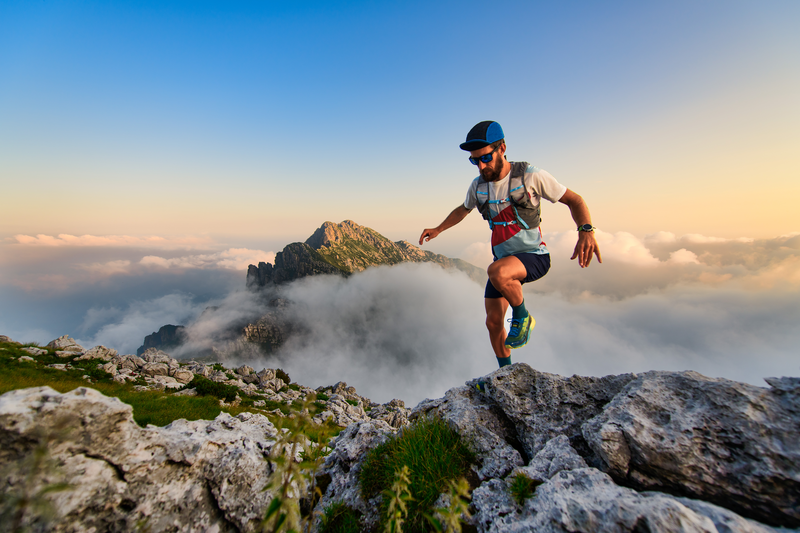
94% of researchers rate our articles as excellent or good
Learn more about the work of our research integrity team to safeguard the quality of each article we publish.
Find out more
REVIEW article
Front. Environ. Sci. , 06 September 2022
Sec. Freshwater Science
Volume 10 - 2022 | https://doi.org/10.3389/fenvs.2022.968546
This article is part of the Research Topic Interactions between Groundwater and Human Communities: Perspectives on the Resources, Environments, Threats and Sustainable Development View all 18 articles
Heavy metal pollution of groundwater has a serious impact on human health, which has become a key issue in the field of water resources and water environment. Permeable reactive barrier (PRB) is an in-situ remediation technology for passive groundwater pollution, with the advantages of economy and efficiency. The reaction material in the PRB wall is the key to the whole system, which can intercept or degrade heavy metals to achieve the removal of pollutants. Among a wide variety of reaction materials, PRB has been successfully used to remove various heavy metal contaminants. In this paper, reaction materials are classified into three broad categories, namely single material, mixed material, and composite material. On this basis, the action mechanism of various materials to remove heavy metal pollutants is analyzed, and the screening method of reaction medium is summarized. Finally, the deficiencies and development directions of PRB reaction medium materials in the removal of heavy metals in groundwater are pointed out, providing reference for the subsequent development and application of PRB reaction medium.
Approximately 2.5 billion people depend directly on groundwater for drinking water on a global scale (Li et al., 2021). In addition, more than half of agricultural irrigation water relies on groundwater (Famiglietti, 2014). In this case, the excessive consumption of groundwater makes it a research hotspot in this field (Jarvis, 2021). In general, heavy metals refer to metals and metalloids with a density greater than 5 g cm−3 or an atomic number greater than 20 in the periodic table, including cadmium, mercury, copper, arsenic, chromium, etc., (Barceló and Poschenrieder, 1990). Sources of heavy metals in groundwater include natural and anthropogenic sources (Hashim et al., 2011). Heavy metals widely present in the earth’s crust can dissolve in groundwater through natural weathering, erosion, or changes in soil pH value (Fedoročková et al., 2021). Moreover, landfill leachate, sewage, mine tailings leachate, deep well treatment liquid waste, industrial waste seepage or industrial leakage may all cause elevated concentrations of heavy metals in groundwater (Ali et al., 2019). Since heavy metals cannot be degraded, their continuous accumulation poses a threat to drinking water safety and human and animal health (Mukherjee et al., 2020). Long-term direct or indirect exposure to heavy metal-contaminated groundwater may lead to the accumulation of heavy metals in various organs, leading to diseases such as osteoporosis, respiratory diseases, skin problems, cardiovascular, and cerebrovascular diseases, and reproductive diseases (Khan et al., 2013). For example, cadmium can cause symptoms such as acute respiratory failure in humans. cadmium, lead, and mercury can damage the kidneys of human body (Salman et al., 2011). As a hidden water source, the discovery of groundwater pollution is delayed (MacDonald and Kavanaugh, 1994).
Groundwater treatment technology is mainly divided into in-situ treatment technology and ex-situ treatment technology. PRB technology and pump-treat technology are typical representatives of groundwater in-situ and ex-situ remediation technologies, respectively. Compared with traditional pump-treat technology, PRB technology relies on underground active reaction medium materials to remove dissolved pollutants in polluted water, which neither requires additional power systems nor damages natural environments such as soil and groundwater. Therefore, it has the advantages of simple operation, reasonable cost and ideal processing effect, which is considered as a promising processing technology (Obiri-Nyarko et al., 2014). However, PRB technology also has some disadvantages. For example, when the media material loses activity or becomes blocked due to precipitation, the media material should be replaced in time, which makes the cost difficult to quantify (Wilkin et al., 2009). During operation, treatment effects need to be closely monitored to ensure PRB effectiveness (Faisal et al., 2018). Moreover, PPR technology is greatly affected by geological conditions and site characteristics (Obiri-Nyarko et al., 2014). As shown in Figure 1, PRB technology is to build a reactive wall in the vertical direction of the groundwater pollution plume, and fill the wall with reactive substances. When the plume flows through the wall, the pollutants undergo a series of physical, chemical or biological reactions with the active reactive substance, thereby intercepting or converting the pollutants into non-toxic substances. According to reports, there have been more than 200 engineering application cases of PRB technology to deal with groundwater pollution around the world.
FIGURE 1. Working principle of PRB technology (Day et al., 1999).
The key to PRB technology to deal with groundwater pollution is the active medium material filled in the wall. In more detail, the reactivity, cost, and lifetime of materials can have a direct impact on the stability, economy, and safety of PRB systems. The choice of reaction medium material mainly depends on the reaction mechanism between pollutants and medium material. In addition, it is also affected by hydrogeological conditions such as pH. Before 2000, the medium material was traditional zero-valent iron (ZVI). Since then, the research focus has turned to the development of new medium materials. According to the structural characteristics of medium material, there are three categories: single material, mixed material and composite material.
Under ideal conditions, the reaction medium should have the following characteristics. 1) The filling material should have stable structure and uniform particle size. In addition, the particle size should not be too small to ensure that the permeability coefficient is higher than the surrounding area. 2) The pollution component can fully react with the filler and be removed. 3) The filling material should be stable and active enough to ensure that the PRB system has a long-term stable treatment effect on pollutants (Asokbunyarat et al., 2017). 4) The product after the interaction between the reaction material and the pollution component should be non-toxic and free from secondary pollution (Jeen et al., 2011). 5) The reaction product is not easy to block the system (Blowes et al., 2000). 6) The filling material is cheap and readily available (Liu et al., 2020).
Scherer et al. (2000) summarized the types and properties of PRB reaction materials, and discussed the feasibility and long-term efficacy of PRB technology. However, some new materials have been developed and applied within the last 20 years (Obiri-Nyarko et al., 2014) reported the application of ZVI, zeolite, activated carbon, apatite and other materials in groundwater remediation, without introducing the application of mixed materials and composite materials (Wu et al., 2020) detailed the application of ZVI as a reactive material in the treatment of heavy metal pollution in groundwater (Song J. et al., 2021) reviewed the PRB technique for remediation of heavy metal-contaminated media materials, but did not indicate the process for screening reactive materials. In this study, reaction materials are classified into three main categories, namely single material, mixed material, and composite material. The research status of various media materials in the treatment of heavy metal pollution in groundwater is summarized, including the types of heavy metals, experimental scale and removal effect. On this basis, the main mechanism of heavy metal removal by reaction materials is revealed and the experimental methods for screening dielectric materials are summarized. Finally, the development direction of reaction materials is pointed out.
With strong electronegativity, high reactivity and fast reaction, ZVI has a good removal effect on heavy metals such as Cr, U, V, Ni, Pb, Cd, Hg, and Cu. ZVI has been used in many practical projects. ZVI easily reacts with oxygen to form FeO(OH) or Fe(OH)3 solids, thus causing the clogging of the reaction medium. Usually, highly permeable substances (such as sand and gravel) are added to the reaction wall to increase the permeability and prolong the service life (Jeen et al., 2011). As shown in Figure 2, the main mechanism of ZVI removal of heavy metals lies in redox, precipitation, adsorption, etc. With the removal of Cr(VI) by ZVI as an example, the reaction equation is as follows (Dries et al., 2005; Lai and Lo, 2008; Soto et al., 2015):
FIGURE 2. Schematic illustration of reaction mechanism of ZVI [modified from (Fu et al., 2014)].
ZVI may have bio-stimulatory effects on the PRB system and downstream microorganisms (Xu et al., 2017). According to (Wang et al., 2022), ZVI promotes the biological reduction of Cr(VI).
Compared with ZVI, nanoscale zero-valent iron (nZVI) has smaller particle size, larger specific surface area and surface energy, one to three orders of magnitude higher reactivity (Jiang et al., 2018), and stronger transport capacity in soil. nZVI can be directly injected into underground aquifers (Mystrioti et al., 2020), which has good application prospects. However, the high reactivity makes nZVI susceptible to oxidation, the magnetic properties make it easy to agglomerate and cause clogging. The modification of nZVI is an important research direction to develop new reaction medium.
A large number of hydroxyl, phenol and carboxyl groups are distributed on the surface of activated carbon (AC), and the internal pores are well developed (Li et al., 2010). Therefore, activated carbon has excellent adsorption and chemical stability (Dong et al., 2016), which is a commonly used material in the early stage of PRB. Moreover, it is widely used in the removal of heavy metals (Hornig et al., 2008). However, the heavy metal removal effect of activated carbon is easily affected by pH (Di Natale et al., 2007). The increase in pH would enhance the binding of water molecules to activated carbon and decrease the adsorption capacity of activated carbon (Obiri-Nyarko et al., 2014). The groundwater component affects the performance of activated carbon. For example, anions such as chloride and sulfate will compete with pollutants for reaction sites, thereby reducing the adsorption capacity of activated carbon (Namasivayam and Sangeetha, 2006).
Another type of carbonaceous material is biochar (BC), with abundant internal pores and negative charges on the surface (Lou et al., 2016). This is prepared from various types of biowaste materials under low pyrolysis temperature and anoxic conditions (Liang et al., 2021). Compared with AC, BC is cheaper to produce and has a stronger affinity. However, the adsorption capacity of BC for Ni, Pb, Cr, and Cd is lower than that of modified AC (Wilson et al., 2006). By loading organic functional groups (Zhou et al., 2013), minerals (Han Y. et al., 2016) and nZVI (Zhou et al., 2014) or activated by acid and alkali solutions, the adsorption capacity of BC can be significantly improved. The heavy metal removal mechanisms include physical adsorption, ion exchange, electrostatic interaction, etc. Typically, the entire removal process is a combination of different mechanisms (Shakoor et al., 2020). The materials used in the preparation of BC are of various types and sources, which have important application value in the restoration of heavy metals in groundwater.
Iron-bearing minerals have become a new type of reaction material for PRB systems, including iron sulfide mineral and iron oxide minerals (Aredes et al., 2012). The removal mechanism of heavy metal ions by natural iron oxide minerals is mainly (irreversible) chemisorption, and to a lesser extent (reversible) physisorption. As a good arsenic removal material, laterite can stably adsorb arsenic in water in the pH range of 4–11 (Aredes et al., 2012). Due to their abundant reserves, low price, and non-toxicity to the environment, iron sulfide mineral is usually used to remove heavy metals in polluted water (Abdul et al., 2019). The effect of groundwater composition on removal efficiency should be fully considered. When humic acid (HA) is contained in, for example, groundwater, HA competes with Cr(VI) for pyrite surface sites, resulting in the reduction of Cr(VI) removal (Kantar et al., 2017). In addition, the heat treatment method can also affect the removal effect of pyrite on heavy metal contaminants. The adsorption capacity of Hg(II) by natural pyrite is 0.19 mg g−1, which could be increased to 54.44 mg g−1 by calcination under nitrogen atmosphere (Zhu et al., 2020). Under acidic conditions, the removal mechanism of Cr(VI) by pyrite is as follows (Gong et al., 2016):
Minerals such as zeolite and apatite have channel or lamellar (chain lamellar) structure inside, with good adsorption performance and ion exchange performance, which can remove a variety of heavy metal ions (Kumara and Kawamoto, 2021). Zeolite is a hydrated framework-like aluminosilicate mineral composed of tetrahedra of AlO4 and SiO4 (Maharana and Sen, 2021), with abundant internal channels (Bailey et al., 1999), as well as catalytic ability and molecular sieving ability (Cerjan Stefanović et al., 2007). It has a negative surface charge (Abdelrahman et al., 2021), and a cation exchange capacity of 2–4 meg g−1 (Liu et al., 2020). With strong adsorption on Cu(II), Pb(II), Cd(II), Ni(II), and Zn(II), and low price, zeolite is considered to be an ideal material for heavy metals removal (Wang and Peng, 2010). At low pH values, an excess of protons can compete effectively with the heavy metal ions for binding sites on zeolite surface (Faisal and Hmood, 2015). The higher the degree of groundwater mineralization, the better the removal efficiency of heavy metal ions by zeolite (Jarvis et al., 2006). Heavy metal cation contaminants can be adsorbed or exchanged into the internal channel structure of channel/lamellar (chain lamellar) structured minerals. Therefore, the voids between the medium material particles are not blocked, thereby maintaining high permeability and extending service life. This feature is of great significance in the practical application of PRB engineering.
