, potassium, and soil organic matter with community indicators. SEM data showed that both phosphorus and nitrogen had a significant effect on richness in all three types of sample plots, nitrogen content had a negative effect on the richness, but phosphorus obviously had a positive effect on the richness (Figure 6). One explanation is that an increase in nitrogen content significantly promotes an increase in biomass, which leads to species’ competitive exclusion, therefore it will reduce the species richness (Zhao et al., 2019). The negative effects of nitrogen on plant richness have been confirmed by experiments, and the long-term accumulative effects on the stability of the whole ecosystem have also been confirmed (Melts et al., 2018). It has also been illustrated that it is the combined effect of nitrogen and phosphorus that will have a positive effect on plant abundance, rather than specific nitrogen to phosphorus ratio (Zhang et al., 2022).
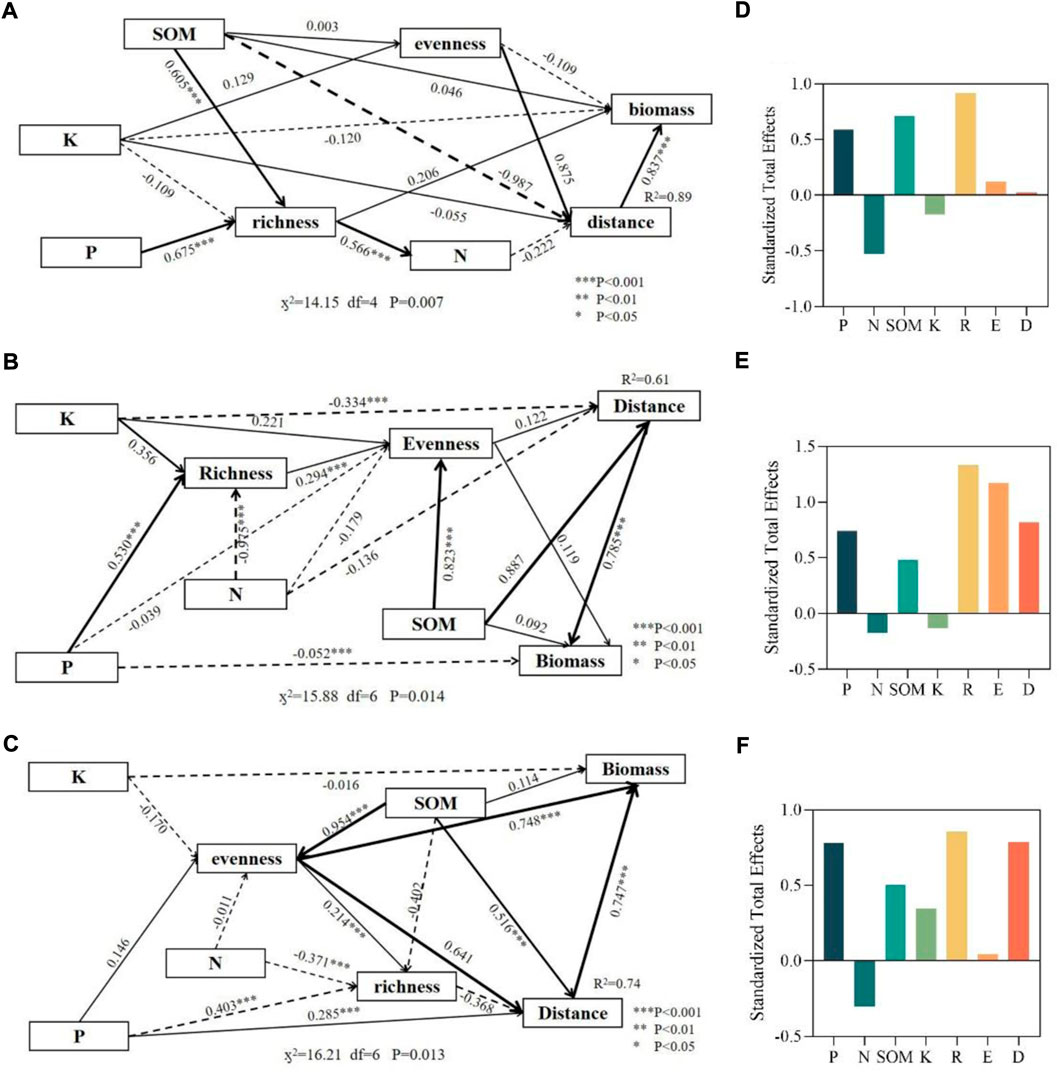
FIGURE 6. SEM investigates the multivariate effects of P (phosphorus), N, K, and SOM on R (richness) and boundary distance. Solid lines indicate positive correlations and dashed lines indicate negative correlations; the thickness of the arrows indicates the magnitude of the normalized path coefficients. (A) and (D) represent typical grasslands, where RMSEA = 0.016, NFI = 0.942, CFI = 0.959; (B) and (E) represent transition zones, where RMSEA = 0.008 NFI = 0.947, CFI = 0.964; (C) and (F) represent desert grasslands, where RMSEA = 0.018, NFI = 0.996, CFI = 0.995. R2 values represent the proportion of variance explained by each variable, RMSEA, root means the square error of approximation.
