- Key Laboratory of Karst Dynamics, MNR/GZAR, Institute of Karst Geology, Chinese Academy of Geological Sciences, Guilin, China
In subtropical karst area, small wetlands are widely distributed owing to the well-developed karst and the high degree of groundwater-surface water interaction. However, detailed plankton community composition, spatial-temporal dynamics, and its assembly mechanism were rarely discussed. To address these, plankton distribution patterns and its relationship with hydrological conditions and environment gradients were analyzed to discover the biological characteristics of wetlands in a typical karst basin in South China. The results showed that the wetlands were diverse in types due to the hydrogeological background. Water quality evaluation according to major ions analyses showed good results, with significant differences among samples and a poor status when measured by biotic indicator such as Escherichia coli. During high and low-flow condition, a total of 163 and 162 species of phytoplankton, and a total of 62 and 36 species of zooplankton, respectively were observed. The dominant species of phytoplankton communities in low-flow condition metamorphosed from Cyclotella sp. to Navicula sp. and Cocconeis placentula; and Pediastrum simplex to Ankistrodesmus spiralis. The metamorphosis in zooplankton community was from Difflugia globulosa to Strobilidium sp. Comparing with other karst water in different climate regimes, the number of plankton species in the subtropical karst wetland was the highest, which is related to the high and stable water temperature, large karst underground spaces, and frequent interaction between groundwater and surface water. The abundance, biomass and community semblance of plankton were increased with an increase in nutrients, precipitating improvement in hydrodynamics and light intensity. This study also suggested that the plankton in karst cave wetlands in subtropical area were characterized by low diversity, attributable to the calcium-rich and weakly alkaline, but poor nutrient status of the water environment, as well as drew attention to the possible functions of wetlands in relation to the quality of connected river.
Introduction
Wetlands are vital for human survival (Xu et al., 2020), providing most of the global ecosystem services value (Costanza et al., 2014). Wetlands are considered as the “kidney of the Earth” and are very important landscape resource and ecosystem owing to their unique role in regulating the global biogeochemical cycle. About 91% of the total research on wetlands have been devoted to the driving factors of wetland change (Birch et al., 2022), ecosystem service function evaluation (Badamfirooz et al., 2021), wetland protection policy and management (Myers et al., 2013). In these studies, 29% were river wetlands, 10.8% were lake wetlands and 19.5% were compound wetlands (Xu et al., 2020). These wetlands are basically surface wetlands.
Wetlands in karst areas often have huge underground space (karst conduit/cave) or are closely associated groundwater. This kind of “half-ground and half-underground” and “half-light and half-dark” wetland is significantly different from riverine and lacustrine wetlands in terms of hydrological process, biodiversity and biogeochemical cycle (Pipan and Culver 2019). Karst wetland is rarely addressed because the complex hydrological processes are often the focus of karst research. The hydrochemistry of karst wetlands, characterized by rich calcium and bicarbonate, was usually well-known (Ford and Williams, 2007), while the biometrics were rarely involved. Durán et al. (2010) reported the hydrogeological characterisation of a karst wetland and offered an explanatory model of its origin and karstic functioning, as well as pointing to the possible causes of its historical disappearance. 17 wetlands in Pennsylvania, United States were examined the ground-water hydrology to determine the contributing area of wetland source waters (Michael et al., 2003). The studies of plankton in karst wetlands were reported mostly in Mediterranean (Jasprica et al., 2006), temperate forest (Okhapkin et al., 2022), and tropical area (Sánchez et al., 2002).
The national standard for “wetland classification” of the People’s Republic of China defined a unique wetland type as “karst cave wetland”, which means that certain water patches are recharged by groundwater where karst caves or conduits are developed (GB/T 24708-2009). According to the definition, the karst cave wetland may include karst springs, karst pools, open stream sections of underground rivers, streams, and so on. This kind of wetland is sporadic, discontinuous, variable (Lan et al., 2018), and highly dependent on hydrogeology and hydrological conditions. Research on karst cave wetland remains very limited except for several big karst wetlands in South China. A karst spring group in Guangxi has been continuously monitored for 12 years and the changes of plankton community was compared (Wei et al., 2022). The landscape of karst wetland (Huang et al., 2016), heavy metal pollution and health risk assessment (Ba et al., 2022), and the diversity of soil fauna communities (Lan et al., 2018) were the common subject of the existing case studies.
The inorganic chemical evaluation results of karst cave wetlands in the Lijiang River Basin of previous studies have been shown to be basically good (Zhang et al., 2018), but the visible water environment is nonetheless bad in some areas, notably characterized by lack of submerged plants and algae floating debris. In this case, biological factors should be considered to accurately evaluate the water environment of karst wetlands (Guo et al., 2019). Phytoplankton communities give more information on changes in water quality than mere nutrient concentrations or chlorophyll a (Chl-a) concentration. As a component of aquatic ecosystems, phytoplankton has been recognized as an indicator of trophic status and water quality of lakes (Reynolds et al., 2002; Palmer et al., 2015). Phytoplankton succession in open lakes depends on the availability of nutrients, hydraulic retention time, temperature, light intensity, and transparency (Vaulot et al., 2008). Phytoplankton communities are sensitive to changes in their environment, consequently, total biomass and many phytoplankton species are used as indicators of water quality (Reynolds et al., 2002; Brettum and Andersen 2005; Reynolds 2006). Considering specific biogeochemical processes in karst wetlands, we hypothesized that the plankton communities are unique and are sensitive to the environmental changes.
This paper was aimed at studying the plankton community structure and diversity of selected karst cave wetlands in Lijiang River basin. The plankton distribution patterns and their correlations with hydrological dynamics and hydrochemistry were explored, in order to determine the specific type of plankton that flourishes in the wetlands under the special environment of karst groundwater recharged with karst cave, and to provide data on the possible effect of karst cave wetlands on the Lijiang River.
Methods and materials
Study area
Guilin, a city located in Southern China, is famous for its glorious karst landform. It has become one of the world’s prominent tourist destinations and was listed as a world natural heritage site in 2014. Lijiang River (LJ), the largest river in Guilin, runs through the city from south to north. It is a famous scenic spot and an important drinking water source in Guilin City (Shahab et al., 2020). Being one of the tributaries of the Pearl River, Lijiang River is approximately 164 km in length (Figure 1). The catchment area of the Lijiang River comprises the section from Xing’an County to Yangshuo County, with a total basin area of 5,585 km2, 49% of which are carbonate area (Zhao 2018). In addition to Huixian, the largest wetlands in the basin, numerous scattered wetlands composed of many karst springs and pools distribute in the basin, which played a critical role in regulating the water volume of the Lijiang River and purifying the water quality. However, at present, research on karst wetlands in the basin is rarely given due attention.
