- 1Department of Biology, Stephen F. Austin State University, Nacogdoches, TX, United States
- 2Arthur Temple College of Forestry and Agriculture, Stephen F. Austin State University, Nacogdoches, TX, United States
Globally, freshwater fish diversity is rapidly changing in response to land use change, including urbanization. Time series of freshwater fish communities can help elucidate the underlying mechanisms of species loss and gains, as well as promote conservation strategies. Traditional approaches to understand the dynamics of fish assemblages is traditionally focused on taxonomic diversity, one aspect among many dimensions of biodiversity. Thus, using complementary analytical methods, such as functional diversity, can help researchers to develop a stronger predictive framework on the effects of the species loss or gains in response to urbanization. We assessed temporal changes of fish assemblages in an urban stream in East Texas between four seasonal surveys of 1989–1990 and 2018–2019. We explored the similarities and differences in species richness, composition, and functional diversity of fishes. Although the species richness and functional richness between the two time periods were similar, we observed changes in species composition. We also observed higher functional dispersion and evenness in the 2018–2019 assemblage compared to 1989–1990 assemblage. Some fish species were locally extirpated, while others colonized the system, increasing the functional diversity of the current assemblage. Urbanization has commonly been associated with declines in diversity and richness, however, our findings suggest that the addition of a few invasive generalist species (e.g., blue tilapia) and native tolerant (e.g., redbreast sunfish, blacktail shiner) in this stream system could be associated with increased human activities in this small, urbanized area in East Texas. Streams in East Texas support very diverse fish assemblages, with some sentinel species (e.g., imperiled shiners: Sabine shiner, a habitat-affinity, broadcast spawner) rapidly responding to stream alterations. Multiple stressors can underlie the dynamics and composition of fish assemblages; thus, it is essential for more comparative studies of current and historical data of fish assemblages. Likewise, studies targeting sentinel fish species may have more utility for the evaluation of freshwater ecosystems.
1 Introduction
The loss and gain of species from local assemblages underlies the dynamics of ecological communities (Post, 2019). Shifts in locally co-occurring species through time are influenced by species traits and ecological processes including environmental filtering, dispersal, and drift (Dornelas et al., 2013; Vellend, 2016). These factors can vary in their relative strength to the organization of aquatic ecosystems, producing diverse effects on biological communities (Boon et al., 2000). Thus, assessing biotic communities over time provides insights about the resiliency and sensitivity of species in natural and novel ecosystems, like urban streams, which is critical to develop a predictive framework on how these assemblages may respond to agents of global change.
Threats to aquatic habitats include deforestation, alterations of flow regimes, agriculture, and urbanization (Carvajal-Quintero et al., 2017; Dudgeon, 2020; Su et al., 2021). In urban landscapes, changes to aquatic habitats include channel modification, loss of riparian vegetation, and increased impervious surface cover. These changes alter flow regime that, in turn, affect the abundance and distribution of fluvial habitat features that serve as sites for foraging, reproduction, and refugia of aquatic fauna (e.g., riffles and pools; Weaver and Garman, 1994; Walters et al., 2005; Booth et al., 2016). Fishes are model organisms to evaluate changes in biological assemblages as their taxonomy is relatively well-known, they exhibit rapid responses to environmental changes, and their capacity for dispersal and colonization is restricted to connectivity among water bodies (Wootton, 1991). Fishes also serve as sentinel species to evaluate stream changes over time (Fitzgerald et al., 1999). Urbanization has been often linked to declines in fish diversity, loss of endemic and stream specialists, increases in the number of tolerant and exotic taxa, and changes in trophic structure (Marchetti and Moyle, 2001; Schweizer and Matlack, 2005; Walsh et al., 2005; Montaña and Schalk, 2018). Despite general knowledge about urbanization impacts on stream ecosystems, how fish assemblages change in these contexts are still not fully understood due to the dynamics of fluvial ecosystems (Jackson et al., 2001). Small urban streams can provide habitat for a number of species, including endemic species, which contribute to the function of these urban ecosystems (Carvajal-Quintero et al., 2019; Van Metre et al., 2019). Therefore, understanding how the diversity and composition of instream biota changes in urban streams is important for biodiversity conservation and the development of stream management plans and restoration initiatives.
Studies that compare biological assemblages often assess taxonomic diversity (TD) (Villéger et al., 2010), although, more recently, functional diversity (FD; i.e., organisms’ trait diversity) has also been examined (e.g., Dornelas et al., 2013; Bower and Winemiller, 2019). Assessing complementary aspects of biodiversity, such as FD, has increased the understanding of changes in biological assemblages, generating more robust conclusions regarding the patterns observed (Cilleros et al., 2016; Jia et al., 2020). For example, taxonomic diversity, as given by species richness and species composition, elucidates important aspects related to species identity or shifts in species occurrence or relative abundance over time (Magurran, 2013). Functional diversity is related to the role of species within their environments as these traits are linked to their performance (e.g., resource acquisition and physiological tolerances; Violle et al., 2007; Villéger et al., 2010). Functional diversity can provide a more effective way to describe biodiversity (Mouchet et al., 2010) and reveal the impacts of habitat changes (e.g., land-use change, habitat homogenization) on biotic assemblages (Toussaint et al., 2018). Organismal functional traits are linked to ecosystem processes (i.e., effects of traits) or to ecosystem stability through resistance and resilience (i.e., response of traits) (Villéger et al., 2010). Studying functional diversity allows researchers to make comparisons among different habitats within ecosystems (e.g., riffles vs. pools) or among ecosystems, despite differences or similarities in the taxonomic composition (Mouchet et al., 2010). Although taxonomic diversity and functional diversity are important to maintain ecosystem function (Tilman et al., 1997), they are not necessarily related (Cadotte et al., 2011). For example, Villéger et al. (2010) measured both TD and FD of fish assemblages along a habitat disturbance gradient in a tropical estuary and found that fish species richness (i.e., TD) increased in more disturbed sites, while functional specialization (i.e., FD) decreased. The species that decreased in abundance had functional traits linked to seagrass habitats. Results from Villéger et al. (2010) highlights the importance of using functional diversity along with TD to provide a more complete signal of the community and ecosystems in presence of or after disturbances.
Rivers and streams in the Southern United States are threatened by drastic changes in land use and increasing urbanization (Van Metre et al., 2019), resulting in major challenges for the conservation of local freshwater biodiversity. In Texas, studies in major river drainages suggest that some fish species have become extirpated, locally extinct, or are at risk of extinction (Anderson et al., 1995; Mayes et al., 2019). Rapid human-induced changes in stream ecosystems can influence all aspects of species diversity, as well as fitness and life history traits (Walters et al., 2003; McKinney, 2006). For instance, a decrease in the magnitude of the water flow and changes in water quality shifted the composition of the local fish assemblage in the upper San Antonio River through species’ loss (of spring-associated fishes) and gains (of both native and non-native species) (Craig and Bonner, 2021). Changes in flow regimes and land use also result in shifts in fish traits. Pease et al. (2018) observed that Guadalupe bass (Micropterus treculii) from the Colorado River in Texas, increased in their body depth over time in response to the alteration of annual flow conditions. Thus, comparisons of historical fish assemblages with contemporary fish surveys can quantify the changes of fish assemblages subjected to increasing anthropogenic stressors (Hughes and Noss, 1992; Perkin and Bonner, 2016; Craig and Bonner, 2021).
