- 1Key Laboratory of Yangtze River Water Environment of the Ministry of Education, College of Environmental Science and Engineering, Tongji University, Shanghai, China
- 2Shanghai Institute of Pollution Control and Ecological Security, Shanghai, China
Organotin compounds (OTCs) are a category of persistent toxic substances with endocrine disrupting effects and may pose a risk to aquatic organisms and human health via water. This study collected 78 surface water samples from the drinking water source in the upper Yangtze River Estuary from July 2018 to November 2019. Out of the nine OTC targets, tributyltin (TBT), dimethyltin (DMT), trimethyltin (TMT), dibutyltin (DBT), and monobutyltin (MBT) were detected from more than 50% of the water samples, with cumulative concentrations of OTCs ranging from 42.5 ± 54.0 to 421 ± 176 ng Sn L−1. The composition and concentrations of OTCs in the stormy months were different from those in other months. The concentrations of OTCs with the ebbing tide exceeded those in the flooding tide and increased when the flooding tide transitioned to the ebbing tide. A pulsed trend in concentrations of OTCs in river water was evident due to the impact of rainfall runoff and tidal currents. Identified sources of OTCs included ship antifouling paints, agricultural pesticides, and plastic waste. TBT in water sources poses a low risk to human health and a risk to the ecosystem, particularly during the stormy months.
Introduction
Safe drinking water is important to human health (Krishna et al., 2020). However, drinking water pollution is becoming a global concern (Chen Z. B. et al., 2019; Ighalo et al., 2021). With increasing development, water quality safety is no longer limited not only to conventional water pollutants but also to contamination by persistent toxic substances (PTSs). Organotin compounds (OTCs) are a category of PTS and have been detected in various water environments, including ports, harbors, and coastal regions (Cao et al., 2009; Anastasiou et al., 2016; Concha-Graña et al., 2021). OTCs persist in the environment for a long time due to their extensive use and resistance to degradation (Dobson et al., 2006; Cole et al., 2018).
OTCs cause various forms of damage to aquatic organisms, such as malformation of shellfish shells (Alzieu, 2000; Garnier et al., 2020), imposex in gastropods (Cao et al., 2009), and obesity metabolic syndrome in fish (Meador et al., 2011). In addition, OTCs have negative effects on mammalian health, and particularly on human health, with damaging effects on reproduction, endocrine function, the liver, the immune system, and the nervous system (Zheng et al., 2011; Graceli et al., 2013). OTCs, and particularly TBT and triphenyltin (TPhT), act as agonists for the peroxisome proliferator-activated receptor (PPAR) γ and promote adipocyte differentiation (Hiromori et al., 2009). Given the toxicity of TBT to organisms and its persistence in the aquatic environment, it has been globally banned since 2008 by the International Convention on the Control of Harmful Anti-fouling Systems on Ships (IMO, 2001). Some other OTCs are also regulated in many products by various countries and regions (IMO, 2001; Concha-Graña et al., 2021). However, pollution problems resulting from OTC contamination of the aquatic environment persist (Antizar-Ladislao, 2008; Cole et al., 2015; Zhang et al., 2017).
The concentrations of OTCs in water generally range from a few to thousands of nanograms per liter (Díez et al., 2005; Gao et al., 2013; Gao et al., 2018; Concha-Graña et al., 2021). OTCs have also been detected in the biota and sediments of the Yangtze River Estuary (Chen et al., 2016; Chen C. Z. et al., 2019) and have been reported in the water of the turbidity maximum zone and hypoxic zone outside of an estuary (Huang et al., 2021; Chen et al., 2022). The tidal reaches of the Yangtze River, also known as the upper reaches of the Yangtze River Estuary, experience strong reciprocating interactions. However, there remains limited information on OTC pollution in the upper Yangtze River Estuary drinking water source and the tidal effects on OTCs. Therefore, the present study aimed to analyze the concentrations, compositions, temporal distribution, source, and risks of OTCs in the tidal drinking water source in the upper Yangtze River Estuary.
Study area, materials, and methods
Materials and chemicals
Organotin standards, including monomethyltin trichloride (MMT, ≥ 95%), dimethyltin dichloride (DMT, ≥ 98%), trimethyltin monochloride (TMT, ≥ 99%), monobutyltin trichloride (MBT, ≥ 96%), dibutyltin dichloride (DBT, ≥ 93%), tributyltin monochloride (TBT, ≥ 96%), monophenyltin trichloride (MPhT, ≥ 95.5%), diphenyltin dichloride (DPhT, ≥ 96%), and triphenyltin monochloride (TPhT, ≥ 99%) were purchased from ANPEL Laboratory Technologies (Shanghai) Inc. In addition, tri-n-propyltin chloride (TPrT, ≥ 95%) as an internal standard, sodium tetraethyl borate (NaBEt4, ≥ 92%), acetic acid [high-performance liquid chromatography (HPLC) grade], methanol (HPLC grade), n-hexane (HPLC grade), sodium acetate [analytical (AR) grade], NaOH (AR grade), KOH (AR grade), and ammonium chloride (AR grade) were purchased from ANPEL. Supplementary Table S1 provides more detailed information on the organotin standards used. An ultrapure water machine (25°C, 18.2 MΩ cm, Merck Millipore, Germany) was used for preparing Milli-Q water.
Study area and sample collection
The study area of the present study is in the upper Yangtze River Estuary (Figure 1). The study area is bounded by farmlands, industrial areas, loading docks, and ports and is tidal. Sampling extended from July 2018 to November 2019, during which 78 surface water samples were collected at a depth of 20 cm (Dai et al., 2022). Supplementary Table S2 provides more detailed information on the seven sampling campaigns implemented in the present study. Tidal samples were collected across the entire sampling period, except for the stormy months of July 2018 and July 2019. All samples were filtered through glass fiber membranes (φ = 30 cm, pore size = 1 μm, and 400°C for 6 h) for pretreatment before OTC analysis.
Sample preparation
The sample pretreatment process followed that of Chen et al. (2022) and is described as follows: from each filtered sample, 1 L was stored in a brown high borosilicate glass bottle and then passed through a strong cation exchange (SCX) column (500 mg/6 ml) at a rate of 2 ml min−1. The SCX columns were activated by 5 ml of methanol in advance. Subsequently, 5 ml of eluent solution containing 0.8 mol L−1 ammonium chloride with a water–methanol ratio (V/V) of 3:7 was used as the solvent to elute the enriched OTCs, and the eluent solution was transferred to a 50-ml brown glass tube. Section 2.3 in the Supplementary Text provides more information. Then, 1 ml of 2 mol L−1 NaOH, 20 ml of 1 mol L−1 acetic acid/sodium acetate buffer solution (CH3COOH/CH3COONa, pH ≈ 4.5), 100 µl of 1 mg L−1 internal standard (TPrT), 4 ml of n-hexane, and 0.6 ml of 1% NaBEt4 solution were added to the 50-ml brown glass tube. The mixed solution was horizontally placed in a shaker for derivatization (150 r·min−1 for 60 min). After derivatization, the mixed solution was allowed to settle for 30 min. Subsequently, 2 ml of the upper organic phase was extracted and transferred to nitrogen tubes. The extracts were concentrated to 0.5 ml by nitrogen blowing to accelerate evaporation of the organic phase. The concentrated samples were then analyzed by gas chromatographic– mass spectrometry (GC-MS).