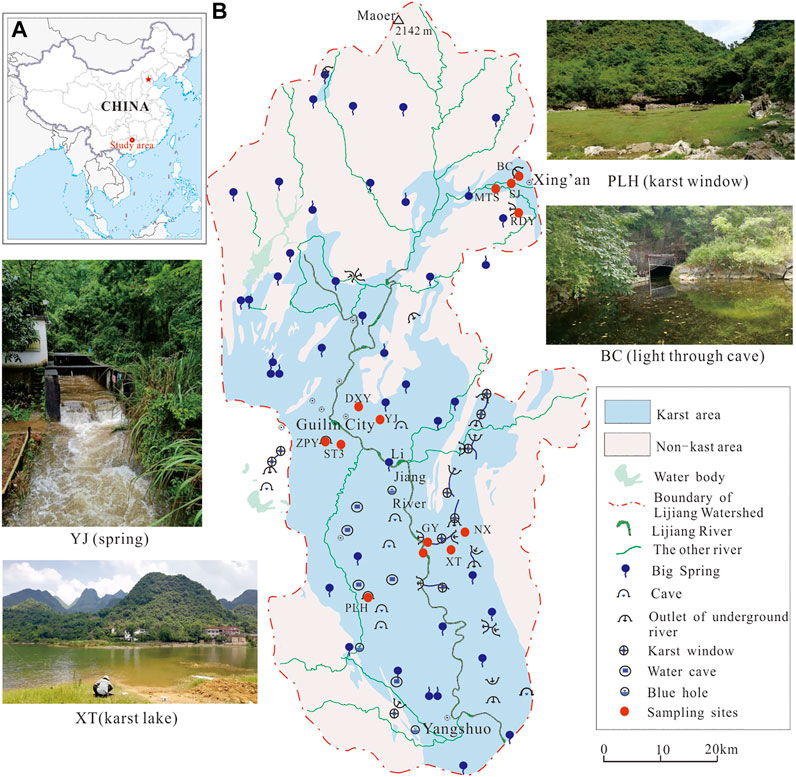
FIGURE 1. (A) Location of the study area; (B) The simple hydrogeology map of Lijiang River watershed. The red circles denote the locations of sampling sites. Four pictures show the features of typical wetlands in the watershed.
The strata exposed in the Lijiang River Basin are briefly distributed as follows: the northern part is composed of Caledonian Granite and Paleozoic strata such as Sinian, Cambrian, Ordovician and Silurian; the southern part is composed of Devonian and Carboniferous limestone and clastic rock. The middle and lower reaches of Lijiang River is composed mainly of the limestone stratum of Rongxian formation and Upper Devonian of Guilin formation (Miao, 1998).
The topography of headwater and the upper reach area is hilly mountains with an elevation of more than 1,000 m. The middle and lower reaches of the basin are karst landforms, characterized by peak-cluster depressions, valleys, and peak forest plains, with elevations ranging from 100 to 500 m (a.s.l). Clastic rock mountains are located at the north edge of Lijiang River Basin, where the streams are considered as allogeneic river. Allogeneic river enters the karst area, turns into underground river through sinkhole, and becomes karst creek along Lijiang River or in the plain area. In addition, there are sinkholes (foot caves) in the dissolution plain, which form pools recharged by groundwater. The water patches with a certain dimension and significant water level variation in karst area were then identified as karst cave wetlands.
The flow rate of Lijiang River varies between 12 m3/s and 8,000 m3/s in different conditions, and the annual average discharge is about 120 m3/s (Chen et al., 2011). The climate of the basin is typically a subtropical monsoon with annual average temperature and rainfall of 19.3°C and 1,930 mm, respectively. Overall, 70% of the rainfall occurs between April and July.
Sample collection and analysis
In May and November 2020, which representing high water condition and low water condition, a total of 13 karst cave wetlands were selected for investigation and sampling. The reasons for choosing these 13 sampling sites were to: cover the edge, middle and most developed karst area in the basin including the major hydrogeology types; examine the different human activities and utilization statuses; and consider the report of previous research (Guo et al., 2021). NX, MTS, LJ are stream/rivers, and the other 10 sites are groundwater with typical karst features (Table 1; Figure 1).
Physicochemical variables, including pH, water temperature (WT), electrical conductivity (EC), pH, oxidation reduction potential (ORP), and dissolved oxygen (DO) were measured employing a Manta multi-parameter water quality instrument (Easy Probe), simultaneously with plankton sampling. The precisions of them are ±0.10 units, ±0.10°C, ±1.0 μs/cm, ±20 mv, ± 0.1 mg/L, respectively. Ca2+ and HCO3− were titrated on site using a Calcium Test kit and a Biocarbonate Test kit (Merck KGaA, Germany), with precisions of 2 mg/L and 0.1 mmol/L, respectively. Chl-a in water was measured employing Turner Cyclops-7F, with minimum detection limits of 0.025 μ g/l.
Water samples (1.5 L) were collected with a Plexiglass water collector (DSC2500, produced by Xiamen Dengxun Co. Ltd.) and stored immediately in cooling boxes before they were transported to the laboratory. The samples were analyzed to determine major ions, including K+, Na+, Ca2+, Mg2+, Cl−, SO42−, HCO3−, total nitrogen (TN), total phosphorus (TP), nitrate (NO3−), nitrite (NO2−), and ammonia (NH4+), according to the standard methods of water analysis monitoring.
5 ml of water sample was collected with sterilized plastic bottle for Escherichia coli (E. coli) concentration culture. 3M Petrifilm™ E. coli/coliform count plates (5 ml) were employed for on-site inoculation, and the plates were placed in an incubator for 24 h at a temperature of 37°C.
A plankton net (10-μm mesh) was applied for several times at a total of 6 min to collect samples at each site from 1 m depth underwater. Thereafter, each 150 ml samples were stored in two plastic bottles and sent to the laboratory for the phytoplankton (fixed with 10% Lugol’s solution) and the zooplankton (fixed with 10% formaldehyde solution) identification. Another 1,000 ml of water sample was collected into a bottle, containing 1% formaldehyde solution, and then stored in the laboratory for 48 h for quantitative phytoplankton analyses. 20,000 ml of water was collected and then filtered by the plankton net to collect 100 ml samples for quantitative zooplankton analyses. The identification of phytoplankton and zooplankton was completely followed by the trade standard of Chinese (The trade standard of the People’s Republic of China), and was referenced to ensure accurate species determination (Hu et al., 2006). The biomass of phytoplankton and zooplankton were converted by volume, and the specific gravity was taken as 1. Results were expressed as cell density of phytoplankton per liter (cells./L) and individual of zooplankton per liter (ind./L). Each cell of filamentous algae was counted as a single cell.
Statistical analysis was carried out using the Excel and SPSS 11.0 package. Relationships between the plankton species and physical-chemical variables were analyzed using CANOCO 4.5 software (Microcomputer Power, New York, United States). Before analysis, all data (except pH) were transformed by lg (x + 1) to satisfy the normal distribution.
