- 1Department of Marine Biology, Texas A&M University at Galveston, Galveston, TX, United States
- 2Institute of Environment, Florida International University, Miami, FL, United States
- 3Institute of Ecology and Evolutionary Biology, National Taiwan University, Taipei, Taiwan
Compensatory wetland restoration is a critical component of holistic, ecosystem-level oil spill response strategies. An important goal of restoration is to rehabilitate food webs in impacted areas, but faunal assemblages and trophic dynamics are rarely included in post-restoration monitoring or assessments of success. Different approaches to wetland restoration, including variations in construction technique, may influence faunal recovery and trophic interactions. To explore these dynamics, we compared emergent plant communities, terrestrial arthropod assemblages, and trophic interactions in restored emergent marshes that were constructed in terrace and mound configurations and in reference areas in the Lower Neches Wildlife Management Area (Texas, United States). Plant community composition differed among all habitat types, with higher diversity on terraces and in reference marshes. Terrestrial arthropod abundance was similar among habitat types, but species composition was distinctly different among habitat types, especially at the herbivore level, where four of the eight herbivore species were found in either reference or restored sites, but not in both habitat types. Herbivores (primarily beetles and planthoppers) were more abundant than detritivores (midges and flies) in all habitat types. Predator (web-building and hunting spiders) abundance and species identity were similar among habitat types. Based on stable isotope analysis, trophic relationships differed among mounds, terraces, and reference areas. Herbivore diets were more variable at the reference sites than in either restored habitat type, aligning with higher plant diversity in reference areas. In contrast, detritivore diets were more variable at restored sites, where they were likely consuming food sources such as benthic algae or sediment organic matter. Predator diets were primarily comprised of herbivores in reference areas and detritivores in restored habitats. Overall, the restoration approaches supported abundant terrestrial arthropod assemblages, but species composition was different. In addition, trophic relationships differed between restored and reference areas, in part due to unique plant species assemblages at restored and reference sites. These results suggest that ecosystem restoration strategies that introduce geomorphological heterogeneity and plant diversity are more likely to support a diverse array of species and functions.
Introduction
Coastal wetlands in the continental United States have been substantially reduced in area over the past 100 years (Dahl, 1990), primarily due to urban and agricultural development, hydrological alterations, and natural and anthropogenic subsidence (Mitsch and Hernandez, 2012). Although the rate of loss has slowed in recent years (Entwistle et al., 2018; Murray et al., 2022), coastal wetlands continue to be vulnerable to disturbance from development, storm events, and offshore oil spills (Morton and Barras, 2011; Turner et al., 2016; Hu et al., 2017). Oil spills have received extensive public attention, with acute and long-term effects across all trophic levels, from plants and insects to iconic megafauna (McCall and Pennings, 2012; Silliman et al., 2012; Haney et al., 2014; Lin et al., 2016; Zengel et al., 2016; Zengel et al., 2022). These highly visible impacts make it critical for state management agencies to have robust, multi-faceted, and effective oil spill response plans that address ecosystem recovery across multiple trophic levels (French McCay and Rowe, 2003). Compensatory wetland restoration that considers a range of ecosystem attributes is a critical long-term component of these response plans (Simenstad and Thom, 1996; Armitage, 2021).
Restoration efforts often focus on the creation of vegetated, low elevation marsh habitat that is of high near-term value to fisheries (e.g., La Peyre et al., 2007; Zeug et al., 2007). In the northern Gulf of Mexico, these projects frequently include mound or terrace formations (Rozas et al., 2005) (Figure 1) that create marsh edge habitat. Despite differences in plant species identity and biomass (Garbutt and Wolters, 2008; Mossman et al., 2012; Staszak and Armitage, 2013), previous investigations of ecosystem-level functions in these types of restored marshes indicate that many metrics, such as aquatic faunal density and carbon sequestration potential, can be similar among mound and terrace formations but are sensitive to the amount of aquatic habitat surrounding the emergent marsh features (Rozas et al., 2005; Madrid et al., 2012; Armitage et al., 2014).
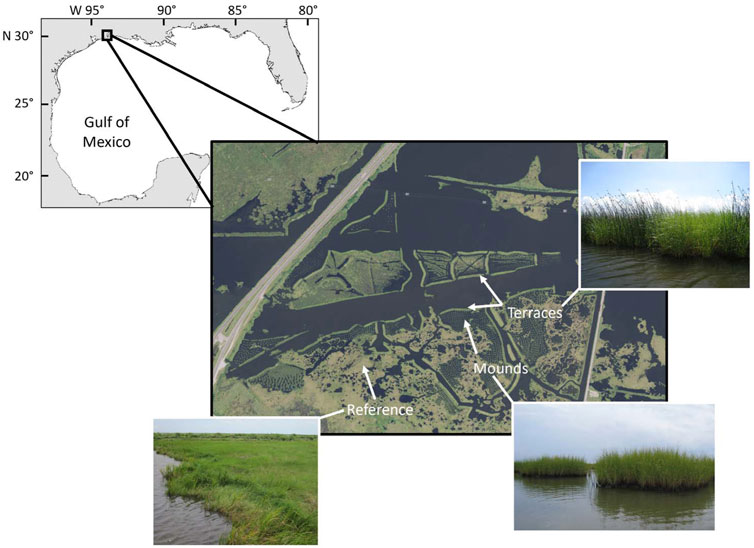
FIGURE 1. Map of study area in the Lower Neches Wildlife Management Area, depicting examples of restored mounds (planted with Spartina alterniflora), restored terraces (planted with Spartina alterniflora and Schoenoplectus californicus), and reference areas (primarily S. alterniflora, Spartina patens, and Bolboschoenus robustus). Inset on upper left indicates study area location in the northern Gulf of Mexico. Aerial image obtained from the United States Department of Agriculture (USDA); Texas NAIP Imagery, 2016–12-15.
An equally important but frequently overlooked goal of compensatory restoration, particularly from public and commercial perspectives, is to rehabilitate impacted wildlife and the food webs that support them. However, the response of lower trophic levels to compensatory restoration is usually assumed but seldom quantified and rarely considered in assessments of restoration success (Weinstein et al., 2005; Loch et al., 2020). In coastal marshes, terrestrial arthropods (insects, spiders, and their kin) are important grazers and prey items in food webs (Post and Greenlaw, 2006; Batzer and Wu, 2020; Ning et al., 2021). Many of these organisms are closely associated with marsh plants, and so faunal use of restored areas may also be affected by marsh plant identity (Talley and Levin, 1999; Wu et al., 2008). For example, insect diversity and abundance are often lower in wetlands invaded by Spartina alterniflora (Ning et al., 2021). Conversely, restoration of S. alterniflora within its native range can increase arthropod abundance and diversity (Gratton and Denno, 2005). For generalist species, plant identity may be less important than plant cover or density (Guiden et al., 2021), though plant structure may be linked to habitat suitability for some predators such as web-building spiders. In some cases, terrestrial arthropod assemblages and trophic interactions recover following habitat restoration (Gratton and Denno, 2006; Watts and Didham, 2006), but in others, food webs can be slow to approach reference conditions (Wozniak et al., 2006; Ning et al., 2021; Wu et al., 2021). On a decadal scale, the character of the food web changes over time, shifting from detrital-based to herbivore-driven as the restored site ages (Schrama et al., 2012).
Despite the large number of restoration projects on the northern Gulf of Mexico coastline, there have been few quantitative comparisons of the values of different construction and planting techniques for associated wildlife at lower trophic levels (but see Madrid et al., 2012; Armitage et al., 2014). Restoration projects on the upper Texas coast have used various combinations of these approaches, providing a unique opportunity to quantify food web development in response to a range of restoration techniques. Understanding food web structure in restored habitats will help resource managers and oil spill response technicians identify appropriate compensatory restoration techniques that are tailored to the taxa or resources that were impacted, maximizing the efficient use of oil spill response funds. Therefore, our broad objective was to identify compensatory wetland restoration techniques that boosted food web development. In this case study within a restored wetland in the early successional stages, we focused on terrestrial arthropod responses to three key aspects of restoration project design: construction type (terraces or mounds), plant species identity, and plant species diversity. Given the young age of the study site, we expected that detritivores would dominate the food web in restored areas (sensu Schrama et al., 2012).