Instrumental analysis
OTCs were detected by GC-MS (QP2010 Plus, Shimadzu, Japan) in the electron ionization mode at 70 eV with a capillary column (HP−5MS, 30 m × 0.25 mm i.d., 0.25-µm film thickness) (Chen et al., 2022). An inlet temperature of 250°C and splitless mode were adopted. An injection volume of 1 μl and a column flow rate of 1.00 ml min−1 with a constant current were adopted. The high-purity helium carrier gas was used (99.99%). The temperatures of the ion source and mass spectrometer interface were 230 and 280°C, respectively. The column temperature for TMT was programmed as 5 min at 40°C and from 40 to 200°C at 10°C min−1. The column temperature for the remaining eight OTCs was programmed as 5 min at 60°C, from 60 to 150°C (1 min) at 10°C min−1, and from 150 to 290°C (2 min) at 8°C min−1. Supplementary Table S1 lists the GC-MS parameters of the quantitative and qualitative ions of OTCs.
Quality assurance/quality control (QA/QC)
No OTC was detected in procedural blanks. The limit of detection (LOD) as the signal-to-noise ratio of 3 (S/N = 3) and limit of quantification (LOQ) as S/N = 10 ranged from 0.91 to 1.37 ng Sn L−1 and 3.02–4.58 ng Sn L−1, respectively. The recoveries of the nine OTCs in spiked samples ranged from 85.4 to 112.4%, within <5.4% of the relative standard derivations (RSDs). The regression coefficients (R2) of nine OTCs were all higher than 0.99 within the tested concentrations (0–500 μg L−1). Supplementary Table S3 provides more detailed information.
Butyltin degradation index
The butyltin degradation index (BDI) was used to estimate the input age and degree of degradation of TBT (Quintas et al., 2019). A BDI ≤1 suggests recent inputs of TBT, whereas BDI >1 suggests TBT degraded to DBT or MBT:
where [MBT], [DBT], and [TBT] are the concentrations of MBT, DBT, and TBT, respectively.
Risk assessment
Ecological risk assessment
The risk quotient I (RQ I) was used to assess the risk to the aquatic ecology associated with chemical pollution. A RQ I > 1.0 indicates a severe risk of OTCs to the ecosystem and the need to implement control measures or remedial actions as soon as possible. A 0.1 < RQ I < 1.0 indicates a moderate risk to the ecosystem, whereas a RQ I < 0.1 indicates a low risk. The RQ I was calculated as:
where MEC95 is in the 95th percentile of the measured environmental concentration (ng Sn L−1) corresponding to a cumulative frequency distribution and PNECfw is the minimum value of the predicted no-effect concentration in freshwater (ng Sn L−1). The PNECfw is based on the quantitative structure–activity relationship (QSAR) prediction and calculation, provided by the NORMAN network (https://www.norman-network.com/nds/ecotox/).
Human health risk assessment
Equations 3, 4 were used to assess the risk posed to human health by OTCs (Fenner et al., 2002; USEPA, 2021). The non-carcinogenic risk posed by OTCs to human health can also be further divided into high, moderate, and low risks according to the RQ II levels RQ II > 1.0, 0.1 < RQ II < 1.0, and RQ II < 0.1, respectively.
where SL is the OTC screening level (µg L−1) and THQ is the target hazard quotient, with a default value of 1. non-carcinogen averaging time (Atn, d) = ED [exposure duration (y)] × 365 d/y. Body weight (BW) and the tap water ingestion rate (IRW) were 65 kg and 1.7 L d−1, respectively. EF (exposure frequency) was 350 days y−1. RQ II is the risk quotient and Ci is the concentration of each OTC. RfDo (mg·kg−1 BW d−1) is the oral reference dose of OTCs, which is often replaced by the tolerable daily intake (TDI) due to the lack of RfDo values for OTCs. No TDI values for MMT, MBT, and TBT were found. The TDI values of TBT and TPhT were 0.25 μg kg−1 BW d−1 and 0.5 μg kg−1 BW d−1, respectively (Penninks, 1993; Tsuda et al., 1995). The TDI values of MBT, DBT, MPhT, and DPhT were 5.42 μg kg−1 BW d−1, 1.75 μg kg−1 BW d−1, 10.84 μg kg−1 BW d−1, and 3.50 μg kg−1 BW d−1, respectively, and were calculated using an in vitro immunotoxicity exposure test (Whalen et al., 1999; Whalen et al., 2000).
Data processing
The present study constructed a map of the study area and sampling site using Surfer 16. Data, figures, and tables were generated in Excel 2019 and Origin 2017. As the data did not conform to a normal distribution (Supplementary Table S4), the Kruskal–Wallis test in IBM SPSS Statistics 23.0 was used for statistical analysis, with a significant level of 0.05. Values below LOD were calculated using the expression LOD/2.
Results
Occurrences of the nine OTCs
Nine of the target OTCs were detected in the drinking water source of the upper Yangtze River Estuary (Table 1). However, their detection frequencies varied from 6.4 to 74%. Five OTCs were detected at a frequency exceeding 50%, namely, TBT (74%), DMT (71%), TMT (63%), DBT (59%), and MBT (54%). The cumulative concentration of the nine OTCs was 191 ± 154 ng Sn L−1, with a maximum of 698 ng Sn L−1. The maximum concentrations of the various OTCs varied from 15.6 ng Sn L−1 (MMT) to 358 ng Sn L−1 (MBT), MEC95 values ranged from 10.8 ng Sn L−1 (MMT) to 287 ng Sn L−1 (MBT), and average concentrations of individual OTCs ranged from 0.97 ng Sn L−1 (MMT) to 72.9 ng Sn L−1 (DMT). The maximum concentrations of TBT and TPhT were relatively similar at 295 ng Sn L−1 and 277 ng Sn L−1, respectively, although their detection frequencies were different at 74 and 14%, respectively.
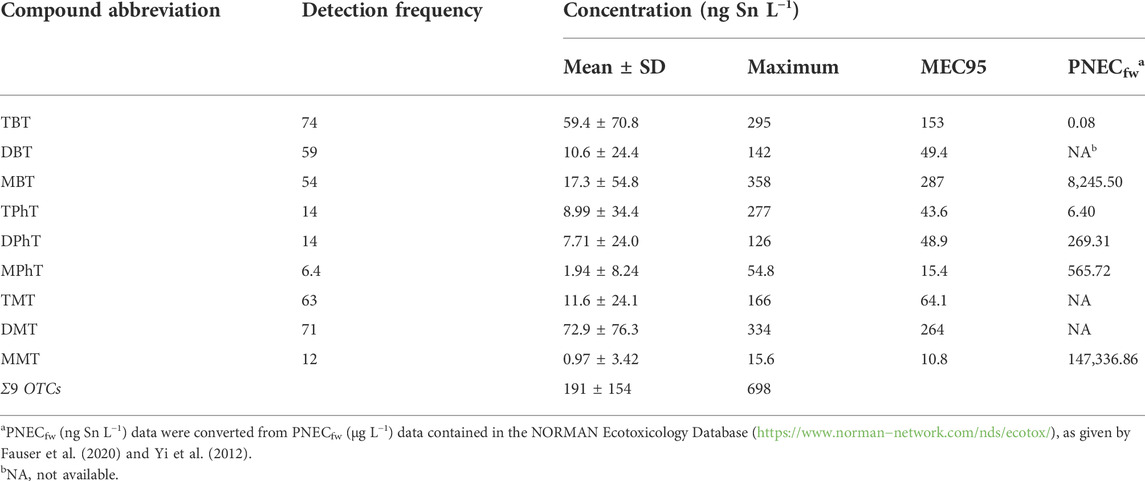
TABLE 1. Statistical summary of organotin compounds (OTCs), including mean, standard deviation (SD), maximum concentrations, 95th percentile of the measured environmental concentration (MEC95), and the lowest value of predicted no-effect concentration in freshwater (PNECfw) in the drinking water source in the upper Yangtze River Estuary.