Hydrological dynamics monitoring
Water level, depth, velocity, and the change of wetland area were surveyed each time water was sampled. Two different kinds of karst wetlands, belonging to spring and foot cave types in the Lijiang River Basin were selected to perform a 1-year hydrological monitoring. The water levels, WT, and EC were automatically recorded employing a Solinst Levelogger (Solinst Canada Ltd.), with precisions of 0.41 cm and 0.1°C, respectively. A Solinst barometer was also used for air pressure correction.
Results
Diversity of wetland type in lijiang river basin
There are many wetland classification methods for conservation and management goals (Finlayson and Valk, 1995). Wetlands can even be divided into flowing/relatively static wetlands or large/small wetland according to the speed of water renewal or the area. A total of 13 wetlands in the Lijiang River basin were classified based on the landform and hydrogeology because the character of wetland in karst area is obviously affected by topographic types and hydrogeological conditions, and the distribution of karst wetlands is closely related to the karst development and hydrogeological conditions. Firstly, according to the topographic feature, wetlands could be classified into peak-cluster and peak forest types. River was considered as a combination type. Secondly, based on hydrogeological conditions, the peak-cluster type could further be classified into four types: light through cave, spring, karst window, and karst lake. The peak forest type could further be classified into foot cave and pool. The diversity of wetlands and their hydrological character are listed in Table 2.
The particularity of karst cave wetlands includes locating in karst areas; having a certain scale of karst cave or conduit space; recharging by karst groundwater or having a close connection with karst groundwater. The schematic diagrams of three main cave wetlands are presented in Figure 2, showing the characteristic of “half-light and half-dark” and “half-surface and half -underground” (Figure 2).
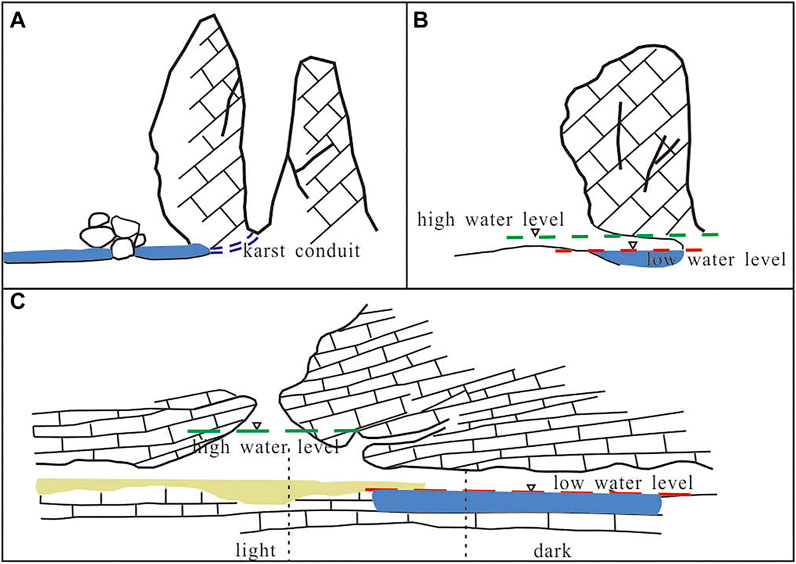
FIGURE 2. The sketch map of three main karst cave wetlands. (A) spring/subterranean river-type wetland; (B) foot-cave-type wetland; (C) karst window-type wetland.
Characteristics of physico-chemical variables and E.coli
The main environmental variables in wetlands are shown in Table 3. The results showed that the wetlands in the study area were weakly alkaline as a whole. Water temperature ranged from 18.55 to 28.21°C. The EC of wetlands ranged from 224.9 to 582.8 μs/cm, and the lowest value of EC of river was only 73.8 μs/cm in allogeneic stream. All the ORP had positive values. The DO content was between 0.86 and 11.36 mg/L. The lowest DO occurred at DXY. Ca2+ and HCO3− were the main ions. The Ca2+ content of rivers ranged from 10 to 60 mg/L, while the Ca2+ contents of wetlands were between 30 and 114 mg/L. The HCO3− content of rivers were between 50.4 and 163.8 mg/L, and the HCO3− content of other karst groundwater ranged from 94.5 to 352.8 mg/L. The values of turbidity ranged from 1.2 to 37.3 NTU, with the highest value recorded in DXY. The content of TP was basically low, with values of 0.02–0.19 mg/L. The NO3− content was between 0.09 and 9.52 mg/L and the content of NH4+ was 0.01–2.10 mg/L, with the highest value recorded in DXY. NO2− could not be detected in five samples, and the rest samples had a high value of 7.71 mg/L. Significant difference in Chl-a content among sites ranged from 0.06 to 23.4 μg/L, with the highest content recorded in DXY.
E.coli was detected in all samples in the two water sampling periods (Figure 3). The E. coli at YJ and NX, where the recharge areas were merely affected by human activities, were the lowest. DXY had the highest average values of E. coli concentration with 50 cells/mL. The sampling sites were polluted to varying degree according to the concentration of E. coli except for YJ and NX.
There was little difference in water chemical parameters among the samples. However, evaluation of biotic indicators such as E. coli and Chl-a indicated significant difference among samples. Sampling points of DXY, XT, SJ, ZPY, and ST3 were in relatively bad water environment. All the sampling points lacked submerged plants; with the E. coli in excess of 10 cells/mL.
Phytoplankton
In May, a total of 163 species of phytoplankton were identified, including 20 species of Cyanobacteria, 67 species of Chlorophyta, 43 species of Bacillariophyta, six species of Pyrrophyta, four species of Cryptophyta, 15 species of Euglenophyta, four species of Chrysophyta, four species of Xanthophyta. The density of Cyanobacteria was 48.07 × 106 cells/L, Chlorophyta was 11.97 × 106 cells/L, Bacillariophyta was 10.54 × 106 cells/L. The total density of the rest species was 1.49 × 106 cells/L. The order of biomass in each phylum was: Bacillariophyta (4.58 mg/L) > Pyrrophyta (2.99 mg/L) > Cyanobacteria (1.63 mg/L) > Euglenophyta (1.07 mg/L) > Chlorophyta (0.92 mg/L) > Chrysophyta (0.11 mg/L).
In November, a total of 162 species of phytoplankton were identified, including 14 species of Cyanobacteria, 56 species of Chlorophyta, 55 species of Bacillariophyta, six species of Pyrrophyta, five species of Cryptophyta, 21 species of Euglenophyta, five species of Chrysophyta. The cells density of Cyanobacteria was 308.10 × 106 cells/L, and Chlorophyta was 11.23 × 106 cells/L, Bacillariophyta was 5.32 × 106 cells/L. The total of the rest species was 5.65 × 106 cells/L. The order of biomass in each phylum was: Euglenophyta (13.11 mg/L) > Cyanobacteria (9.89 mg/L) > Pyrrophyta (5.62 mg/L) > Bacillariophyta (4.09 mg/L) > Chlorophyta (2.40 mg/L) > Cryptophyta (0.84 mg/L) > Chrysophyta (0.06 mg/L).