Because of its wide source, non-toxic, cheap, and simple preparation process, apatite, a common phosphate material, is often used to remove divalent heavy metal ions. Dong et al. (2013), which is considered as a high-quality inorganic ion lattice adsorption and exchange material. When the environmental pH is neutral or weakly acidic, hydroxyl apatite (HAP) can remove heavy metal contaminants through surface adsorption and ion exchange. When the environmental pH is less than 4, apatite removes heavy metal pollutants through dissolution-precipitation reactions and other pathways (Oliva et al., 2010; Elkady et al., 2018; Nijhawan et al., 2018).
Due to the abundant channels, synthetic HAP is often used in the removal of target pollutants (Asri et al., 2010). HAP is the main inorganic component of animal bones, and the content can reach 70%. Therefore, HAP obtained from discarded animal bones has a cost advantage (Ngueagni et al., 2020). Studies have shown that HAP prepared from animal bones such as fish bones and bovine bones are mostly used for remediation of heavy metals in industrial wastewater and soil (Hernández-Cocoletzi et al., 2020; Wang et al., 2021). It is necessary to research biological HAP as PRB reaction materials. HAP can form stable minerals with metal cations, with high stability under oxidative or reducing conditions (Wellman et al., 2008). Without considering the coexistence of other anions and cations, the reaction between HAP and uranium has the following three main mechanisms: 1) Dissolution and precipitation. Under acidic conditions, hydroxyapatite dissolves part of Ca2+ and PO43−, and precipitate with UO22+. New species are formed, such as Ca [(UO2) (PO4)]2·nH2O and H2 [(UO2) (PO4)]2·nH2O (Kong et al., 2019). 2) Ion exchange. Divalent metal ions in solution are prone to ion exchange reaction with hydroxyapatite. Calcium sites on the surface of HAP can ion-exchange with uranium ions (Stötzel et al., 2009). 3) Adsorption. UO22+ achieves the adsorption of uranium by occupying surface active sites on hydroxyapatite (Simon et al., 2008).
Permeable concrete is a highly porous special concrete prepared from cement slurry and coarse aggregate (particle size greater than 4.75 mm) (Alighardashi, 2018; Wijeyawardana et al., 2022). The innovative development of mineral materials is to act as a reactive barrier to remove heavy metals and nitrates in groundwater (Shabalala et al., 2017; Alighardashi, 2018). The heavy metals removal mechanism of permeable concrete is mainly to generate metal hydroxide precipitation (Holmes et al., 2017). In addition, microbial action and adsorption also facilitate the removal of heavy metals (Yousefi and Matavos-Aramyan, 2018).
Ion exchange resin has the characteristics of high adsorption capacity, long-lasting activity and fast reaction rate, which is often used for the removal of heavy metal ions in sewage (Vilensky et al., 2002; Ahmad and Sharma, 2019). Through continuous research on resin modification, selective adsorption and regeneration are significantly improved, which increases the possibility of application in groundwater remediation technology. As a promising PRB reaction medium, ion exchange resin is often used to treat uranium in groundwater, where the treatment process is not easily disturbed by groundwater components (Stucker et al., 2011; Zawierucha and Nowik-Zajac, 2019). With PHREEQE geochemical software (Barton et al., 2004), predicted the existence of uranium in groundwater under different pH conditions. On this basis, they proposed that H+ on the cation exchange resin can be replaced by cations (Na+, K+, Ca2+, and Mg2+) in groundwater, thereby reducing the pH of groundwater and promoting the formation of UO22+ and adsorption on the resin. However, the main removal mechanism of uranium by anion resins is electrostatic action. However, ion exchange resins are not suitable for treating wastewater with excessively high concentrations of pollutants. Expensive price is the main reason to limit its application. In this regard, the development of inexpensive ion exchange resins becomes an important research direction.
In recent years, solid waste have been used in the reaction medium, which plays a role in reducing the hazard of heavy metals in groundwater, as well as the cost of solid waste treatment and PRB operation. Solid waste such as waste sludge (Chien et al., 2021), fly ash (Yang et al., 2020), and recycled concrete (Indraratna et al., 2010) etc., has become a popular research object for the development of new reaction media. Solid waste in line with the concept of sustainable development is selected as the medium material. However, the composition of solid waste is complex, which may increase the risk of secondary pollution. For the development of solid waste reaction media, the removal capacity and removal mechanism of the material, and the stability of the generated product are important concerns (Faisal et al., 2018). Basic oxygen furnace sludge (BOFS) is rich in ZVI and iron oxides such as FeO and Fe3O4 (Omran et al., 2020). BOFS has small particle size, large specific surface area, and positive charge on the surface, which is conducive to the adsorption of CrO42− and Cr2O72− in groundwater (Frade et al., 2018). In addition, co-precipitation can occur between Cr3+and Fe3+ (Blowes et al., 2000). Therefore, the removal mechanisms of Cr(VI) in groundwater by BOFS are mainly adsorption, reduction and co-precipitation. The possible reaction processes are as follows (Wilkin and Puls, 2003; Puls, 2006; Han C. et al., 2016):
For example, (Frade et al., 2018), found that steelmaking converter sludge is rich in iron oxides such as ZVI and FeO. At pH 5.5, its adsorption capacity for Cr (Ⅵ) is 0.213 mg g−1. Calcium content in BOFS is up to 13.9% (w/w). Calcium can promote the release of OH− the increase of pH, and the precipitation of Cr(OH)3. However, high pH is not conducive to the reduction of Cr(VI). In addition (Araujo et al., 2019), also confirmed that BOFS and ZVI have similar removal effects on high concentrations of arsenic in acidic wastewater.
The advantages and disadvantages of single reaction medium used within PRB have been summed up in Table 1.
TABLE 1. Analysis of the advantages and disadvantages of single reaction medium to remove heavy metals.
Although there are many types of single media and are widely used in groundwater heavy metal pollution control, it is difficult to meet complex environmental conditions. Two or more of ZVI, mineral material and carbonaceous material are mixed in a certain proportion to form the mixed material, which can enhance the penetration ability of the reaction medium, the type of removal mechanism, the removal rate and the service life.
When ZVI is used as a single active substance, it is easy to cause passivation and blockage. The mixing of ZVI and mineral material can not only increase the permeability coefficient of the PRB system, but also effectively reduce the aggregation of ZVI. When ZVI is mixed with inert mineral materials such as sand and gravel, the permeability coefficient inside the PRB system is improved. In the study by (Wang et al., 2022), a mixed material consisting of ZVI (20%), gravel (20%), and sand (60%) was treated as a PRB filler for Cr(VI). At this time, the concentration of Cr(VI) can be reduced from 27.29–242.65 mg L−1 to below 0.1 mg L−1. In addition, this study also found that ZVI could induce changes in the structure and composition of the downstream native microbial community, thereby promoting the biological reduction of Cr(VI).
Pumice and trass have the advantages of abundant channels and large specific surface area, which are often used as adsorbents or filter media for sewage treatment. The mixing of ZVI with porous minerals not only increases the permeability coefficient, but also solves the blocking problem of PRB walls. According to the study of (Faisal et al., 2020), when the initial concentration of nickel is 8 mg L−1, the life expectancy of the PRB system with ZVI/pumice mixed material as the reaction medium is more than 26 months, while the life expectancy of the PRB system with only ZVI as the reaction medium is only 3.6 months. In addition, the pyrite/ZVI mixed material as a reaction medium can inhibit the increase of pH, promote the regeneration of Fe(II), and prolong the service life (Lü et al., 2018).
Subterranean environment is rich in microorganisms. Bioremediation is an important technology for remediation of soil and groundwater. Compost materials have attracted much attention due to their rich microbial species and high cation exchange capacity (Cancelo-González et al., 2017). Most heavy metal sulfides have low solubility. Sulfate reduction is often used to solve the problem of heavy metal pollution in mining wastewater. This provides a basis for the application of sulfate reducing bacteria (SRB) in PRB technology, and its removal mechanism of heavy metals is shown in Figure 3 (Wilopo et al., 2008) used ZVI, sheep manure, compost and sawdust as fillers to study the removal of arsenic and manganese by PRB. Inoculated SRB may promote the precipitation of part of arsenic in the form of Fe-As-S, but it is not conducive to the solidification of manganese. Inoculation with SRB increases the lifespan of PRB to 15.76 years, which is much higher than that of PRB without SRB (6.74 years). Appropriate pH is a necessary condition for microbial growth. Good permeability is conducive to the normal operation of the PRB system (Gibert et al., 2013) constructed a field-scale PRB system with calcite, plant compost, ZVI, and sludge as fillers, with over 98% heavy metal removal. However, the complex media composition easily leads to the inhomogeneity of the filling material, which can produce clogging and preferential flow channel phenomena. The particle size distribution of the filling material is adjusted to improve this by adding inert materials such as sand and stones.
FIGURE 3. Removal mechanism of heavy metal (loid)s by reaction materials containing ZVI and SRB [modified from (Xu and Chen, 2020; Song J. et al., 2021)].
The growth of microorganisms requires a suitable growth environment. Therefore, suitable pH range and temperature and sufficient nutrients are beneficial to enhance the microbial action in the PRB system. Moreover, the toxic effects of the constituents of the pollutants on microorganisms need to be taken into account.
The cost of ZVI-containing fillers is relatively high. In order to improve the economics of PRB technology, solid wastes from agricultural or industrial production processes can be used as precursors to prepare PRB reaction medium (Faisal et al., 2020) mixed waste foundry sand (WFS) with a mass ratio of 82% and filter sand (FS) with a mass ratio of 18% as a reaction medium to treat wastewater containing lead and nickel. Among them, the removal mechanism of the former is mainly chemical adsorption, with the maximum adsorption capacities of 13.966 and 4.227 mg g−1. In order to reduce the secondary pollution, more and more attention has been paid to the research and development of environmentally friendly materials (Fan et al., 2018) developed a new type of environmentally friendly filler, with corn straw, fly ash, synthetic zeolite, loess ferromanganese nodules and other mixed materials as active media. Its removal mechanism is mainly complexation and electrostatic attraction, with greater than 90% removal rate of Pb(II) and Cd(II).
In contrast, the preparation of mixed material is simpler than that of composite material. The key step in the preparation of mixed material lies in the selection and addition of a variety of single materials. As shown in Table 2, there are differences in the heavy metal treatment capacity and removal mechanism of the reaction medium composed of different types of materials. The selection of active materials should fully consider the removal mechanism of target pollutants. In order to reasonably construct the reaction medium of the PRB, it is necessary to take into account the environmental conditions such as groundwater component and permeability of the surrounding soil.
The ZVI surface is plated with another metal with high reduction potential (such as copper, platinum, and nickel) to form bimetallic material, which act as galvanic cells during the reaction to speed up the reaction rate. The bimetallic system composed of nZVI can reduce the agglomeration between nZVI and the activation energy during the reaction, thereby increasing the reduction rate (Maamoun et al., 2021). Shubair et al. (2018) demonstrated that the removal mechanism of cesium by nZVI and nZVI/Cu is mainly chemical adsorption, with a removal rate of more than 99%. In contrast, the reaction rate of nZVI/Cu is faster (Xie et al., 2021) synthesized nZVI/Pt bimetallic particles, which showed good removal of heavy metals and chlorophenols (Zhu et al., 2017) demonstrated that nZVI/Ni bimetallic material can reduce the effect of agglomeration, with 99% Cr(VI) removal rate. Copper can promote the electron transfer of Fe0 and the generation of Fe2+, thereby accelerating Cr(VI) removal. With the remove of Cr(VI) by the bimetallic system composed of nZVI/Cu as an example, the chemical reaction formula is as follows (Maamoun et al., 2021):
Moreover, copper can inhibit the oxidation of Fe0 and increase the electrons obtained by Cr(VI). As shown in Table 3, The research on removing heavy metals from groundwater with ZVI bimetal as reaction media is still in the laboratory stage. It has extensive removal of heavy metals, and its removal mechanism mainly consists of adsorption and reduction. Compared with ZVI and nZVI, bimetallic particles have a faster reaction rate and overcome the disadvantage of easy agglomeration. However, its preparation process is complicated. In addition, some metals are expensive (such as silver and platinum) or cause secondary pollution to the environment (copper and nickel). These factors above limit the application of bimetallic materials.