Boundary description
In all the grasslands studied in this study, geomorphic changes between patches are very obvious. The mutation itself is visually obvious, and it is accompanied by the mutation of species richness and biomass, which forms a transition zone. However, the mutation locations of the indicators in these transition zones are not identical, nor are they completely consistent in space. In the plot of soil indicator variables, the peak is the mutation at the transition zone (Figure 5), the mutation is the boundary, and most indicators peaked at 0–1 m, which is the junction between vegetation patches and bare ground patches. Hobbs, E. R suggested as early as 1986 that there are two relatively different boundaries (Hobbs, 1986). One is the boundary between two different patches (shrub-grassland, meadow-grassland, or bare land-grassland) marked by geomorphic changes in vegetation, and they are usually strongly related to the structure and properties of the soil. However, there is also a kind of boundary that exists, generally without abrupt changes in the landscape, but identified by gradual changes in the dominant plant species, and this kind of boundary generally exists in places with large disturbances, such as long-term grazing, pressure mining, etc.
The soils of the types of grasslands studied in this study are relatively stable (undisturbed), so all properties reflect long-term formation conditions and are much less sensitive to short-term changes in certain indicators. In addition, different vegetation community characteristics reflect the underlying processes occurring at different boundaries within such environments. Because plant community characteristics are much more variable than soil characteristics, the joint action of different indicators (mainly species richness and biomass) will determine the location of boundaries. It can be seen that in determining stable and healthy ecological boundaries, soil indicator parameters are more suitable for reference than vegetation community characteristics.
Landscape dynamic characteristics of the patches
From the perspective of the landscape, the formation, development, and reduction of patch boundaries on grassland will determine the dynamic change of patch expansion or disappearance (Jäkäläniemi et al., 2005; Cao et al., 2019). When the patch expands or contracts dynamically, it changes the location of the patch boundary. In the grassland landscape of this study, patch boundaries are closely related to some soil indexes, but not completely consistent. The dynamic direction of the boundary is reflected in the succession pattern of the boundary, and it can be found that the vegetation patches are evolving to bare ground patches in this study. At the sampling site, a few independent Poaceae appeared in bare patches, and some Poaceae at the boundary were evolving into bare patches, which is why the species richness at the boundary was higher than that in the two patches. At the same time, this evolution is more conducive to the dynamic growth of vegetation in the patch. So underlying changes in soil properties set the direction of community evolution. In this environmental change, micro-scale interactions ultimately drive the development of patch boundary formation and changes in landscape patterns (Junhong et al., 2008; Lin et al., 2010).
The direction of change in landscape patches
This paper will combine the results of this study with the conclusions of previous scholars to summarize the dynamic process of patches in the landscape. Figure 7 summarizes a conceptual model that can only characterize the patch variation in the region at this stage of the study, only examining the correlation between soil conditions and species composition at this stage, and the interaction between the two, resulting in a particular transition path between the two patch types. The direction of vegetation change is significantly correlated with the distance of each environmental factor, and this result is also clearly verified by SEM in Figures 6A–C. Our analysis of the characteristics of plant species in the continuous quadrat also showed that changes in species richness and distance had a very large effect. Previous studies have confirmed that such changes in species richness indicated that there had been a process of succession and iteration in this region (Collins et al., 2009). A clear successional trend in flora change can be seen: perennial grasses-perennial grasses + annuals grasses-Bare. Therefore, it can be seen that vegetation is developing into bare patches and there is a process of planting restoration (Figure 7). In combination with the field survey, one or several rare annual plants oRDAsionally appeared in bare patches, indicating that the succession of plants to bare patches was similar to the spatial sequence of succession to the boundary of vegetation patches. And conversely, the process of degenerate succession can be summarized as Bare-perennial grasses + annuals grasses-perennial grasses. This area may have been disturbed as the grassland degraded.