The number of phytoplankton species was always high in DXY and XT, and low in YJ, NX, and ZPY in either May or November (Figure 4). Comparatively, the density and biomass of phytoplankton were highest at XT and DXY in May and highest at XT and NX in November.
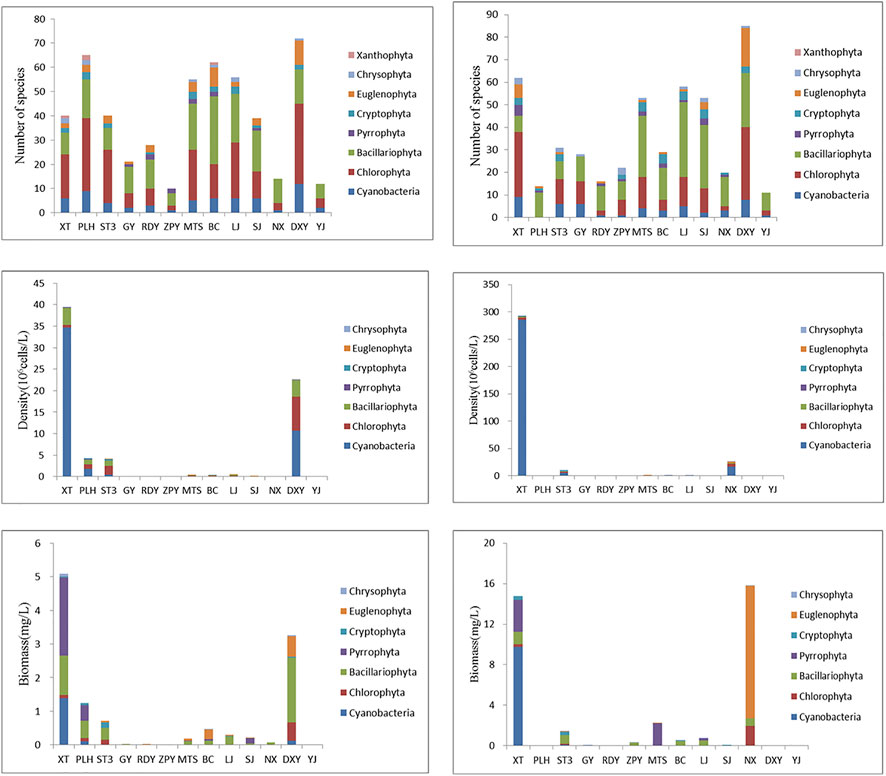
FIGURE 4. Phytoplankton community composition of each sampling sites. The left column was the results in May and the right column was results in November.
Zooplankton
In May, a total of 62 zooplankton species were observed in all samples, which consisted of 15 species protozoon, 33 species rotifers, nine species cladocerans, five species copepods. Totally 36 species of zooplankton were observed in November, which consisted of 10 species protozoon, 20 species rotifers, four species cladoserans, two species copepods.
In May, the total of zooplankton density was 1941.43 ind./L, and the order of each group was: rotifers (1,110.00 ind./L) > protozoon (810 ind./L) > copepods (19.50 ind./L) > cladocerans (1.93 ind./L). The biomass of zooplankton was 1.52 mg/L, and the order of each group was: rotifers 1.33 mg/L> copepods 0.11 mg/L> protozoon 0.04 mg/L> cladocerans 0.04 mg/L. In November, the total of zooplankton density was 1,446.60 ind./L, and the order of each group was: protozoon (720 ind./L) > rotifers (720 ind./L) > cladocerans (0.05 ind./L) > copepods (6.55 ind./L). The biomass of zooplankton was 0.95 mg/L, and the order of each group was: protozoon (0.04 mg/L) > rotifers (0.86 mg/L) > copepods (0.04 mg/L) > cladocerans (0.0 mg/L).
In May, the number of zooplankton species was the highest at PLH, followed by DXY and BC, while DXY had the highest species in November, followed by XT. The zooplankton density and biomass showed highest values at DXY in May, while XT and NX had the highest values in November (Figure 5).
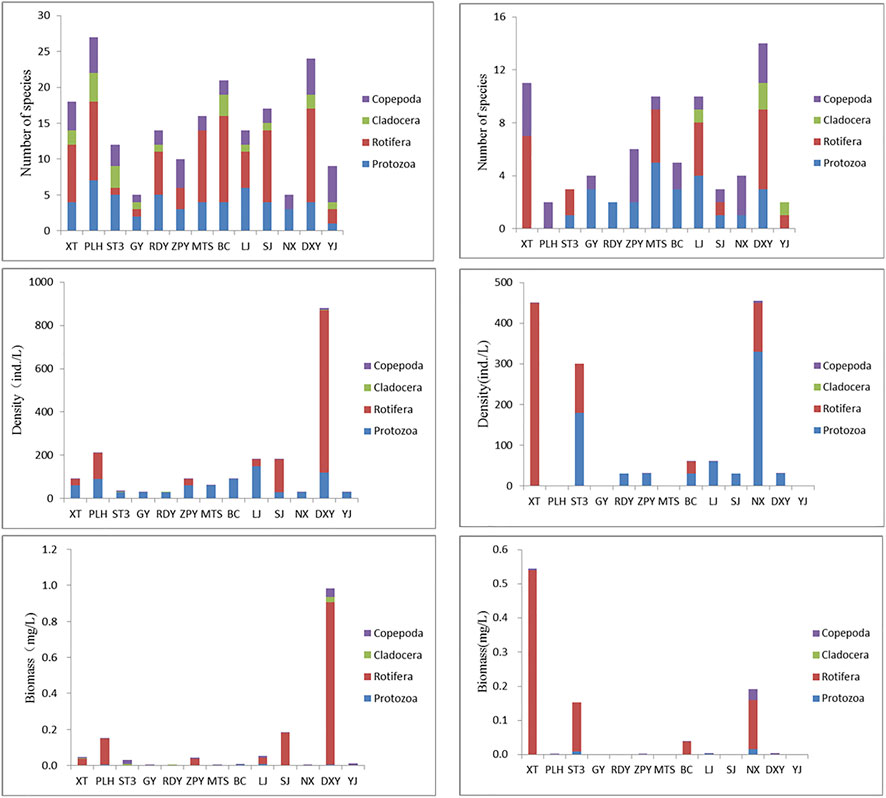
FIGURE 5. Zooplankton community composition of each sampling sites. The left column was the results in May and the right column was results in November.