In order to overcome the shortcoming of easy agglomeration in nZVI, a series of ZVI-loaded solid materials were developed. Reported loaded materials include pyrite (Lü et al., 2018), zeolite (Tasharrofi et al., 2020), bentonite (Zhang et al., 2012), clay (Jia et al., 2011), montmorillonite (Jia and Wang, 2013), activated carbon (Wang M. et al., 2019), biochar (Liu Y. et al., 2019), and graphene (Xing et al., 2016). The application of ZVI-loaded material has been summed up in Table 4.
Magnetite and pyrite alone can be used as reaction medium for PRB system, or loaded material for ZVI. Magnetite is an octahedral crystal rich in Fe3O4 and having strong magnetism. Magnetite can attach nZVI to its surface, thereby effectively preventing nZVI from agglomerating. The results of (Wu et al., 2009) showed that when Fe3O4:Fe0 is 40:1, the reaction rate of composite material and Cr(VI) is the fastest. Furthermore, the reaction rate is related to the pH in the solution. Alkaline conditions are not conducive to the removal of Cr(VI). Pyrite is rich in FeS2, with strong electron transfer ability and reducibility (Min et al., 2021) prepared a ZVI-loaded pyrite composite material (ZVI/FeS2) using pyrite and ZVI as raw materials by ball milling, with over 99% Cr(VI) removal rate and long-term stability.
Silicon-containing minerals such as zeolite and clay are important heavy metal adsorbents for water. Natural zeolite has high internal porosity and surface active sites, which is suitable as a loaded material for nZVI to eliminate aggregation of nZVI (Li et al., 2018). Clay minerals such as kaolinite, illite, pyrophyllite and montmorillonite can overcome the disadvantage of easy agglomeration in nZVI (Ezzatahmadi et al., 2017). (Bhowmick et al., 2014) prepared a nZVI-loaded montmorillonite, which has a maximum adsorption capacity of 59.9 and 45.5 mg L−1 for As(III) and As(V) in a wide pH range (4–8). As(V) and As(III) form complexes on the surface of composites. As(III) is all oxidized to As(V), but As(V) is not reduced. NaOH solution (0.1 M) can be used for the regeneration of composites. After five cycles of test, the properties of composites don’t change significantly. Therefore, it is considered an ideal reaction medium for handling arsenic containing groundwater.
BC and AC are porous organic carbonaceous materials with various functional groups on their surfaces. With their large heavy metal adsorption capacity, they are often used as remediation materials for heavy metal pollution in the environment. The ZVI-containing composite material with BC and AC as loaded materials has good removal effect on arsenic, strontium, chromium, and lead (Mandal et al., 2020) prepared nZVI-loaded green tea biochar. The stabilizing effect of Pb(II) is sorted as nZVI-loaded green tea biochar > pristine nZVI > green tea biochar. The load of ZVI to BC is greater than AC. ZVI affects the redox process of target heavy metals. With the removal of selenium as an example, the removal effect of ZVI-loaded BC on Se(IV) and Se(VI) is higher than that of ZVI-loaded AC. In addition, pH significantly affected the removal of Se(IV) and Se(VI) by ZVI-loaded BC and ZVI-loaded AC (Tan et al., 2019). Although the nZVI-loaded BC has an ideal removal effect of heavy metals, the preparation procedure is complicated (Zhang et al., 2022) prepared composite materials with biochar and nZVI using a one-step pyrolysis method, enabling large-scale commercial applications. Furthermore, the activated carbon fiber (Qu et al., 2017) and graphene oxide (GO) (Yang et al., 2021) can also be used as the loaded material of ZVI.
With carbonaceous material as ZVI loading material, the ZVI-loaded BC and AC have more negative charges than the BC and AC surfaces, which has stronger adsorption capacity for cations. The main heavy metal removal mechanism is adsorption. As support material, GO can reduce ZVI aggregation. The abundant functional groups on its surface can well adsorb heavy metals. For Cr(VI) and As(V) in aqueous solution, the another main removal mechanism is reduction. With magnetite and pyrite as ZVI support materials, the main mechanisms for removing heavy metals are adsorption and reduction. HAP is rich in phosphate, and its main mechanism for heavy metal removal is adsorption and precipitation. When zeolite with abundant internal pores is used as ZVI support material, the main mechanisms of heavy metal removal are adsorption, precipitation and ion exchange.
As shown in Table 5, ZVI can also be coated by other materials, which has a good removal effect on heavy metals in groundwater. Surfactants, natural or synthetic polymers can be used to coat the surface of nZVI, which can effectively overcome the agglomeration phenomenon by changing the surface charge of the particles (Reginatto et al., 2020). The nZVI coated by surfactant has good dispersion and transport, fast reaction rate, and wide application range. In addition to continuous reactive walls, injectable suspensions can be used to remediate groundwater (Truex et al., 2011). According to Li et al. (2020), two surfactants polyvinylpyrrolidone (PVP) and sodium oleate (NaOA) were used to coat nZVI. The two active materials exhibited a synergistic effect on Cr(VI) removal.
Surfactant and nZVI are easily desorbed in complex groundwater environments. Polyelectrolytes such as sodium carboxymethyl cellulose (CMC) (Yu et al., 2020), polyacrylic acid (PAA) (Jiemvarangkul et al., 2011), and polyacrylamide (APAM) (Liu et al., 2016) can exist stably for a long time (Zhao et al., 2020). prepared a CMC-wrapped ZVI composite material. When the initial concentration of U(VI) was 25 mg L−1 and the pH was 6, the removal rate was close to 100%. According to the XPS spectra of uranium-loaded nanoparticles CMC-ZVI, U(IV) and U(VI) coexist on the surface of the composite material. The main removal mechanisms are reduction, adsorption and precipitation. Bicarbonate extraction is used to extract the precipitated and adsorbed U(VI). The removal mechanism of uranium by composite materials is as follows:
In summary, the classification of composite material related to ZVI is shown in Figure 4.
FIGURE 4. Classification of composite materials related to ZVI [modified from (Qu et al., 2017) (Zhang et al., 2021)].
The removal effect of a single mineral medium on different pollutants has a huge difference. Many researchers have used nanoparticles-loaded mineral, surfactant modification, and polymer-encapsulated minerals to prepare composite materials to remove complex groundwater pollution (Zeng et al., 2020) made an environmentally friendly and economical SiO2/FeC2O4 composite material using biotite as raw material by one-step synthesis. When the initial concentration was 20 mg L−1, the removal rate of Cr(VI) was close to 100% in the pH range of 2–10 (Wang et al., 2020) modified bentonite with cetyltrimethylammonium bromide (CTMAB), which increased the maximum adsorption capacity for Cr(VI) to 19-fold compared to before modification. In addition, the feasibility of its application in PRB was demonstrated by column experiments (Bambaeero and Bazargan-Lari, 2021) prepared a HAP/chitosan composite material from shrimp shell, bovine bone and snail shell, which has good adsorption capacity for Zn(II) and Cu(II) (Vahdat et al., 2019) loaded Fe3O4 on the surface of HAP prepared from chicken thigh bone, and successfully prepared a nano composite material, which has adsorption capacity on lead as high as 109.89 mg g−1. According to the desorption experiment, EDTA solution (0.001 M) is an ideal desorption reagent, and its adsorption capacity to composites only decreases by 19% after five cycles.
Microorganisms are abundant in nature. For example, both SRB and iron-reducing bacteria have been shown to have the ability to reduce Fe(III) to Fe(II) (Cao et al., 2010). Co-processing of heavy metal pollution in groundwater with mineral composite materials and microorganisms has become an important research direction. In the study of (Xu et al., 2022), SRB were used to synthesize biogenic FeS kaolin composite material, which has ideal adsorption effect on various heavy metals such as Pb(II), Sb(III), and Cd(II). The introduction of microorganisms can often save costs. During the operating life cycle of PRB, it is necessary to strengthen the control of environmental factors such as pH, redox potential and toxin substance.
As shown in Table 6, mineral-related composites can extensively remove heavy metal ions from groundwater. There are differences in the removal mechanisms of heavy metals by composites prepared from different substances and mineral materials. In general, clay has strong adsorption and ion exchange capacities. Zeolite has ideal adsorption, ion exchange and catalytic capabilities. Apatite can provide sufficient phosphate. Mineral materials can improve the comprehensive properties of materials while retaining the advantages of carrier materials, which can not only be embedded in polymers, but also be used as support materials for nanoparticles such as FeC2O4, FeS, and Fe3O4.
TABLE 6. Application of mineral-related composite materials in PRB treatment of groundwater heavy metals.
Carbon composite material is another important medium material. The application of the carbon composite material have been summed up in Table 7. AC and BC have high porosity and large specific surface area, which are ideal loaded materials (Shapira and Zucker, 2022) loaded MoS2 on AC, which achieved an adsorption capacity for mercury of 1280 mg g−1. In addition, this kind of composite material has a good recycling effect (Song X. et al., 2021) prepared biochar by microwave in-situ synthesis with reed as raw material and modified it by nano-magnetite. The maximum adsorption capacity of Cr(VI) by the modified composite material was 9.92 mg g−1, which was higher than that of unmodified biochar (8.03 mg g−1).
TABLE 7. Application of carbon-related composite materials in PRB treatment of groundwater heavy metals.
In general, polymers such as surfactant and polyelectrolyte are rich in functional groups. Such substances can be used to modify AC and BC (Inyang et al., 2016). Chen et al. (2022) loaded chitosan on the surface of BC to synergize the hydroxyl groups of chitosan with the carbonyl groups of pristine BC, thereby enhancing the adsorption capacity for Sb(III). According to the research of (Yi et al., 2019), chitosan-coated AC has broad prospects in the treatment of groundwater and soil cadmium pollution remediation.
With large specific surface area, abundant functional groups, and strong hydrophilicity, GO can efficiently adsorb heavy metals. However, the characteristics of easy aggregation and difficult separation limit its scope of application. In addition to composite materials such as magnetic GO and ZVI-loaded GO, composite materials such as chitosan-coated GO (Yang et al., 2014), alginate-modified GO, silica-loaded GO (GO-SiO2), and thiol-modified GO have potential application value of removing heavy metals from groundwater (Zhang et al., 2020). Alginate is a hydrophilic biosorbent material rich in carboxylic acid groups. Despite its poor stability in the environment, it can be hydrogen-bonded with GO to prepare three-dimensional porous composite materials (Pan et al., 2018). The gel properties of alginate can ensure that the composite material is separated from the water environment, thereby avoiding secondary pollution of the water body (Pan et al., 2019). The composite of two novel materials, GO and SiO2, can complement each other’s advantages (Yang et al., 2016) introduced amino groups into the GO-SiO2, which showed an adsorption capacity of up to 158.9 mg g−1 for Cu(II) under the optimal adsorption conditions. On the one hand, the GO composite material has strong adsorption capacity for heavy metals. On the other hand, it has the disadvantages of complicated process and high cost. Therefore, it is necessary to develop new fabrication processes to reduce costs.
Carbonaceous material is light in weight and high in strength, and its large surface area can effectively disperse nanoparticles (such as MoS2 and nano-magnetite). The surface properties and electron distribution of carbonaceous materials have positive effect on electron migration. In more detail, the electron transfer of heavy metal pollutants and their intermediates is effectively promoted during the adsorption process. Due to its environmental friendliness, it is considered as an ideal material for PRB reaction media.
In summary, there are differences in the removal mechanism and removal effect of heavy metals by different reaction media. Reasonable selection of medium materials should be based on actual conditions, such as groundwater quality and geological conditions. The advantages and disadvantages of the three types of reaction media are compared in Table 8.
The removal effect of PRB on heavy metals in groundwater mainly depends on the reaction medium filled inside. Under reaction medium adsorption, ion exchange, and chemical reaction, heavy metals can be converted into low-toxic substances or fixed in PRB, so as to achieve the purpose of reducing toxic effects. Thus, the key to PRB technology lies in the choice of reaction medium. The screening of reaction medium mainly has three stages, including static batch test, dynamic column test, and simulated slot test. The removal ability and principle, permeability, long-term effect and other properties of the reaction medium were studied.