Concentration, composition, and proportion analysis of the nine OTCs
During the period July 2018–November 2019 (Figure 2A), the cumulative concentrations of the nine target OTCs (Σ9 OTCs) were higher on average in the stormy months (389 ± 241 ng Sn L−1 in July 2018; 421 ± 176 ng Sn L−1 in July 2019) than in the remaining months. Low Σ9 OTCs values were detected in the cold months (42.5 ± 54.0 ng Sn L−1 in December 2018; 65.4 ± 79.8 ng Sn L−1 in February 2019) and were significantly different (p < 0.05) from those in the stormy months. The mean concentrations of Σ9 OTCs in the remaining months (May 2019; September 2019; November 2019) ranged from 165 ± 88.7 ng Sn L−1 to 189 ± 131 ng Sn L−1. As shown in Figure 2B, the mean Σ9 OTCs in the ebbing tide exceeded those in the flooding tide, indicating that OTCs mainly originated from river runoff. While there was no significant difference between flooding tide and ebbing tide OTCs within the same sampling campaign (p > 0.05), some significant differences in the mean concentration of Σ9 OTCs were detected among the different sampling campaigns [ebbing tide in May 2019 vs. flooding tide and ebbing tide in the cold months (December 2018; February 2019)].
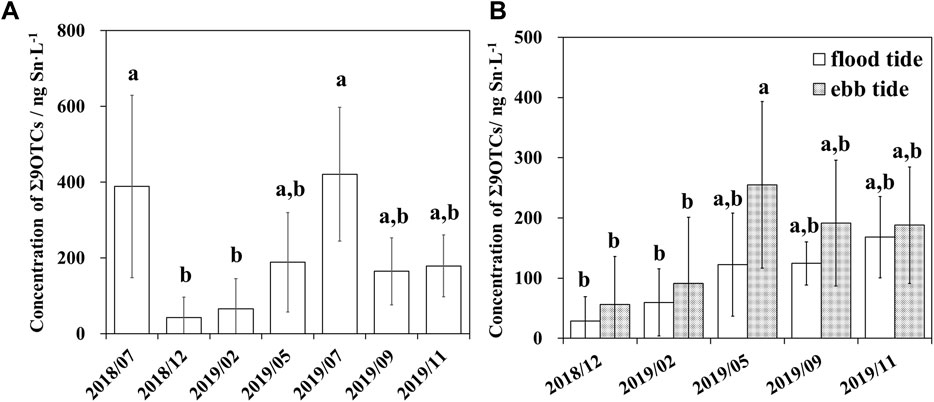
FIGURE 2. Differences in mean cumulative concentrations of nine organotin compounds (Σ9 OTCs) among different sampling campaigns (A) and different tidal phases (B) in the drinking water source in the upper Yangtze River Estuary. Letters “a” and “b” represent significant differences (p < 0.05).
As shown in Table 2, OTCs were mainly dominated by TBT in stormy months (July 2018; July 2019) and DMT in the remaining months. MPhT was only detected in September 2019, whereas DPhT and TPhT were only detected in the stormy months (July 2018; July 2019), and MMT was detected in the cold months (December 2018; February 2019) and in the stormy months (July 2018; July 2019). Thus, particular attention needs to be focused on DMT and TBT, whereas focus on MMT, MPhT, DPhT, and TPhT should differ among the different months of the year.
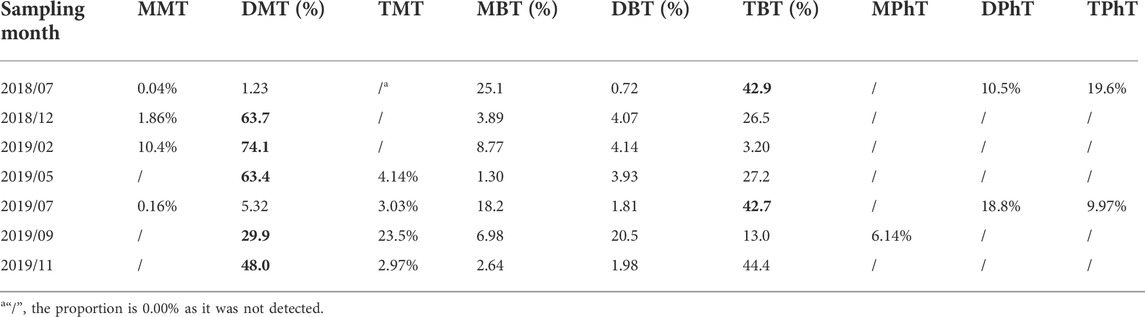
TABLE 2. Composition and proportion of detected organotin compounds (OTCs) in the drinking water source in the upper Yangtze River Estuary.
Tidal pulsing of the nine OTCs
As shown in Figure 3, the largest changes in concentration among OTCs occurred in DMT and TBT. The concentration of DMT increased substantially from the flooding tide to the ebbing tide in each sampling month, whereas it showed little change in November 2019. Meanwhile, there was an obvious increase in the concentration of TBT, except during September 2019 when there was no change. A higher concentration of DBT was detected in September 2019. There was a clear change in the composition of OTCs in the flooding tide in September 2019 in comparison to the remaining months due to the high proportion of TMT. MPhT was only detected during the flooding tide in September 2019. The concentrations of OTCs generally increased at the transition from the flooding tide to the ebbing tide and vice versa.
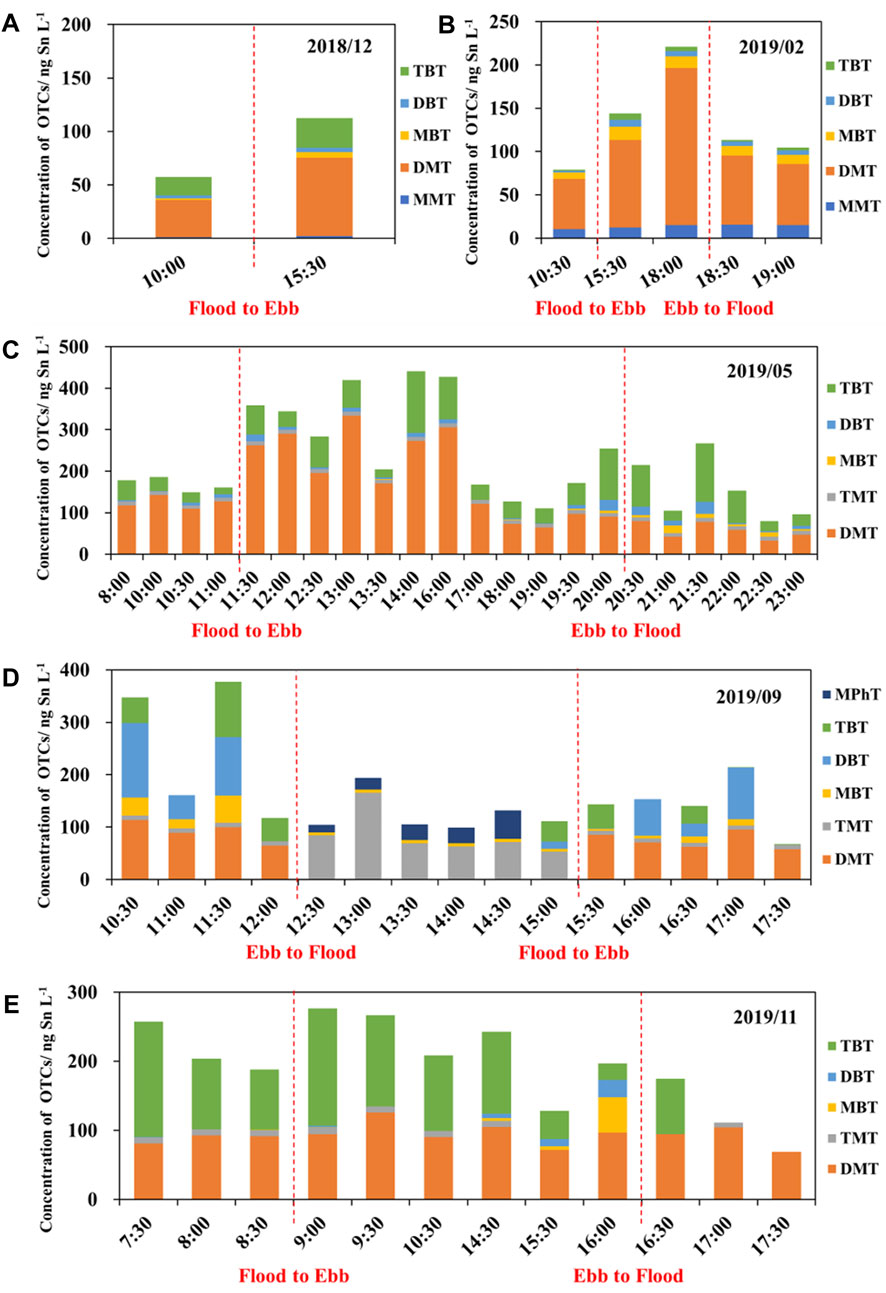
FIGURE 3. Compositions and concentrations of organotin compounds (OTCs) in the drinking water source of the Yangtze River Estuary under semi-diurnal tidal cycle for different months from December 2018 (A) to November 2019 (E).