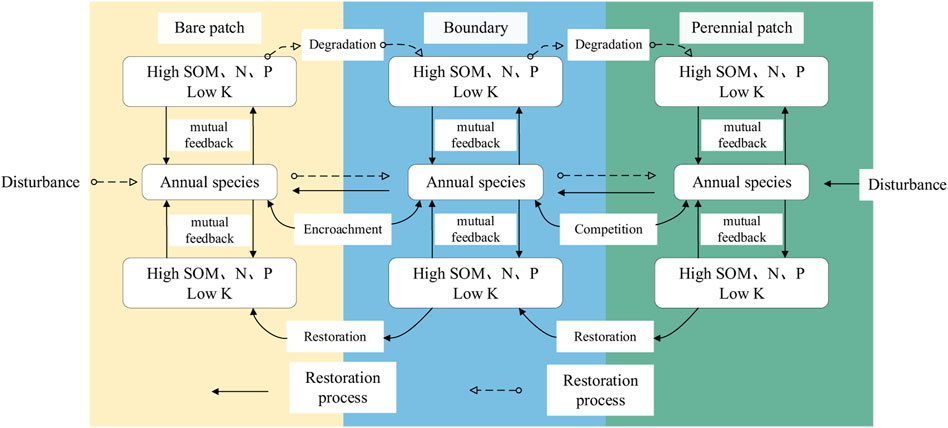
FIGURE 7. Conceptual framework for the boundary dynamics of bare ground patches and perennial patches.
Patchy grasslands are formed by different vegetation left in different successional stages, and these patchy boundaries are the key to influencing the change of landscape patches in this area (Arroyo-Rodríguez et al., 2017). Although the vegetation types of the three different grassland types in this study are not all the same, there are some parts of the same plant species. This indicated that the direction of patch change was the same from the perspective of landscape succession, even if the type of grassland was different. Meanwhile, from the results of the present study, soil nitrogen and phosphorus content may be the main factor limiting the success of grassland patches in the study area (Figures 6A–C). This may be because nitrogen and phosphorus levels influence the carbon cycle, which affects vegetation growth and interactions and may have unintended effects on the landscape (Wassen et al., 2013; Hedwall et al., 2017). Based on the data of community indicators and soil properties of the patch boundaries, combined with the plant species composition survey of the sample area showed that: compared with patches with only perennial vegetation, patches with annual and perennial vegetation had more plant species, which created conditions for the restoration of vegetation to bare patches. The scattered perennial patches were random and irregular in all areas of this sampling. In the course of patch succession, perennial vegetation excludes other types of vegetation by factors such as nutrient level (Sun et al., 2017). In particular, Poaceae, the most abundant type in the study area, are not conducive to plant diversity, but they spread easily and accelerate the process of succession (Bartha et al., 2014; Sojneková and Chytry, 2015). At the same time, soil, in turn, affects plant growth due to changes in soil properties caused by plants, enabling the formation of a reciprocal soil-plant feedback mechanism. Scholars studying the Songnen Grassland have long concluded, that perennial Poaceae plants are the dominant species in alkali-saline grassland. They develop habitats by influencing boundaries through vegetation-soil coupling mechanisms (Zhang et al., 2014; Zhao, et al., 2017). However, this does not mean that perennial herbs have no positive effect on succession, that perennial Poaceae can improve soil conditions through vegetation-soil feedback, allowing species to reduce competition and promote succession. In general, different mechanisms of soil-vegetation formation act on different spatial scales, It promotes patch production, forming dynamic boundaries.