The static batch test mainly explores the removal ability of the reaction medium to target pollutants through a series of condition control experiments, and obtains the preferred medium (Tasharrofi et al., 2020) carried out batch tests for the removal of Cd(II) from native zeolite and different modified zeolites. According to the research results, sodium zeolite had the most ideal adsorption effect on Cd(II) with an initial concentration of 50 mg L−1, with a maximum adsorption capacity of 22 mg g−1, which was selected as the adsorbent for subsequent experiments.
After completing the preliminary screening, batch test can be used to determine the optimal reaction conditions and reaction principle, thus providing a basis for later dynamic experiments (Chattanathan et al., 2013) prepared HAP from catfish bone. The results of batch test showed that the medium was ideal for the removal of U(VI) when the pH was in the range of 5.5–7. In addition, the smaller the particle size and the larger the surface area, the higher the removal rate of U(VI) (Liu et al., 2014) conducted a batch test study of arsenic removal from ox bone spodium. The adsorption behavior fits the Langmuir isotherm, with an R2 of 0.97. Its main adsorption mechanism is monolayer chemisorption.
The dynamic column test mainly studies the effect of the medium on the removal of pollutants by the reaction medium under hydrodynamic conditions. Through a 9-months Column test, (Liu and Lo, 2011) found that HA could promote the removal of Cr(VI) in a PRB with ZVI as the reaction medium. In addition, calcium in the groundwater component affects the action of HA. Calcium in groundwater would cause precipitates to easily aggregate on the ZVI surface, thereby inhibiting electron transfer and affecting Cr(VI) removal. Second, column tests are often used to simulate the longevity of PRB when aquiclude runs. The long-term study of the reaction medium mainly involves the change of the activity of the reaction medium and the blockage of the medium pores during the service period of the PRB. According to the findings of (Kuma et al., 2016), in the PRB system with ZVI as the reaction medium, the reason that affects the long-term effect of PRB is the blockage of the medium pores caused by the precipitation of secondary minerals. In addition, the column test can study the long-term effect of the medium, as well as the influence of the initial concentration of heavy metals, hydraulic retention time, flow rate, dissolved oxygen content, and organic matter content on the operation effect of PRB (Huang et al., 2020) carried out column tests with SGO/Fe-Mn as the reaction medium to study the removal ability and influencing factors of mercury. According to the research results, the higher initial concentration of mercury, the lower pore velocity, and the lower dissolved organic matter and dissolved oxygen content in the groundwater, the stronger removal ability. On the basis of column experiments, (Obiri-Nyarko et al., 2015) simulated the behavior of Pb2+ with the help of PHREEQC, which confirmed that zeolite is an effective media material for the removal of lead contamination in groundwater.
According to the hydraulic characteristics, the column test simulates a 1D groundwater flow situation. The pilot-scale test can reflect the removal effect of the reaction medium on target pollutants under 3D water flow, with a more realistic simulation effect. Unlike batch and column experiments, the Pilot-scale test can reveal pollutant removal mechanisms in complex field environments, especially the effects of subsurface microorganisms (Beak and Wilkin, 2009). Therefore, pilot-scale test is necessary. Through Geochemist’s Workbench and X-ray absorption spectroscopy, (Beak and Wilkin, 2009) found that the removal mechanism of arsenic by PRB with zero-valent iron as the reaction medium is more complicated than the results of previous laboratory simulation studies. Sulfate is reduced to sulfide under the action of microorganisms in the subterranean environment. The formation of FeS provides more mineral surface for arsenic removal (Rhee et al., 2010)conducted a pilot-scale test using ZVI-loaded zeolite as the reaction medium. According to the test results, ZVI-loaded zeolite can efficiently remove Cd(II) and Cr(VI), which is an ideal active medium for processing complex groundwater polluted by heavy metals (Ludwig et al., 2009) conducted a 30-months experiment with leaf compost and ZVI as the active media for the PRB pilot-scale test to explore the removal effect of As, Pb, Cd, Zn, and Ni and other pollutants in groundwater. The test results show that the removal rate of each pollutant is more than 99%. The cycle of batch and column tests is too short to accurately predict the lifetime of the reaction medium and the long-term performance of the PRB.
The above three types of tests are widely used in the screening process of PRB reaction medium materials. The exploration of the reaction mechanism of the reaction medium and contaminants and the long-term performance of the reaction medium is advanced through the combination with numerical simulation.
PRB is a cost-effective remediation technology for heavy metal pollution in groundwater, in which the reaction medium is an important component. The development of cheap, efficient and environmentally friendly new reaction medium materials has far-reaching significance for the treatment of groundwater and soil heavy metal pollution. Despite extensive research on reaction medium materials, there are still some deficiencies, which hamper the application of PRB technology.
(1) Most of the development of mixed material and composite material reaction medium for dealing with heavy metal pollution revolves around ZVI. The choice of reaction medium is relatively small. The development and research of a single new material is an important direction.
(2) The types of active materials containing natural ore materials and industrial and agricultural solid waste are increasing. Despite good economics, the composition of such materials is complex. Besides the difficulty in controlling the reaction rate between active materials and heavy metals, there is unclear reaction mechanism as well as potential risk of environmental pollution.
(3) The introduction of microbial action can enhance the long-term effect of PRB. The co-treatment of groundwater and soil heavy metal pollution with active materials and microorganisms is beneficial for cost savings. In addition, it is necessary to strengthen the control of pH, redox potential, toxin substance and other environmental factors.
(4) For composite materials, the complex preparation process and high cost will limit the application of PRB technology. The development of composite materials with simple preparation process, strong adsorption capacity, good chemical stability, and easy recycling will be the main development direction of reaction medium materials, especially nano-composite materials.
(5) At present, the research on the treatment of heavy metal pollution in groundwater with reaction medium mainly relies on indoor batch test and column test. The pilot-scale test should be paid attention to, which can more accurately examine the removal effect of the reaction material on the target pollutant. In addition, geochemical numerical modelling is worth researchers’ attention, which can more accurately analyze the removal mechanism, evaluate the service life and potential environmental risks of the reaction material, and determine the potential and limitations of use.
HL and QL contributed to the writing of the manuscript. HL reviewed and edited the manuscript.
This research was funded by Research Fund for high-level talents of Luzhou Vocational and Technical College (ZLYGCC202101).
Wei Du of Luzhou Vocational and Technical College, was of great assistance during data collection.
The authors declare that the research was conducted in the absence of any commercial or financial relationships that could be construed as a potential conflict of interest.
All claims expressed in this article are solely those of the authors and do not necessarily represent those of their affiliated organizations, or those of the publisher, the editors and the reviewers. Any product that may be evaluated in this article, or claim that may be made by its manufacturer, is not guaranteed or endorsed by the publisher.
Abdelrahman, E. A., Abou El-Reash, Y. G., Youssef, H. M., Kotp, Y. H., and Hegazey, R. M. (2021). Utilization of rice husk and waste aluminum cans for the synthesis of some nanosized zeolite, zeolite/zeolite, and geopolymer/zeolite products for the efficient removal of Co(II), Cu(II), and Zn(II) ions from aqueous media. J. Hazard. Mater. 401, 123813. doi:10.1016/j.jhazmat.2020.123813
Abdelrahman, E. A., and Hegazey, R. M. (2019). Exploitation of Egyptian insecticide cans in the fabrication of Si/Fe nanostructures and their chitosan polymer composites for the removal of Ni(II), Cu(II), and Zn(II) ions from aqueous solutions. Compos. Part B Eng. 166, 382–400. doi:10.1016/j.compositesb.2019.02.027
Abdul, N., Abdul-Talib, S., and Amir, A. (2019). Nano-pyrite as a reductant to remove chromium in groundwater. KSCE J. Civ. Eng. 23, 992–999. doi:10.1007/s12205-019-1034-x
Abukhadra, M. R., Dardir, F. M., Shaban, M., Ahmed, E. A., and Soliman, M. F. (2018). Superior removal of Co2+, Cu2+ and Zn2+ contaminants from water utilizing spongy Ni/Fe carbonate–fluorapatite; preparation, application and mechanism. Ecotoxicol. Environ. Saf. 157, 358–368. doi:10.1016/j.ecoenv.2018.03.085
Aghaei, E., Wang, Z., Tadesse, B., Tabelin, C. B., Quadir, Z., and Alorro, R. D. (2021). Performance evaluation of Fe-Al bimetallic particles for the removal of potentially toxic elements from combined acid mine drainage-effluents from refractory gold ore processing. Minerals 11 (6), 590. doi:10.3390/min11060590
Ahmad, W., and Sharma, S. (2019). Synthesis, characterisation and ion exchange properties of hybrid organic–inorganic composite material: Polyacrylamide zirconium (IV) iodosulphosalicylate. Int. J. Environ. Anal. Chem. 99 (15), 1604–1614. doi:10.1080/03067319.2019.1628950
Ali Alyaa, F., and Abd Ali Ziad, T. (2020). Sustainable use of concrete demolition waste as reactive material in permeable barrier for remediation of groundwater: Batch and continuous study. J. Environ. Eng. New. York. 146 (7), 04020048. doi:10.1061/(ASCE)EE.1943-7870.0001714
Ali, H., Khan, E., and Ilahi, I. (2019). Environmental chemistry and ecotoxicology of hazardous heavy metals: Environmental persistence, toxicity, and bioaccumulation. J. Chem. 2019, 1–14. doi:10.1155/2019/6730305
Alighardashi, A., Mehrani, M. J., and Ramezanianpour, A. M. (2018). Pervious concrete reactive barrier containing nano-silica for nitrate removal from contaminated water. Environ. Sci. Pollut. Res. 25, 29481–29492. doi:10.1007/s11356-018-3008-9
Araujo, S. F., Caldeira, C. L., Ciminelli, V. S. T., Silva, A., and Amorim, C. C. (2019). Versatility of iron-rich steel waste for the removal of high arsenic and sulfate concentrations in water. Environ. Sci. Pollut. Res. 26 (5), 4266–4276. doi:10.1007/s11356-018-3168-7
Aredes, S., Klein, B., and Pawlik, M. (2012). The removal of arsenic from water using natural iron oxide minerals. J. Clean. Prod. 29-30, 208–213. doi:10.1016/j.jclepro.2012.01.029
Asokbunyarat, V., Lens, P. N. L., and Annachhatre, A. (2017). “Permeable reactive barriers for heavy metal removal,” in Environmental chemistry for a sustainable world, 65–100.