Changes in BDI values
The source of OTCs can be reflected by the BDI since TBT is a typical OTC. As shown in Figure 4, a large proportion of BDI was <1. The BDI values for all months fluctuated around 1, except for February 2019 and July 2019 when the BDIs exceeded 1 and were less than 1, respectively. The mean BDIs for the different sampling months were 0.76 ± 1.44, 1.28 ± 1.14, 1.83 ± 2.34, 0.56 ± 0.78, 0.57 ± 0.24, 5.68 ± 5.76, and 0.82 ± 1.20, respectively. BDIs <1 were mainly concentrated in May 2019, November 2019, and during the stormy months (July 2018; July 2019). In contrast, BDIs >1 were concentrated in September 2019 and during the cold months (December 2018; February 2019).
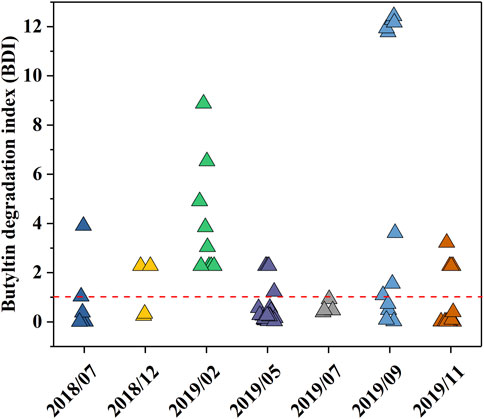
FIGURE 4. Variation in the butyltin degradation index (BDI) among different sampling months in the drinking water source in the upper Yangtze River Estuary.
Risk assessment
Ecological risk assessment
Figure 5 compares the ecological risks of OTCs among the different sampling months. Evaluation of the ecological risks posed by OTCs was quantitatively evaluated for MMT, MBT, TBT, MPhT, DPhT, and TPhT due to the lack of PNECfw values for the remaining OTCs. The RQ I values of MMT, MBT, and MPhT were all lower than 0.1 for each sampling month, indicating that these three OTCs have a low ecological risk. The RQ I value of DPhT was between 0.1 and 1 in the stormy months (July 2018; July 2019), indicating a moderate ecological risk. The RQ I value of TPhT exceeded 1, indicating a high ecological risk. The RQ I value of TBT exceeded 1 for each sampling month.
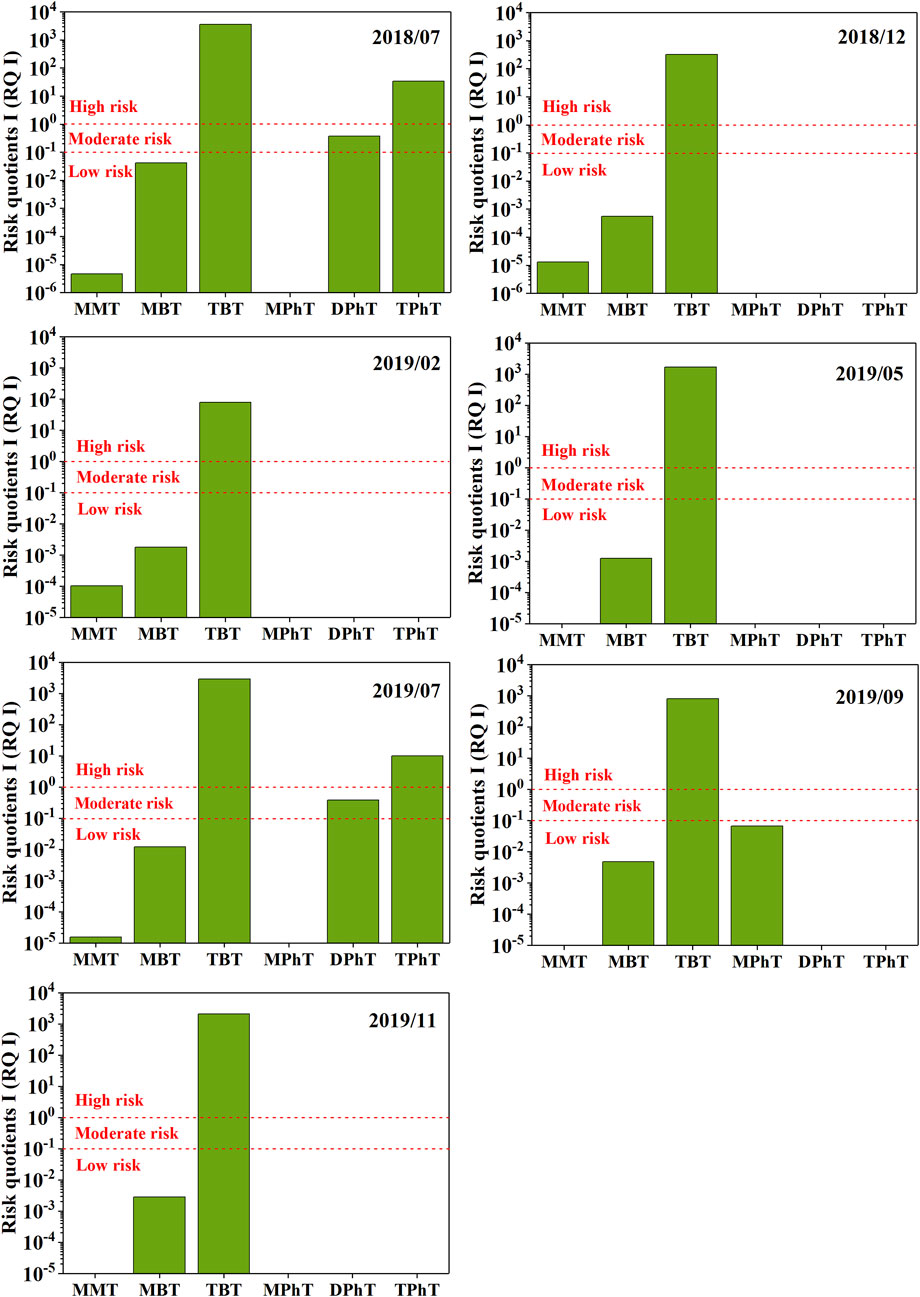
FIGURE 5. Risk quotient I (RQ I) for the ecological risk assessment of organotin compounds (OTCs) in the drinking water source in the upper Yangtze River Estuary.