Discussion
The influence of hydrogeology background and hydrological condition on plankton
Previous studies have shown that hydrological connectivity is one of the factors determining the plankton community structure (Xiao et al., 2021). The hydrological conditions were distinctly different in different wetlands’ type. For the wetland in peak-cluster depression, groundwater recharges more quickly than those in peak-forest plain. For instance, the dye tracer tests showed that the groundwater velocity was 102–104 m/d in YJ spring, while groundwater movement velocity in ZPY, which belongs to peak-forest plain in topographic state, was 10–102 m/d (Jiang et al., 2016). According to the long-term monitoring outcomes of selected groundwater in Lijiang River Basin, the annual hydrodynamics of groundwater level in spring in peak-cluster depression was between 1 and 2 m, and 1–3 m in foot cave type wetland in peak-forest plain. The variation of water level in karst window was more significant. For example, at the PLH wetland, the water level in rainy season was 7 m higher than that in dry season.
Both YJ spring and ZPY foot cave are located in the Lijiang River Basin, with a distance of only 12 km. However, YJ belongs to the karst spring system of peak-cluster depression, while ZPY belongs to the foot cave system of peak-forest plain. The response of groundwater level dynamics to rainfall was basically consistent, indicating that rainwater was the primary recharge source of groundwater in this area. The difference was due to the fact that the hydrological curve of YJ spring was steeper and sharper, and the attenuation of the groundwater level peak of ZPY was slower (Figure 6), indicating that the karst spring system in peak-cluster depression responds more drastically to rainfall and has the characteristics of rising and decline quickly, while the regulation and storage capacity of the foot cave type in peak-forest plain is stronger.
Another characteristic of karst cave wetland is that the hydrologic dynamics changes greatly. The flow difference between rainy season and dry season is significant, and the flow speed of groundwater is also different. In rainy season, the water level of wetland is high, and the flow is fast. In dry season, the water level is shallow or even dry. For instance, the discharge rate of YJ spring was 12.8 L/s on May 20 and 0.4 L/s on 13 November 2020. The water condition in May and November was considered as high-flow and low-flow, respectively due to the average discharge rate which was 1–2 L/s during the normal water flow period. The change in water level at individual sampling points led to appropriate adjustment in sampling locations. For example, groundwater only accumulated at the cave entrance of the YJ spring in November. Consequently, the sampling in November was done at the cave entrance, while the sampling in May was done at the flowing stream away from the entrance of 20 m. This factor could probably affect the community composition and biomass of plankton, culminating in the metamorphosis of dominant species in high water level period and low water level period. The predominant species were Achnanthes, Nitzschia, and Gomphonema species of Bacillariophyta in the two periods (Supplementary Table S1). The difference in Bacillariophyta metamorphosed from Cyclotella sp. to Navicula sp. and Cocconeis placentula between May and November. The dominant species of Chlorophyta metamorphosed from Pediastrum simplex to Ankistrodesmus spiralis. More importantly, Pseudoanabaena species of cyanobacteria showed up in November. Pseudoanabaena is the dominant species of cyanobacteria bloom phenomenon. It thrives in low water level and slow flowing water conditions, indicating that hydrological condition is a shaping force in structuring the plankton community in the water systems (Phlips et al., 2008).
Relationships between community composition and hydrochemistry environmental variables in karst cave wetlands
Detrended correspondence analysis (DCA) was intended to find out the relationship between environmental variables and plankton distribution. The DCA analysis results showed the eigenvalue of axis one and axis two were 0.779 and 0.392 for phytoplankton, respectively. And the first two axes contributed 25.2% of variance of phytoplankton species data and 37.9% of species-environment relation. For zooplankton, the eigenvalue of the axis one and axis two were 0.914 and 0.652, respectively. And the first two axes contributed 21.3% of variance of phytoplankton species data and 36.6% of species-environment relation.
DCA of plankton abundance and environmental factors showed that water temperature, ORP, and NO3− were the main factors influencing the abundance of phytoplankton, followed by NH4+, NO2−, DO, and turbidity (Figure 7). Most of the phytoplankton species were controlled by water temperature, and only a few species were controlled by ORP, NO3−, and turbidity. Water temperature, pH, turbidity and NO2− were the main factors that affected the abundance of zooplankton, followed by NO3− and COD. Most of the zooplankton species were closed related to water temperature, turbidity and NO2−, and only a few species were controlled by water temperature and pH. Furthermore, there were some other species which did not correlate with these environmental variables.
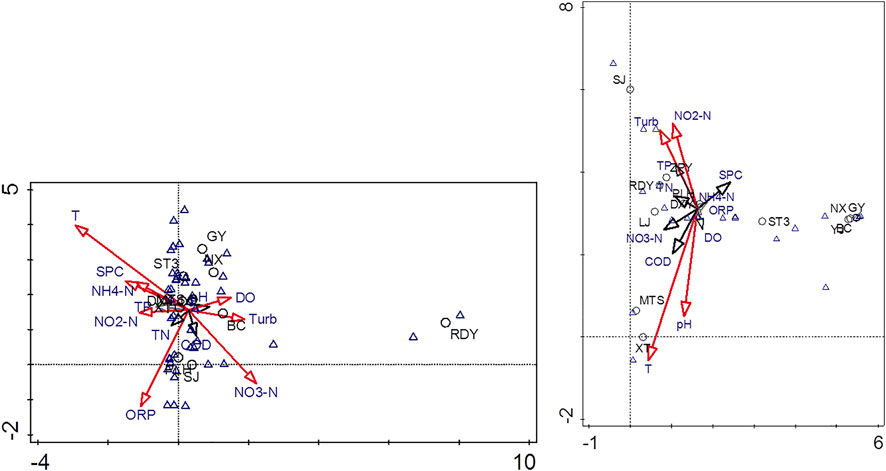
FIGURE 7. The ordination of DCA between environmental factors and plankton species (left: phytoplankton; right: zooplankton) in the Lijiang River watershed.
For wetlands recharged by karst groundwater, there was little difference between the water temperature inside the aquifer and the groundwater outlet. The temperature was relatively stable, mostly distributed between 17 and 19°C in the study area. In theory, therefore, water temperature should not be a restriction factor for the plankton species, as has been showed in other wetlands (Sun and Wang, 2021). However, the measured water temperature ranged from 18.55 to 28.21°C, which was due to the transformation of water bodies/pools of different sizes after groundwater outflow. The rising range of water temperature was different due to the effect of many factors such as light, depth of water patches and so on (Jin et al., 2022). We did not measure the exact outlet of groundwater frequently due to limitation of site conditions. Consequently, the water temperature was higher than that of the aquifer, which explains why the water temperature was also a factor controlling phytoplankton and zooplankton species.