Materials and methods
Study area
We conducted this study in the Lower Neches Wildlife Management Area (latitude 30o 0′ 5″ N, longitude 93o 51’ 49” W), Texas, United States (Figure 1). The study area consisted of reference (not actively planted or amended) and restored brackish marshes, where two types of restored marsh structures, mounds and terraces, were created in spring 2008. Both mounds and terraces were constructed with material excavated from adjacent bottom sediment. Mounds were circular formations (10–20 m in diameter) and were planted with the smooth cordgrass, Spartina alterniflora cv. Vermilion. Terraces were long, narrow structures (5 m x > 100 m) that were planted with Spartina alterniflora cv. Vermilion and Schoenoplectus californicus. Each of the three habitat types (reference, mounds, terraces) were distinct from each other (separated by at least 100 m) but were within the same hydrological management unit and experienced similar tidal influence. More detailed information about the study site and restoration project was reported by Armitage et al. (2014).
Plant and arthropod sampling
To examine whether reference and restored marshes differed in trophic structure, in summer 2010, we sampled five replicate sites within each of the three habitat types: reference sites, restored mounds, and restored terraces. Replicate sites were at least 30 m apart from each other. Sampling in these habitats provided an opportunity to examine the effect of different restoration configurations (i.e., mounds vs terraces) on plant and arthropod communities and food web structure.
The aboveground biomass of each emergent marsh plant species in each habitat type was determined by clipping all stems at the ground level in representative 10 cm x 20 cm quadrats at each site (n = 5 per habitat type). All plant samples were collected over a 2-day period in June 2010. Stems were rinsed, dried, and weighed to determine biomass (kg/m2). Additional detail on plant community characterization is described in Armitage et al. (2014). For isotopic analyses, we collected fresh plants and plant detritus from each sampling site, all of which were immediately preserved in coolers with ice. Focal species included the dominant plants in each habitat, such as S. alterniflora, Spartina patens, and Bolboschoenus robustus in reference sites, S. alterniflora cv. Vermilion on mounds, and S. alterniflora cv. Vermilion and S. californicus on terraces. For each plant species, we haphazardly collected 6 leaves at each site for stable isotopic analysis. For plant detritus, it was infeasible to identity to species level and therefore detritus samples were pooled within sampling sites.
To characterize arthropod assemblages, we used an insect vacuum fitted with a sampling head (7 cm in diameter), haphazardly selected a starting point within a sampling site, and vacuumed all arthropods from the bottom to the top of vegetation cylinder (13 cm in diameter). We sampled 10 non-overlapping vegetation cylinders per sampling site; all arthropods were collected over a 2-day period in July 2010. This sampling method is effective for capturing species directly associated with the vegetation (Buffington and Redak, 1998). Samples were placed on ice for transport to the lab for identification and processing. Arthropods were identified to the lowest practical taxonomic level. When possible, arthropods were assigned to a trophic level (herbivore, detritivore, or predator) based on the literature and identification guides (Arnett Jr, 2000; Gratton and Denno, 2005).
Stable isotope analysis
To examine trophic linkages among species in the restored wetlands, we analyzed the stable carbon and nitrogen isotope ratios (δ13C and δ15N) of plants, detritus, and common arthropods (herbivores, detritivores, predators) from each habitat type. Plant and detritus samples collected from the field were rinsed with water at the laboratory before stable isotope analysis. All samples were dried at 60°C for 48 h and ground using a mortar and pestle or electric mill. Plant samples from each site were analyzed separately for each species, and detritus was pooled within sampling sites, since detrital material could not be identified to species level. We pooled arthropod species from the same trophic level at each sampling site to provide enough material for stable isotope analysis; this approach yielded results that reflected the overall signature of each trophic level within each habitat type. Herbivores in our analysis included Ischnodemus conicus (Family Blissidae), Megamelus sp. and Prokelisia sp. (Family Delphacidae), and unidentified members of Families Chrysomelidae, Curculionidae, Elachistidae, Membracidae, and Miridae. Detritivores included members of Families Chironomidae, Drosophilidae, and Ephydridae. Predators included web-building and hunting spiders (Families Araneidae, Lycosidae, Salticidae, Tetragnathidae, and Thomisidae). To reduce analysis bias introduced by various fat content in animals (Post et al., 2007), we treated all arthropod samples with lipid extraction using an Accelerated Solvent Extractor (Dionex) at Texas A&M University at Galveston, Texas, United States. All plant and animal samples were sent to the University of California Davis Stable Isotope Facility for stable isotopic analysis of carbon (C) and nitrogen (N), using a PDZ Europa ANCA-GSL elemental analyzer interfaced to a PDZ Europa 20–20 isotope ratio mass spectrometer. Vienna Pee Dee Belemnite and air were used as standards for carbon and nitrogen, respectively.
Statistical analyses
Total plant biomass was analyzed with one-way univariate PERMANOVA following square root transformation using the adonis2 routine in the vegan package in R version 4.2.0 (R Core Team, 2020), where habitat type (reference, mounds, or terraces) was the independent variable. Plant assemblages were analyzed with two-way multivariate PERMANOVA, where species identity and habitat type were the independent variables. Arthropod abundances were analyzed with separate one-way PERMANOVA for each functional group (herbivores, detritivores, predators), where habitat type was the independent variable.
Stable isotope values were used to evaluate trophic relationships for herbivores and predators in each of the three habitat types using Bayesian mixing models in the package MixSIAR (v 3.1.12, Stock et al., 2018) in R version 4.1.2 (R Core Team, 2020). We were not confident that we fully sampled all likely food sources for detritivores; therefore mixing models were not performed for this trophic group. Each model was run in three chains with 1,000,000 iterations and a burn-in of 500,000 and was thinned by 500 each iteration. For herbivores, different end members (food sources) were available in restored and reference sites, so separate models were run in each habitat type. For predators, end members were the same at all sites, so a single model was run to determine the contribution of herbivores and detritivores to predator diets in each habitat type. The biomass (of plants) or the abundance (of herbivores and detritivores) of each dietary source within each habitat type was incorporated into each model as an informative prior. Detrital biomass was not recorded, but based on studies in similar climate zones (Netto and Lana, 1999), it was assumed to be equivalent to live biomass of the species present at each sampling site. Spartina alterniflora and S. patens were not isotopically distinct from each other, so in the reference habitat type where both plants were present, they were combined into a single Spartina source in the model as recommended by MixSIAR. We used trophic discrimination factors of 0.4 ± 1.3 for carbon and 2.0 ± 1.8 for nitrogen as suggested by McCutchan et al. (2003) for terrestrial arthropod consumers.
To supplement the mixing model outputs, we constructed dual isotope graphs for visual assessments of trophic relationships. In addition, we analyzed absolute differences in δ13C and δ15N values for all three trophic groups among habitat types with one-way univariate PERMANOVA as described above.
Results
Plants
Marsh plant biomass was 50–100% higher in restored (mounds 1.9 ± 0.3 kg m−2; terraces 2.9 ± 0.3 kg m−2) than in reference areas (1.3 ± 0.2 kg m−2; PERMANOVA df = 2, F = 5.58, p = 0.007). The distribution of biomass among species differed among habitat types (Figure 2; PERMANOVA df = 2, F = 6.42, p = 0.002). In restored mounds and in reference sites, S. alterniflora was the dominant species. In restored terraces, S. alterniflora and S. californicus were co-dominant. Small amounts of two other species, S. patens and B. robustus, were found in reference areas (Figure 2).