Different soil conditions are the main abiotic environmental factors that determine patch spatial structure. We found that the boundary change interval of plant and soil properties is different. This result is different from previous studies, which have shown that the boundary between vegetation and soil changes in the same range (Good et al., 2013). The difference in the results suggests that our above hypothesis is correct. That is, if the vegetation and soil change almost identically, it means that the vegetation has not changed much in the past. However, if the region is disturbed and the balance of vegetation-soil feedback is disrupted, then the transition zone of the patch tends to be a dynamic process (Liu et al., 2016). According to the boundary dynamics view (de Agar et al., 2016), there are two more different extremes between the patches, and the boundary also maintains a balance in this extreme state. However, if disturbed, the dynamic balance of the boundary is broken, and the patch landscape will change. Specifically, the undisturbed state is more conducive to the growth of perennial patches. However, after the disturbance, the disturbance changed the richness of vegetation, and then affected the change of soil index, therefore, the boundary will move and the pattern of patches will gradually change. According to this study, the most important influencing factor should be nitrogen and phosphorus content. To articulate the above relationships, this study proposes a conceptual flow framework that attempts to clarify the underlying mechanisms of the patch boundaries (Figure 7). However, this study only provides a framework, and further disturbance experiments on grassland patches are needed to demonstrate that disturbance leads to changes in the soil-vegetation feedback predicted by the framework, which in turn changes the landscape of the boundary. The concept of the framework is basically that the structure of the patch is mainly determined by the soil properties and the abundance of plants is mainly determined by the species of the patch. Soil-vegetation mutual feedbacks have an important influence on plant succession and soil development within patches. At the same time, the boundaries are dynamically changing and major abiotic factors constrain the aforementioned reciprocal mutual feedback. This process will eventually change the characteristics of patches, affect the change of boundaries and drive the change of landscape pattern. These findings are more useful for understanding the process of patch boundary occurrence and have implications for predicting changes in landscape patterns and thus preventing such changes in advance.
Conclusion
There is no pattern in the location of mutations in vegetation soil indicators of the three types of grasslands. The biomass of the three grassland types increased with the distance. The species richness of the typical steppe also showed an increasing trend. The mutation position of species richness in desert grassland and transition zone was between −1.5 m and 0 m. The mutation positions of the Pielou index of the three grassland species ranged from −1 m to 0 m. And the Shannon-Wiener index is almost the same, between −1 m and 0 m. The organic matter in typical grassland increased continuously, while in desert grassland and transition zone, the variation ranged from −0.5–1 m. All available phosphorus mutations were in the range of from −0.5–1 m. Desert grassland and typical grassland nitrogen showed an overall increasing trend, but the transition zone mutation remained at 0–1 m. In contrast, the trends of the three types of fast-acting potassium were completely different.
NMDS and RDA analysis charts showed that organic matter contributed the most in typical grassland and desert grassland, accounting for 76.8% and 77.6%, respectively. Typical grasslands were 11.9% total phosphorus, 2.7% alkaline nitrogen, and 1.9% total nitrogen. Desert grasslands were 13.9% total nitrogen and 4.2% total phosphorus. However, the transition zone was completely different from the first two, with the highest contribution of 51.1% of fast-acting phosphorus, followed by total nitrogen and phosphorus with 7.9% and 3.0%, respectively. However, in terms of distance, all three were the same, with high rates of phosphorus and nitrogen as well as potassium positively correlated with the increase in biomass.
The boundary peak calculated by Euclidean distance did not coincide with the vegetation community characteristics and soil indexes. The boundary peak value of the community index appeared between -1 m and 0 m. The peak values of soil indexes were between -0.5 and 1 m. Combined with NMDS and SEM, it can be seen that soil indexes are the main factors affecting the change in patch boundary, and the changes in organic matter, nitrogen, and phosphorus are the most important factors.
Data availability statement
The original contributions presented in the study are included in the article/supplementary material, further inquiries can be directed to the corresponding author.
Author contributions
NW and ZM contributed conception and design of the study. HQ and HZ performed the statistical analysis. XR reviewed and revised the manuscript. All authors contributed to manuscript revision, read and approved the submitted version. YJ Correction and improvement of the grammar at the end of the whole text, XD gave advice on reworking.
Funding
This research was supported by the National Natural Science Foundation of China (42067015), the Natural Science Foundation of Inner Mongolia Autonomous Region (2020MS03038), and Desert Ecosystem Conservation and Restoration Innovation Team.
Conflict of interest
The authors declare that the research was conducted in the absence of any commercial or financial relationships that could be construed as a potential conflict of interest.
Publisher’s note
All claims expressed in this article are solely those of the authors and do not necessarily represent those of their affiliated organizations, or those of the publisher, the editors and the reviewers. Any product that may be evaluated in this article, or claim that may be made by its manufacturer, is not guaranteed or endorsed by the publisher.