To provide a long-term assessment of changes in local fish assemblages, we compared results from fish surveys conducted in La Nana Creek, an urban stream in East Texas across a 30-year period (1989–1990 vs. 2018–2019). We evaluated the changes in this fish assemblage using both taxonomic and functional diversity approaches to provide inferences in the potential shifts of these assemblages across the time period. La Nana Creek runs through a small urban area, the City of Nacogdoches, Texas, United States. While the population size has only slightly increased from 1990 to 2019 in this small city (US Census Bureau, 2011, US Census Bureau, 2020), the impervious surface cover surrounding the creek has more than doubled in area (Supplementary Figure S1). Impervious surface cover has served as a proxy for urbanization (Montaña and Schalk, 2018), suggesting that this stream has experienced an increase in urbanization between these two survey periods. We predicted that 2018–2019 surveys would yield lower values of both the taxonomic and functional diversity when compared to 1989–1990 surveys. Over the past 30 years, increased human population density and urban development may have altered stream substrates, water flow, and temperature consequently affecting the occurrence of some sentinel fishes (e.g., imperiled shiners). A sentinel species is described as one that can be used to evaluate changes that have occurred in the system over time (Fitzgerald et al., 1999). Background sampling in east Texas streams and in La Nana Creek watershed suggest that Sabine shiner (Notropis sabinae) can be identified as a suitable sentinel species. The Sabine shiner is listed as endangered species by the Missouri Department of Conservation and a species of greatest conservation concern in Texas (Williams and Bonner, 2007). This species is highly associated with clear, shallow slow flowing water such as runs and riffles of small streams (Williams and Bonner, 2006). Sabine shiner is identified as a broadcast spawner, which requires flowing water for eggs and larvae to disperse downstream via drift. Therefore, alteration of flow regimes and habitat fragmentation potentially disrupt their reproductive process by limiting downstream drift of early stages and upstream migration of adults. Current surveys of the imperiled Sabine shiner in Banita and La Nana Creek, Nacogdoches County, suggest a high decrease in the relative occurrence of this imperiled species in the main channel of La Nana Creek (Hutchens and Montaña unpublished data), a location where the species was considered stable approximately 20-years ago (Williams and Bonner 2006).
In view of the needs for understanding changes in urban stream assemblages, this study seeks to describe and inform patterns of fish assemblages over 30-year period. Continued urban development can negatively affect local fish assemblages, resulting in lower species richness, in which the remaining species in La Nana Creek may be a subset of 1989–1990 species. We predicted that fish species from 2018–2019 surveys would exhibit greater homogeneity in their trait diversity compared to fish species from the 1989–1990 survey.
2 Material and methods
2.1 Study area
La Nana Creek is a perennial, third-order stream, and tributary of the Angelina River in East-Central Texas. La Nana Creek watershed located within a mixture of urban and rural land uses, with forest covering approximately 37% of the land followed by pastures (28%) and urban development (25%), with the City of Nacogdoches predominantly covering the middle portion of the watershed (TWDB Texas Water Development Board, 2021). The stream is located in the City of Nacogdoches (Texas), an urban area (US Census Bureau, 2011; Lombardi et al., 2017). Developed and altered areas within the city include a mixture of commercial and industrial businesses, multiple-family dwellings, university campus, and urban managed green space. The stream is 51.5 km long, originates in the north of the city of Nacogdoches (Nacogdoches County, Texas) and flows south until merging with the Angelina River. The stream is characterized by shallow water with a mixture of sand, silt, and bedrock substrates. Several mesohabitats also occur within the stream including riffles, slow-flowing runs, and pools. Stream reaches running through the city of Nacogdoches contain debris (e.g., tires, furniture) and concrete associated with human disposal, but also lack vegetation along the riparian zones.
We surveyed fishes at two sites (upstream and downstream) in the main channel of La Nana Creek (Figure 1), over four seasons for 1 year (i.e., fall 2018, winter 2018, spring 2019, summer 2019). The sites and sampling methods were replicated based on 1989–1990 surveys by Ahle et al. (1991). These sites within the stream were selected because they appear to be surrounded by different land uses. For example, the upstream site (31⁰39′42.0″ N - 94⁰38′18.4″ W), which is located about 4.5 km upstream from the city of Nacogdoches, is dominated by pasture with riparian vegetation covering approximately 10% of the stream channel and composed primarily of hardwood trees. The downstream site (31°37′03.5″ N - 94°38′29.7″ W) located within the city of Nacogdoches, is surrounded by urban development. At this site, the stream is channelized, with very few bends and meanders, and stream banks lack forest vegetation. To better understand whether changes in land uses happened in La Nana Creek watershed between the two surveyed periods, we examined Landsat color images from 1991 and 2016 (USGS National Land Cover Database, upper and lower Angelina Sub-watersheds, HUC 1202002 and 12020005). We were unable to obtain Landsat images from our specific survey period 1989–1990 and 2018–2019. However, with the images from 1991 and 2016, we estimated the developed land cover using different degrees of intensity such as high, medium, low intensities, as well as open space (see Supplementary Figure S1 for details). Development within the watershed and around surveyed sites has increased from 2,892 Ha (1991) to 7,476 Ha (2016), an increase of 159% (Supplementary Figure S1).
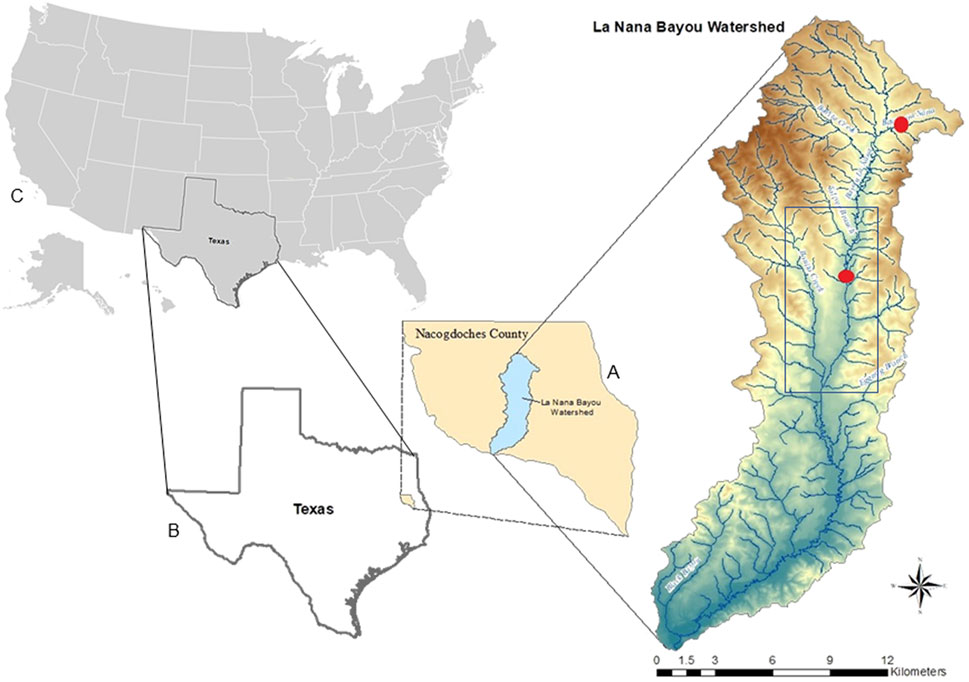
FIGURE 1. Location of La Nana Creek watershed in Nacogdoches County (A) in east Texas, State of Texas (B), United States (C). Red circles represent the two surveyed sites within the watershed (upstream and downstream). Color pattern in the watershed represents upper sub-watershed (brown) and lower sub-watershed (blue). Open rectangle on La Nana watershed depicts the actual location of the city of Nacogdoches within the watershed.