Human health risk assessment
As shown in Figure 6, the RQ II values of OTCs in the drinking water source exceeded those in tap water from the drinking water treatment plant (DWTP) based on the OTC removal rate (Supplementary Table S5). However, both were lower than 0.1, indicating that OTCs in the study area pose a low risk to human health. Nevertheless, a focus on OTCs in the stormy months (July 2018; July 2019) is needed due to their relatively high RQ values.
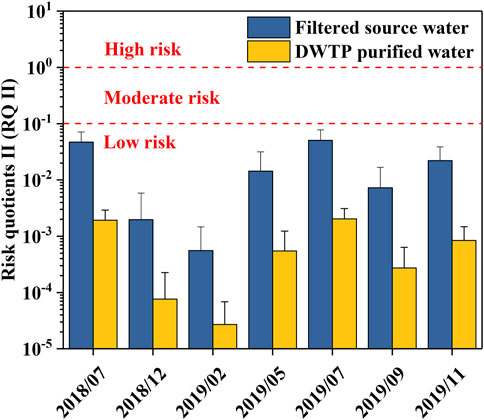
FIGURE 6. Risk quotient II (RQ II) for the assessment of the risk to human health by organotin compounds (OTCs) from the filtered source water and treated water from the drinking water treatment plant (DWTP).
Discussion
Effects of runoff and tidal currents
During the study period, the highest concentrations of OTCs were detected in the stormy months (Figure 2A and Table 2). These results suggested that rainfall runoff during the stormy months may be a source of OTCs in the river. Past studies have shown that rainfall runoff can increase the concentration of pollutants in the environment (Sharma et al., 2009; Xiao et al., 2012). Therefore, there is a need to focus on the stormy months when considering changes in concentrations and compositions of OTCs.
The present study observed no significant increases in the concentrations of OTCs in the upper reaches of the Yangtze River Estuary during the tidal transition, whereas concentrations in the ebbing tide generally exceeded those during the flooding tide (Figures 2B, 3). However, the concentrations of OTCs in the ebbing tide were lower than those in the flooding tide in the turbidity maximum zone and hypoxia zone outside the Yangtze Estuary. The concentration of dissolved OTCs and particulate OTCs in water will sharply increase due to sediment resuspension and the turbulent action of the hypoxic bottom water mass during the tidal transition, particularly from the flooding tide to the ebbing tide. This increase in concentration can be several orders of magnitude higher in the hypoxic zone and even up to a factor of 40 higher in the turbidity maximum zone (Huang et al., 2021; Chen et al., 2022).
There were clear differences in the changes in the concentrations of OTCs between the upper reach and the middle/lower reaches of the Yangtze River Estuary. This result indicated a different source of OTCs in the freshwater section compared with that in the saltwater section, and differences in sediment resuspension among different sections resulting from tidal action in the estuary.
Source analysis of OTCs
TBT is widely used in antifouling coatings for ships or related equipment, with the coatings painted onto ship hulls to reduce the growth of marine organisms (de Castro et al., 2012). Active antifouling compounds are exposed to water during ship maintenance or sailing and may release TBT (Kim et al., 2014). The results showed that the concentrations of TBT released increased during the stormy months (July 2018; July 2019), which indicates that the release of TBT in antifouling coatings is enhanced through scouring by rainfall (Figure 2A and Table 2). The majority of BDI was less than 1 (Figure 4), further confirming that the main inputs of TBT in the study area were from exogenous sources. The main sources of OTCs have been identified as intense shipping activities and stormwater, not only in this estuary but also in other places, such as Hong Kong (Ho et al., 2016).
The concentration of DBT as a product of TBT degradation increased in September 2019. The warmer temperature in September 2019 may have facilitated some biodegradation of TBT (Harino et al., 1997; Burton et al., 2004). In contrast, the high BDI values (BDI > 1) indicated that exogenous inputs were likely the main sources of MBT and DBT in the cold months (December 2018; February 2019). MBT and DBT are widely used in industrial and agricultural activities, mainly as industrial catalysts, wood preservatives, pesticides, fungicides, and stabilizers in plastics and polyurethane foam production (Hoch, 2001; Bhosle et al., 2006; Wang et al., 2010; Okoro et al., 2011; Cruz et al., 2015; Fang et al., 2017; Kucklick and Ellisor, 2019).
DMT and TMT were detected at high concentrations and frequencies in September 2019 (Tables 1, 2). Methyltins (MTs) are often released from polyvinyl chloride (PVC) films since MTs are also used as stabilizers in these products (Fristachi et al., 2009; Mahmood et al., 2020). In addition, MTs can originate from the methylation of inorganic tin during fermentation by different bacteria or under sulfate-reducing conditions (Makkar and Cooney, 1990; Bridou et al., 2018). The methylation process can occur in the bottom anaerobic environment, and the methylation products will be released into the surface water by flow disturbance.
Phenyltins (PhTs), including TPhT, DPhT, and MPhT, should not be ignored. The present study only detected TPhT in the stormy months (Table 2). This result indicated that rainfall promoted the input of agricultural TPhT into the river. TPhT is associated with agricultural activities, such as pesticide and insecticide usage (Sheikh et al., 2007; Gao et al., 2019). Since farmland is distributed around this drinking water source, TPhT and its degradation products (DPhT and MPhT) entered the river with surface runoff. Moreover, there is an increasing demand for TPhT usage in agricultural fields (Cao et al., 2009), which will increase its input into rivers.
MPhT as a degradation product of TPhT and DPhT was only detected in September 2019, especially during the flooding tide (Figure 3). PhTs are also related to fisheries and aquaculture. There is developed aquaculture, and particularly mariculture downstream of the water source (Wang et al., 2014). A large area of farmland is also present downstream of the water source. In addition, rainfall in September 2019 was more abundant than that in the remaining months, except for the stormy months. Thus, more MPhT was detected at the flooding tide in September 2019.
Pollution level and risk assessment of OTCs
The different sources of OTCs lead to differences in OTC concentrations and compositions. The types and concentrations of OTCs in the upper reaches of the Yangtze River Estuary exceed those in Austria, in which each detected OTC was at a concentration of less than 10 ng L−1 (Zoboli et al., 2019). However, they are similar to those in the Bay of South Korea, except for TBT (Kim et al., 2014). In addition, during the stormy months, the concentrations of OTCs were lower than those in a port in Argentina, those in the secondary anabranches in the Three Gorges Reservoir Region, China, and in the turbidity maximum zone of the Yangtze River Estuary (Gao et al., 2019; Quintas et al., 2019; Chen et al., 2022).
MBT, DBT, and MPhT are the main OTCs in the Three Gorges Reservoir Region (Gao et al., 2019). TBT and TPhT dominate the OTCs in the turbidity maximum zone, whereas DMT and TBT were the dominant OTCs in the present study (Huang et al., 2021; Chen et al., 2022). Butyltins and phenyltins are generally the main OTC species in the Yangtze River and its estuary.
The risks of OTCs to the ecology and human health will differ among the different OTCs. The parent substances and degradation products of OTCs are toxic, with TBT showing the highest acute toxicity to marine invertebrates (Furdek et al., 2016; Zhang et al., 2021). The US Environmental Protection Agency (EPA) standard for TBT in freshwater is 63 ng L−1 (USEPA, 2002), whereas the European Environmental Quality Standards (EQS) formulated by the EU Water Framework Directive state a limit to the annual average concentration of TBT in any surface water being 0.2 ng L−1 and a maximum allowable concentration of TBT in unfiltered water being 1.5 ng L−1 (EU, 2008; Tunç et al., 2021). The results of the present study showed that the maximum concentration of TBT exceeded the relevant limits (Table 1), consistent with the situation off the Spanish coast in which the TBT concentration also exceeds the maximum allowable concentration (Concha-Graña et al., 2021).