References
Arroyo‐Rodríguez, V., Melo, F. P., Martínez‐Ramos, M., Bongers, F., Chazdon, R. L., Meave, J. A., et al. (2017). Multiple successional pathways in human‐modified tropical landscapes: New insights from forest succession, forest fragmentation and landscape ecology research. Biol. Rev. 92 (1), 326–340. doi:10.1111/brv.12231
Bai, Z., Gao, Y., Xing, F., Sun, S., Jiao, D., Wei, X., et al. (2015). Responses of two contrasting saline‐alkaline grassland communities to nitrogen addition during early secondary succession. J. Veg. Sci. 26 (4), 686–696. doi:10.1111/jvs.12282
Bao, H. M. (2018). Remote sensing monitoring of grassland vegetation greenupand the correlativity between greenup and meteorological factors: A case study of Xilingol League. Inn. Mong. Agric. Univ. 40, 2190–2211. doi:10.1080/01431161.2018.1506185
Bartha, S., Szentes, S., Horváth, A., Házi, J., Zimmermann, Z., Molnár, C., et al. (2014). Impact of mid‐successional dominant species on the diversity and progress of succession in regenerating temperate grasslands. Appl. Veg. Sci. 17 (2), 201–213. doi:10.1111/avsc.12066
Bátori, Z., Erdős, L., Kelemen, A., Deák, B., Valkó, O., Gallé, R., et al. (2018). Diversity patterns in sandy forest-steppes: A comparative study from the Western and central palaearctic. Biodivers. Conserv. 27 (4), 1011–1030. doi:10.1007/s10531-017-1477-7
Botha, M., Archibald, S., and Greve, M. (2020). What drives grassland‐forest boundaries? Assessing fire and frost effects on tree seedling survival and architecture. Ecol. Evol. 10 (19), 10719–10734. doi:10.1002/ece3.6730
Burst, M., Chauchard, S., Dambrine, E., Dupouey, J. L., and Amiaud, B. (2020). Distribution of soil properties along forest-grassland interfaces: Influence of permanent environmental factors or land-use after-effects? Agric. Ecosyst. Environ. 289, 106739. doi:10.1016/j.agee.2019.106739
Cao, Y., Wang, D., Heino, M., Li, X., Zhu, H., Liu, J., et al. (2019). Fine-scale characteristics of the boundaries between annual patches and perennial patches in a meadow steppe. Landsc. Ecol. 34 (4), 811–825. doi:10.1007/s10980-019-00805-y
Chandra, L. R., Gupta, S., Pande, V., and Singh, N. (2016). Impact of forest vegetation on soil characteristics: A correlation between soil biological and physico-chemical properties. 3 Biotech. 6 (2), 188–212. doi:10.1007/s13205-016-0510-y
Chen, Z., Liang, K., Ding Steven, X., Yang, C., Peng, T., and Yuan, X. (2021). A comparative study of deep neural network-aided canonical correlation analysis-based process monitoring and fault detection methods. IEEE Trans. Neural Netw. Learn. Syst. 1, 15. doi:10.1109/TNNLS.2021.3072491
Collins, C. D., Holt, R. D., and Foster, B. L. (2009). Patch size effects on plant species decline in an experimentally fragmented landscape. Ecology 90 (9), 2577–2588. doi:10.1890/08-1405.1
de Agar, P. M., Ortega, M., and de Pablo, C. L. (2016). A procedure of landscape services assessment based on mosaics of patches and boundaries. J. Environ. Manag. 180, 214–227. doi:10.1016/j.jenvman.2016.05.020
DeMalach, N. (2018). Toward a mechanistic understanding of the effects of nitrogen and phosphorus additions on grassland diversity. Perspect. Plant Ecol. Evol. Syst. 32, 65–72. doi:10.1016/j.ppees.2018.04.003
Didham, R. K., and Lawton, J. H. (1999). Edge structure determines the magnitude of changes in microclimate and vegetation structure in tropical forest fragments. Biotropica 31 (1), 17–30. doi:10.2307/2663956
Dovčiak, M., and Brown, J. (2014). Secondary edge effects in regenerating forest landscapes: Vegetation and microclimate patterns and their implications for management and conservation. New For. 45 (5), 733–744. doi:10.1007/s11056-014-9419-7