2.2 Fish collections
Fishes were collected along a reach of approximately 300 m and all available habitats were surveyed using a seine (4.6 m × 1.8 m, 5-mm mesh) and backpack electrofisher (LR-24 Smith-Root®). All fish specimens were identified, counted, and released downstream. When available, a maximum of 12 individuals per species were anesthetized using clove oil for preservation in a 10% buffered formalin solution, and then transported to the laboratory for further morphological analysis. We classified fish species as native, native invaders, and introduced species. We used taxonomic keys to identify fish species in the field. In addition, we used the Catalog of Fishes (Fricke et al., 2022) to verify the validity of taxonomic names of fish species.
2.3 Functional traits
For all species reported in this study, we measured 15 morphological traits from two to 12 adult individuals per fish species (average ± SD; 6.0 ± 3.0; Supplementary Table S1). Morphometric measurements were made using a digital caliper (precision = .01 mm). We chose 15 morphological measurements (body standard length, head height, gape width, interorbital distance, snout length shut, snout length open, peduncle width, peduncle length, dorsal height, anal length, anal height, caudal length, pectoral length, pectoral height, and mouth position) because they are known to reflect various facets of habitat use, trophic ecology, and performance of fish individuals (Winemiller, 1991; Montaña and Winemiller, 2010; Montaña et al., 2014). While most of the measurements we made were from fish specimens collected during the 2018–2019 surveys, there were cases in which specimens were not available or were insufficient to be used in the functional diversity analysis. Therefore, we used fish specimens that were collected from the same sites, but in different years that were close to the year of our collection in the study (Supplementary Table S1). All specimens used and measured were deposited at the fish collection at Stephen F. Austin State University, Nacogdoches, Texas. Thus, because the measurements of morphological traits made on adult specimens of a given species were virtually consistent, we calculated species means for 15 morphological attributes and used these averages in subsequent analyses.
2.4 Data analysis
Our sampling unit was locality (n = 2 sites) during each season (n = 4 seasons) for both 1989–1990 and 2018–2019 surveys (i.e., eight sampling units per given site and period of time). First, we used a generalized linear model (GLM) with the Gaussian distribution to examine fish species richness (dependent variable) between 1989–1990 and 2018–2019 surveys (independent variables). We evaluated the residuals from this model using KS, dispersion, and outlier tests from DHARMA package (Hartig, 2021); however, they were not significant (p > .05). Then, we evaluated the differences in species composition between 1989–1990 and 2018–2019 surveys using the permutational multivariate analysis of variance (PERMANOVA) to test for significant clustering of the time and computed 999 permutations. Prior to PERMANOVA, we removed observations that had few species, and then applied the Hellinger transformation to the data. We used non-metric multidimensional scaling (NMDS), using relative abundance data and the Bray Curtis dissimilarity index, to graphically visualize the variability of species composition between surveys (1989–1990 versus 2018–2019). We classified fish species into four groups based on their frequency performing a multinomial species classification using the CLAM test (Chazdon et al., 2011). Fish species were grouped by their high prevalence during one survey period, ‘Restricted to 1989–1990’ and ‘Restricted to 2018–2019’, high abundance across both 1989–1990 and 2018–2019 surveys (‘Consistent’), and low abundance across both periods (‘Too rare’). We used vegan (Oksanen et al., 2020), usedist (Bittinger, 2020), and labdsv (Roberts, 2019) in R (R Core Team, 2017).
We assessed the functional diversity in a multivariate distance-based framework using morphological trait averages for each species. With these data, we calculated functional richness (FRIC), functional evenness (FEVE), and functional dispersion (FDIS). FRIC is the estimation of trait richness of the smallest sampling unit, i.e., assemblage of a sampling point of a season, based on n-dimensional hypervolumes. FEVE measures the evenness of species abundances within the kernel density hypervolume (Mason et al., 2005). FDIS is the average distance of each species between the centroid of all species within the kernel density hypervolume, taking into account the relative abundances of species (Mason et al., 2005). We chose FDIS rather than functional diversity because FDIS can be a better indicator of trait dispersion in an assemblage of species (Swenson, 2014). Also, the 15 morphological traits (listed above) used in the analysis were chose because of their relevance in explaining ecological aspects of species such as habitat use, feeding ecology, and performance (Montaña and Winemiller 2010; Bower and Winemiller 2019). To avoid the high variability of measurements among species with different body sizes, we standardized the measures of all continuous traits in relation to the standard length of each specimen and used these ratios in the analysis. Functional traits included both quantitative and categorical variables, therefore we used the Gower’s dissimilarity index from the natural logarithm of all traits as a functional distance matrix between species. We performed a principal coordinates analysis (PCoA) using the Gower’s index as distance measure, from which the axes were used to calculate FRIC and FEVE. We removed three time periods from each survey (1989–1990 and 2018–2019), six in total, because observations with few species must be removed prior to hypervolume estimation (Cardoso et al., 2021). We obtained the hypervolume from the kernel density of the three first axes of the PCoA for the abundance of fish species with the Gaussian method. We obtained the estimation of FRIC based on n-dimensional hypervolumes from each time (‘kernel.alpha’), the FDIS from the average distance to the centroid, or dissimilarity between random points within the boundaries of the kernel density hypervolume (“kernel.dispersion”), and the FEVE from the measure of the regularity of stochastic points distribution within the total functional space (‘kernel.evenness’). Finally, we compared the values of FRIC, FEVE, and FDIS between the 1989–1990 and 2018–2019 surveys with a paired t-test. Before the t-test was performed, we assured the data met the assumptions, using variance and a test of normality (Shapiro-Wilk test). We based our analysis of functional diversity on the BAT package (Cardoso et al., 2021), complemented by the packages StatMatch (D’Orazio, 2020), labdsv (Roberts, 2019), hypervolume (Blonder and Harris, 2019), and ggplot2 (Wickham, 2016) in R (R Core Team, 2017).
3 Results
3.1 Taxonomic diversity
We collected a total of 34 fish species, from 20 genera and 10 families across both the 1989–1990 and 2018–2019 surveys (Table 1). The greatest number of species collected occurred in the families Leuciscidae (formally family Cyprinidae) and Centrarchidae (Table 1). The genus Lepomis (sunfishes) contained the greatest number of species (eight species) followed by the genera Micropterus, Cyprinella, Lythrurus, Notropis, Fundulus, Ameiurus, and Etheostoma with two species each, and the remaining genera contained only one species each (Table 1). When considering the four seasons per sampling sites (i.e., upstream and downstream) and survey period (1989–1990 and 2018–2019), there were no significant differences in fish species richness between 1989 and 1990 (average ± SD; 10.5 ± 3.3) and 2018–2019 (10.3 ± 9.5; GLM. nb: χ2 = .004, df = 1,14, p = .95) surveys (Figure 2). However, we observed shifts in the species composition between 1989–1990 and 2018–2019 fish assemblages (PERMANOVA: Pseudo-F1,8 = 3.53, p < .01; Figure 3). According to CLAM analysis (Table 1; Figure 4), five species (14.7%) out of the 34 fish species recorded were detected in the 1989–1990 surveys (Restricted to 1989–1990) and included Lepomis microlophus, Micropterus salmoides, Cyprinella lutrensis, Notropis sabinae, and Pimephales vigilax, while nine species (26.4%) were detected only during the 2018–2019 surveys (Restricted to 2018–2019) and included Moxostoma poecilurum, L. auritus, L. megalotis, C. venusta, Semotilus atromaculatus, Fundulus olivaceus, Ameiurua natalis, Noturus nocturnus, and Etheostoma artesiae. Of the remaining 21 species, seven species (20.5%) were abundant across both survey periods (time Consistent) and included Gambusia affinis, F. notatus, L. cyanellus, L. gulosus, L. macrochirus, Lythrurus umbratilis, N. atrocaudalis; and 14 species (41.2%) occurred in low abundance across both times to be assigned to a preference class (i.e., Too rare, Figure 4). The occurrence of two species of greatest conservation concern such as the Sabine shiner (N. sabinae) varied from 84 individuals in surveys of 1989–1990 to 0 individuals in surveys of 2018–2019. Likewise, the occurrence of blackspot shiner (N. atrocaudalis) varied between surveyed periods from 341 to 136 individuals, respectively. However, the occurrence of blacktail shiner (C. venusta), a native invader in La Nana Creek, appeared to increase from 1989-1990 to 2018–2019 surveys (Table 1).