This result, in combination with the identified main sources of TBT, TPhT, and DPhT, indicates that exogenous inputs (such as the release of antifouling paint and agricultural activities) pose a risk to aquatic organisms in the drinking water source. Studies have shown that TMT and its derivatives are highly toxic to insects and mammals (Hoch, 2001; Song et al., 2006). DMT and DBT are the degradation products of TMT and TBT, respectively. Thus, a potential ecological risk may exist, despite the reduced toxicity of degradation products (Zhang et al., 2021). TMT accounted for a large proportion of OTCs in September 2019 (Table 2 and Figure 3D), indicating that a specific focus on TMT is needed during some months. The detection frequencies and concentrations of trisubstituted and disubstituted organotin, and particularly for TBT, DMT, TMT, and DBT, were higher than those of the remaining OTCs (Table 1). Therefore, there should be increased attention to trisubstituted organotin (TBT and TMT) and its degradation products (DMT and DBT).
OTCs also pose a risk to human health due to their extensive use (Yi et al., 2012; Zhang et al., 2017). Humans may be exposed to OTCs through not only the food chain but also the consumption of drinking water (Tang et al., 2010; Jadhav et al., 2011; Gao et al., 2013). Although the risk posed to human health by OTCs in purified water is at least an order of magnitude lower than that in untreated water, the risks posed by OTCs in the drinking water source to human health were low in all sampling months, indicating that the drinking water is safe. However, the RQ value indicated moderate risk during the stormy months (July 2018; July 2019), which suggests there is a chance of reaching a moderate risk to human health during the stormy months and the need for particular focus on mitigation and treatment steps during this period.
Conclusion
The results of the present study indicated OTC contamination in the upper reaches of the Yangtze River Estuary. Rainfall runoff was identified as a driver of increased concentrations and diversity of OTCs, particularly in the stormy months. The concentrations of OTCs in the ebbing tide exceeded those in the flooding tide and increased during the tidal transition from the flooding tide to ebbing tide. This information can guide efforts to avoid peaks in OTC concentrations during the withdrawal of water from the drinking water source. OTCs mainly originate from marine antifouling coatings, agricultural activities, and plastic wastes. A higher focus on TBT, TPhT, and DPhT is needed due to their moderate to high ecological risk. Although the risk of OTCs in drinking water to human health is low at present, efforts to mitigate OTC contamination may be needed during the stormy months in the future (Yamabe et al., 2000; Zhou et al., 2001).
Data availability statement
The original contributions presented in the study are included in the article/Supplementary Material; further inquiries can be directed to the corresponding author.
Author contributions
QD: validation, formal analysis, data curation, and writing—original draft. LC: supervision and writing—review and editing. PL: project administration and investigation. SX: project administration, and funding acquisition. YW: investigation. QH: conceptualization, writing—original draft, writing—review and editing, and funding acquisition. All authors contributed to manuscript revision, read, and approved the submitted version.
Funding
The project was funded by the National Science and Technology “Water Pollution Control and Treatment” Major Project of China by the Ministry of Science and Technology of the People’s Republic of China (No. 2017ZX07201002) and the China Three Gorges Corporation (CTG 201903173).
Acknowledgments
The authors would like to thank all the reviewers who participated in the review, as well as MJEditor (www.mjeditor.com) for providing English editing services during the preparation of this article. The project was funded by the National Science and Technology “Water Pollution Control and Treatment” Major Project of China by the Ministry of Science and Technology of the People’s Republic of China (No. 2017ZX07201002).
Conflict of interest
The authors declare that the research was conducted in the absence of any commercial or financial relationships that could be construed as a potential conflict of interest.
Publisher’s note
All claims expressed in this article are solely those of the authors and do not necessarily represent those of their affiliated organizations, or those of the publisher, the editors, and the reviewers. Any product that may be evaluated in this article, or claim that may be made by its manufacturer, is not guaranteed or endorsed by the publisher.
Supplementary material
The Supplementary Material for this article can be found online at: https://www.frontiersin.org/articles/10.3389/fenvs.2022.964486/full#supplementary-material
References
Alzieu, C. (2000). Impact of tributyltin on marine invertebrates. Ecotoxicology 9 (1), 71–76. doi:10.1023/A:1008968229409
Anastasiou, T. I., Chatzinikolaou, E., Mandalakis, M., and Arvanitidis, C. (2016). Imposex and organotin compounds in ports of the Mediterranean and the Atlantic: Is the story over? Sci. Total Environ. 569-570, 1315–1329. doi:10.1016/j.scitotenv.2016.06.209
Antizar-Ladislao, B. (2008). Environmental levels, toxicity and human exposure to tributyltin (TBT)-contaminated marine environment. A review. Environ. Int. 34 (2), 292–308. doi:10.1016/j.envint.2007.09.005
Bhosle, N. B., Garg, A., Harji, R., Jadhav, S., Sawant, S. S., Krishnamurthy, V., et al. (2006). Butyltins in the sediments of Kochi and Mumbai harbours, west coast of India. Environ. Int. 32 (2), 252–258. doi:10.1016/j.envint.2005.08.020
Bridou, R., Rodriguez-Gonzalez, P., Stoichev, T., Amouroux, D., Monperrus, M., Navarro, P., et al. (2018). Methylation and dealkykation of tin compounds by sulfate- and nitrate-reducing bacteria. Chemosphere 208, 871–879. doi:10.1016/j.chemosphere.2018.06.030
Burton, E. D., Phillips, I. R., and Hawker, D. W. (2004). Sorption and desorption behavior of tributyltin with natural sediments. Environ. Sci. Technol. 38 (24), 6694–6700. doi:10.1021/es049291s
Cao, D. D., Jiang, G. B., Zhou, Q. F., and Yang, R. Q. (2009). Organotin pollution in China: An overview of the current state and potential health risk. J. Environ. Manage. 90, S16–S24. doi:10.1016/j.jenvman.2008.06.007
Chen, Z. Y., Chen, L., Chen, C. Z., Huang, Q. H., Wu, L. L., and Zhang, W. (2016). Organotin contamination in sediments and aquatic organisms from the Yangtze estuary and adjacent marine environments. Environ. Eng. Sci. 34 (4), 227–235. doi:10.1089/ees.2016.0370
Chen, C. Z., Chen, L., Li, F. P., Huang, Q. H., Wu, L. L., and Zhang, W. (2019a). Urgent caution to trace organometal pollution: Occurrence, distribution and sources of methyltins, butyltins and phenyltins in sediments from South Hangzhou Bay, China. Environ. Pollut. 246, 571–577. doi:10.1016/j.envpol.2018.12.037
Chen, Z. B., Zhang, H., and Liao, M. X. (2019b). Integration multi-model to evaluate the impact of surface water quality on city sustainability: A case from maanshan city in China. Processes 7 (1), 25. doi:10.3390/pr7010025
Chen, C. Z., Chen, L., Huang, Q. H., Zhang, W., and Leung, K. M. Y. (2022). Pulsed distribution of organotins in the turbidity maximum zone of the Yangtze Estuary throughout a tidal cycle. Mar. Pollut. Bull. 178, 113600. doi:10.1016/j.marpolbul.2022.113600
Cole, R. F., Mills, G. A., Parker, R., Bolam, T., Birchenough, A., Kroger, S., et al. (2015). Trends in the analysis and monitoring of organotins in the aquatic environment. Trends Environ. Anal. Chem. 8, 1–11. doi:10.1016/j.teac.2015.05.001
Cole, R. F., Mills, G. A., Hale, M. S., Parker, R., Bolam, T., Teasdale, P. R., et al. (2018). Development and evaluation of a new diffusive gradients in thin-films technique for measuring organotin compounds in coastal sediment pore water. Talanta 178, 670–678. doi:10.1016/j.talanta.2017.09.081
Concha-Graña, E., Moscoso-Pérez, C., Fernández-González, V., López-Mahía, P., Gago, J., León, V. M., et al. (2021). Phthalates, organotin compounds and per-polyfluoroalkyl substances in semiconfined areas of the Spanish coast: Occurrence, sources and risk assessment. Sci. Total Environ. 780, 146450. doi:10.1016/j.scitotenv.2021.146450
Cruz, A., Anselmo, A. M., Suzuki, S., and Mendo, S. (2015). Tributyltin (TBT): A review on microbial resistance and degradation. Crit. Rev. Environ. Sci. Technol. 45 (9), 970–1006. doi:10.1080/10643389.2014.924181
Dai, Q., Wang, Y. Y., Chen, L., Li, P., Xia, S. J., and Huang, Q. H. (2022). Contamination of 16 priority polycyclic aromatic hydrocarbons (PAHs) in urban source water at the tidal reach of the Yangtze River. Environ. Sci. Pollut. Res. 29, 61222–61235. in press. doi:10.1007/s11356-022-20052-0
de Castro, Í. B., Perina, F. C., and Fillmann, G. (2012). Organotin contamination in South American coastal areas. Environ. Monit. Assess. 184 (3), 1781–1799. doi:10.1007/s10661-011-2078-7
Díez, S., Lacorte, S., Viana, P., Barceló, D., and Bayona, J. M. (2005). Survey of organotin compounds in rivers and coastal environments in Portugal 1999–2000. Environ. Pollut. 136 (3), 525–536. doi:10.1016/j.envpol.2004.12.011
Dobson, S., Howe, P., and Floyd, P. (2006). “Mono- and disubstituted methyltin, butyltin, and octyltin compounds,” in IPCS concise international chemical assessment document 73. (Cambridgeshire: World Health Organization Press).