Erdős, L., Krstonošić, D., Kiss, P. J., Bátori, Z., Tölgyesi, C., and Škvorc, Ž. (2019). Plant composition and diversity at edges in a semi-natural forest-grassland mosaic. Plant. Ecol. 220 (3), 279–292. doi:10.1038/ismej.2015.118
Fenu, G., Carboni, M., Acosta, A. T., and Bacchetta, G. (2013). Environmental factors influencing coastal vegetation pattern: New insights from the mediterranean basin. Folia Geobot. 48 (4), 493–508. doi:10.1007/s12224-012-9141-1
Ferro, I., and Morrone, J. J. (2014). Biogeographical transition zones: A search for conceptual synthesis. Biol. J. Linn. Soc. Lond. 113 (1), 1–12. doi:10.1111/bij.12333
Ford, H., Roberts, A., and Jones, L. (2016). Nitrogen and phosphorus co-limitation and grazing moderate nitrogen impacts on plant growth and nutrient cycling in sand dune grassland. Sci. Total Environ. 542, 203–209. doi:10.1016/j.scitotenv.2015.10.089
Good, M. K., Reid, N., Price, J., Clarke, P., Wilson, B., and Hunter, J. (2013). Plant community patterns and population dynamics of coolibah woodlands. Natural Resource Management. Thesis. Available at: https://hdl.handle.net/1959.11/13484
Guo, J. (2016). Remote sensing monitoring of grassland vegetation greenup and the correlativity between greenup and meteorological factors: A case study of Xilingol League. Beijing, China: China National Knowledge Infrastructure, Chinese Academy of Agricultural Sciences.
Güsewell, S. (2004). N: P ratios in terrestrial plants: Variation and functional significance. New phytol. 164 (2), 243–266. doi:10.1111/j.1469-8137.2004.01192.x
Hedwall, P. O., Bergh, J., and Brunet, J. (2017). Phosphorus and nitrogen co-limitation of forest ground vegetation under elevated anthropogenic nitrogen deposition. Oecologia 185 (2), 317–326. doi:10.1007/s00442-017-3945-x
Hobbs, E. R. (1986). Characterizing the boundary between California annual grassland and coastal sage scrub with differential profiles. Vegetatio 65 (2), 115–126. doi:10.1007/bf00044882
Jäkäläniemi, A., Tuomi, J., Siikamäki, P., and Kilpiä, A. (2005). Colonization‐extinction and patch dynamics of the perennial riparian plant, Silene tatarica. J. Ecol. 93 (4), 670–680. doi:10.1111/j.1365-2745.2005.01001.x
Junhong, B., Hua, O., Baoshan, C., Qinggai, W., and Hui, C. (2008). Changes in landscape pattern of alpine wetlands on the Zoige Plateau in the past four decades. Acta Ecol. Sin. 28 (5), 2245–2252. doi:10.1016/s1872-2032(08)60046-3
Kim, J. H., Fourcaud, T., Jourdan, C., Maeght, J. L., Mao, Z., Metayer, J., et al. (2017). Vegetation as a driver of temporal variations in slope stability: The impact of hydrological processes. Geophys. Res. Lett. 44 (10), 4897–4907. doi:10.1002/2017gl073174
Ladwig, L. M., Bell-Dereske, L. P., Bell, K. C., Collins, S. L., Natvig, D. O., and Taylor, D. L. (2021). Soil fungal composition changes with shrub encroachment in the northern Chihuahuan Desert. Fungal Ecol. 53, 101096. doi:10.1016/j.funeco.2021.101096
Li, J., Liu, Y., Hai, X., Shangguan, Z., and Deng, L. (2019). Dynamics of soil microbial C: N: P stoichiometry and its driving mechanisms following natural vegetation restoration after farmland abandonment. Sci. Total Environ. 693, 133613. doi:10.1016/j.scitotenv.2019.133613
Li, X., and Zhang, Y. (2018). Grey relation analysis on soil fertility as influenced by edge effects of moss crust patch in a temperate desert. J. desert Res. 39 (03), 17–24.
Liding, C., Jianying, X., Bojie, F., and Yihe, L. (2004). Quantitative assessment of patch edge effects and its ecological implications. Acta Ecol. Sin. 24 (9), 1827–1832.