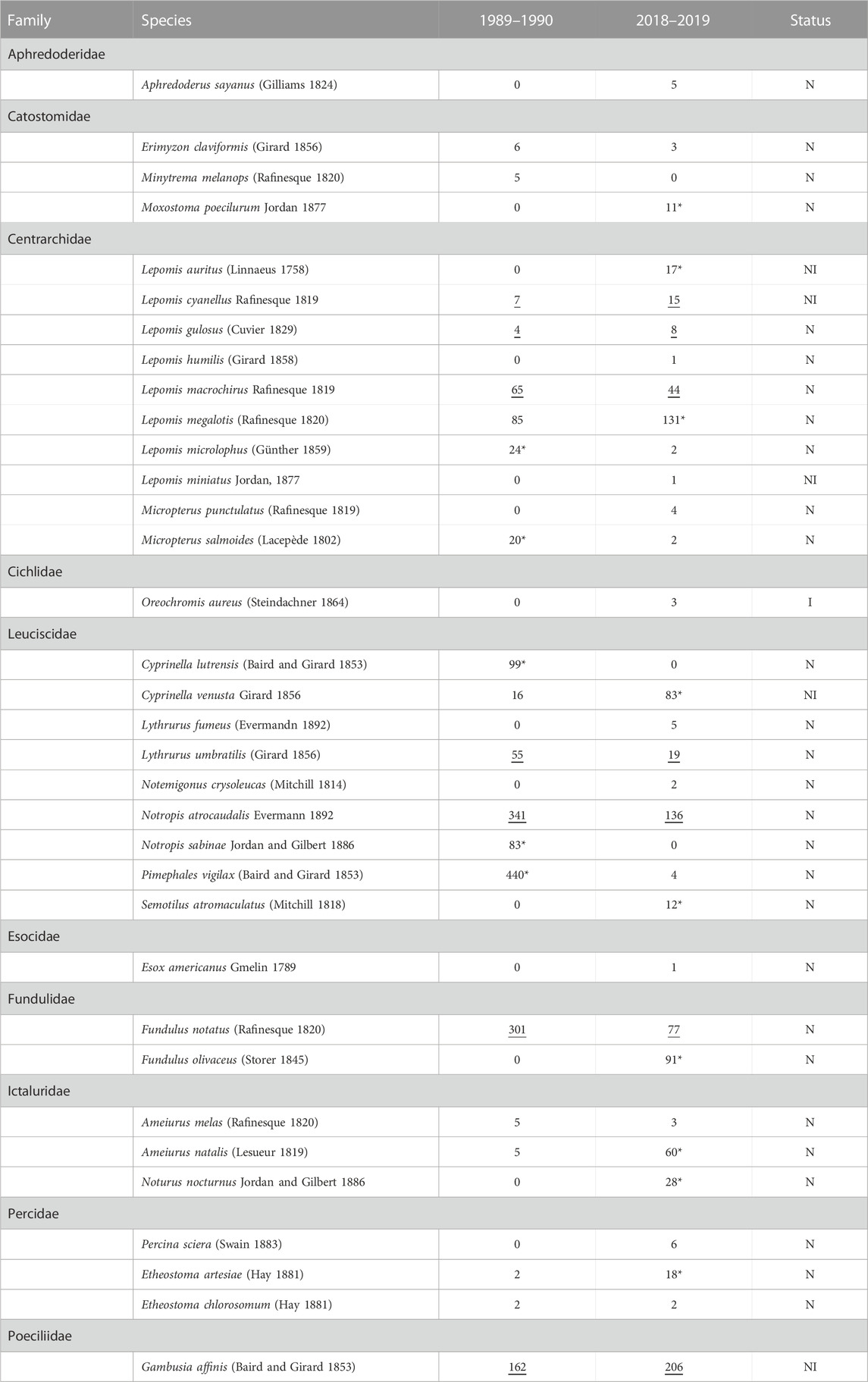
TABLE 1. Occurrence of fish species in the 1989–1990 and 2018–2019 surveys. Asterisks (*) refer to time “Restricted to” species of 1989–1990 and 2018–2019 surveys. Underlined (−) values refer to “Consistent” species over the two surveys according to CLAM analysis classification. “N” refers to native species, “I” refers to introduced species, and “NI” to native invader species from the United States, but introduced in the La Nana Creek basin, Nacogdoches Texas, United States.
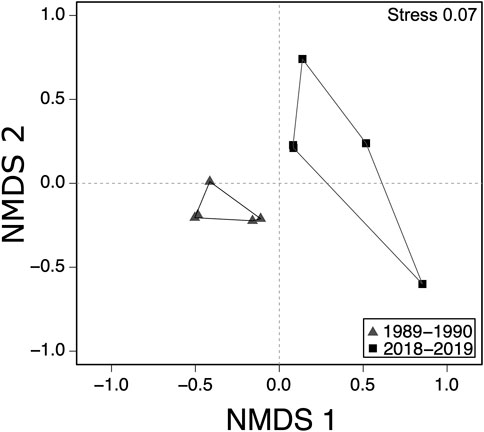
FIGURE 3. Non-metric multidimensional scaling (NMDS) ordination based on species composition between the 1989–1990 and (grey triangles) and 2018–2019 (black squares) surveys.
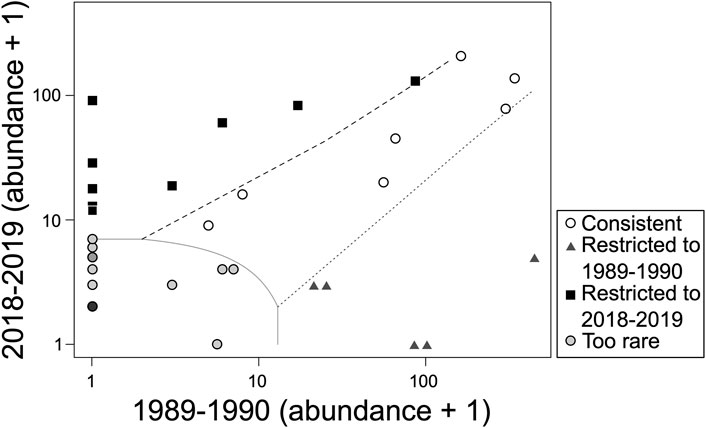
FIGURE 4. Classification of the 34 fish species according to their occurrence in the 1989–1990 and 2018–2019 surveys using CLAM analysis: Consistent (open circles); species too rare to be assigned to a specific period (grey circles); Restricted to 1989–1990 survey (grey triangles) and Restricted to 2018–2019 survey (black squares).
3.2 Functional diversity
Using the hypervolumes of the eight assemblages from the two sampling periods, we observed high overlap of species in functional trait space (Figure 5). Likewise, we found a statistically similar functional richness between the 1989–1990 (average ± SD; .013 ± .007) and 2018–2019 assemblages (.023 ± .015; F1,6 = 1.81, p = .23; Figure 6A). However, functional dispersion increased over time (1989–1990 assemblage: .151 ± .222; 2018–2019 assemblage: .190 ± .138; F1,6 = 9.89, p = .02; Figure 6B). Functional evenness also increased over time (1989–1990 assemblage: .095 ± .060; 2018–2019 assemblage: .220 ± .111; F1,6: = 6.78, p = .04; Figure 6C).