Fang, L. P., Xu, C. H., Li, J., Borggaard, O. K., and Wang, D. S. (2017). The importance of environmental factors and matrices in the adsorption, desorption, and toxicity of butyltins: A review. Environ. Sci. Pollut. Res. 24 (10), 9159–9173. doi:10.1007/s11356-017-8449-z
Fauser, P., Strand, J., and Vorkamp, K. (2020). Risk assessment of added chemicals in plastics in the Danish marine environment. Mar. Pollut. Bull. 157, 111298. doi:10.1016/j.marpolbul.2020.111298
Fenner, K., Kooijman, C., Scheringer, M., and Hungerbühler, K. (2002). Including transformation products into the risk assessment for chemicals: The case of nonylphenol ethoxylate usage in Switzerland. Environ. Sci. Technol. 36 (6), 1147–1154. doi:10.1021/es015576i
Fristachi, A., Xu, Y., Rice, G., Impellitteri, C. A., Carlson-Lynch, H., and Little, J. C. (2009). Using probabilistic modeling to evaluate human exposure to organotin in drinking water transported by polyvinyl chloride pipe. Risk Anal. 29 (11), 1615–1628. doi:10.1111/j.1539-6924.2009.01307.x
Furdek, M., Mikac, N., Bueno, M., Tessier, E., Cavalheiro, J., and Monperrus, M. (2016). Organotin persistence in contaminated marine sediments and porewaters: In situ degradation study using species-specific stable isotopic tracers. J. Hazard. Mat. 307, 263–273. doi:10.1016/j.jhazmat.2015.12.037
Gao, J. M., Zhang, Y., Guo, J. S., Jin, F., and Zhang, K. (2013). Occurrence of organotins in the Yangtze River and the jialing river in the urban section of chongqing, China. Environ. Monit. Assess. 185 (5), 3831–3837. doi:10.1007/s10661-012-2832-5
Gao, J. M., Chen, X. L., Sun, X. Q., Zhang, K., Chen, Y. P., Guo, J. S., et al. (2018). Occurrence of organotins in the aquatic environment during an operating cycle of the Three Gorges Reservoir, China. Environ. Sci. Pollut. Res. 25 (2), 1731–1741. doi:10.1007/s11356-017-0585-y
Gao, J. M., Chen, X. L., Ren, C. R., Qiu, H., Zhang, K., Guo, J. S., et al. (2019). Organotins in the aquatic media of secondary anabranches in the three Gorges Reservoir region, China. Chemosphere 217, 232–242. doi:10.1016/j.chemosphere.2018.10.204
Garnier, A., Bancon-Montigny, C., Delpoux, S., Spinelli, S., Avezac, M., and Gonzalez, C. (2020). Study of passive sampler calibration (Chemcatcher®) for environmental monitoring of organotin compounds: Matrix effect, concentration levels and laboratory vs in situ calibration. Talanta 219, 121316. doi:10.1016/j.talanta.2020.121316
Graceli, J. B., Sena, G. C., Lopes, P. F. I., Zamprogno, G. C., da Costa, M. B., Godoi, A. F. L., et al. (2013). Organotins: A review of their reproductive toxicity, biochemistry, and environmental fate. Reprod. Toxicol. 36, 40–52. doi:10.1016/j.reprotox.2012.11.008
Harino, H., Fukushima, M., Kurokawa, Y., and Kawai, S. i. (1997). Susceptibility of bacterial populations to organotin compounds and microbial degradation of organotin compounds in environmental water. Environ. Pollut. 98 (2), 157–162. doi:10.1016/S0269-7491(97)00133-4
Hiromori, Y., Nishikawa, J., Yoshida, I., Nagase, H., and Nakanishi, T. (2009). Structure-dependent activation of peroxisome proliferator-activated receptor (PPAR) γ by organotin compounds. Chem. Biol. Interact. 180 (2), 238–244. doi:10.1016/j.cbi.2009.03.006
Ho, K. K. Y., Zhou, G. J., Xu, E. G. B., Wang, X. H., and Leung, K. M. Y. (2016). Long-Term spatio-temporal trends of organotin contaminations in the marine environment of Hong Kong. PLoS One 11 (5), e0155632. doi:10.1371/journal.pone.0155632
Hoch, M. (2001). Organotin compounds in the environment — An overview. Appl. Geochem. 16 (7), 719–743. doi:10.1016/S0883-2927(00)00067-6
Huang, Q. H., Wen, X., Dai, Q., Chen, L., Wang, R., Yin, D. Q., et al. (2021). Occurrence of organotin in bottom water of the Yangtze River estuary and its behavior in the seasonally hypoxic zone. J. Tongji Univ. Nat. Science)--(in Chin. 49 (10), 1374–1382. doi:10.11908/j.issn.0253-374x.21244
Ighalo, J. O., Adeniyi, A. G., Adeniran, J. A., and Ogunniyi, S. (2021). A systematic literature analysis of the nature and regional distribution of water pollution sources in Nigeria. J. Clean. Prod. 283, 124566. doi:10.1016/j.jclepro.2020.124566
IMO (2001). The international convention on the control of harmful anti-fouling systems on ships. London. International Maritime Organization.
Jadhav, S., Bhosale, D., and Bhosle, N. (2011). Baseline of organotin pollution in fishes, clams, shrimps, squids and crabs collected from the west coast of India. Mar. Pollut. Bull. 62 (10), 2213–2219. doi:10.1016/j.marpolbul.2011.06.023
Kim, N. S., Hong, S. H., Yim, U. H., Shin, K. H., and Shim, W. J. (2014). Temporal changes in TBT pollution in water, sediment, and oyster from Jinhae Bay after the total ban in South Korea. Mar. Pollut. Bull. 86 (1), 547–554. doi:10.1016/j.marpolbul.2014.06.035
Krishna, R. S., Mishra, J., and Ighalo, O. J. (2020). Rising demand for rain water harvesting system in the world: A case study of joda town, India. World Sci. News 146, 47–59. Available at: https://www.researchgate.net/publication/341709163.