Asri, S. E., Laghzizil, A., Coradin, T., Saoiabi, A., Alaoui, A., and M’hamedi, R. (2010). Conversion of natural phosphate rock into mesoporous hydroxyapatite for heavy metals removal from aqueous solution. Colloids Surfaces A Physicochem. Eng. Aspects 362 (1), 33–38. doi:10.1016/j.colsurfa.2010.03.036
Bailey, S. E., Olin, T. J., Bricka, R. M., and Adrian, D. D. (1999). A review of potentially low-cost sorbents for heavy metals. Water Res. 33 (11), 2469–2479. doi:10.1016/S0043-1354(98)00475-8
Bambaeero, A., and Bazargan-Lari, R. (2021). Simultaneous removal of copper and zinc ions by low cost natural snail shell/hydroxyapatite/chitosan composite. Chin. J. Chem. Eng. 33, 221–230. doi:10.1016/j.cjche.2020.07.066
Barceló, J., and Poschenrieder, C. (1990). Plant water relations as affected by heavy metal stress: A review. J. Plant Nutr. 13 (1), 1–37. doi:10.1080/01904169009364057
Barton, C. S., Stewart, D. I., Morris, K., and Bryant, D. E. (2004). Performance of three resin-based materials for treating uranium-contaminated groundwater within a PRB. J. Hazard. Mater. 116 (3), 191–204. doi:10.1016/j.jhazmat.2004.08.028
Beak, D. G., and Wilkin, R. T. (2009). Performance of a zerovalent iron reactive barrier for the treatment of arsenic in groundwater: Part 2. Geochemical modeling and solid phase studies. J. Contam. Hydrology 106 (1), 15–28. doi:10.1016/j.jconhyd.2008.12.003
Bhowmick, S., Chakraborty, S., Mondal, P., Van Renterghem, W., Van den Berghe, S., Roman-Ross, G., et al. (2014). Montmorillonite-supported nanoscale zero-valent iron for removal of arsenic from aqueous solution: Kinetics and mechanism. Chem. Eng. J. 243, 14–23. doi:10.1016/j.cej.2013.12.049
Bilardi, S., Calabrò, P. S., and Moraci, N. (2019). The removal efficiency and long-term hydraulic behaviour of zero valent iron/lapillus mixtures for the simultaneous removal of Cu2+, Ni2+ and Zn2+. Sci. Total Environ. 675, 490–500. doi:10.1016/j.scitotenv.2019.04.260
Blowes, D. W., Ptacek, C. J., Benner, S. G., McRae, C. W. T., Bennett, T. A., and Puls, R. W. (2000). Treatment of inorganic contaminants using permeable reactive barriers11Disclaimer: The U. S. Environment Protection Agency through its Office of Research and Development partially funded and collaborated in the research described here under assistance agreement number CR-823017 to the University of Waterloo. J. Contam. Hydrology 45 (1), 123–137. doi:10.1016/s0169-7722(00)00122-4
Cancelo-González, J., Martiñá-Prieto, D., Hernández-Huerta, D., and Barral, M. T. (2017). Metal removal by pine bark compost using a permeable reactive barrier device at laboratory scale. Environ. Chem. 14 (5), 310–318. doi:10.1071/EN17028
Cao, F., Li, F. B., Liu, T. X., Huang, D. Y., Wu, C. Y., Feng, C. H., et al. (2010). Effect of aeromonas hydrophila on reductive dechlorination of DDTs by zero-valent iron. J. Agric. Food Chem. 58 (23), 12366–12372. doi:10.1021/jf102902f
Cerjan Stefanović, Š., Zabukovec Logar, N., Margeta, K., Novak Tušar, N., Arčon, I., Maver, K., et al. (2007). Structural investigation of Zn2+ sorption on clinoptilolite tuff from the Vranjska Banja deposit in Serbia. Microporous Mesoporous Mater. 105 (3), 251–259. doi:10.1016/j.micromeso.2007.04.033
Chattanathan, S. A., Clement, T. P., Kanel, S. R., Barnett, M. O., and Chatakondi, N. (2013). Remediation of uranium-contaminated groundwater by sorption onto hydroxyapatite derived from catfish bones. Water Air Soil Pollut. 224 (2), 1429. doi:10.1007/s11270-012-1429-5
Chen, H., Gao, Y., El-Naggar, A., Niazi, N. K., Sun, C., Shaheen, S. M., et al. (2022). Enhanced sorption of trivalent antimony by chitosan-loaded biochar in aqueous solutions: Characterization, performance and mechanisms. J. Hazard. Mater. 425, 127971. doi:10.1016/j.jhazmat.2021.127971
Chien, S.-W. C., Li, Y.-P., and Liu, C.-C. (2021). Permeable reactive barrier of waste sludge from wine processing utilized to block a metallic mixture plume in a simulated aquifer. Water Sci. Technol. 84 (9), 2472–2485. doi:10.2166/wst.2021.440
Day, S. R., O’Hannesin, S. F., and Marsden, L. (1999). Geotechnical techniques for the construction of reactive barriers. J. Hazard. Mater. 67 (3), 285–297. doi:10.1016/S0304-3894(99)00044-8
Di Natale, F., Lancia, A., Molino, A., and Musmarra, D. (2007). Removal of chromium ions form aqueous solutions by adsorption on activated carbon and char. J. Hazard. Mater. 145 (3), 381–390. doi:10.1016/j.jhazmat.2006.11.028
Dong, L., Liu, W., Jiang, R., and Wang, Z. (2016). Study on the adsorption mechanism of activated carbon removing low concentrations of heavy metals. Desalination Water Treat. 57 (17), 7812–7822. doi:10.1080/19443994.2015.1100140
Dong, L., Zhu, Z., Qiu, Y., and Zhao, J. (2013). Advance in research of heavy metals removal by hydroxyapatite and its composite. Chem. Bull./ Huaxue Tongbao 76, 405–410.
Dries, J., Bastiaens, L., Springael, D., Agathos, S. N., and Diels, L. (2005). Combined removal of chlorinated ethenes and heavy metals by zerovalent iron inbatch and continuous flow column systems. Environ. Sci. Technol. 39 (21), 8460–8465. doi:10.1021/es050251d
Duan, J., Ji, H., Zhao, X., Tian, S., Liu, X., Liu, W., et al. (2020). Immobilization of U(VI) by stabilized iron sulfide nanoparticles: Water chemistry effects, mechanisms, and long-term stability. Chem. Eng. J. 393, 124692. doi:10.1016/j.cej.2020.124692
Elkady, M., Shokry, H., and Hamad, H. (2018). Microwave-assisted synthesis of magnetic hydroxyapatite for removal of heavy metals from groundwater. Chem. Eng. Technol. 41 (3), 553–562. doi:10.1002/ceat.201600631
Ezzatahmadi, N., Ayoko, G. A., Millar, G. J., Speight, R., Yan, C., Li, J., et al. (2017). Clay-supported nanoscale zero-valent iron composite materials for the remediation of contaminated aqueous solutions: A review. Chem. Eng. J. 312, 336–350. doi:10.1016/j.cej.2016.11.154
Faisal, A. A. H., Abbas, T. R., and Jassam, S. H. (2015). Removal of zinc from contaminated groundwater by zero-valent iron permeable reactive barrier. Desalination Water Treat. 55 (6), 1586–1597. doi:10.1080/19443994.2014.928908
Faisal, A. A. H., and Abd Ali, Z. T. (2016). Groundwater protection from lead contamination using granular dead anaerobic sludge biosorbent as permeable reactive barrier. Desalination Water Treat. 57 (9), 3891–3903. doi:10.1080/19443994.2014.990928
Faisal, A. A. H., Al-Ridah, Z. A., Naji, L. A., Naushad, M., and El-Serehy, H. A. (2020). Waste foundry sand as permeable and low permeable barrier for restriction of the propagation of lead and nickel Ions in groundwater. J. Chem. 2020, 1–13. doi:10.1155/2020/4569176
Faisal, A. A. H., and Hmood, Z. A. (2015). Groundwater protection from cadmium contamination by zeolite permeable reactive barrier. Desalination Water Treat. 53 (5), 1–10. doi:10.1080/19443994.2013.855668
Faisal, A. A. H., Sulaymon, A. H., and Khaliefa, Q. M. (2018). A review of permeable reactive barrier as passive sustainable technology for groundwater remediation. Int. J. Environ. Sci. Technol. (Tehran). 15 (5), 1123–1138. doi:10.1007/s13762-017-1466-0
Famiglietti, J. S. (2014). The global groundwater crisis. Nat. Clim. Chang. 4 (11), 945–948. doi:10.1038/nclimate2425
Fan, C., Gao, Y., Zhang, Y., Dong, W., and Lai, M. (2018). Remediation of lead and cadmium from simulated groundwater in loess region in northwestern China using permeable reactive barrier filled with environmentally friendly mixed adsorbents. Environ. Sci. Pollut. Res. 25 (2), 1486–1496. doi:10.1007/s11356-017-0587-9
Fang, J., Gu, Z., Gang, D., Liu, C., Ilton, E. S., and Deng, B. (2007). Cr(VI) removal from aqueous solution by activated carbon coated with quaternized poly(4-vinylpyridine). Environ. Sci. Technol. 41 (13), 4748–4753. doi:10.1021/es061969b
Fedoročková, A., Raschman, P., Sučik, G., Švandová, M., and Doráková, A. (2021). Reactive, sparingly soluble calcined magnesia, tailor-made as the reactive material for heavy metal removal from contaminated groundwater using permeable reactive barrier. Minerals 11 (11), 1153. doi:10.3390/min11111153
Flint, L. C., Arias-Paić, M. S., and Korak, J. A. (2021). Removal of hexavalent chromium by anion exchange: Non-target anion behavior and practical implications. Environ. Sci. Water Res. Technol. 7 (12), 2397–2413. doi:10.1039/D1EW00382H
Frade, P. R., Notini, L., Santos, S. V., Moreira, R. F. P. M., Leão, M. M. D., and Amorim, C. C. (2018). Feasibility study of the use of basic oxygen furnace sludge in a permeable reactive barrier. J. Hazard. Mater. 351, 188–195. doi:10.1016/j.jhazmat.2018.02.035
Fu, F., Dionysiou, D. D., and Liu, H. (2014). The use of zero-valent iron for groundwater remediation and wastewater treatment: A review. J. Hazard. Mater. 267, 194–205. doi:10.1016/j.jhazmat.2013.12.062
Futalan, C. M., Kan, C., Dalida, M. L., Pascua, C., and Wan, M. (2011). Fixed-bed column studies on the removal of copper using chitosan immobilized on bentonite. Carbohydr. Polym. 83 (2), 697–704. doi:10.1016/j.carbpol.2010.08.043
Gibert, O., Cortina, J. L., de Pablo, J., and Ayora, C. (2013). Performance of a field-scale permeable reactive barrier based on organic substrate and zero-valent iron for in situ remediation of acid mine drainage. Environ. Sci. Pollut. Res. 20 (11), 7854–7862. doi:10.1007/s11356-013-1507-2
Gong, Y., Tang, J., and Zhao, D. (2016). Application of iron sulfide particles for groundwater and soil remediation: A review. Water Res. 89, 309–320. doi:10.1016/j.watres.2015.11.063
Gupta, A., Yunus, M., and Sankararamakrishnan, N. (2012). Zerovalent iron encapsulated chitosan nanospheres – a novel adsorbent for the removal of total inorganic Arsenic from aqueous systems. Chemosphere 86 (2), 150–155. doi:10.1016/j.chemosphere.2011.10.003
Han, C., Jiao, Y., Wu, Q., Yang, W., Yang, H., and Xue, X. (2016a). Kinetics and mechanism of hexavalent chromium removal by basic oxygen furnace slag. J. Environ. Sci. 46, 63–71. doi:10.1016/j.jes.2015.09.024
Han, Y., Cao, X., Ouyang, X., Sohi, S. P., and Chen, J. (2016b). Adsorption kinetics of magnetic biochar derived from peanut hull on removal of Cr (VI) from aqueous solution: Effects of production conditions and particle size. Chemosphere 145, 336–341. doi:10.1016/j.chemosphere.2015.11.050
Han, Y. S., Jeong, H. Y., Demond, A. H., and Hayes, K. F. (2011). X-ray absorption and photoelectron spectroscopic study of the association of As(III) with nanoparticulate FeS and FeS-coated sand. Water Res. 45 (17), 5727–5735. doi:10.1016/j.watres.2011.08.026
Hashim, M. A., Mukhopadhyay, S., Sahu, J. N., and Sengupta, B. (2011). Remediation technologies for heavy metal contaminated groundwater. J. Environ. Manag. 92 (10), 2355–2388. doi:10.1016/j.jenvman.2011.06.009
He, X., Min, X., Peng, T., Ke, Y., Zhao, F., Sillanpää, M., et al. (2020). Enhanced adsorption of antimonate by ball-milled microscale zero valent iron/pyrite composite: Adsorption properties and mechanism insight. Environ. Sci. Pollut. Res. 27 (14), 16484–16495. doi:10.1007/s11356-020-08163-y