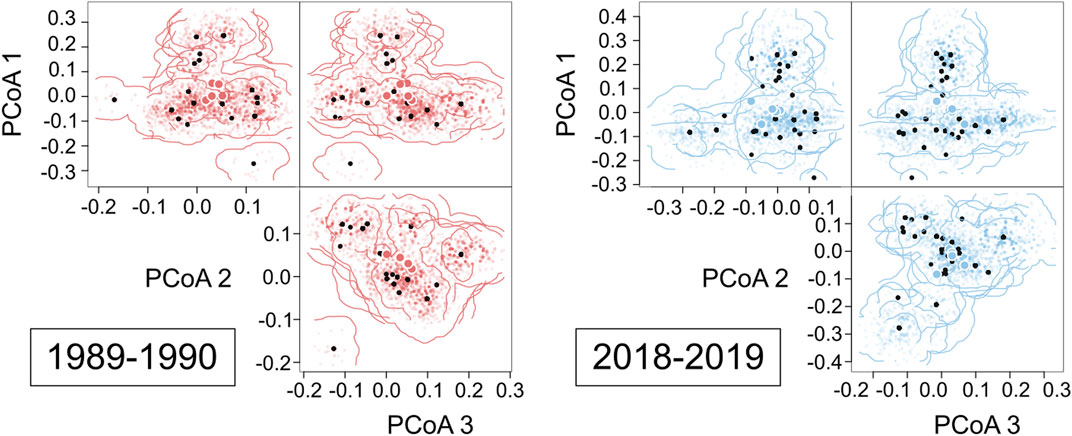
FIGURE 5. A 3-dimensional hypervolume of fish assemblages from 1989–1990 and 2018–2019 surveys. Large circles with white borders represent the centroid of 1989–1990 (red) and 2018–2019 (blue) hypervolumes (due to the proximity of centroids, some circles are overlapped). The shape and boundaries of each hypervolume are defined by 300 random points.
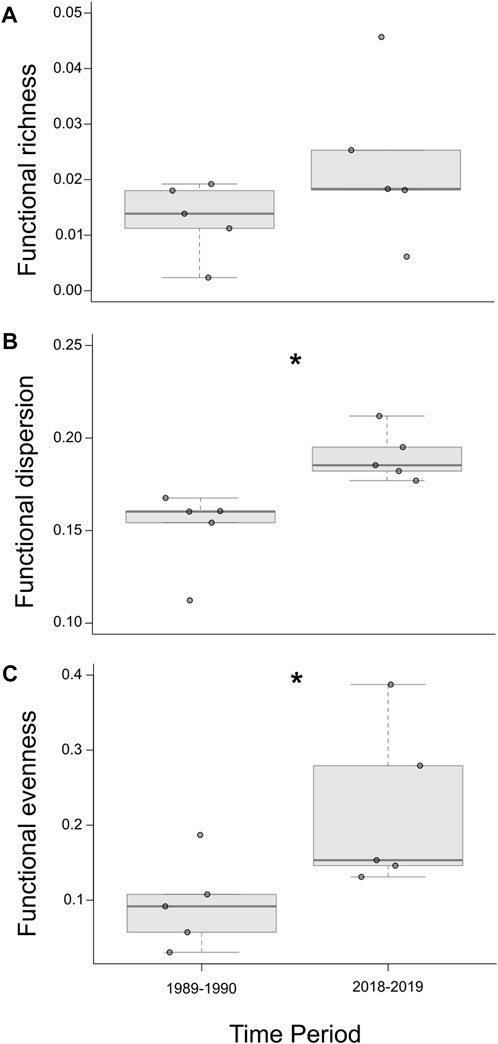
FIGURE 6. Boxplots of overall functional diversity comparisons: functional richness (A), dispersion (B), and evenness (C) between 1989–1990 and 2018–2019 surveys. A significant difference between metrics is designated by an asterisk (*; p < .05).
4 Discussion
Urbanization alters fish assemblages through a variety of direct and indirect effects. For instance, direct alteration of physical structure of the stream including stream channelization, bank stability, removal of riparian vegetation, can influence the abundance and distribution of stream fish species (Walsh et al., 2005). Indirect effects include changes in upstream land cover that result in sedimentation, increased temperatures, and primary production due to greater light incidence. In this study, we used taxonomic and functional approaches to understand the dynamics of local fish assemblage inhabiting an urbanized stream across time. Although the species richness was similar between the two surveys, changes in the species composition and functional diversity of fish assemblages varied between the survey periods. These changes included the loss of species recorded in the initial surveys as well as the addition of species in the recent surveys, including either native-invaders (i.e., species that in their native range have increased in abundance beyond a level those previously observed; Simberloff, 2011; Carey et al., 2012) or non-native species.
Species richness was similar in both surveys, a finding that was not consistent with our initial predictions. While some native species were not reported in 2018–2019 surveys, the addition of new species considered native invaders such as the cyprinid C. venusta and sunfishes L. auritus, L. cyanellus, and L. miniatus and the invasive species Oreochromis aureus, resulted in similarity of species richness between compared surveyed periods. Similar to our expectations, but contrary to our findings, a long-term study comparing freshwater fish assemblages in Texas rivers observed decreased diversity over time in eastern Texas streams (Anderson et al., 1995). Likewise, decreased species richness in relation to increasing urbanization has also been previously reported (Onorato et al., 2000; Wang et al., 2000; Meador et al., 2005). The stability of species richness over time can be attributed to environmental changes, which underpin the fish species locally, such as habitat heterogeneity and resource availability (May 1986). Nevertheless, surveys from 2018–2019 suggested higher variation around the average (SD) for species richness compared to surveys of 1989–1990, inferring a spatial (i.e., by sites) and temporal (i.e., by season) variation of fish assemblages, a trend that could be attributed to either change in water flows (Craig and Bonner, 2021) or habitat fragmentation (Williams and Bonner, 2006). Urbanization alters instream conditions, and such changes may facilitate population growth of native species and allow generalists, tolerant species to become more common in these human degraded habitats. Populations of C. venusta and L. auritus have become dominant in these eastern Texas streams (Linam et al., 2002; Swanson et al., 2022; Montaña unpublished data), and although their impacts in these local fish communities have not been evaluated, it is important to note that the dominance of these native invaders could eventually reduce or extirpate populations of other native species including those listed as species of conservation concern in east Texas streams (e.g., N. sabinae, N. atrocaudalis). The Sabine shiner (N. sabinae), a broadcast spawner and habitat specialist (Williams and Bonner, 2006), was historically abundant and stable in east Texas streams. Our 2018–2019 surveys did not yield occurrence of this shiner in La Nana Creek. Even more recently, a year-long survey re-assessing their population status in the Banita-La Nana Creek system have resulted in a very low catch per unit effort (Montaña, 2020–2021 unpublished data) compared to past surveys (i.e., Williams and Bonner, 2006).