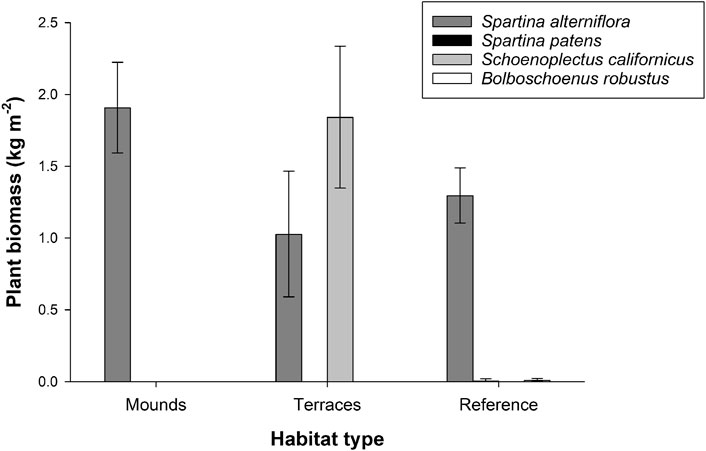
FIGURE 2. Plant biomass at two restored habitat types (mounds and terraces) and in reference areas. Error bars depict standard error.
Arthropods
Arthropod abundance tended to be lower on terraces than on mounds or in reference areas, but there was high variability within habitat types and no statistical difference among any restored or reference areas (Herbivore PERMANOVA df = 2, F = 1.25, p = 0.321; Detritivores df = 2, F = 1.25, p = 0.322; Predators df = 2, F = 1.93, p = 0.179). Herbivores were more abundant than detritivores at all sites and had similar abundances as predators (Figure 3). Common herbivores included typical salt marsh taxa such as Ischnodemus conicus (true bugs, Family Blissidae) and Prokelisia spp. (planthoppers, Family Delphacidae) (Table 1). Detritivores were less abundant and had lower species richness than herbivores, primarily comprised of cosmopolitan consumers of decaying plant material (Table 1). Predators were web-building and hunting spiders (Table 1).
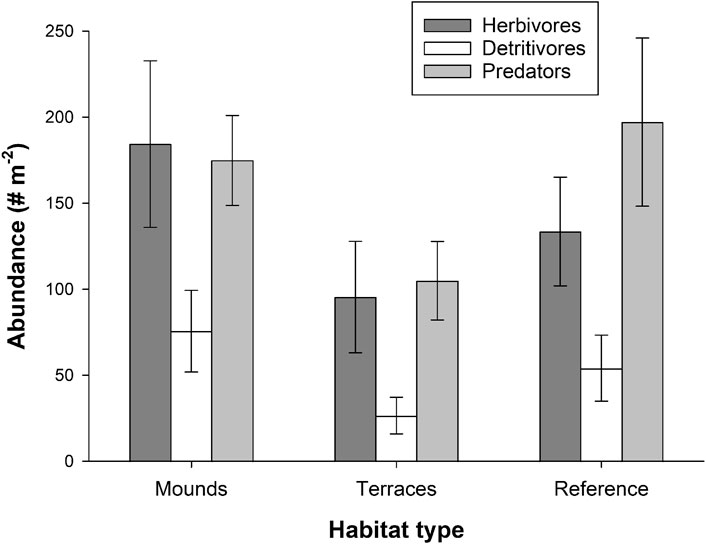
FIGURE 3. Abundance of terrestrial arthropod functional groups at two restored habitat types (mounds and terraces) and in reference areas. Error bars depict standard error.
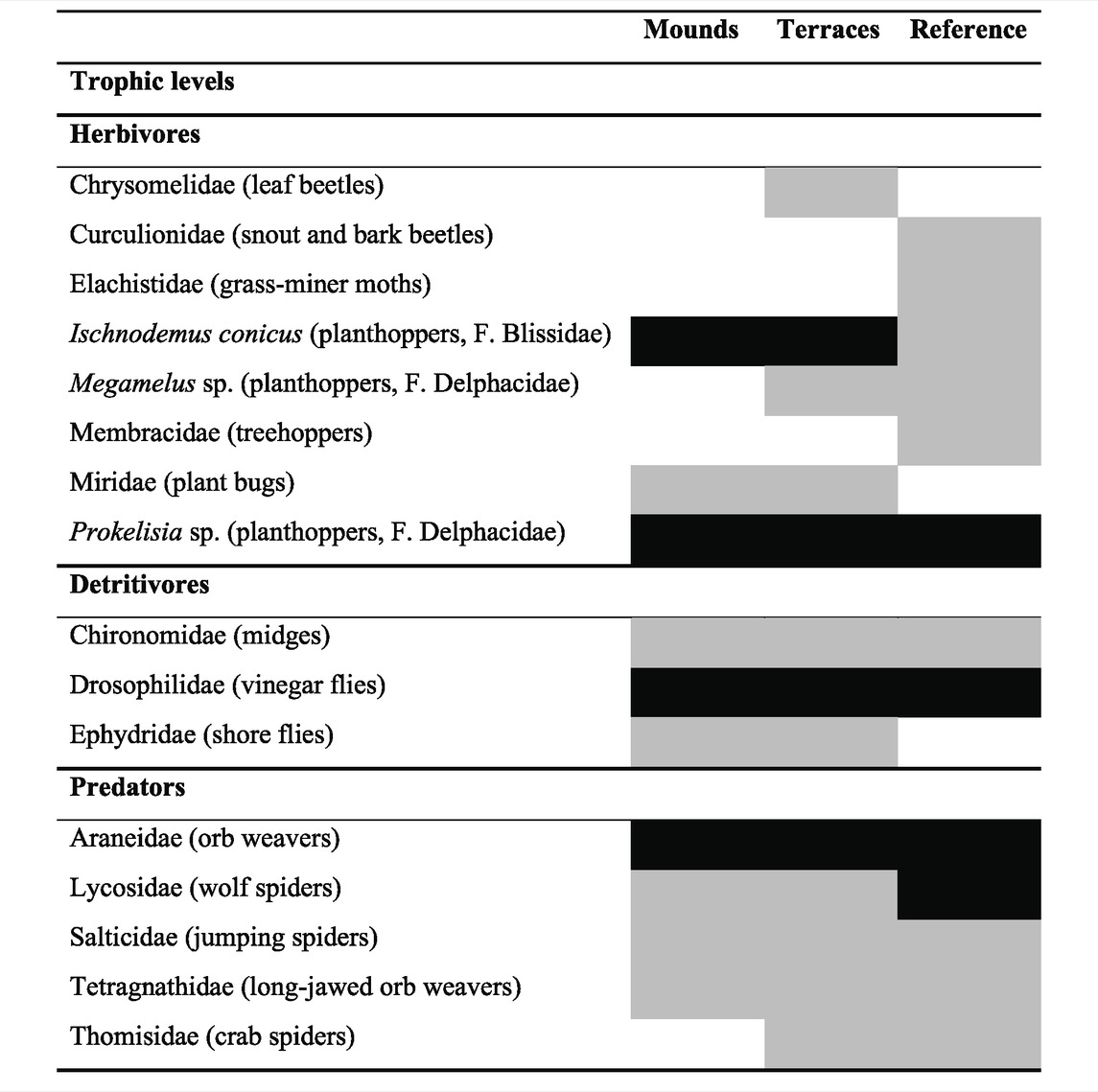
TABLE 1. Relative abundance of terrestrial arthropod taxa collected in each habitat type (restored mounds and terraces, reference marsh). In most cases, identification was made to family level; when individuals could be identified to species, common names and families are indicated in parentheses. Black indicates common taxa (>5 m−2), grey indicates uncommon (<5 m−2), and white indicates that taxa was absent from that habitat type.