Hernández-Cocoletzi, H., Salinas, R. A., Águila-Almanza, E., Rubio-Rosas, E., Chai, W. S., Chew, K. W., et al. (2020). Natural hydroxyapatite from fishbone waste for the rapid adsorption of heavy metals of aqueous effluent. Environ. Technol. Innovation 20, 101109. doi:10.1016/j.eti.2020.101109
Holmes, R. R., Hart, M. L., and Kevern, J. T. (2017). Removal and breakthrough of lead, cadmium, and zinc in permeable reactive concrete. Environ. Eng. Sci. 35 (5), 408–419. doi:10.1089/ees.2017.0160
Hornig, G., Northcott, K., Snape, I., and Stevens, G. (2008). Assessment of sorbent materials for treatment of hydrocarbon contaminated ground water in cold regions. Cold Regions Sci. Technol. 53 (1), 83–91. doi:10.1016/j.coldregions.2007.08.004
Huang, Y., Wang, M., Gong, Y., and Zeng, E. Y. (2020). Efficient removal of mercury from simulated groundwater using thiol-modified graphene oxide/Fe–Mn composite in fixed-bed columns: Experimental performance and mathematical modeling. Sci. Total Environ. 714, 136636. doi:10.1016/j.scitotenv.2020.136636
Indraratna, B., Regmi, G., Nghiem Long, D., and Golab, A. (2010). Performance of a PRB for the remediation of acidic groundwater in acid sulfate soil terrain. J. Geotech. Geoenviron. Eng. 136 (7), 897–906. doi:10.1061/(ASCE)GT.1943-5606.0000305
Inyang, M. I., Gao, B., Yao, Y., Xue, Y., Zimmerman, A., Mosa, A., et al. (2016). A review of biochar as a low-cost adsorbent for aqueous heavy metal removal. Crit. Rev. Environ. Sci. Technol. 46 (4), 406–433. doi:10.1080/10643389.2015.1096880
Jarvis, A. P., Moustafa, M., Orme, P. H. A., and Younger, P. L. (2006). Effective remediation of grossly polluted acidic, and metal-rich, spoil heap drainage using a novel, low-cost, permeable reactive barrier in Northumberland, UK. Environ. Pollut. 143 (2), 261–268. doi:10.1016/j.envpol.2005.11.028
Jarvis, T. (2021). Global groundwater: Source, scarcity, sustainability, security, and solutions. Groundwater 59, 479–480. doi:10.1111/gwat.13113
Jeen, S. W., Gillham, R. W., and Przepiora, A. (2011). Predictions of long-term performance of granular iron permeable reactive barriers: Field-scale evaluation. J. Contam. Hydrology 123 (1), 50–64. doi:10.1016/j.jconhyd.2010.12.006
Jia, H., Gu, C., Boyd, S. A., Teppen, B. J., Johnston, C. T., Song, C., et al. (2011). Comparison of reactivity of nanoscaled zero-valent iron formed on clay surfaces. Soil Sci. Soc. Am. J. 75 (2), 357–364. doi:10.2136/sssaj2010.0080nps
Jia, H., and Wang, C. (2013). Comparative studies on montmorillonite-supported zero-valent iron nanoparticles produced by different methods: Reactivity and stability. Environ. Technol. 34 (1), 25–33. doi:10.1080/09593330.2012.679698
Jiang, D., Zeng, G., Huang, D., Chen, M., Zhang, C., Huang, C., et al. (2018). Remediation of contaminated soils by enhanced nanoscale zero valent iron. Environ. Res. 163, 217–227. doi:10.1016/j.envres.2018.01.030
Jiemvarangkul, P., Zhang, W.-x., and Lien, H.-L. (2011). Enhanced transport of polyelectrolyte stabilized nanoscale zero-valent iron (nZVI) in porous media. Chem. Eng. J. 170 (2), 482–491. doi:10.1016/j.cej.2011.02.065
Kantar, C., Bulbul, M. S., and Keskin, S. (2017). Role of humic substances on Cr(VI) removal from groundwater with Pyrite. Water Air Soil Pollut. 228 (1), 48. doi:10.1007/s11270-016-3233-0
Khan, K., Lu, Y., Khan, H., Ishtiaq, M., Khan, S., Waqas, M., et al. (2013). Heavy metals in agricultural soils and crops and their health risks in Swat District, northern Pakistan. Food Chem. Toxicol. 58, 449–458. doi:10.1016/j.fct.2013.05.014
Kong, L., Ruan, Y., Zheng, Q., Su, M., Diao, Z.-H., Chen, D., et al. (2019). Uranium extraction using hydroxyapatite recovered from phosphorus containing wastewater. J. Hazard. Mater. 382, 120784. doi:10.1016/j.jhazmat.2019.120784
Kong, X., Huang, G., Han, Z., Xu, Y., Zhu, M., and Zhang, Z. (2017). Evaluation of zeolite-supported microscale zero-valent iron as a potential adsorbent for Cd2+ and Pb2+ removal in permeable reactive barriers. Environ. Sci. Pollut. Res. 24 (15), 13837–13844. doi:10.1007/s11356-017-8974-9
Kouotou, D., Gharibi, E. K., Bailón-García, E., and Ghalit, M. (2022). Improved Cd (II) ions removal performance from aqueous solution using cerium doped activated carbon. Mater. Today Proc. 51, 1957–1965. doi:10.1016/j.matpr.2021.04.298
Kuma, N., Millot, R., Battaglia-Brunet, F., Omoregie, E., Chaurand, P., Borschneck, D., et al. (2016). Microbial and mineral evolution in zero valent iron-based permeable reactive barriers during long-term operations. Environ. Sci. Pollut. Res. 23 (6), 5960–5968. doi:10.1007/s11356-015-5712-z
Kumara, G. M. P., and Kawamoto, K. (2021). Use of natural zeolite and its mixtures to refine high-concentrated heavy metal-contaminated wastewater: An investigation of simultaneous removal of Cd (II) and Pb (II) by batch Adsorption method. Water Air Soil Pollut. 232 (11), 463. doi:10.1007/s11270-021-05420-9
Lai, K. C. K., and Lo, I. M. C. (2008). Removal of chromium (VI) by acid-washed zero-valent iron under various groundwater geochemistry conditions. Environ. Sci. Technol. 42 (4), 1238–1244. doi:10.1021/es071572n
Li, J., Fan, M., Li, M., and Liu, X. (2020). Cr(VI) removal from groundwater using double surfactant-modified nanoscale zero-valent iron (nZVI): Effects of materials in different status. Sci. Total Environ. 717, 137112. doi:10.1016/j.scitotenv.2020.137112
Li, P., Karunanidhi, D., Subramani, T., and Srinivasamoorthy, K. (2021). Sources and consequences of groundwater contamination. Arch. Environ. Contam. Toxicol. 80 (1), 1–10. doi:10.1007/s00244-020-00805-z
Li, Y., Du, Q., Wang, X., Zhang, P., Wang, D., Wang, Z., et al. (2010). Removal of lead from aqueous solution by activated carbon prepared from Enteromorpha prolifera by zinc chloride activation. J. Hazard. Mater. 183 (1), 583–589. doi:10.1016/j.jhazmat.2010.07.063
Li, Z., Wang, L., Meng, J., Liu, X., Xu, J., Wang, F., et al. (2018). Zeolite-supported nanoscale zero-valent iron: New findings on simultaneous adsorption of Cd(II), Pb(II), and As(III) in aqueous solution and soil. J. Hazard. Mater. 344, 1–11. doi:10.1016/j.jhazmat.2017.09.036
Liang, M., Lu, L., He, H., Li, J., Zhu, Z., and Zhu, Y. (2021). Applications of biochar and modified biochar in heavy metal contaminated soil: A descriptive review. Sustainability 13 (24), 14041. doi:10.3390/su132414041
Liu, H., Liao, L., Lu, G., Wang, L., Mei, L., and Guo, Q. (2020). Research progresses of the application of mineral materials as reactive media in the permeable reactive barrier technology. Acta mineral. sin. 40, 274–280. doi:10.16461/j.cnki.1000-4734.2020.40.119
Liu, J., Huang, X., Liu, J., Wang, W., Zhang, W., and Dong, F. (2014). Adsorption of arsenic(V) on bone char: Batch, column and modeling studies. Environ. Earth Sci. 72 (6), 2081–2090. doi:10.1007/s12665-014-3116-x
Liu, J., Liu, A., and Zhang, W.-x. (2016). The influence of polyelectrolyte modification on nanoscale zero-valent iron (nZVI): Aggregation, sedimentation, and reactivity with Ni(II) in water. Chem. Eng. J. 303, 268–274. doi:10.1016/j.cej.2016.05.132
Liu, M., Sun, F., Lv, Y., Xu, Y., Li, M., Wang, Y., et al. (2021). Remediation of arsenic-contaminated soil by nano-zirconia modified biochar. Environ. Sci. Pollut. Res. 28 (48), 68792–68803. doi:10.1007/s11356-021-15362-8
Liu, T., and Lo, I. M. C. (2011). Influences of humic acid on Cr(VI) removal by zero-valent iron from groundwater with various constituents: Implication for long-term PRB performance. Water Air Soil Pollut. 216 (1), 473–483. doi:10.1007/s11270-010-0546-2
Liu, X., Lai, D., and Wang, Y. (2019a). Performance of Pb(II) removal by an activated carbon supported nanoscale zero-valent iron composite at ultralow iron content. J. Hazard. Mater. 361, 37–48. doi:10.1016/j.jhazmat.2018.08.082
Liu, Y., Mou, H., Chen, L., Mirza, Z. A., and Liu, L. (2015). Cr(VI)-contaminated groundwater remediation with simulated permeable reactive barrier (PRB) filled with natural pyrite as reactive material: Environmental factors and effectiveness. J. Hazard. Mater. 298, 83–90. doi:10.1016/j.jhazmat.2015.05.007
Liu, Y., Sohi, S. P., Liu, S., Guan, J., Zhou, J., and Chen, J. (2019b). Adsorption and reductive degradation of Cr(VI) and TCE by a simply synthesized zero valent iron magnetic biochar. J. Environ. Manag. 235, 276–281. doi:10.1016/j.jenvman.2019.01.045
Lou, K., Rajapaksha, A. U., Ok, Y. S., and Chang, S. X. (2016). Pyrolysis temperature and steam activation effects on sorption of phosphate on pine sawdust biochars in aqueous solutions. Chem. Speciat. Bioavailab. 28 (1-4), 42–50. doi:10.1080/09542299.2016.1165080
Lou, Y., Cai, Y., Tong, Y., Hsieh, L., Li, X., Xu, W., et al. (2019). Interaction between pollutants during the removal of polychlorinated biphenyl-heavy metal combined pollution by modified nanoscale zero-valent iron. Sci. Total Environ. 673, 120–127. doi:10.1016/j.scitotenv.2019.04.064
Lü, Y., Li, Z., Li, J., Chen, K., Dong, H., Shou, J., et al. (2018). Synergetic effect of pyrite on Cr(VI) removal by zero valent iron in column experiments: An investigation of mechanisms. Chem. Eng. J. 349, 522–529. doi:10.1016/j.cej.2018.05.133
Ludwig, R. D., Smyth, D. J. A., Blowes, D. W., Spink, L. E., Wilkin, R. T., Jewett, D. G., et al. (2009). Treatment of arsenic, heavy metals, and acidity using a mixed ZVI-compost PRB. Environ. Sci. Technol. 43 (6), 1970–1976. doi:10.1021/es802394p
Maamoun, I., Falyouna, O., Eljamal, R., Bensaida, K., and Eljamal, O. (2021). Optimization modeling of nFe0/Cu-PRB design for Cr(VI) removal from groundwater. Int. J. Environ. Sci. Dev. 12, 131–138. doi:10.18178/ijesd.2021.12.5.1330
MacDonald, J. A., and Kavanaugh, M. C. (1994). Restoring contaminated groundwater: An achievable goal? Environ. Sci. Technol. 28 (8), 362A–368A. doi:10.1021/es00057a001
Madadrang, C. J., Kim, H. Y., Gao, G., Wang, N., Zhu, J., Feng, H., et al. (2012). Adsorption behavior of EDTA-graphene oxide for Pb (II) removal. ACS Appl. Mat. Interfaces 4 (3), 1186–1193. doi:10.1021/am201645g
Maharana, M., and Sen, S. (2021). Magnetic zeolite: A green reusable adsorbent in wastewater treatment. Mater. Today Proc. 47, 1490–1495. doi:10.1016/j.matpr.2021.04.370
Mandal, S., Pu, S., Shangguan, L., Liu, S., Ma, H., Adhikari, S., et al. (2020). Synergistic construction of green tea biochar supported nZVI for immobilization of lead in soil: A mechanistic investigation. Environ. Int. 135, 105374. doi:10.1016/j.envint.2019.105374
Min, X., Li, Q., Zhang, X., Liu, L., Xie, Y., Guo, L., et al. (2021). Characteristics, kinetics, thermodynamics and long-term effects of zerovalent iron/pyrite in remediation of Cr(VI)-contaminated soil. Environ. Pollut. 289, 117830. doi:10.1016/j.envpol.2021.117830
Mukherjee, A., Guo, H., Langan, S., Mckenzie, A., and Aureli, A. (2020). Global groundwater: Source, scarcity, sustainability, security, and solutions.