Shifts in species composition was observed between surveyed periods. Changes in species composition between surveys of 1989–1990 and 2018–2019, combined with the maintenance of species richness over time may indicate that some species were locally extirpated, while others were added to the system. The shifts in fish assemblages are often related to declines in specialists and concomitant increases in generalists (Parks, 2014). Habitat specialists, such as the shiners N. sabinae and N. atrocaudalis, that once were stable in La Nana Creek are now listed as imperiled fish species due to population declines in east Texas streams (Williams and Bonner, 2007). Alteration of the water flow and fragmentation of the main stream channel of La Nana Creek by urban development and increased input of anthropogenic debris impede the reproduction of Sabine shiner, as the species needs flowing water for downstream drifting of the eggs and larvae and upstream migration of adults (Williams and Bonner, 2006). Although many cyprinids (e.g., minnows and shiners) and percids (e.g., darters) seem to persist through time, their occurrence appears to have declined when compared to 1989–1990 surveys. Many habitat generalists were reported only in 2018–2019 surveys including M. poecilurum, L. auritus, F. olivaceus, and N. nocturnus. The cyprinid S. atromaculatus and catastomid M. poecilurum are tolerant to disturbances (Stair et al., 1984) and higher temperatures (Taniguchi et al., 1998), and often colonize new water bodies through interconnections between the drainage systems (Boizard et al., 2009), other generalist species can become a threat for native fishes. For example, the redbreast sunfish L. auritus has been introduced in Texas streams since the 1960s, and already has threatened many congeners and other native fauna (Fuller et al., 1999; Bonner et al., 2005). Indeed, tolerant species have increased their occurrence in this urban stream system with some centrarchid (e.g., L. megalotis) and cyprinid (e.g., C. venusta) fishes becoming more abundant over time. Changes in habitat conditions and the presence of introduced species have been shown as primary causes threatening local fish assemblages (Jia et al., 2020). Thus, the shift in habitat conditions through time, due to the increase in urbanization and stream hydrological variability, potentially allows the maintenance of habitat generalist species that can demonstrate a shift in more generalized feeding strategies and greater tolerance to flow changes; hence these stream conditions likely constrained the habitat specialists to remain in the local fish assemblage studied (Rahel et al., 2008).
Functional richness (FRIC) was consistent between survey periods. The stability of FRIC emphasizes that the niche space available to be occupied by fish species was similar between the two surveys (Mouchet et al., 2010). However, we found higher values of FDIS and FEVE for the 2018–2019 surveys compared to 1989–1990 surveys. Greater values of FDIS may reflect an expansion of the niche space occupied by 2018–2019 survey’s fish assemblages, which included the addition of native invader and invasive species. Increasing FEVE, especially associated with stable FRIC, indicates positive consequences for ecosystem functioning because it means that species traits (i.e., functions), are more evenly distributed in the 2018–2019 survey than in the 1989–1990 survey. Increasing the functional evenness means that functions are more regularly represented by different species occupying the assemblage (Villeger et al., 2010). Results suggest that our 1989–1990 and 2018–2019 local fish assemblages can harbor equally diverse fish communities, with a redundancy of functional and life history traits. It is assumed that generalist cyprinid species are more resilient and less sensitive to changes in prey diversity, habitat substrates, and flow regimes (Walters et al., 2003). Increased evenness, as a consequence of local stream disturbance (e.g., urbanization), has been also associated to greater shifts in functional composition (Villéger et al., 2010). In our surveys, the high values FEVE for the 2018–2019 surveys are associated with high overlap within trait space between common native species (e.g., sunfishes, shiners) and those being added such as native invaders and invasive fishes, indicating high niche overlap and potential functional redundancy (Montaña et al., 2014).
Fish assemblages in urban water bodies are subject to drastic changes worldwide (e.g., Walters et al., 2003). Disentangling the biodiversity components associated with such changes remains a critical ecological endeavor. Despite the challenges associated with urbanization, the urban stream fish assemblages evaluated indicates the stability of taxonomic and functional richness over time, with differences in species composition (taxonomic), and functional dispersion and evenness over the 30-year survey. We highlighted the importance to evaluate changes in fish assemblages using complementary approaches, e.g., taxonomic and functional, in order to improve the inferences and conclusions of changes and similarities over time (Nakamura et al., 2018). Future studies will benefit from more comprehensive surveys including more streams and across large areas and the incorporation of behavioral and life history traits to better understand the current state of freshwater fish species on a regional scale, but also to fill gaps concern trait-based ecology in the stability of our freshwater ecosystems.
Data availability statement
The original contributions presented in the study are included in the article/Supplementary Material, further inquiries can be directed to the corresponding author.
Ethics statement
The animal study was reviewed and approved by IACUC Chair, Nathan Sparkman. SFASU Institutional Animal Care and Use Committee.
Author contributions
CGM conceived and designed the study. CGM, CMS, DP conducted the fieldwork, sorted, identified, and took the measurements of the fishes. RA performed the statistical analysis under the guidance of CGM. RA and CGM wrote the first draft of the manuscript. All authors contributed to the manuscript revision and read and approved the submitted version.
Acknowledgments
We thank Zachary Hutchens, Jordan Griffin, Valerie Castillo, and Juan Lara for valuable help during 2018-2019 field collections and laboratory work. We also thank Rony Peterson Santos Almeida for help and discussion of the functional diversity analyses. Ryka Shea, Tristan Brownjohn, and Daniel Unger for help with the geographic distribution map and land cover maps. Finally, we thank two reviewers for their constructive comments on the manuscript.
Conflict of interest
The authors declare that the research was conducted in the absence of any commercial or financial relationships that could be construed as a potential conflict of interest.
Publisher’s note
All claims expressed in this article are solely those of the authors and do not necessarily represent those of their affiliated organizations, or those of the publisher, the editors and the reviewers. Any product that may be evaluated in this article, or claim that may be made by its manufacturer, is not guaranteed or endorsed by the publisher.
Supplementary material
The Supplementary Material for this article can be found online at: https://www.frontiersin.org/articles/10.3389/fenvs.2022.965291/full#supplementary-material
References
Ahle, R. C. (1991). A study of three creeks in Nacogdoches County, Texas using an index of biological integrity. Unpubl. M.S. thesis. Nacogdoches, (TX): Stephen F. Austin State University.
Anderson, A. A., Hubbs, C., Winemiller, K., and Edwards, R. J. (1995). Texas freshwater fish assemblages following three decades of environmental change. Southwest. Nat 40, 314
Bittinger, K. (2020). usedist: Distance matrix utilities. R package version 0.4.0 Available at: https://CRAN.R-project.org/package=usedist.
Blonder, B., and Harris, D. J. (2019). hypervolume: High dimensional geometry and set operations using kernel density estimation, support vector machines, and convex hulls. R package version 2.0.12 Available at: https://CRAN.R-project.org/package=hypervolume.
Boizard, J., Magnan, P., and Angers, B. (2009). Effects of dynamic landscape elements on fish dispersal: The example of creek chub (Semotilus atromaculatus). Mol. Ecol. 18, 430–441. doi:10.1111/j.1365-294X.2008.04044.x
Bonner, T. H., Thomas, C., Williams, C. S., and Karges, J. P. (2005). Temporal assessment of a West Texas stream fish assemblage. Southwest. Nat. 50, 74–78. doi:10.1894/0038-4909(2005)050<0074:taoawt>2.0.co;2
Boon, P. J., Davies, B. R., and Petts, G. E. (2000). Global perspectives on river conservation: Science, policy and practice. Chichester: Wiley-Blackwell.
Booth, D. B., Roy, A. H., Smith, B., and Capps, K. A. (2016). Global perspectives on the urban stream syndrome. Freshw. Sci. 35, 412–420. doi:10.1086/684940
Bower, L. M., and Winemiller, K. O. (2019). Intercontinental trends in functional and phylogenetic structure of stream fish assemblages. Ecol. Evol. 9, 13862–13876. doi:10.1002/ece3.5823
Cadotte, M. W., Carscadden, K., and Mirotchnick, N. (2011). Beyond species: Functional diversity and the maintenance of ecological processes and services. J. Appl. Ecol. 48, 1079–1087. doi:10.1111/j.1365-2664.2011.02048.x
Cardoso, P., Mammola, S., Rigal, F., and Carvalho, J. (2021). Bat: Biodiversity assessment tools. R package version 2.7.1. Available at: https://CRAN.R-project.org/package=BAT.