Trophic relationships
Herbivore diets were more variable at the reference sites than in either restored habitat type (Figure 4). The dominant herbivore food source in all habitat types was S. alterniflora (or a combination of the two Spartina species at the reference sites) (Table 2). The mixing models suggested that detritus comprised a substantial portion of herbivore diets on mounds, but the mixing models may not have been able to differentiate between live and detrital S. alterniflora contributions (Figure 4A). Herbivore δ13C signatures differed among habitat types but δ15N signatures were consistent across sites (Table 3). Herbivore δ13C (ranging from -11 to -13.5‰) was closely aligned with S. alterniflora signatures in both restored habitats (Figure 4). In reference sites, herbivore δ13C signatures were more variable (ranging from -12 to -26‰), reflecting a wider array of food sources such as B. robustus) or unsampled food sources (e.g., aquatic vegetation or algae).
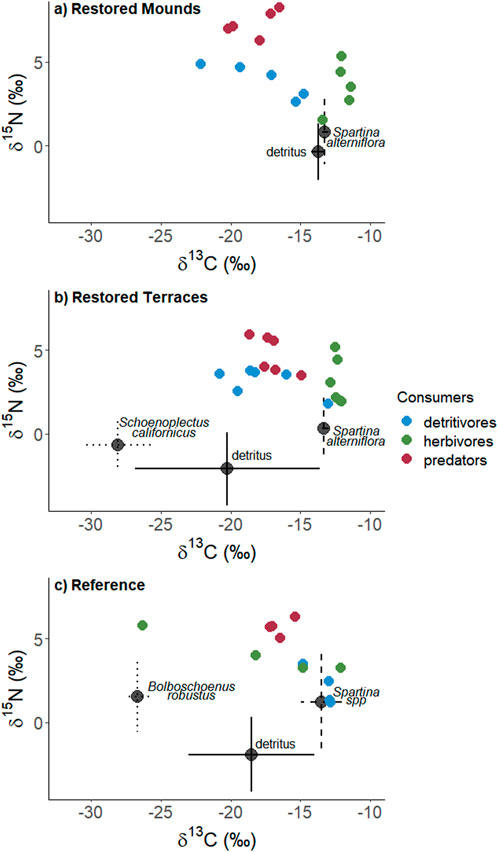
FIGURE 4. Dual isotope graphs of consumers and food sources in (A) restored mounds, (B) restored terraces, and (C) reference sites. Error bars depict standard deviation.
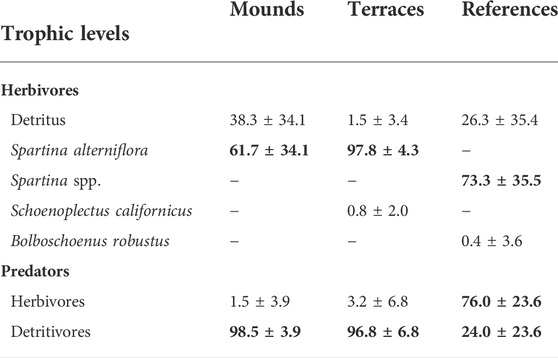
TABLE 2. Mixing model estimates of end member contributions to herbivore and predator diets in reference and restored (mound or terrace) marshes (mean ± SD). Bolded values indicate the primary contributor to the diet, and blank cells indicate that a source did not occur in a given habitat type.
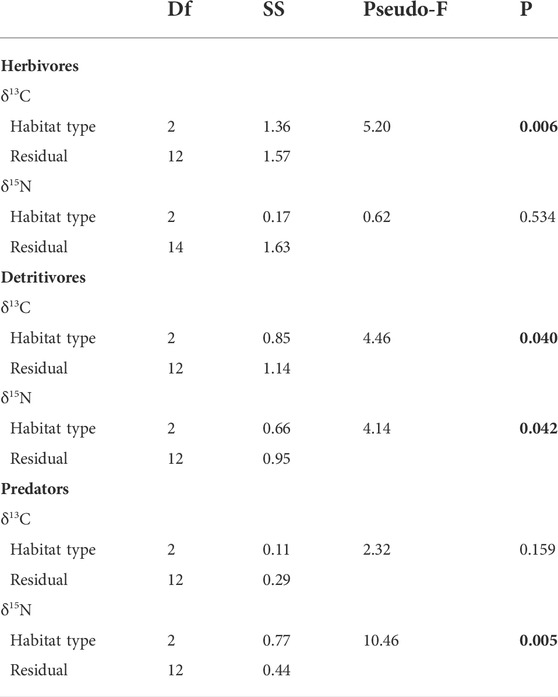
TABLE 3. Results from one-way PERMANOVA of absolute differences in δ13C and δ15N values for three trophic groups among reference and restored (mound or terrace) habitat types. p-values less than 0.05 are indicated in bold type.
Detritivore δ13C and δ15N signatures varied among habitat types (Table 3). In restored mounds and terraces, δ13C signatures indicated that detritivore diets were variable, ranging from -13 to -22‰ (Figure 4). Detritivore isotopic ratios at mounded sites frequently fell outside the range of collected end member values, indicating the presence of additional food sources (e.g., benthic algae or sediment organic matter) that were not sampled; these missing end members prevented the accurate calculation of diet contributions with mixing models. In reference areas, detritivore δ13C signatures were less variable (ranging from -13 to -15‰) and were more closely aligned with Spartina spp. signatures (Figure 4). Detritivore δ15N signatures were lower in reference areas (average 2.1‰ ± 0.5 SE) than on mounds (3.9‰ ± 0.4) or terraces (3.2‰ ± 0.3), indicating that detritivores were consuming a more enriched organic end member in restored areas.
Predators showed a clear dietary shift with restoration (Table 2). Predator δ13C signatures were similar in all habitat types but were more closely aligned with detritivores in restored habitat types and with herbivores in the reference areas (Table 3; Figure 4). Predator δ15N signatures were enriched on mounds (average 7.3‰ ± 0.3 SE), relative to terraces (4.8‰ ± 0.4) and reference areas (5.7‰ ± 0.3) (Table 3). The higher predator δ15N signature on mounds corresponded with higher detritivore δ15N signatures in those areas.
Discussion
Despite some high-level similarities between restored and reference areas in terms of plant biomass and cover and terrestrial arthropod abundance (Figures 2, 3; Armitage et al., 2014), there were clear distinctions in species composition and trophic relationships in the restored sites. Terrestrial arthropod assemblages are notoriously heterogeneous over space and time (David et al., 2016; Hacala et al., 2019), but abundance does tend to be linked to plant assemblage characteristics. In habitats ranging from tidal marshes to terrestrial grasslands, restored sites with plant communities that resemble reference areas are more likely to develop similar faunal communities (Mortimer et al., 2002; Gratton and Denno, 2005; Gerber et al., 2008; Watts et al., 2008; Moir et al., 2010). Accordingly, the successful development of food webs in restored habitats is closely linked to plant and animal species identity (Gratton and Denno, 2006; Watts and Didham, 2006).
Based on the similarity in arthropod abundance between restored and reference areas, and the relatively young age (2 years) of the restored sites, it appears that terrestrial arthropods quickly colonized the restored areas. However, abundance metrics do not tell the full story about the restoration of arthropod assemblages (Burkhalter et al., 2013; Maoela et al., 2016; Hacala et al., 2019). In fact, arthropod taxonomic identities were distinctly different among habitat types, especially among herbivores. Four of the eight herbivore species were found in either reference or restored sites, but not in both types of sites. Only two herbivorous genera were found in all three habitat types; both of those species are known to be closely associated with S. alterniflora: the sap-feeder Prokelisia and the planthopper Ischnodemus (Denno and Grissell, 1979; Slater and Baranowski, 1990). Predator and detritivore assemblages were more similar between restored and reference areas, with four out of five predators and two out of three detritivores found in all habitat types. Notably, arthropod assemblages in restored areas were not dominated by detritivores as expected, despite the young age of the restored sites (Schrama et al., 2012). Asynchronous recovery of different trophic levels in arthropod assemblages is influenced by many factors, including dispersal ability, food availability for specialist species, and abiotic conditions, as well as plant identity and characteristics (Gratton and Denno, 2006; Simao et al., 2010; Dibble et al., 2013; Pétillon et al., 2014).