Lin, Y., Hong, M., Han, G., Zhao, M., Bai, Y., and Chang, S. X. (2010). Grazing intensity affected spatial patterns of vegetation and soil fertility in a desert steppe. Agric. Ecosyst. Environ. 138 (3-4), 282–292. doi:10.1016/j.agee.2010.05.013
Liu, C., Song, X., Wang, L., Wang, D., Zhou, X., Liu, J., et al. (2016). Effects of grazing on soil nitrogen spatial heterogeneity depend on herbivore assemblage and pre‐grazing plant diversity. J. Appl. Ecol. 53 (1), 242–250. doi:10.1111/1365-2664.12537
Liu, S., Bliss, N., Sundquist, E., and Huntington, T. G. (2003). Modeling carbon dynamics in vegetation and soil under the impact of soil erosion and deposition. Glob. Biogeochem. Cycles 17 (2), 43. doi:10.1029/2002gb002010
Mariotte, P., Cresswell, T., Johansen, M. P., Harrison, J. J., Keitel, C., and Dijkstra, F. A. (2020). Plant uptake of nitrogen and phosphorus among grassland species affected by drought along a soil available phosphorus gradient. Plant Soil 448 (1), 121–132. doi:10.1007/s11104-019-04407-0
Mason, J. A., Jacobs, P. M., Gruley, K. E., Reyerson, P., and Hanson, P. R. (2016). Parent material influence on soil response to vegetation change, Southeastern Minnesota, USA. Geoderma 275, 1–17. doi:10.1016/j.geoderma.2016.04.004
Maas, K. R., Mohn, W. W., and Leung, H. T. C. (2016). Long-term effects of timber harvesting on hemicellulolytic microbial populations in coniferous forest soils. ISME. J. Multidiscip. J. Microb. Ecol., 363–375. doi:10.1038/ismej.2015.118
Melts, I., Lanno, K., Sammul, M., Uchida, K., Heinsoo, K., Kull, T., et al. (2018). Fertilising semi‐natural grasslands may cause long‐term negative effects on both biodiversity and ecosystem stability. J. Appl. Ecol. 55 (4), 1951–1955. doi:10.1111/1365-2664.13129
Moore, E. K. (2015). Red deer (Cervus elaphus) grazing on vegetation mosaics grazing patterns and implications for conservation management. Biological Science. Thesis.
Moreno‐de las Heras, M., Turnbull, L., and Wainwright, J. (2016). Seed‐bank structure and plant‐recruitment conditions regulate the dynamics of a grassland‐shrubland Chihuahuan ecotone. Ecology 97 (9), 2303–2318. doi:10.1002/ecy.1446
Müller, S. C., Overbeck, G. E., Blanco, C. C., de Oliveira, J. M., and Pillar, V. D. (2012). “South Brazilian forest-grassland ecotones: Dynamics affected by climate, disturbance, and woody species traits,” in Ecotones between forest and grassland (New York, NY: Springer), 167–187.
Ni, J. (2001). Plant functional types and biomes of China at a regional scale. Acta Bot. Sin. 43 (4), 419–425.