To understand the level of plankton species or abundance in the subtropical karst wetland, numbers of plankton species in karst water under several climate types in the world were compared. It was found that the number of plankton species in the subtropical karst wetland in southern China was the highest (Table 4). This may be related to the high and stable water temperature of the subtropical karst wetland, the large karst underground spaces (dominated by karst conduits and caves), and the frequent interaction between groundwater and surface water. The species numbers or dominant species of phytoplankton have a great relationship with the water environment and nutrient elements (Phlips et al., 2008). In clean water, Bacillariophyta usually accounts for an absolute predominance (Góngora and Silveira, 2006). Although the phyla with the largest proportion of zooplankton are rotifers, the dominant species are different, which are related to the biomass of phytoplankton, groundwater flow, and other environmental conditions.
Based on the relationship between sample distribution and phytoplankton species, NX and SJ were grouped as one type. BC and RDY were grouped into two separate types, and the rest could be grouped into one type. For zooplankton, the relationship between sample distribution and zooplankton species were similar in LJ, MTS, XT, which could be grouped into one type. ST3, BC, NX, GY were grouped into another type and the rest sampling were grouped into other types. From the classification results, it was found that environmental factors did affect the community structure of plankton. Among the factors, plankton had the greatest relationship with turbidity, NH4+, NO2−, and these indicators reflect the impact of local land use patterns and human activities on the wetland water environment. The chemical fertilizer employed in farmland farming and domestic sewage discharged were the main reasons for the deterioration in water chemical parameters. They were also the primary factors for determining whether there were submerged plants and plankton communities in the wetland. The shifting of dominant species was also associated with the water environment. The predominant species of zooplankton were nauplius and copepod larva of cyclops of copepods. The difference was that, in May, the secondary dominant species were Arcella vulgaris and Difflugia globulosa, and some rotifers species. In November, the secondary dominant species were Strobilidium sp. and Arcella megastoma of protozoon (Supplementary Table S2). The water environment factor is one of the factors leading to the change in plankton community structure since the oligotrophic water patch is the most suitable living environment for Arcella vulgaris.
The indicative significance of plankton in karst cave wetlands to the water environment of river
Non-karst area was distributed in the edge of the Lijiang River Basin, and the karst area was distributed in the middle and the southern region of the basin. Rivers from the non-karst area entered the karst area, resulting in high karst development in the middle and southern regions of the basin, forming two typical karst landscapes: peak-cluster depression and peak-forest plain. The karst formations in peak-cluster depression were sinkhole, karst spring/subterranean river, karst lake, and karst window; while karst formation in peak-forest plain were dominated by light through cave and foot cave (Figure 8). All these karst formations, along with karst conduits, were the main water storage space. The investigation showed that numerous karst cave wetlands were distributed in the Lijiang River Basin because of the well-developed karst.
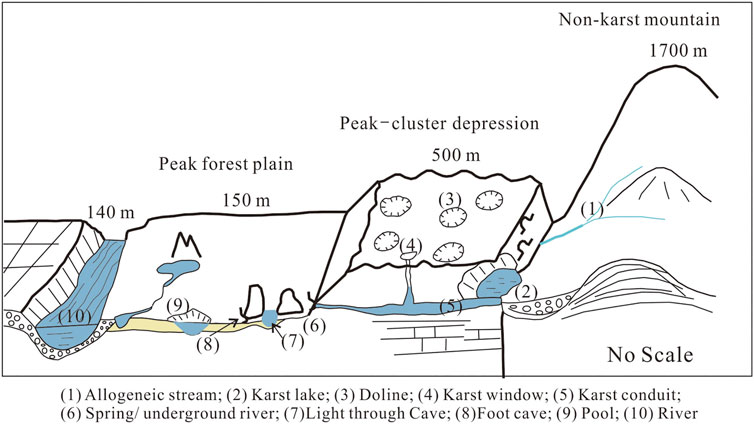
FIGURE 8. The sketch map of karst wetlands and their relationship between rivers in Lijiang River watershed.
For the 13 sampling sites, LJ and one of its primary tributaries- MTS, and NX belonged to the river type of wetlands. The other 10 sampling sites were attributed to small and dispersed wetlands. NX river originates from the mountain stream with an altitude of 1701 m, flows through 10 km to the sampling place, and then goes underground after about 2 km. One part became the source of XT Lake and the other part is the source of GY subterranean river. A total of 15 and 20 species of phytoplankton were identified in NX in May and November 2020, respectively. By way of comparison, the richness and diversity of plankton in MST were almost equivalent to those of LJ River, with higher average species of plankton compared to other wetlands. From the viewpoint of spaces, the diversity of phytoplankton was higher in the downstream of the basin than those in the edge and the middle of the river basin (Figure 9).
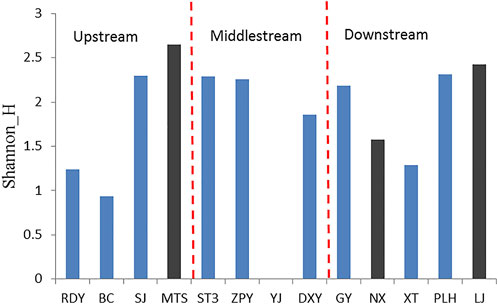
FIGURE 9. The Shannon Weiner index of phytoplankton in sampling sites. The black bars indicated the river wetland and the blue bars indicated the karst cave wetland.
From the hydrological relationship between wetland and LJ in the watershed scale, NX is a stream from the clastic rock mountain, and MTS is the primary tributary of LJ River in the plain. NX had the least number of plankton species, which may be attributed to the fact that the source of the stream is the mountainous area covered with forest, the nutrient in the stream is poor, human activities are slight, and the riverbed is cobblestone, with strong hydrodynamic force and no submerged plants. In this case, plankton abundance was low and water quality was excellent. MTS is a secondary river in the plain area. The land use type included a large area of farmland, orchards and villages. The river experienced a gradual increase in nutrients. However, due to strong hydrodynamic force, the river was also rich in submerged plants, and plankton could not bloom in large numbers. LJ is the largest river in the Guilin basin. As a world-famous scenic spot, LJ has been well protected. However, as the river flows through urban and rural areas, it inevitably receives the input of various nutrients. Lijiang River has a wide section, with large flow in rainy season and plenty of submerged plants. Consequently, it still has strong self-purification ability. The species of plankton remain at a medium level and the species diversity is high, implying a relatively healthy river.
Although the connections among these wetlands were low from the viewpoint of spaces, the water environment of wetlands would have effects on the whole aquifer or the basin on the long-run, because of the frequent groundwater—surface water interaction in the karst water system. Basically, the present condition of the water environment in karst wetlands is good, but the deterioration of the water environment caused by local pollution still exist, resulting in a large number of plankton species but low diversity, such as DXY and BC. Therefore, the impact on the aquatic ecological environment of the Lijiang River Basin needs to be evaluated through continuous monitoring of the plankton.