Mystrioti, C., Ntrouka, A., Thymi, S., Papassiopi, N., and Xenidis, A. (2020). Effect of limestone grain size on the mobility of green nanoiron suspension. Appl. Geochem. 122, 104759. doi:10.1016/j.apgeochem.2020.104759
Naji, L. A., Faisal, A. A. H., Rashid, H. M., Naushad, M., and Ahamad, T. (2020). Environmental remediation of synthetic leachate produced from sanitary landfills using low-cost composite sorbent. Environ. Technol. Innovation 18, 100680. doi:10.1016/j.eti.2020.100680
Namasivayam, C., and Sangeetha, D. (2006). Removal and recovery of vanadium(V) by adsorption onto ZnCl2 activated carbon: Kinetics and isotherms. Adsorption 12 (2), 103–117. doi:10.1007/s10450-006-0373-3
Ngueagni, P. T., Kumar, P. S., Woumfo, E. D., and Prasanth, S. M. (2020). “Adsorption of Pb(II) and Cd(II) ions onto modified biogenic slaughterhouse waste: Equilibrium and kinetic analysis,” in International journal of environmental analytical chemistry, 1–20. doi:10.1080/03067319.2020.1784409
Nicola, M., Stefania, B., and Calabro, P. S. (2017). Fe0/pumice mixtures: From laboratory tests to permeable reactive barrier design. Environ. Geotech. 4 (4), 245–256. doi:10.1680/jenge.15.00002
Nijhawan, A., Butler, E., and Sabatini, D. (2018). Hydroxyapatite ceramic adsorbents: Effect of pore size, regeneration, and selectivity for Fluoride. J. Environ. Eng. New. York. 144, 04018117. doi:10.1061/(ASCE)EE.1943-7870.0001464
Obiri-Nyarko, F., Grajales-Mesa, s., and Malina, G. (2014). An overview of permeable reactive barriers for in situ sustainable groundwater remediation. Chemosphere 111, 243–259. doi:10.1016/j.chemosphere.2014.03.112
Obiri-Nyarko, F., Kwiatkowska-Malina, J., Malina, G., and Kasela, T. (2015). Geochemical modelling for predicting the long-term performance of zeolite-PRB to treat lead contaminated groundwater. J. Contam. Hydrology 177-178, 76–84. doi:10.1016/j.jconhyd.2015.03.007
Oliva, J., De Pablo, J., Cortina, J.-L., Cama, J., and Ayora, C. (2010). The use of Apatite II™ to remove divalent metal ions zinc(II), lead(II), manganese(II) and iron(II) from water in passive treatment systems: Column experiments. J. Hazard. Mater. 184 (1), 364–374. doi:10.1016/j.jhazmat.2010.08.045
Omran, M., Fabritius, T., Yu, Y., and Chen, G. (2020). Thermal and mass spectroscopic analysis of BF and BOF sludges: Study of their behavior under air and inert atmosphere. Metals 10 (3), 397. doi:10.3390/met10030397
Pan, L., Wang, Z., Yang, Q., and Huang, R. (2018). Efficient removal of lead, copper and cadmium ions from water by a porous calcium alginate/graphene oxide composite aerogel. Nanomaterials 8 (11), 957. doi:10.3390/nano8110957
Pan, L., Wang, Z., Zhao, X., and He, H. (2019). Efficient removal of lead and copper ions from water by enhanced strength-toughness alginate composite fibers. Int. J. Biol. Macromol. 134, 223–229. doi:10.1016/j.ijbiomac.2019.05.022
Paranavithana, G. N., Kawamoto, K., Inoue, Y., Saito, T., Vithanage, M., Kalpage, C. S., et al. (2016). Adsorption of Cd2+ and Pb2+ onto coconut shell biochar and biochar-mixed soil. Environ. Earth Sci. 75 (6), 484. doi:10.1007/s12665-015-5167-z
Park, M. H., Jeong, S., Lee, G., Park, H., and Kim, J. Y. (2019). Removal of aqueous-phase Pb(II), Cd(II), As(III), and As(V) by nanoscale zero-valent iron supported on exhausted coffee grounds. Waste Manag. 92, 49–58. doi:10.1016/j.wasman.2019.05.017
Park, M., Park, J., Kang, J., Han, Y.-S., and Jeong, H. Y. (2018). Removal of hexavalent chromium using mackinawite (FeS)-coated sand. J. Hazard. Mater. 360, 17–23. doi:10.1016/j.jhazmat.2018.07.086
Pawluk, K., Fronczyk, J., and Garbulewski, K. (2015). Reactivity of nano zero-valent iron in permeable reactive barriers. Pol. J. Chem. Technol. 17 (1), 7–10. doi:10.1515/pjct-2015-0002
Puls, R. W. (2006). “Long-term performance of permeable reactive barriers: Lessons learned on design, contaminant treatment, longevity, performance monitoring and cost - an overview,” in Soil and water pollution monitoring, protection and remediation.
Qian, L., Zhang, W., Yan, J., Han, L., Chen, Y., Ouyang, D., et al. (2017). Nanoscale zero-valent iron supported by biochars produced at different temperatures: Synthesis mechanism and effect on Cr(VI) removal. Environ. Pollut. 223, 153–160. doi:10.1016/j.envpol.2016.12.077
Qin, N., Zhang, Y., Zhou, H., Geng, Z., Liu, G., Zhang, Y., et al. (2016). Enhanced removal of trace Cr(VI) from neutral and alkaline aqueous solution by FeCo bimetallic nanoparticles. J. Colloid Interface Sci. 472, 8–15. doi:10.1016/j.jcis.2016.03.025
Qu, G., Kou, L., Wang, T., Liang, D., and Hu, S. (2017). Evaluation of activated carbon fiber supported nanoscale zero-valent iron for chromium (VI) removal from groundwater in a permeable reactive column. J. Environ. Manag. 201, 378–387. doi:10.1016/j.jenvman.2017.07.010
Rajendran, M., and Thangavelu, D. (2021). Removal of As(V) from water using galvanically coupled sacrificial metals. J. Hazard. Mater. 409, 124564. doi:10.1016/j.jhazmat.2020.124564
Reginatto, C., Cecchin, I., Salvagni Heineck, K., Thomé, A., and Reddy, K. R. (2020). Influence of nanoscale zero-valent iron on hydraulic conductivity of a residual clayey soil and modeling of the filtration parameter. Environ. Sci. Pollut. Res. 27 (9), 9288–9296. doi:10.1007/s11356-019-07197-1
Rhee, S.-S., Lee, S., and Park, J. (2010). Simultaneous removal of Cd and Cr(VI) in the subsurface using permeable reactive barrier filled with Fe-loaded zeolite: Soil box experiment. J. Korean Geotechnical Soc. 26, 61–68.
Salman, M., Rehman, R., Mahmud, T., Zaman, W., Shafique, U., and Ali, S. (2011). Assessment of concentration of lead, cadmium, chromium and selenium in blood serum of cancer and diabetic patients of Pakistan. J. Chem. Soc. Pak. 33, 869–873.
Scherer, M. M., Richter, S., Valentine, R. L., and Alvarez, P. J. J. (2000). Chemistry and microbiology of permeable reactive barriers for in situ groundwater clean up. Crit. Rev. Microbiol. 26 (4), 221–264. doi:10.1080/10408410091154237
Shabalala, A. N., Ekolu, S. O., Diop, S., and Solomon, F. (2017). Pervious concrete reactive barrier for removal of heavy metals from acid mine drainage − column study. J. Hazard. Mater. 323, 641–653. doi:10.1016/j.jhazmat.2016.10.027
Shakoor, M. B., Ali, S., Rizwan, M., Abbas, F., Bibi, I., Riaz, M., et al. (2020). A review of biochar-based sorbents for separation of heavy metals from water. Int. J. Phytoremediation 22 (2), 111–126. doi:10.1080/15226514.2019.1647405
Shapira, K., and Zucker, I. (2022). Emerging investigator series: Molybdenum disulfide-enabled activated carbon - a multifunctional adsorbent for practical water treatment applications. Environ. Sci. Nano 9 (2), 477–488. doi:10.1039/D1EN00897H
Shubair, T., Eljamal, O., Khalil, A. M. E., Tahara, A., and Matsunaga, N. (2018). Novel application of nanoscale zero valent iron and bimetallic nano-Fe/Cu particles for the treatment of cesium contaminated water. J. Environ. Chem. Eng. 6 (4), 4253–4264. doi:10.1016/j.jece.2018.06.015
Simon, F. G., Biermann, V., and Peplinski, B. (2008). Uranium removal from groundwater using hydroxyapatite. Appl. Geochem. 23 (8), 2137–2145. doi:10.1016/j.apgeochem.2008.04.025
Song, J., Huang, G., Han, D., Hou, Q., Gan, L., and Zhang, M. (2021a). A review of reactive media within permeable reactive barriers for the removal of heavy metal(loid)s in groundwater: Current status and future prospects. J. Clean. Prod. 319, 128644. doi:10.1016/j.jclepro.2021.128644
Song, X., Zhang, Y., Cao, N., Sun, D., Zhang, Z., Wang, Y., et al. (2021b). Sustainable chromium (VI) removal from contaminated groundwater using nano-magnetite-modified biochar via rapid microwave synthesis. Molecules 26 (1), 103. doi:10.3390/molecules26010103
Soto, C., Nakano, K., Leon Romero, M., Aikawa, Y., Arai, S., and Nishimura, O. (2015). Differences in the removal mechanisms of Undaria pinnatifida and Phragmites australis as biomaterials for lead removal. Water Sci. Technol. 72, 1226–1233. doi:10.2166/wst.2015.334
Stötzel, C., Müller, F. A., Reinert, F., Niederdraenk, F., Barralet, J. E., and Gbureck, U. (2009). Ion adsorption behaviour of hydroxyapatite with different crystallinities. Colloids Surfaces B Biointerfaces 74 (1), 91–95. doi:10.1016/j.colsurfb.2009.06.031
Stucker, V., Ranville, J., Newman, M., Peacock, A., Cho, J., and Hatfield, K. (2011). Evaluation and application of anion exchange resins to measure groundwater uranium flux at a former uranium mill site. Water Res. 45 (16), 4866–4876. doi:10.1016/j.watres.2011.06.030
Sulaymon, A. H., Faisal, A. A. H., and Khaliefa, Q. M. (2015). Cement kiln dust (CKD)-filter sand permeable reactive barrier for the removal of Cu(II) and Zn(II) from simulated acidic groundwater. J. Hazard. Mater. 297, 160–172. doi:10.1016/j.jhazmat.2015.04.061
Tan, G., Mao, Y., Wang, H., Junaid, M., and Xu, N. (2019). Comparison of biochar- and activated carbon-supported zerovalent iron for the removal of Se(IV) and Se(VI): Influence of pH, ionic strength, and natural organic matter. Environ. Sci. Pollut. Res. 26 (21), 21609–21618. doi:10.1007/s11356-019-05497-0
Tasharrofi, S., Rouzitalab, Z., Maklavany, D. M., Esmaeili, A., Rabieezadeh, M., Askarieh, M., et al. (2020). Adsorption of cadmium using modified zeolite-supported nanoscale zero-valent iron composites as a reactive material for PRBs. Sci. Total Environ. 736, 139570. doi:10.1016/j.scitotenv.2020.139570
Truex, M. J., Vermeul, V. R., Mendoza, D. P., Fritz, B. G., Mackley, R. D., Oostrom, M., et al. (2011). Injection of zero-valent iron into an unconfined aquifer using shear-thinning fluids. Ground Water Monit. remediat. 31 (1), 50–58. doi:10.1111/j.1745-6592.2010.01319.x
Turner, M., Sogorka, A., Dave, N., Duchene, M., Dwyer, B., Hubble, D., et al. (2005). Technical/regulatory guidelines : Permeable reactive barriers: Lessons learned/new directions.
Vahdat, A., Ghasemi, B., and Yousefpour, M. (2019). Synthesis of hydroxyapatite and hydroxyapatite/Fe3O4 nanocomposite for removal of heavy metals. Environ. Nanotechnol. Monit. Manag. 12, 100233. doi:10.1016/j.enmm.2019.100233
Vilensky, M., Berkowitz, B., and Warshawsky, A. (2002). In situ remediation of groundwater contaminated by heavy- and transition-metal ions by selective ion-exchange methods. Environ. Sci. Technol. 36, 1851–1855. doi:10.1021/es010313+
Wang, F., Xu, W., Xu, Z., and Liu, H. (2020). CTMAB-modified bentonite–based PRB in remediating Cr(VI) contaminated groundwater. Water Air Soil Pollut. 231 (1), 20. doi:10.1007/s11270-019-4386-4
Wang, H., Yan, K., Xing, H., Chen, J., and Lu, R. (2021). Effective removal of Cu2+ from aqueous solution by synthetic abalone shell hydroxyapatite microspheres adsorbent. Environ. Technol. Innovation 23, 101663. doi:10.1016/j.eti.2021.101663
Wang, M., Cheng, W., Wan, T., Hu, B., Zhu, Y., Song, X., et al. (2019a). Mechanistic investigation of U(VI) sequestration by zero-valent iron/activated carbon composites. Chem. Eng. J. 362, 99–106. doi:10.1016/j.cej.2018.12.138
Wang, Q., Song, X., Wei, C., Jin, P., Chen, X., Tang, Z., et al. (2022). In situ remediation of Cr(VI) contaminated groundwater by ZVI-PRB and the corresponding indigenous microbial community responses: A field-scale study. Sci. Total Environ. 805, 150260. doi:10.1016/j.scitotenv.2021.150260
Wang, S., and Peng, Y. (2010). Natural zeolites as effective adsorbents in water and wastewater treatment. Chem. Eng. J. 156 (1), 11–24. doi:10.1016/j.cej.2009.10.029
Wang, T., Liu, Y., Wang, J., Wang, X., Liu, B., and Wang, Y. (2019b). In-situ remediation of hexavalent chromium contaminated groundwater and saturated soil using stabilized iron sulfide nanoparticles. J. Environ. Manag. 231, 679–686. doi:10.1016/j.jenvman.2018.10.085
Wellman, D. M., Glovack, J. N., Parker, K., Richards, E. L., and Pierce, E. M. (2008). Sequestration and retention of uranium(VI) in the presence of hydroxylapatite under dynamic geochemical conditions. Environ. Chem. 5 (1), 40–50. doi:10.1071/en07060
Wen, Z., Ke, J., Xu, J., Guo, S., Zhang, Y., and Chen, R. (2018). One-step facile hydrothermal synthesis of flowerlike Ce/Fe bimetallic oxides for efficient As(V) and Cr(VI) remediation: Performance and mechanism. Chem. Eng. J. 343, 416–426. doi:10.1016/j.cej.2018.03.034
Wijeyawardana, P., Nanayakkara, N., Gunasekara, C., Karunarathna, A., Law, D., and Pramanik, B. K. (2022). Improvement of heavy metal removal from urban runoff using modified pervious concrete. Sci. Total Environ. 815, 152936. doi:10.1016/j.scitotenv.2022.152936
Wilkin, R., and Puls, R. (2003). Capstone report on the application, monitoring, and performance of permeable reactive barriers for ground-water remediation.