Carey, M. P., Sanderson, B. L., Barnas, K. A., and Olden, J. D. (2012). Native invaders- challenges for science, management, policy, and society. Front. Ecol. Environ. 10, 373–381. doi:10.1890/110060
Carvajal-Quintero, J. D., Januchowski-Hartley, S. R., Maldonado-Ocampo, J. A., Jézéquel, C., Delgado, J., and Tedesco, P. A. (2017). Damming fragments species’ ranges and heightens extinction risk. Conserv. Lett. 10, 708–716. doi:10.1111/conl.12336
Carvajal-quintero, J. D., Villalobos, F., Oberdorff, T., Grenouillet, G., Brosse, S., Hugueny, B., et al. (2019). Drainage network position and historical connectivity explain global patterns in freshwater fishes’ range size. PNAS 116, 13434–13439. doi:10.1073/pnas.1902484116
Chazdon, R. L., Chao, A., Colwell, R. K., Lin, S. Y., Norden, N., Letcher, S. G., et al. (2011). A novel statistical method for classifying habitat generalists and specialists. Ecology 92, 1332–1343. doi:10.1890/10-1345.1
Cilleros, K., Allard, L., Grenouillet, G., and Brosse, S. (2016). Taxonomic and functional diversity patterns reveal different processes shaping European and Amazonian stream fish assemblages. J. Biogeogr. 43, 1832–1843. doi:10.1111/jbi.12839
Craig, C. A., and Bonner, T. H. (2021). Spring flow lost: A historical and contemporary perspective of an urban fish community. Urban Ecosyst. 24, 417–427. doi:10.1007/s11252-020-01047-6
D’Orazio, M. (2020). StatMatch: Statistical matching or data fusion. R package version 1.4.Available at: https://CRAN.R-project.org/package=StatMatch.
Dornelas, M., Magurran, A. E., Buckland, S. T., Chao, A., Chazdon, R. L., Colwell, R. K., et al. (2013). Quantifying temporal change in biodiversity: Challenges and opportunities. Proc. R. Soc. B Biol. Sci. 280, 20121931. doi:10.1098/rspb.2012.1931
Fitzgerald, D. G., Lanno, R. P., and Dixon, G. (1999). A comparison of a sentinel species evaluation using creek chub (Sematilus atromaculatus Mitchill) to a fish community evaluation for the initial identification of environmental stressors in small streams. Ecotoxicology 8, 33–48. doi:10.1023/a:1008853413528
R. Fricke, W. N. Eschmeyer, and R. Van der Laan (Editors) (2022). Eschmeyer's catalog of fishes: Genera, species, references. Available at: http://researcharchive.calacademy.org/research/ichthyology/catalog/fishcatmain.asp). Electronic version (accessed October 20, 2022).
Fuller, P. L., Nico, L. G., and Williams, J. D. (1999). Nonindigenous fishes introduced into inland waters of the United States. Bethesda: American Fisheries Society.
Hartig, F. (2021). DHARMa: Residual diagnostics for hierarchical (Multi-Level/mixed) regression models. R package version 0.4.Available at: https://CRAN.R-project.org/package=DHARMa.
Hughes, R. M., and Noss, R. F. (1992). Biological diversity and biological integrity: Current concerns for lakes and streams. Fisheries 17, 11–19. doi:10.1577/1548-8446(1992)017<0011:bamonr>2.0.co;2
Jackson, D. A., Peres-Neto, P. R., and Olden, J. D. (2001). What controls who is where in freshwater fish communities the roles of biotic, abiotic, and spatial factors. Can. J. Fish. Aquat. Sci. 58, 157–170. doi:10.1139/f00-239
Jia, Y., Kennard, M. J., Liu, Y., Sui, X., Li, K., Wang, G., et al. (2020). Human disturbance and long-term changes in fish taxonomic, functional and phylogenetic diversity in the Yellow River, China. Hydrobiologia 847, 3711–3725. doi:10.1007/s10750-020-04244-8
Linam, G. W., Kleinsasser, L. J., and Mayes, K. (2002). Regionalization of the index of biotic integrity for Texas streams. Austin, TX: Report. Texas Parks and Wildlife Department.
Lombardi, J. V., Comer, C. E., Scognamillo, D. G., and Conway, W. C. (2017). Coyote, fox, and bobcat response to anthropogenic and natural landscape features in a small urban area. Urban Ecosyst. 20, 1239–1248. doi:10.1007/s11252-017-0676-z
Marchetti, M. P., and Moyle, P. B. (2001). Effects of flow regime on fish assemblages in a regulated California stream. Ecol. Appl. 11, 530–539. doi:10.1890/1051-0761(2001)011[0530:eofrof]2.0.co;2
Mason, N. W., Mouillot, D., Lee, W. G., and Wilson, J. B. (2005). Functional richness, functional evenness and functional divergence: The primary components of functional diversity. Oikos 111, 112–118. doi:10.1111/j.0030-1299.2005.13886.x
May, R. M. (1986). The search for patterns in the balance of nature: Advances and retreats. Ecology 67, 1115–1126. doi:10.2307/1938668
Mayes, K. B., Wilde, G. R., McGarrity, M. E., Wolaver, B. D., and Caldwell, T. G. (2019). Watershed-scale conservation of native fishes in the brazos river basin, Texas. Am. Fish. Soc. Symp. 91, 315–343.
McKinney, M. L. (2006). Urbanization as a major cause of biotic homogenization. Biol. Conserv. 127, 247–260. doi:10.1016/j.biocon.2005.09.005
Meador, M. R., Coles, J. F., and Zappia, H. (2005). Fish assemblage responses to urban intensity gradients in contrasting metropolitan areas: Birmingham, Alabama and boston, Massachusetts. Am. Fish. Soc. Symp. 47, 409–423.
Montaña, C. G., and Schalk, C. M. (2018). Correlates of elemental isotopic composition of stream fishes: The importance of land use, species identity and body size. J. Fish. Biol. 62, 944–960. doi:10.1111/jfb.13554
Montaña, C. G., and Winemiller, K. O. (2010). Local-scale habitat influences morphological diversity of species assemblages of cichlid fishes in a tropical floodplain river. Ecol. Freshw. Fish. 19, 216–227. doi:10.1111/j.1600-0633.2010.00406.x
Montaña, C. G., Winemiller, K. O., and Sutton, A. (2014). Intercontinental comparison of fish ecomorphology: Null model tests of community assembly at the patch scale in rivers. Ecol. Monogr. 84, 91–107. doi:10.1890/13-0708.1
Mouchet, M. A., Villéger, S., Mason, N. W. H., and Mouillot, D. (2010). Functional diversity measures: An overview of their redundancy and their ability to discriminate community assembly rules. Funct. Ecol. 24, 867–876. doi:10.1111/j.1365-2435.2010.01695.x
Nakamura, G., Vicentin, W., and Suarez, Y. R. (2018). Functional and phylogenetic dimensions are more important than the taxonomic dimension for capturing variation in stream fish communities. Austral Ecol. 43, 2–12. doi:10.1111/aec.12529
Oksanen, J., Blanchet, F. G., Friendly, M., Kindt, R., Legendre, P., McGlinn, D., et al. (2020). vegan: Community ecology package. R package version 2.5-Available at: https://CRAN.R-project.org/package=vegan.
Onorato, D., Angus, R. A., and Marion, K. R. (2000). Historical changes in the ichthyofaunal assemblages of the upper Cahaba River in Alabama associated with extensive urban development in the watershed. J. Freshw. Ecol. 15, 47–63. doi:10.1080/02705060.2000.9663721
Parks, T. P., Quist, M. C., and Pierce, C. L. (2014). Historical changes in fish assemblage structure in midwestern non wadeable rivers. Am. Midl. Nat. 171, 27–53. doi:10.1674/0003-0031-171.1.27
Pease, J. E., Grabowski, T. B., Pease, A. A., and Bean, P. T. (2018). Changing environmental gradients over forty years alter ecomorphological variation in Guadalupe Bass Micropterus treculii throughout a river basin. Ecol. Evol. 8, 8508–8522. doi:10.1002/ece3.4349
Perkin, J. S., and Bonner, T. H. (2016). Historical changes in fish assemblage composition following water quality improvement in the mainstem Trinity River of Texas. River Res. Appl. 32, 85–99. doi:10.1002/rra.2852
R Core Team (2017). R: A language and environment for statistical computing. Vienna, Austria: R Foundation for Statistical Computing.