Conclusion
This study was carried out in diverse types of karst wetlands in Lijiang River Basin, a well-known karst scenic landscape in the world. We analyzed the relationship between plankton distribution patterns and hydrological conditions and discussed the effect of hydrochemistry variances on the plankton community structures. Abundance and biomass of phytoplankton and zooplankton were highly related to the nutrients and hydrodynamics of wetlands: highest quantities were detected at the light type through caves which showed high nutrients and weak hydrodynamics conditions; lowest amounts were detected at spring type original from karst mountain where human activities are low. Achnanthes sp., Cyclotella sp., Cocconeis placentula, Gomphonema sp. dominated the phytoplankton community, and copepod larva of cyclops and nauplius dominated the zooplankton community. High water flow favoured Cyclotella sp. and Pediastrum, while low water flow favoured Navicula and Ankistrodesmus spiralis. Arcella vulgaris and Difflugia globulosa were favored by high water flow, while Strobilidium sp. and Arcella megastoma bloomed in low water flow conditions.
This study suggests that the plankton in karst cave wetlands of subtropical area is characterized by relatively high number of species, comparing to other karst water in different climate regimes. However, it has low diversity of plankton, which can be attributed to the water environment that is rich in calcium, alkali but poor in nutrients. Moreover, the “half-surface and half-underground” wetlands, combined with limited light and low dissolved oxygen was not conducive for the growth of macrophyte, but might promote the growth of plankton. Therefore, the plankton distribution patterns in karst cave wetlands help understand the vulnerability of karst ecosystem. In addition, it is necessary to safeguard the native submerged plants to inhibit the growth of cyanobacterial phytoplankton such as pseudoanabaena.
Data availability statement
The original contributions presented in the study are included in the article/Supplementary Material, further inquiries can be directed to the corresponding author.
Author contributions
FG: Investigation, Data curation, Writing- original draft preparation, Editing. GJ: Conceptualization, Investigation, Writing-reviewing, Revision. FL: Sampling, Data curation.
Funding
This study was funded by National Key Research and Development Project of China (2019YFC0507502), Guangxi Natural Science Foundation (2020GXNSFDA238013), and National Natural Science Foundation of China (42172287 and 41977168).
Acknowledgments
Special thanks go to the three reviewers whose invaluable comments greatly improved the manuscript.
Conflict of interest
The authors declare that the research was conducted in the absence of any commercial or financial relationships that could be construed as a potential conflict of interest.
Publisher’s note
All claims expressed in this article are solely those of the authors and do not necessarily represent those of their affiliated organizations, or those of the publisher, the editors and the reviewers. Any product that may be evaluated in this article, or claim that may be made by its manufacturer, is not guaranteed or endorsed by the publisher.
Supplementary material
The Supplementary Material for this article can be found online at: https://www.frontiersin.org/articles/10.3389/fenvs.2022.970485/full#supplementary-material
References
Ba, J. J., Gao, F. F., Peng, C., and Li, J. (2022). Characteristics of nitrate and heavy metals pollution in Huixian Wetland and its health risk assessment. Alexandria Eng. J. 61 (11), 9031–9042. doi:10.1016/j.aej.2022.02.045
Badamfirooz, J., Mousazadeh, R., and Sarkheil, H. (2021). A proposed framework for economic valuation and assessment of damages cost to national wetlands ecosystem services using the benefit-transfer approach. Environ. Challenges 5, 100303. doi:10.1016/j.envc.2021.100303
Birch, W. S., Drescher, M., Pittman, J., and Rooney, C. R. (2022). Trends and predictors of wetland conversion in urbanizing environments. J. Environ. Manag. 310, 114723. doi:10.1016/j.jenvman.2022.114723
Brettum, P., and Andersen, T. (2005). The use of phytoplankton as indicators of water quality. NIVA-report SNO 4818. Oslo: Norwegian Institute for Water Research.
Chalkia, E., Zacharias, I., Thomatou, A. A., and Kehayias, G. (2012). Zooplankton dynamics in a gypsum karst lake and interrelation with the abiotic environment. Biologia 67, 151–163. doi:10.2478/s11756-011-0147-6
Chen, Q. W., Yang, Q. R., and Lin, Y. Q. (2011). Development and application of a hybrid model to analyze spatial distribution of macroinvertebrates under flow regulation in the Lijiang River. Ecol. Inf. 6, 407–413. doi:10.1016/j.ecoinf.2011.08.001
China Standards Press, (2009). National standard of the People's Republic of China: Wetland classification (GB/T 24708-2009). China: China Standards Press.
Costanza, R., Groot, de. R., Sutton, P., Ploegvan der, S., Anderson, J. S., Kubiszewski, I., et al. (2014). Changes in the global value of ecosystem services. Glob. Environ. Change 26, 152–158. doi:10.1016/j.gloenvcha.2014.04.002
Demir, N., and Kirkagac, U. M. (2005). Plankton composition and water quality in a pond of spring origin in Turkey. Limnology 6, 189–194. doi:10.1007/s10201-005-0156-7
Durán, J. J., Domingo, de G. A., and Linares, L. (2010). A disappearing wetland of karst origin: He laguna de la Alberca (ronda, málaga) in Advances in research in karst media. (Berlin, Germany: Springer).
Finlayson, C. M., and Valk, van der A. G. (1995). Wetland classification and inventory: A summary. Vegetatio 118, 185–192. doi:10.1007/bf00045199
Ford, D., and Williams, P. (2007). Karst hydrogeology and geomorphology. Chichester: John Wiley & Sons.
Góngora, Á. C., and Silveira, H. A. J. (2006). Variations of phytoplankton community structure related to water quality trends in a tropical karstic coastal zone. Mar. Pollut. Bull. 52 (1), 48–60. doi:10.1016/j.marpolbul.2005.08.006
Guo, F., Jiang, G. H., and Liu, F. (2021). Biological sulfate reduction in an epigene karst aquifer and its impact on cave environment. J. Hydrology 602, 126804. doi:10.1016/j.jhydrol.2021.126804
Guo, F., Jiang, G. H., Zhao, H. L., Polk, J., and Liu, S. (2019). Physicochemical parameters and phytoplankton as indicators of the aquatic environment in karstic springs of South China. Sci. Total Environ. 659, 74–83. doi:10.1016/j.scitotenv.2018.12.329
Hu, H., Li, R., Wei, Y., Zhu, C., Chen, J., and Shi, Z. (2006). The freshwater algae of China: Systematics, taxonomy and ecology. Beijing: Science press.
Huang, H., Dai, Y., and Sun, Y. (2016). Wetland landscape research of karst cave—To yunnan puzhehei wetland as an example. J. Shandong For. Sci. Technol. 224 (3), 99–102.
Jasprica, N., Hafner, D., CariJ, M., and Rimac, A. (2006). A preliminary investigation of phytoplankton of karstic pools (Dugi otok island, Croatia). Acta Bot. Croat. 65 (2), 181–190.