Oliveras, I., and Malhi, Y. (2016). Many shades of green: The dynamic tropical forest–savannah transition zones. Phil. Trans. R. Soc. B 371 (1703), 20150308. doi:10.1098/rstb.2015.0308
Oom, S. P., Hester, A. J., and Legg, C. J. (2010). Grazing across grass: Shrub boundaries: Can spatial heterogeneity increase resistance? Agric. Ecosyst. Environ. 139 (1-2), 159–166. doi:10.1016/j.agee.2010.07.014
Osman, R., Masubelele, M., Khomo, L., and Chimphango, S. B. (2019). Soil and vegetation differences across ecological boundaries in an arid South African ecosystem. Afr. J. Ecol. 57 (3), 344–352. doi:10.1111/aje.12612
Parks, K. E., and Mulligan, M. (2010). On the relationship between a resource based measure of geodiversity and broad scale biodiversity patterns. Biodivers. Conserv. 19 (9), 2751–2766. doi:10.1007/s10531-010-9876-z
Sojneková, M., and Chytrý, M. (2015). From arable land to species-rich semi-natural grasslands: Succession in abandoned fields in a dry region of central Europe. Ecol. Eng. 77, 373–381. doi:10.1016/j.ecoleng.2015.01.042
Sun, C., Chai, Z., Liu, G., and Xue, S. (2017). Changes in species diversity patterns and spatial heterogeneity during the secondary succession of grassland vegetation on the Loess Plateau, China. Front. Plant Sci. 8, 1465. doi:10.3389/fpls.2017.01465
Teague, W. R., Dowhower, S. L., Baker, S. A., Haile, N., DeLaune, P. B., and Conover, D. M. (2011). Grazing management impacts on vegetation, soil biota and soil chemical, physical and hydrological properties in tall grass prairie. Agric. Ecosyst. Environ. 141 (3-4), 310–322. doi:10.1016/j.agee.2011.03.009
Ting, Z., and Shaolin, P. (2008). Spatial scale types and measurement of edge effects in ecology. Acta Ecol. Sin. 28 (7), 3322–3333. doi:10.1016/s1872-2032(08)60071-2
Veldman, J. W., and Putz, F. E. (2011). Grass-dominated vegetation, not species-diverse natural savanna, replaces degraded tropical forests on the southern edge of the Amazon Basin. Biol. Conserv. 144 (5), 1419–1429. doi:10.1016/j.biocon.2011.01.011
Wassen, M. J., de Boer, H. J., Fleischer, K., Rebel, K. T., and Dekker, S. C. (2013). Vegetation-mediated feedback in water, carbon, nitrogen and phosphorus cycles. Landsc. Ecol. 28 (4), 599–614. doi:10.1007/s10980-012-9843-z
Yuan, Z. Q., Fang, C., Zhang, R., Li, F. M., Javaid, M. M., and Janssens, I. A. (2019). Topographic influences on soil properties and aboveground biomass in lucerne-rich vegetation in a semi-arid environment. Geoderma 344, 137–143. doi:10.1016/j.geoderma.2019.03.003
Zhang, J. T., Li, X. J., Liu, Z., Li, X. Y., Gao, Z. W., and Mu, C. S. (2014). Growth forms of Leymus chinesis (P oaceae) at the different developmental stages of the natural population. Plant Species Biol. 29 (3), 263–271. doi:10.1111/1442-1984.12023
Zhang, W. P., Fornara, D., Liu, G. C., Peñuelas, J., Sardans, J., Sun, J. H., et al. (2022). Interspecific interactions affect N and P uptake rather than N: P ratios of plant species: Evidence from intercropping. J. Plant Ecol. 15 (2), 223–236. doi:10.1093/jpe/rtab084
Zhao, X., Zhu, H., Dong, K., and Li, D. (2017). Plant community and succession in lowland grasslands under saline–alkali conditions with grazing exclusion. Agron. J. 109 (5), 2428–2437. doi:10.2134/agronj2016.12.0734
Zhao, Y., Yang, B., Li, M., Xiao, R., Rao, K., Wang, J., et al. (2019). Community composition, structure and productivity in response to nitrogen and phosphorus additions in a temperate meadow. Sci. Total Environ. 654, 863–871. doi:10.1016/j.scitotenv.2018.11.155
Zhongming, W., Lees, B. G., Feng, J., Wanning, L., and Haijing, S. (2010). Stratified vegetation cover index: A new way to assess vegetation impact on soil erosion. Catena 83 (1), 87–93. doi:10.1016/j.catena.2010.07.006
Keywords: grassland, edge effect, shrub patch, fine-scale, plant-soil feedback
Citation: Wang N, Jing Y, Ren X, Qiao H, Zhang H, Dang X and Meng Z (2022) Fine-scale analysis of edge effect of shrub patch in different grassland types. Front. Environ. Sci. 10:971598. doi: 10.3389/fenvs.2022.971598
Received: 17 June 2022; Accepted: 06 September 2022;
Published: 28 September 2022.
Edited by:
Arnaldo Marín, University of Murcia, SpainCopyright © 2022 Wang, Jing, Ren, Qiao, Zhang, Dang and Meng. This is an open-access article distributed under the terms of the Creative Commons Attribution License (CC BY). The use, distribution or reproduction in other forums is permitted, provided the original author(s) and the copyright owner(s) are credited and that the original publication in this journal is cited, in accordance with accepted academic practice. No use, distribution or reproduction is permitted which does not comply with these terms.
*Correspondence: Zhongju Meng, bWVuZ3pob25nanVAMTI2LmNvbQ==