Rahel, F. J., Bierwagen, B., and Taniguchi, Y. (2008). Managing aquatic species of conservation concern in the face of climate change and invasive species. Conserv. Biol. 22 (3), 551–561. doi:10.1111/j.1523-1739.2008.00953.x
Roberts, D. W. (2019). labdsv: Ordination and multivariate analysis for ecology. R package version 2.0-Available at: https://CRAN.R-project.org/package=labdsv.
Schweizer, P. E., and Matlack, G. R. (2005). Annual variation in fish assemblages of watersheds with stable and changing land use. Am. Midl. Nat. 153, 293–308. doi:10.1674/0003-0031(2005)153[0293:avifao]2.0.co;2
Simberloff, D. (2011). “Native invaders,” in Encyclopedia of biological invasions. Editors D. Simberloff, and M. Rejmanek (Berkeley, CA: University of California Press), 472.
Stair, D. M., Tolbert, V. R., and Vaughan, G. L. (1984). Comparison of growth, population structure, and food of the creek chub Semotilus atromaculatus in undisturbed and surface-mining-disturbed streams in Tennessee. Environ. Pollut. 35, 331–343. doi:10.1016/0143-1471(84)90078-3
Su, G., Logez, M., Xu, J., Tao, S., Villéger, S., and Brosse, S. (2021). Human impacts on global freshwater fish biodiversity. Science 371, 835–838. doi:10.1126/science.abd3369
Swanson, J. (2022). Fish community assemblage at multiple spatial scales in east Texas streams. Unpubl. M.S. thesis. Nacogdoches, (TX) Stephen F. Austin State University.
Taniguchi, Y., Rahel, F. J., Novinger, D. C., and Gerow, K. G. (1998). Temperature mediation of competitive interactions among three fish species that replace each other along longitudinal stream gradients. Can. J. Fish. Aquat. Sci. 55, 1894–1901. doi:10.1139/f98-072
Tilman, D., Knops, J., Wedin, D., Reich, P., Ritchie, M., and Siemann, E. (1997). The influence of functional diversity and composition on ecosystem processes. Science 277, 1300–1302. doi:10.1126/science.277.5330.1300
Toussaint, A., Charpin, N., Beauchard, O., Grenouillet, G., Oberdorff, T., Tedesco, P. A., et al. (2018). Non-native species led to marked shifts in functional diversity of the world freshwater fish faunas. Ecol. Lett. 21 (11), 1649–1659. doi:10.1111/ele.13141
TWDB Texas Water Development Board (2021). Regional water plan county population projections. Available at: https://www3.twdb.texas.gov/apps/reports/Projections/2022%20Reports/pop_county (Accessed October 18, 2021).
U.S. Census Bureau (2011). Statistical abstract of the United States: 2012. 131st edition. Washington DC.
U.S. Census Bureau (2020). Statistical of Nacogdoches County Texas 2020. Available at: https://www.census.gov/quickfacts/fact/table/nacogdochescitytexas/POP010220#POP010220.
Van-Metre, P. C., Waite, I. R., Qi, S., Mahler, B., Terando, A., Wieczorek, M., et al. (2019). Projected urban growth in the southeastern USA puts small streams at risk. PloS One 14, e0222714. doi:10.1371/journal.pone.0222714
Villéger, S., Miranda, J. R., Hernández, D. F., and Mouillot, D. (2010). Contrasting changes in taxonomic vs. functional diversity of tropical fish communities after habitat degradation. Ecol. Appl. 20, 1512–1522. doi:10.1890/09-1310.1
Violle, C., Navas, M. L., Vile, D., Kazakou, E., Fortunel, C., Hummel, I., et al. (2007). Let the concept of trait be functional. Oikos 116, 882–892. doi:10.1111/j.0030-1299.2007.15559.x
Walsh, C. J., Roy, A. H., Feminella, J. W., Cottingham, P. D., Groffman, P. M., and Morgan, R. P. (2005). The urban stream syndrome: Current knowledge and the search for a cure. J. North Am. Benthol. Soc. 24, 706–723. doi:10.1899/04-028.1
Walters, D. M., Freeman, M. C., Leigh, D. S., Freeman, B. J., and Pringle, C. M. (2005). Urbanization effects on fishes and habitat quality in a southern Piedmont River basin. Am. Fish. Soc. Symp. 47, 69.
Walters, D. M., Leigh, D. S., and Bearden, A. B. (2003). “Urbanization, sedimentation, and the homogenization of fish assemblages in the Etowah River Basin, USA,” in The interactions between sediments and water. Editor B. Kronvang (Dordrecht, Netherlands: Springer), 5.
Wang, L., Lyons, J., Kanehi, P., Bannerman, R., and Emmons, E. (2000). Watershed urbanization and changes in fish communities in southeastern Wisconsin streams. J. Am. Water Resour. Assoc. 36, 1173–1189. doi:10.1111/j.1752-1688.2000.tb05719.x
Weaver, L. A., and Garman, G. C. (1994). Urbanization of a watershed and historical changes in a stream fish assemblage. Trans. Am. Fish. Soc. 123, 162–172. doi:10.1577/1548-8659(1994)123<0162:UOAWAH>2
Williams, C. S., and Bonner, T. H. (2006). Habitat associations, life history and diet of the Sabine shiner Notropis sabinae in an east Texas drainage. Am. Midl. Nat. 155, 84–102. doi:10.1674/0003-0031(2006)155[0084:HALHAD]2.0.CO;2
Williams, C. S., and Bonner, T. H. (2007). Threatened fishes of the world: Notropis sabinae (Jordan and gilbert 1886) (Cyprinidae). Environ. Biol. Fishes 80, 7–8. doi:10.1007/s10641-006-9118-0
Winemiller, K. O. (1991). Ecomorphological diversification in lowland freshwater fish assemblages from five biotic regions. Ecol. Monogr. 61, 343–365. doi:10.2307/2937046
Keywords: functional richness, east texas, freshwater fishes, niche specialist, species composition, temporal changes
Citation: Antoniazzi R, Montaña CG, Peterson D and Schalk CM (2023) Changes in taxonomic and functional diversity of an urban stream fish assemblage: A 30-year perspective. Front. Environ. Sci. 10:965291. doi: 10.3389/fenvs.2022.965291
Received: 09 June 2022; Accepted: 15 December 2022;
Published: 06 January 2023.
Edited by:
Juan Jose Rosso, Consejo Nacional de Investigaciones Científicas y Técnicas (CONICET), ArgentinaReviewed by:
Casey Pennock, Utah State University, United StatesPablo Scarabotti, National Institute of Limnology (INALI), Argentina
Copyright © 2023 Antoniazzi, Montaña, Peterson and Schalk. This is an open-access article distributed under the terms of the Creative Commons Attribution License (CC BY). The use, distribution or reproduction in other forums is permitted, provided the original author(s) and the copyright owner(s) are credited and that the original publication in this journal is cited, in accordance with accepted academic practice. No use, distribution or reproduction is permitted which does not comply with these terms.
*Correspondence: Carmen G. Montaña, bW9udGFuYXNjZ0BzZmFzdS5lZHUg
†Present address: Christopher M. Schalk, Southern Research Station, USDA Forest Service, Nacogdoches, TX, United States