Jiang, G. H., Guo, F., Tang, Q. J., Li, X., and Zeng, X. R. (2016). Application of tracer test techniques in hydrogeological survey in karst area. J. Nanjing Univ. Nat. Sci. 52 (3), 503–511.
Jin, L., Chen, H. H., Xue, Y. Y., Soininen, J., and Yang, J. (2022). The scale-dependence of spatial distribution of reservoir plankton communities in subtropical and tropical China. Sci. Total Environ. 845, 157179. doi:10.1016/j.scitotenv.2022.157179
Lan, H. B., Ran, J. C., Yao, W. Q., Wang, W. H., Yao, Z. M., and Meng, H. L. (2018). The diversity of soil fauna communities in the karst cave wetland of maolan nature reserve. J. Landsc. Res. 10 (6), 66–70.
Miao, Z. L. (1998). The development and evolution of Lijiang River and its relations to its adjacent basins. Carsologica Sin. 17 (4), 385–391.
Michael, A., O’Driscoll, A. M., 1, Richard, R., and Parizek, R. R. (2003). The hydrologic catchment area of a chain of karst wetlands in central Pennsylvania, USA. Wetlands 23 (1), 171–179. doi:10.1672/0277-5212(2003)023[0171:thcaoa]2.0.co;2
Ministry of Agriculture of the People’s Republic of China (2010). The trade standard of the People's Republic of China: Specifications for freshwater plankton survey (SC/T-9402-2010). China: Ministry of Agriculture of the People’s Republic of China.
Myers, S. C., Clarkson, B. R., Reeves, P. N., and Clarkson, B. D. (2013). Wetland management in New Zealand: Are current approaches and policies sustaining wetland ecosystems in agricultural landscapes? Ecol. Eng. 56, 107–120. doi:10.1016/j.ecoleng.2012.12.097
Okhapkin, A., Sharagina, E., Kulizin, P., Startseva, N., and Vodeneeva, E. (2022). Phytoplankton community structure in highly-mineralized small gypsum karst lake (Russia). Microorganisms 10, 386. doi:10.3390/microorganisms10020386
Palmer, C. J. S., Hunter, D. P., Lankester, T., Hubbard, S., Spyrakos, E., Tyler, N. A., et al. (2015). Validation of Envisat MERIS algorithms for chlorophyll retrieval in a large, turbid and optically-complex shallow lake. Remote Sens. Environ. 157, 158–169. doi:10.1016/j.rse.2014.07.024
Phlips, E. J., Havens, K. E., and Lopes, M. R. l. M. (2008). Seasonal dynamics of phytoplankton in two amazon flood plain lakes of varying hydrologic connectivity to the main river channel. Fundam. Appl. Limnol. 172 (2), 99–109. doi:10.1127/1863-9135/2008/0172-0099
Pipan, T., and Culver, C. D. (2019). “Chapter 135: Wetlands in cave and karst regions,” in Encyclopedia of caves. Third Edition (Massachusetts, United States: Academic Press).
Reynolds, C. S., Huszar, V., Kruk, C., Naselli-Flores, L., and Melo, S. (2002). Towards a functional classification of the freshwater phytoplankton. J. Plankton Res. 24, 417–428. doi:10.1093/plankt/24.5.417
Sánchez, M., Alcocer, J., Escobar, E., and Lugo, A. (2002). Phytoplankton of cenotes and anchialine caves along a distance gradient from the northeastern coast of Quintana Roo, Yucatan Peninsula. Hydrobiologia 467, 79–89. doi:10.1023/a:1014936714964
Shahab, A., Zhang, H., Ullah, H., Rashid, A., Rad, S., Li, J., et al. (2020). Pollution characteristics and toxicity of potentially toxic elements in road dust of a tourist city, Guilin, China: Ecological and health risk assessment. Environ. Pollut. 266, 115419. doi:10.1016/j.envpol.2020.115419
Sun, X., and Wang, W. (2021). The impact of environmental parameters on phytoplankton functional groups in northeastern China. Ecol. Eng. 64, 106209. doi:10.1016/j.ecoleng.2021.106209
Tian, L. H., Qin, Y. Y., Tian, F., Wu, H. J., and Liang, S. C. (2014). Environ. Sci. Surv. 33 (4), 49–52.
Vaulot, D., Eikrem, W., Viprey, M., and Moreau, H. (2008). The diversity of small eukaryotic phytoplankton(≤ 3µm) in marine ecosystems. FEMS Microbiol. Rev. 32, 795–820. doi:10.1111/j.1574-6976.2008.00121.x
Wei, L. Q., Guo, F., and Jiang, G. H. (2022). Responses of plankton community to water environment changes in karst springs in Wuming Basin, Guangxi Province. J. Lake Sci. 34 (3), 777–790. doi:10.18307/2022.0307
Xiao, H., Shahab, A., Xi, B. D., Chang, Q. X., You, S. H., Li, J. Y., et al. (2021). Heavy metal pollution, ecological risk, spatial distribution, and source identification in sediments of the Lijiang River, China. Environ. Pollut. 269, 116189. doi:10.1016/j.envpol.2020.116189
Xu, X. B., Chen, M. K., Yang, G. S., Jiang, B., and Zhang, J. (2020). Wetland ecosystem services research: A critical review. Glob. Ecol. Conservation 22, e01027. doi:10.1016/j.gecco.2020.e01027
Zhang, C., Xiao, Q., Miao, Y., Guo, Y. L., Tang, Q. J., and Hao, Y. P. (2018). Day and night aqueous chemical changes and their impact on karst carbon cycle at typical monitoring sites of the Lijiang River, Guilin, Guangxi. Acta Geosci. Sin. 39 (5), 613–621.
Keywords: phytoplankton, zooplankton, hydrological conditions, nutrients, karst cave wetlands
Citation: Guo F, Jiang G and Liu F (2022) Plankton distribution patterns and the indicative significance of diverse cave wetlands in subtropical karst basin. Front. Environ. Sci. 10:970485. doi: 10.3389/fenvs.2022.970485
Received: 16 June 2022; Accepted: 27 July 2022;
Published: 25 August 2022.
Edited by:
Paul Meulenbroek, University of Natural Resources and Life Sciences Vienna, AustriaReviewed by:
Elisabeth Bondar-Kunze, Wasser Cluster Lunz, AustriaNi Maofei, Guizhou Minzu University, China
Zhi-Feng Zhang, Institute of Microbiology (CAS), China
Copyright © 2022 Guo, Jiang and Liu. This is an open-access article distributed under the terms of the Creative Commons Attribution License (CC BY). The use, distribution or reproduction in other forums is permitted, provided the original author(s) and the copyright owner(s) are credited and that the original publication in this journal is cited, in accordance with accepted academic practice. No use, distribution or reproduction is permitted which does not comply with these terms.
*Correspondence: Fang Guo, Z2ZrYXJzdEAxMjYuY29t