Emergent plant species identities varied among habitat types, with the fewest species in mounded areas and the most in reference areas. This variation in species composition was reflected in biomass and cover measures (Figure 2; Armitage et al., 2014) as well as in the detrital isotopic signatures (Figure 4). These results are typical in restored wetlands, where differences in plant species identity and biomass can persist for many years (Garbutt and Wolters, 2008; Mossman et al., 2012; Staszak and Armitage, 2013). In this study area, the emergent plant communities in restored areas were intentionally different from the reference area, and from each other. The goal of planting in coastal wetland restoration is typically to rapidly generate a plant canopy that meets some cover level stipulated in the associated permit (Cole and Shafer, 2002). Thus, the primary plant species in the restored sites (S. alterniflora) was chosen to jump-start plant canopy restoration, despite the relative rarity of S. alterniflora in other portions of the reference area, where S. patens and B. robustus dominated in terms of cover (Armitage, 2021). Although S. alterniflora was not abundant throughout the reference area, the Vermilion strain selected was a particularly robust variant that grows much taller than local strains (Fine et al., 2000). Thus, even at low abundance, S. alterniflora comprised a substantial portion of the emergent plant biomass at our study sites and was therefore a potentially important and readily available end member for both herbivores and detritivores in all habitat types.
The food sources supporting the terrestrial arthropod food web likely included more than just emergent marsh plants. In young restored sites, detritus can play a particularly important role in food web support (Schrama et al., 2012). In addition, marine subsidies can make substantial contributions to arthropod food webs in tidal wetlands (Paetzold et al., 2008), and aquatic vegetation, algae, and particulate organic matter were abundant in all habitat types (Armitage et al., 2014), though these sources likely varied in importance for different consumer groups. Accordingly, the variation in detrital δ13C signatures in terraced and reference habitat types indicated that there was a wide array of organic matter sources available to consumers. These alternate food sources were not sampled in this study, preventing the use of mixing models to specifically characterize their importance in consumer diets. Regardless, consumer isotopic signatures can be useful in making relative comparisons in trophic relationships among habitat types, and provide insight into how similar (or dissimilar) the restored areas are to target reference conditions.
In general, isotopic signatures indicated that consumer diets differed between restored and reference areas, but each consumer group showed unique patterns. Herbivore dietary diversity was relatively low in restored habitat types, with δ13C signatures closely clustered near S. alterniflora values. Although the mixing models suggested that detritus comprised a portion of herbivore diets on restored mounds, the detrital and live S. alterniflora δ13C signatures were very close together, suggesting that the mixing models were unable to differentiate between live plant and detrital contributions. Based on the natural history of two common herbivores at this site (Armitage et al., 2013), it is unlikely that detritus was an important component of herbivore diets. Prokelisia is a sap-feeding specialist on S. alterniflora (Denno and Grissell, 1979) and Ischnodemus conicus typically lives cryptically under S. alterniflora basal leaf sheaths (Slater and Baranowski, 1990). Model outputs suggesting detrital consumption may have also been confounded by the sap-sucking nature of Prokelisia. Sap-suckers tend to have different trophic discrimination factors than insects that consume leaf tissue (McCutchan et al., 2003) but there was no way to separate out the two feeding methods in the mixing models. Evidence of strong herbivore affiliations with S. alterniflora was clear in terraced sites, where S. californicus was also an abundant plant, but δ13C signatures indicated that herbivores avoided that species in favor of S. alterniflora. In the reference areas, a wider array of available emergent plant species corresponded with a wider spread (and lower absolute average) in herbivore δ13C values, indicating more diet heterogeneity.
In contrast to herbivores, detritivore diet diversity was highest, as indicated by a wider spread in δ13C values, in restored areas. Detritivore isotopic signatures were similar in mounds and terraces, despite differences in the signatures of available detritus. Detrital signatures in restored mounds were closely aligned with S. alterniflora. In contrast, the detritus on terraces was likely a mix between the two abundant plant species, S. alterniflora and S. californicus. Additional detritus from subtidal sources may have accumulated on terraces but not on mounds due to their smaller size and lower elevation profile. To some degree, detritivores in both sites were likely consuming some detrital vascular plant material, but unsampled end members were also likely to be relevant diet components in both restored areas. In particular, sediment organic matter may have been an important (but unsampled) end member for these consumers (Page et al., 2010). In contrast to restored areas, detritivores in reference areas appeared to have distinctly different diets, as detritivore signatures were closely aligned with S. alterniflora, suggesting that those taxa may have primarily consumed detritus from that plant species.
Based on isotope signatures and mixing models, predator diets were more closely aligned with detritivores in restored areas and herbivores in reference areas. The spider taxa in Gulf Coast tidal marsh ecosystems are typically generalists, utilizing either webs or active hunting strategies (Gratton and Denno, 2005). Some predatory taxa in these tidal ecosystems may engage in intraguild predation (Matsumura et al., 2004), but based on isotopic signatures, either herbivores or detritivores comprised the bulk of predator diets. As such, shifts in predator diets following restoration likely tracked changes in abundance or biomass of prey items within these two consumer groups (Pétillon et al., 2005; Gratton and Denno, 2006). Although detritivores were not the most abundant group (Figure 3), some of the taxa in restored areas had relatively large body sizes (e.g., shore flies in Family Ephydridae), and their biomass may have been substantial enough to offset their relative rarity in the arthropod assemblage. The co-occurring herbivore species in restored sites tended to be more cryptic or stationary (Denno and Grissell, 1979; Slater and Baranowski, 1990), and thus were less susceptible to predation from either passive web builders or active hunters. In contrast, in reference areas, members of Family Ephydridae were absent and other smaller taxa in Families Chironomidae and Drosophilidae were more common. Accordingly, the biomass of detritivores available to predators in reference areas was lower, relative to restored areas.
Implications for restoration
The intent of compensatory coastal wetland restoration is to mitigate for structural or functional loss of habitat within the watershed, often following major disturbance events such as the Deepwater Horizon oil spill (Van den Bosch and Matthews, 2017; Zengel et al., 2021). The establishment of a specified level of emergent plant cover is a standard metric of restoration success (Ruiz-Jaen and Aide, 2005; Matthews and Endress, 2008). However, plant cover rarely corresponds with a broad spectrum of functional characteristics such as species diversity, nutrient cycling, or trophic energy flow (Craft et al., 1999; Zedler and Lindig-Cisneros, 2000; Levrel et al., 2012). In this study, plant and terrestrial arthropod communities were distinctly different between restored and reference areas, and those differences contributed to dissimilarity in food web structure among habitat types. Terrestrial arthropods play important roles in controlling productivity and supporting higher trophic levels, including fish and avian predators (Post and Greenlaw, 2006; Batzer and Wu, 2020; Ning et al., 2021). In some cases, terrestrial arthropod abundances rapidly re-establish following habitat restoration (Gratton and Denno, 2005; David et al., 2016), but restored assemblages are often dissimilar in terms of diversity and distribution among trophic guilds (Pétillon et al., 2014; Ning et al., 2021; Farfán-Beltrán et al., 2022). Restored areas with plant assemblages that are distinct from those in reference areas are especially likely to yield persistent dissimilarities in associated fauna (Talley and Levin, 1999; David et al., 2016; Leonard et al., 2021).