Wilkin, R. T., Acree, S. D., Ross, R. R., Beak, D. G., and Lee, T. R. (2009). Performance of a zerovalent iron reactive barrier for the treatment of arsenic in groundwater: Part 1. Hydrogeochemical studies. J. Contam. Hydrology 106 (1), 1–14. doi:10.1016/j.jconhyd.2008.12.002
Wilopo, W., Sasaki, K., Hirajima, T., and Yamanaka, T. (2008). Immobilization of arsenic and manganese in contaminated groundwater by permeable reactive barriers using zero valent iron and sheep manure. Mat. Trans. 49 (10), 2265–2274. doi:10.2320/matertrans.M-MRA2008827
Wilson, K., Yang, H., Seo, C. W., and Marshall, W. E. (2006). Select metal adsorption by activated carbon made from peanut shells. Bioresour. Technol. 97 (18), 2266–2270. doi:10.1016/j.biortech.2005.10.043
Wu, Y., Guan, C.-Y., Griswold, N., Hou, L.-y., Fang, X., Hu, A., et al. (2020). Zero-valent iron-based technologies for removal of heavy metal(loid)s and organic pollutants from the aquatic environment: Recent advances and perspectives. J. Clean. Prod. 277, 123478. doi:10.1016/j.jclepro.2020.123478
Wu, Y., Zhang, J., Tong, Y., and Xu, X. (2009). Chromium (VI) reduction in aqueous solutions by Fe3O4-stabilized Fe0 nanoparticles. J. Hazard. Mater. 172 (2), 1640–1645. doi:10.1016/j.jhazmat.2009.08.045
Xiang, G., Long, S., Liu, H., and Wu, X. (2021). Cd(II) removal from aqueous solutions by pomelo peel derived biochar in a permeable reactive barrier: Modelling, optimization and mechanism. Mat. Res. Express 8 (11), 115508. doi:10.1088/2053-1591/ac386b
Xiang, S., Cheng, W., Nie, X., Ding, C., Yi, F., Asiri, A. M., et al. (2018). Zero-valent iron-aluminum for the fast and effective U(VI) removal. J. Taiwan Inst. Chem. Eng. 85, 186–192. doi:10.1016/j.jtice.2018.01.039
Xie, J., Lei, C., Chen, W., Xie, Q., Guo, Q., and Huang, B. (2021). Catalytic properties of transition metals modified nanoscale zero-valent iron for simultaneous removal of 4-chlorophenol and Cr(VI): Efficacy, descriptor and reductive mechanisms. J. Hazard. Mater. 403, 123827. doi:10.1016/j.jhazmat.2020.123827
Xing, M., Xu, L., and Wang, J. (2016). Mechanism of Co(II) adsorption by zero valent iron/graphene nanocomposite. J. Hazard. Mater. 301, 286–296. doi:10.1016/j.jhazmat.2015.09.004
Xu, R., Li, Q., Yang, Y., Jin, S., Liao, L., Wu, Z., et al. (2022). Removal of heavy metal(loid)s from aqueous solution by biogenic FeS–kaolin composite: Behaviors and mechanisms. Chemosphere 299, 134382. doi:10.1016/j.chemosphere.2022.134382
Xu, Y.-N., and Chen, Y. (2020). Advances in heavy metal removal by sulfate-reducing bacteria. Water Sci. Technol. 81 (9), 1797–1827. doi:10.2166/wst.2020.227
Xu, Y., Wang, C., Hou, J., Wang, P., You, G., Miao, L., et al. (2017). Application of zero valent iron coupling with biological process for wastewater treatment: A review. Rev. Environ. Sci. Biotechnol. 16 (4), 667–693. doi:10.1007/s11157-017-9445-y
Yang, L., Hu, J., Wu, W., Tang, J., Ding, K., and Li, J. (2016). In situ NH2-functionalized graphene oxide/SiO2 composites to improve Cu(II) removal from ammoniacal solutions. Chem. Eng. J. 306, 77–85. doi:10.1016/j.cej.2016.07.035
Yang, X., Hu, L., Bai, J., Mao, X., Chen, X., Wang, X., et al. (2021). Increased structural defects of graphene oxide compromised reductive capacity of ZVI towards hexavalent chromium. Chemosphere 277, 130308. doi:10.1016/j.chemosphere.2021.130308
Yang, Y., Wu, W., Zhou, H., Huang, Z., Ye, T., Liu, R., et al. (2014). Adsorption behavior of cross-linked chitosan modified by graphene oxide for Cu(II) removal. J. Cent. South Univ. 21 (7), 2826–2831. doi:10.1007/s11771-014-2246-3
Yang, Z., Dong, Y., and Li, Z. (2020). “Acid mine drainage remediation with simulated permeable reactive barrier filled with fly ash and its composite materials as reactive media,” in Energy sources, Part A: Recovery, utilization, and environmental effects, 1–14. doi:10.1080/15567036.2020.1801901
Yi, N., Wu, Y., Fan, L., and Hu, S. (2019). Remediating Cd-contaminated soils using natural and chitosan-introduced zeolite, bentonite, and activated carbon. Pol. J. Environ. Stud. 28 (3), 1461–1468. doi:10.15244/pjoes/89577
Yousefi, A., and Matavos-Aramyan, S. (2018). Mix design optimization of silica fume-based pervious concrete for removal of heavy metals from wastewaters. Silicon 10 (4), 1737–1744. doi:10.1007/s12633-017-9663-5
Yu, Q., Guo, J., Muhammad, Y., Li, Q., Lu, Z., Yun, J., et al. (2020). Mechanisms of enhanced hexavalent chromium removal from groundwater by sodium carboxymethyl cellulose stabilized zerovalent iron nanoparticles. J. Environ. Manag. 276, 111245. doi:10.1016/j.jenvman.2020.111245
Zawierucha, I., and Nowik-Zajac, A. (2019). Evaluation of permeable sorption barriers for removal of Cd(II) and Zn(II) ions from contaminated groundwater. Water Sci. Technol. 80 (3), 448–457. doi:10.2166/wst.2019.288
Zeng, H., Lu, L., Gong, Z., Guo, Y., Mo, J., Zhang, W., et al. (2019). Nanoscale composites of hydroxyapatite coated with zero valent iron: Preparation, characterization and uranium removal. J. Radioanal. Nucl. Chem. 320 (1), 165–177. doi:10.1007/s10967-019-06451-7
Zeng, Q., Huang, Y., Huang, L., Hu, L., Xiong, D., Zhong, H., et al. (2020). Efficient removal of hexavalent chromium in a wide pH range by composite of SiO2 supported nano ferrous oxalate. Chem. Eng. J. 383, 123209. doi:10.1016/j.cej.2019.123209
Zhang, F., Wang, X., Xionghui, J., and Ma, L. (2016). Efficient arsenate removal by magnetite-modified water hyacinth biochar. Environ. Pollut. 216, 575–583. doi:10.1016/j.envpol.2016.06.013
Zhang, J., Yang, X., Shi, J., Zhao, M., Yin, W., Wang, X., et al. (2022). Carbon matrix of biochar from biomass modeling components facilitates electron transfer from zero-valent iron to Cr(VI). Environ. Sci. Pollut. Res. 29 (16), 24309–24321. doi:10.1007/s11356-021-17713-x
Zhang, Q., Hou, Q., Huang, G., and Fan, Q. (2020). Removal of heavy metals in aquatic environment by graphene oxide composites: A review. Environ. Sci. Pollut. Res. 27 (1), 190–209. doi:10.1007/s11356-019-06683-w
Zhang, W., Dong, Y., Wang, H., Guo, Y., Zeng, H., and Zan, J. (2021). Removal of uranium from groundwater using zero-valent-iron coated quartz sands. J. Radioanal. Nucl. Chem. 327 (1), 1–12. doi:10.1007/s10967-020-07523-9
Zhang, W., Qian, L., Ouyang, D., Chen, Y., Han, L., and Chen, M. (2019). Effective removal of Cr(VI) by attapulgite-supported nanoscale zero-valent iron from aqueous solution: Enhanced adsorption and crystallization. Chemosphere 221, 683–692. doi:10.1016/j.chemosphere.2019.01.070
Zhang, Y., Li, Y., Li, J., Sheng, G., Zhang, Y., and Zheng, X. (2012). Enhanced Cr(VI) removal by using the mixture of pillared bentonite and zero-valent iron. Chem. Eng. J. 185-186, 243–249. doi:10.1016/j.cej.2012.01.095
Zhao, X., Liu, W., Cai, Z., Fu, J., Duan, J., Zhao, D., et al. (2020). Reductive immobilization of uranium by stabilized zero-valent iron nanoparticles: Effects of stabilizers, water chemistry and long-term stability. Colloids Surfaces A Physicochem. Eng. Aspects 604, 125315. doi:10.1016/j.colsurfa.2020.125315
Zhou, Y., Gao, B., Zimmerman, A. R., Chen, H., Zhang, M., and Cao, X. (2014). Biochar-supported zerovalent iron for removal of various contaminants from aqueous solutions. Bioresour. Technol. 152, 538–542. doi:10.1016/j.biortech.2013.11.021
Zhou, Y., Gao, B., Zimmerman, A. R., Fang, J., Sun, Y., and Cao, X. (2013). Sorption of heavy metals on chitosan-modified biochars and its biological effects. Chem. Eng. J. 231, 512–518. doi:10.1016/j.cej.2013.07.036
Zhu, F., Li, L., Ren, W., Deng, X., and Liu, T. (2017). Effect of pH, temperature, humic acid and coexisting anions on reduction of Cr(Ⅵ) in the soil leachate by nZVI/Ni bimetal material. Environ. Pollut. 227, 444–450. doi:10.1016/j.envpol.2017.04.074
Keywords: reaction medium, permeable reactive barrier, heavy metals, removal mechanism, groundwater
Citation: Li H and Liu Q (2022) Reaction medium for permeable reactive barrier remediation of groundwater polluted by heavy metals. Front. Environ. Sci. 10:968546. doi: 10.3389/fenvs.2022.968546
Received: 14 June 2022; Accepted: 08 August 2022;
Published: 06 September 2022.
Edited by:
Chengcheng Li, China University of Geosciences Wuhan, ChinaReviewed by:
Jian Liu, Southwest Jiaotong University, ChinaCopyright © 2022 Li and Liu. This is an open-access article distributed under the terms of the Creative Commons Attribution License (CC BY). The use, distribution or reproduction in other forums is permitted, provided the original author(s) and the copyright owner(s) are credited and that the original publication in this journal is cited, in accordance with accepted academic practice. No use, distribution or reproduction is permitted which does not comply with these terms.
*Correspondence: Hong Li, bGlob25nMDkxMEAxMjYuY29t
Disclaimer: All claims expressed in this article are solely those of the authors and do not necessarily represent those of their affiliated organizations, or those of the publisher, the editors and the reviewers. Any product that may be evaluated in this article or claim that may be made by its manufacturer is not guaranteed or endorsed by the publisher.
Research integrity at Frontiers
Learn more about the work of our research integrity team to safeguard the quality of each article we publish.