The broad objective of this study was to identify compensatory wetland restoration techniques that would boost food web development. We focused on terrestrial arthropod responses to three key aspects of restoration project design: construction type (terraces or mounds), plant species identity, and plant species diversity. The two construction types each yielded unique terrestrial arthropod assemblages and food web structure. In contrast to expectations, neither restored habitat type had a food web supported primarily by detritus. Furthermore, neither restored habitat type closely resembled reference conditions. The unique assemblages and trophic relationships within each habitat type were likely linked to differences in plant communities and localized geomorphology (this study; Rozas et al., 2005; Armitage et al., 2014). Although neither restored site successfully achieved absolute parity with the reference area, all three habitat types contributed to ecosystem-level diversity at the lower trophic levels. This outcome highlights the importance of integrating heterogeneity into ecosystem restoration design to boost a diverse array of species and functions (Rozas et al., 2005; Armitage et al., 2007; Pétillon and Garbutt, 2008; Madrid et al., 2012; Weisberg et al., 2012; Lengyel et al., 2020; Armitage, 2021). Restoration approaches that strategically include topographic and vegetation heterogeneity are more likely to achieve a broader range of ecosystem benefits.
Data availability statement
The raw data supporting the conclusion of this article will be made available by the authors, without undue reservation.
Author contributions
AA and C-KH contributed to conception and design of the study. C-KH collected the data. AA and JG performed the statistical analyses. AA wrote the first draft of the manuscript. All authors contributed to manuscript revision and approved the submitted version.
Funding
This study was funded in part by the Research and Development program of the Texas General Land Office Oil Spill Prevention and Response Division under Grant No. 09–060-000–3396. Funding support for publication costs was provided by the Ministry of Science and Technology (now National Science and Technology Council) to C.-K. Ho (108–2621-B-002–003-MY3).
Acknowledgments
Logistical support and access to the Old River Unit of the Lower Neches Wildlife Management Area were provided by the Wildlife Division of the Texas Parks and Wildlife Department. We thank the dedicated field crew from the Coastal and Wetlands Ecology Laboratory at TAMUG for their assistance. This is contribution #1489 from the Institute of Environment at Florida International University.
Conflict of interest
The authors declare that the research was conducted in the absence of any commercial or financial relationships that could be construed as a potential conflict of interest.
Publisher’s note
All claims expressed in this article are solely those of the authors and do not necessarily represent those of their affiliated organizations, or those of the publisher, the editors and the reviewers. Any product that may be evaluated in this article, or claim that may be made by its manufacturer, is not guaranteed or endorsed by the publisher.
References
Armitage, A. R., Ho, C.-K., Madrid, E. N., Bell, M. T., and Quigg, A. (2014). The influence of habitat construction technique on the ecological characteristics of a restored brackish marsh. Ecol. Eng. 62, 33–42. doi:10.1016/j.ecoleng.2013.10.021
Armitage, A. R., Ho, C. K., and Quigg, A. (2013). The interactive effects of pulsed grazing disturbance and patch size vary among wetland arthropod guilds. PLoS One 8 (10), e76672. doi:10.1371/journal.pone.0076672
Armitage, A. R., Jensen, S. M., Yoon, J. E., and Ambrose, R. F. (2007). Wintering shorebird assemblages and behavior in restored tidal wetlands in southern California. Restor. Ecol. 15 (1), 139–148. doi:10.1111/j.1526-100x.2006.00198.x
Armitage, A. R. (2021). Perspectives on maximizing coastal wetland restoration outcomes in anthropogenically altered ecosystems. Estuaries Coasts 44, 1699–1709. doi:10.1007/s12237-021-00907-4
Arnett, R. H. (2000). American insects: A handbook of the insects of America north of Mexico. Boca Raton, FL: CRC Press.
Batzer, D. P., and Wu, H. (2020). Ecology of terrestrial arthropods in freshwater wetlands. Annu. Rev. Entomol. 65, 101–119. doi:10.1146/annurev-ento-011019-024902
Buffington, M. L., and Redak, R. A. (1998). A comparison of vacuum sampling versus sweep-netting for arthropod biodiversity measurements in California coastal sage scrub. J. Insect Conserv. 2, 99–106. doi:10.1023/A:1009653021706
Burkhalter, J. C., Moon, D. C., and Rossi, A. M. (2013). Diversity and community similarity of arthropods in response to the restoration of former pine plantations. Southeast. Nat. 12 (1), 121–136. doi:10.1656/058.012.0110
Cole, C. A., and Shafer, D. (2002). Section 404 wetland mitigation and permit success criteria in Pennsylvania, USA, 1986-1999. Environ. Manag. 30 (4), 508–515. doi:10.1007/s00267-002-2717-4
Craft, C., Reader, J., Sacco, J. N., and Broome, S. W. (1999). TWENTY-FIVE years of ecosystem development of constructedspartina alterniflora(loisel) marshes. Ecol. Appl. 9 (4), 1405–1419. doi:10.1890/1051-0761(1999)009[1405:tfyoed]2.0.co;2
Dahl, T. E. (1990). Wetlands losses in the United States 1780s to 1980s. Washington, D.C.: U.S. Department of the Interior, Fish and Wildlife Service.
David, A. T., Goertler, P. A., Munsch, S. H., Jones, B. R., Simenstad, C. A., Toft, J. D., et al. (2016). Influences of natural and anthropogenic factors and tidal restoration on terrestrial arthropod assemblages in west coast North American estuarine wetlands. Estuaries Coasts 39 (5), 1491–1504. doi:10.1007/s12237-016-0091-3
Denno, R. F., and Grissell, E. E. (1979). The adaptiveness of wing-dimorphism in the salt marsh-inhabiting planthopper, Prokelisia marginata (Homoptera: Delphacidae),. Ecology 60 (1), 221–236. doi:10.2307/1936482
Dibble, K. L., Pooler, P. S., and Meyerson, L. A. (2013). Impacts of plant invasions can be reversed through restoration: A regional meta-analysis of faunal communities. Biol. Invasions 15 (8), 1725–1737. doi:10.1007/s10530-012-0404-9
Entwistle, C., Mora, M. A., and Knight, R. (2018). Estimating coastal wetland gain and losses in Galveston county and cameron county, Texas, USA. Integr. Environ. Assess. Manag. 14 (1), 120–129. doi:10.1002/ieam.1973
Farfán-Beltrán, M. E., Chávez-Pesqueira, M., Hernández-Cumplido, J., and Cano-Santana, Z. (2022). A quick evaluation of ecological restoration based on arthropod communities and trophic guilds in an urban ecological preserve in Mexico City. Rev. Chil. Hist. Nat. 95 (1), 8. doi:10.1186/s40693-022-00108-8
Fine, G., and Thomassie, G. (2000). Release brochure vermilion smooth cordgrass (Spartina alterniflora). Galliano, LA: USDA.
French McCay, D. P., and Rowe, J. J. (2003). Habitat restoration as mitigation for lost production at multiple trophic levels. Mar. Ecol. Prog. Ser. 264, 233–247. doi:10.3354/meps264233
Garbutt, A., and Wolters, M. (2008). The natural regeneration of salt marsh on formerly reclaimed land. Appl. Veg. Sci. 11 (3), 335–344. doi:10.3170/2008-7-18451
Gerber, E., Krebs, C., Murrell, C., Moretti, M., Rocklin, R., and Schaffner, U. (2008). Exotic invasive knotweeds (Fallopia spp.) negatively affect native plant and invertebrate assemblages in European riparian habitats. Biol. Conserv. 141 (3), 646–654. doi:10.1016/j.biocon.2007.12.009
Gratton, C., and Denno, R. F. (2006). Arthropod food web restoration following removal of an invasive wetland plant. Ecol. Appl. 16 (2), 622–631. doi:10.1890/1051-0761(2006)016[0622:afwrfr]2.0.co;2
Gratton, C., and Denno, R. F. (2005). Restoration of arthropod assemblages in a Spartina salt marsh following removal of the invasive plant Phragmites australis. Restor. Ecol. 13 (2), 358–372. doi:10.1111/j.1526-100X.2005.00045.x
Guiden, P. W., Barber, N. A., Blackburn, R., Farrell, A., Fliginger, J., Hosler, S. C., et al. (2021). Effects of management outweigh effects of plant diversity on restored animal communities in tallgrass prairies. Proc. Natl. Acad. Sci. U.S.A. 118 (5), e2015421118. doi:10.1073/pnas.2015421118
Hacala, A., Le Roy, M., Sawtschuk, J., and Pétillon, J. (2019). Comparative responses of spiders and plants to maritime heathland restoration. Biodivers. Conserv. 29 (1), 229–249. doi:10.1007/s10531-019-01880-y
Haney, J. C., Geiger, H. J., and Short, J. W. (2014). Bird mortality from the Deepwater Horizon oil spill. II. Carcass sampling and exposure probability in the coastal Gulf of Mexico. Mar. Ecol. Prog. Ser. 513, 239–252. doi:10.3354/meps10839
Hu, S., Niu, Z., Chen, Y., Li, L., and Zhang, H. (2017). Global wetlands: Potential distribution, wetland loss, and status. Sci. Total Environ. 586, 319–327. doi:10.1016/j.scitotenv.2017.02.001
La Peyre, M. K., Gossman, B., and Nyman, J. A. (2007). Assessing functional equivalency of nekton habitat in enhanced habitats: Comparison of terraced and unterraced marsh ponds. Estuaries Coasts J ERF 30 (3), 526–536. doi:10.1007/bf03036518
Lengyel, S., Mester, B., Szabolcs, M., Szepesváry, C., Szabó, G., Polyák, L., et al. (2020). Restoration for variability: Emergence of the habitat diversity paradigm in terrestrial ecosystem restoration. Restor. Ecol. 28 (5), 1087–1099. doi:10.1111/rec.13218
Leonard, E. E., Mast, A. M., Hawkins, C. P., and Kettenring, K. M. (2021). Arthropod assemblages in invasive and native vegetation of Great Salt Lake wetlands. Wetlands 41 (5), 1. doi:10.1007/s13157-021-01446-1
Levrel, H., Pioch, S., and Spieler, R. (2012). Compensatory mitigation in marine ecosystems: Which indicators for assessing the “no net loss” goal of ecosystem services and ecological functions? Mar. Policy 36 (6), 1202–1210. doi:10.1016/j.marpol.2012.03.004
Lin, Q., Mendelssohn, I. A., Graham, S. A., Hou, A., Fleeger, J. W., and Deis, D. R. (2016). Response of salt marshes to oiling from the deepwater Horizon spill: Implications for plant growth, soil surface-erosion, and shoreline stability. Sci. Total Environ. 557-558, 369–377. doi:10.1016/j.scitotenv.2016.03.049
Loch, J. M. H., Walters, L. J., and Cook, G. S. (2020). Recovering trophic structure through habitat restoration: A review. Food Webs 25, e00162. doi:10.1016/j.fooweb.2020.e00162
Madrid, E. N., Quigg, A., and Armitage, A. R. (2012). Marsh construction techniques influence net plant carbon capture by emergent and submerged vegetation in a brackish marsh in the northwestern Gulf of Mexico. Ecol. Eng. 42, 54–63. doi:10.1016/j.ecoleng.2012.02.001
Maoela, M. A., Roets, F., Jacobs, S. M., and Esler, K. J. (2016). Restoration of invaded Cape Floristic Region riparian systems leads to a recovery in foliage-active arthropod alpha- and beta-diversity. J. Insect Conserv. 20 (1), 85–97. doi:10.1007/s10841-015-9842-x
Matsumura, M., Trafelet-Smith, G. M., Gratton, C., Finke, D. L., Fagan, W. F., and Denno, R. F. (2004). Does intraguild predation enhance predator performance? A stoichiometric perspective. Ecology 85 (9), 2601–2615. doi:10.1890/03-0629
Matthews, J. W., and Endress, A. G. (2008). Performance criteria, compliance success, and vegetation development in compensatory mitigation wetlands. Environ. Manag. 41 (1), 130–141. doi:10.1007/s00267-007-9002-5
McCall, B. D., and Pennings, S. C. (2012). Disturbance and recovery of salt marsh arthropod communities following BP Deepwater Horizon oil spill. PLOS ONE 7 (3), e32735. doi:10.1371/journal.pone.0032735
McCutchan, J. H., Lewis, W. M., Kendall, C., and McGrath, C. C. (2003). Variation in trophic shift for stable isotope ratios of carbon, nitrogen, and sulfur. Oikos 102 (2), 378–390. doi:10.1034/j.1600-0706.2003.12098.x
Mitsch, W. J., and Hernandez, M. E. (2012). Landscape and climate change threats to wetlands of North and Central America. Aquat. Sci. 75 (1), 133–149. doi:10.1007/s00027-012-0262-7
Moir, M. L., Brennan, K. E. C., Majer, J. D., Koch, J. M., and Fletcher, M. J. (2010). Plant species redundancy and the restoration of faunal habitat: Lessons from plant-dwelling bugs. Restor. Ecol. 18, 136–147. doi:10.1111/j.1526-100X.2010.00654.x
Mortimer, S. R., Booth, R. G., Harris, S. J., and Brown, V. K. (2002). Effects of initial site management on the Coleoptera assemblages colonising newly established chalk grassland on ex-arable land. Biol. Conserv. 104 (3), 301–313. doi:10.1016/S0006-3207(01)00195-1
Morton, R. A., and Barras, J. A. (2011). Hurricane impacts on coastal wetlands: A half-century record of storm-generated features from southern Louisiana. J. Coast. Res. 275 (6A), 27–43. doi:10.2112/jcoastres-d-10-00185.1
Mossman, H. L., Davy, A. J., and Grant, A. (2012). Does managed coastal realignment create saltmarshes with 'equivalent biological characteristics' to natural reference sites? J. Appl. Ecol. 49 (6), 1446–1456. doi:10.1111/j.1365-2664.2012.02198.x
Murray, N. J., Worthington, T. A., Bunting, P., Duce, S., Hagger, V., Lovelock, C. E., et al. (2022). High-resolution mapping of losses and gains of Earth's tidal wetlands. Science 376 (6594), 744–749. doi:10.1126/science.abm9583
Netto, S. A., and Lana, P. C. (1999). The role of above- and below-ground components of Spartina alterniflora (Loisel) and detritus biomass in structuring macrobenthic associations of Paranaguá Bay (SE, Brazil). Hydrobiologia 400, 167–177. doi:10.1023/a:1003753001411
Ning, Z., Chen, C., Xie, T., Zhu, Z., Wang, Q., Cui, B., et al. (2021). Can the native faunal communities be restored from removal of invasive plants in coastal ecosystems? A global meta‐analysis. Glob. Change Biol. 27 (19), 4644–4656. doi:10.1111/gcb.15765
Paetzold, A., Lee, M., and Post, D. M. (2008). Marine resource flows to terrestrial arthropod predators on a temperate island: The role of subsidies between systems of similar productivity. Oecologia 157 (4), 653–659. doi:10.1007/s00442-008-1098-7
Page, H. M., Lastra, M., Rodil, I. F., Briones, M. J. I., and Garrido, J. (2010). Effects of non-native Spartina patens on plant and sediment organic matter carbon incorporation into the local invertebrate community. Biol. Invasions 12 (11), 3825–3838. doi:10.1007/s10530-010-9775-y
Pétillon, J., and Garbutt, A. (2008). Success of managed realignment for the restoration of salt-marsh biodiversity: Preliminary results on ground-active spiders. J. Arachnol. 36 (2), 388–393. doi:10.1636/CT07-113.1
Pétillon, J., Potier, S., Carpentier, A., and Garbutt, A. (2014). Evaluating the success of managed realignment for the restoration of salt marshes: Lessons from invertebrate communities. Ecol. Eng. 69, 70–75. doi:10.1016/j.ecoleng.2014.03.085
Pétillon, J., Ysnel, F., Lefeuvre, J.-C., and Canard, A. (2005). Are salt marsh invasions by the grass Elymus athericus a threat for two dominant halophilic wolf spiders? J. Arachnology 33 (2), 236–242. doi:10.1636/ct-04-121.1
Post, D. M., Layman, C. A., Arrington, D. A., Takimoto, G., Quattrochi, J., and Montaña, C. G. (2007). Getting to the fat of the matter: Models, methods and assumptions for dealing with lipids in stable isotope analyses. Oecologia 152 (1), 179–189. doi:10.1007/s00442-006-0630-x
Post, W., and Greenlaw, J. S. (2006). Nestling diets of coexisting salt marsh sparrows: Opportunism in a food-rich environment. Estuaries Coasts 29 (5), 765–775. doi:10.1007/BF02786527
R Core Team (2020). R: A language and environment for statistical computing. Vienna, Austria: R Foundation for Statistical Computing. AvaliableAt: https://www.R-project.org/: R Foundation for Statistical Computing.
Rozas, L. P., Caldwell, P., and Minello, T. J. (2005). The fishery value of salt marsh restoration projects. J. Coast. Res. SI 40, 37–50.
Ruiz‐Jaen, M. C., and Aide, T. M. (2005). Restoration success: How is it being measured? Restor. Ecol. 13 (3), 569–577.
Schrama, M., Berg, M. P., and Olff, H. (2012). Ecosystem assembly rules: The interplay of green and Brown webs during salt marsh succession. Ecology 93 (11), 2353–2364. doi:10.1890/11-1102.1
Silliman, B. R., van de Koppel, J., McCoy, M. W., Diller, J., Kasozi, G. N., Earl, K., et al. (2012). Degradation and resilience in Louisiana salt marshes after the BP- Deepwater Horizon oil spill. Proc. Natl. Acad. Sci. U.S.A. 109 (28), 11234–11239. doi:10.1073/pnas.1204922109
Simao, M. C. M., Flory, S. L., and Rudgers, J. A. (2010). Experimental plant invasion reduces arthropod abundance and richness across multiple trophic levels. Oikos 119 (10), 1553–1562. doi:10.1111/j.1600-0706.2010.18382.x
Simenstad, C. A., and Thom, R. M. (1996). Functional equivalency trajectories of the restored Gog-Le-Hi-Te estuarine wetland. Ecol. Appl. 6 (1), 38–56. doi:10.2307/2269551
Slater, J. A., and Baranowski, R. M. (1990). “Lygaeidae of Florida (Hemiptera: Heteroptera),” in Contribution bureau of entomology (Gainesville, FL: Division of Plant Industry).
Staszak, L. A., and Armitage, A. R. (2013). Evaluating salt marsh restoration success with an index of ecosystem integrity. J. Coast. Res. 287 (2), 410–418. doi:10.2112/jcoastres-d-12-00075.1
Stock, B. C., Jackson, A. L., Ward, E. J., Parnell, A. C., Phillips, D. L., and Semmens, B. X. (2018). Analyzing mixing systems using a new generation of Bayesian tracer mixing models. PeerJ 6, e5096. doi:10.7717/peerj.5096
Talley, T. S., and Levin, L. A. (1999). Macrofaunal succession and community structure in Salicornia marshes of southern California. Estuar. Coast. Shelf Sci. 49 (5), 713–731. doi:10.1006/ecss.1999.0553
Turner, R. E., McClenachan, G., and Tweel, A. W. (2016). Islands in the oil: Quantifying salt marsh shoreline erosion after the deepwater Horizon oiling. Mar. Pollut. Bull. 110 (1), 316–323. doi:10.1016/j.marpolbul.2016.06.046
Van den Bosch, K., and Matthews, J. W. (2017). An assessment of long-term compliance with performance standards in compensatory mitigation wetlands. Environ. Manag. 59 (4), 546–556. doi:10.1007/s00267-016-0804-1
Watts, C. H., Clarkson, B. R., and Didham, R. K. (2008). Rapid beetle community convergence following experimental habitat restoration in a mined peat bog. Biol. Conserv. 141 (2), 568–579. doi:10.1016/j.biocon.2007.12.008
Watts, C. H., and Didham, R. K. (2006). Rapid recovery of an insect-plant interaction following habitat loss and experimental wetland restoration. Oecologia 148 (1), 61–69. doi:10.1007/s00442-005-0344-5
Weinstein, M. P., Litvin, S. Y., and Guida, V. G. (2005). Considerations of habitat linkages, estuarine landscapes, and the trophic spectrum in wetland restoration design. J. Coast. Res. SI 40, 51–63. https://www.jstor.org/stable/25736615.
Weisberg, P. J., Mortenson, S. G., and Dilts, T. E. (2012). Gallery forest or herbaceous wetland? The need for multi‐target perspectives in riparian restoration planning. Restor. Ecol. 21 (1), 12–16. doi:10.1111/j.1526-100X.2012.00907.x
Wozniak, A. S., Roman, C. T., Wainright, S. C., McKinney, R. A., and James-Pirri, M. J. (2006). Monitoring food web changes in tide-restored salt marshes: A carbon stable isotope approach. Estuaries Coasts 29 (4), 568–578. doi:10.1007/bf02784283
Wu, H. T., Yang, M. Y., Lu, K. L., and Batzer, D. P. (2021). Effects of ecological restoration on trophic dynamics in estuarine wetlands. Wetlands 41 (1), 10. doi:10.1007/s13157-021-01408-7
Wu, Y.-T., Wang, C.-H., Zhang, X.-D., Zhao, B., Jiang, L.-F., Chen, J.-K., et al. (2008). Effects of saltmarsh invasion by Spartina alterniflora on arthropod community structure and diets. Biol. Invasions 11 (3), 635–649. doi:10.1007/s10530-008-9279-1
Zedler, J. B., and Lindig-Cisneros, R. (2000). “Functional equivalency of restored and natural salt marshes,” in Concepts and controversies in tidal marsh Ecology. Editors M. P. Weinstein, and D. A. Kreeger (Dordrecht, Netherlands: Kluwer Academic Publishers), 569–582.
Zengel, S., Pennings, S. C., Silliman, B., Montague, C., Weaver, J., Deis, D. R., et al. (2016). Deepwater Horizon oil spill impacts on salt marsh fiddler crabs (Uca spp.). Estuaries Coasts 39 (4), 1154–1163. doi:10.1007/s12237-016-0072-6
Zengel, S., Rutherford, N., Bernik, B. M., Weaver, J., Zhang, M., Nixon, Z., et al. (2021). Planting after shoreline cleanup treatment improves salt marsh vegetation recovery following the Deepwater Horizon oil spill. Ecol. Eng. 169, 106288. doi:10.1016/j.ecoleng.2021.106288
Zengel, S., Weaver, J., Mendelssohn, I. A., Graham, S. A., Lin, Q., Hester, M. W., et al. (2022). Meta‐analysis of salt marsh vegetation impacts and recovery: A synthesis following the deepwater Horizon oil spill. Ecol. Appl. 32 (1), e02489. doi:10.1002/eap.2489
Keywords: Spartina, Deepwater Horizon oil spill, Chenier Plains, Salt Bayou ecosystem, insect, spider, planthopper
Citation: Armitage AR, Goeke JA and Ho C-K (2022) Does compensatory mitigation restore food webs in coastal wetlands? A terrestrial arthropod case study on the Upper Texas Coast. Front. Environ. Sci. 10:965557. doi: 10.3389/fenvs.2022.965557
Received: 09 June 2022; Accepted: 08 September 2022;
Published: 06 October 2022.
Edited by:
Brian Joseph Roberts, Louisiana Universities Marine Consortium, United StatesReviewed by:
Shannon Murphy, University of Denver, United StatesJulien Pétillon, University of Rennes 1, France
Copyright © 2022 Armitage, Goeke and Ho. This is an open-access article distributed under the terms of the Creative Commons Attribution License (CC BY). The use, distribution or reproduction in other forums is permitted, provided the original author(s) and the copyright owner(s) are credited and that the original publication in this journal is cited, in accordance with accepted academic practice. No use, distribution or reproduction is permitted which does not comply with these terms.
*Correspondence: Anna R. Armitage, armitage@tamu.edu