Kucklick, J. R., and Ellisor, M. D. (2019). A review of organotin contamination in arctic and subarctic regions. Emerg. Contam. 5, 150–156. doi:10.1016/j.emcon.2019.04.003
Mahmood, Z., Yousif, E., Alias, M., El-Hiti, G., and Ahmed, S. D. (2020). Synthesis, characterization, properties, and use of new fusidate organotin complexes as additives to inhibit poly(vinyl chloride) photodegradation. J. Polym. Res. 27, 267. doi:10.1007/s10965-020-02245-8
Makkar, N. S., and Cooney, J. J. (1990). Methylation of monomethyltin by a bacterial coculture. Geomicrobiol. J. 8 (2), 101–107. doi:10.1080/01490459009377883
Meador, J. P., Sommers, F. C., Cooper, K. A., and Yanagida, G. (2011). Tributyltin and the obesogen metabolic syndrome in a salmonid. Environ. Res. 111 (1), 50–56. doi:10.1016/j.envres.2010.11.012
Okoro, H. K., Fatoki, O. S., Adekola, F. A., Ximba, B. J., Snyman, R. G., and Opeolu, B. (2011). in Reviews of environmental contamination and Toxicology volume 213. Editor D. M. Whitacre (New York: Springer), 27–54.
Penninks, A. H. (1993). The evaluation of data‐derived safety factors for bis(tri‐n‐butyltin)oxide. Food Addit. Contam. 10 (3), 351–361. doi:10.1080/02652039309374157
Quintas, P. Y., Alvarez, M. B., Arias, A. H., Garrido, M., and Marcovecchio, J. E. (2019). Spatiotemporal distribution of organotin compounds in the coastal water of the Bahía Blanca estuary (Argentina). Environ. Sci. Pollut. Res. 26 (8), 7601–7613. doi:10.1007/s11356-019-04181-7
Sharma, V. K., Anquandah, G. A. K., Yngard, R. A., Kim, H., Fekete, J., Bouzek, K., et al. (2009). Nonylphenol, octylphenol, and bisphenol-A in the aquatic environment: A review on occurrence, fate, and treatment. J. Environ. Sci. Health, Part A 44 (5), 423–442. doi:10.1080/10934520902719704
Sheikh, M. A., Tsuha, K., Wang, X. C., Sawano, K., Imo, S. T., and Oomori, T. (2007). Spatial and seasonal behaviour of organotin compounds in protected subtropical estuarine ecosystems in Okinawa, Japan. Int. J. Environ. Anal. Chem. 87 (12), 847–861. doi:10.1080/03067310701415629
Song, X. Q., Zapata, A., and Eng, G. (2006). Organotins and quantitative-structure activity/property relationships. J. Organomet. Chem. 691 (8), 1756–1760. doi:10.1016/j.jorganchem.2005.12.003
Tang, C. H., Hsu, C. H., and Wang, W. H. (2010). Butyltin accumulation in marine bivalves under field conditions in Taiwan. Mar. Environ. Res. 70 (2), 125–132. doi:10.1016/j.marenvres.2010.03.011
Tsuda, T., Inoue, T., Kojima, M., and Aoki, S. (1995). Daily intakes of THbutyltin and THphenyltin compounds from meals. J. AOAC Int. 78, 941–943. doi:10.1093/jaoac/78.4.941
Tunç, M., Ay, Ü., Can, S. Z., Bingöl, D., and Ün, İ. (2021). Quantification of tributyltin in seawater using triple isotope dilution gas chromatography–inductively coupled plasma mass spectrometry achieving high accuracy and complying with European Water Framework Directive limits. J. Chromatogr. A 1637, 461847. doi:10.1016/j.chroma.2020.461847
USEPA (2021). Regional screening levels (RSLs) - user's guide. Washington: United States Environmental Protection Agency. Available at: https://www.epa.gov/risk/regional-screening-levels-rsls.
USEPA (2002). National recommended water quality criteria 2002. Washington: United States Environmental Protection Agency.
Wang, X. H., Fang, C., Hong, H. S., and Wang, W. X. (2010). Gender differences in TBT accumulation and transformation in Thais clavigera after aqueous and dietary exposure. Aquat. Toxicol. 99 (3), 413–422. doi:10.1016/j.aquatox.2010.06.001
Wang, L., Coles, N. A., Wu, C. F., and Wu, J. P. (2014). Spatial variability of heavy metals in the coastal soils under long-term reclamation. Estuar. Coast. Shelf Sci. 151, 310–317. doi:10.1016/j.ecss.2014.07.001
Whalen, M. M., Loganathan, B. G., and Kannan, K. (1999). Immunotoxicity of environmentally relevant concentrations of butyltins on human natural killer cells in vitro. Environ. Res. 81 (2), 108–116. doi:10.1006/enrs.1999.3968
Whalen, M. M., Hariharan, S., and Loganathan, B. G. (2000). Phenyltin inhibition of the cytotoxic function of human natural killer cells. Environ. Res. 84 (2), 162–169. doi:10.1006/enrs.2000.4083
Xiao, F., Simcik, M. F., and Gulliver, J. S. (2012). Perfluoroalkyl acids in urban stormwater runoff: Influence of land use. Water Res. 46 (20), 6601–6608. doi:10.1016/j.watres.2011.11.029
Yamabe, Y., Hoshino, A., Imura, N., Suzuki, T., and Himeno, S. (2000). Enhancement of androgen-dependent transcription and cell proliferation by tributyltin and triphenyltin in human prostate cancer cells. Toxicol. Appl. Pharmacol. 169 (2), 177–184. doi:10.1006/taap.2000.9067
Yi, A. X. L., Leung, K. M. Y., Lam, M. H. W., Lee, J. S., and Giesy, J. P. (2012). Review of measured concentrations of triphenyltin compounds in marine ecosystems and meta-analysis of their risks to humans and the environment. Chemosphere 89 (9), 1015–1025. doi:10.1016/j.chemosphere.2012.05.080
Zhang, C. N., Zhang, J. L., Huang, Y., Ren, H. T., Guan, S. H., and Zeng, Q. H. (2017). Dibutyltin depressed immune functions via NF-κB, and JAK/STAT signaling pathways in zebrafish (Danio rerio). Environ. Toxicol. 33, 104–111. doi:10.1002/tox.22502
Zhang, S. Q., Li, P., and Li, Z. H. (2021). Toxicity of organotin compounds and the ecological risk of organic tin with co-existing contaminants in aquatic organisms. Comp. Biochem. Physiology Part C Toxicol. Pharmacol. 246, 109054. doi:10.1016/j.cbpc.2021.109054
Zheng, H. Z., Chen, S. Z., Wu, T., Zhang, J. L., Su, Y., Chen, Y. X., et al. (2011). Tributyltin causes obesity and hepatic steatosis in male mice. Environ. Toxicol. 26, 79–85. doi:10.1002/tox.20531
Zhou, Q. F., Jiang, G. B., and Liu, J. Y. (2001). Small-scale survey on the contamination status of butyltin compounds in seafoods collected from seven Chinese cities. J. Agric. Food Chem. 49 (9), 4287–4291. doi:10.1021/jf010438y
Keywords: organotin compounds, surface water, temporal distribution, source analysis, risk assessment
Citation: Dai Q, Chen L, Li P, Xia S, Wang Y and Huang Q (2022) Occurrence and risk assessment of organotin compounds in the surface water of the upper Yangtze River Estuary. Front. Environ. Sci. 10:964486. doi: 10.3389/fenvs.2022.964486
Received: 08 June 2022; Accepted: 30 August 2022;
Published: 20 September 2022.
Edited by:
Pedro Neves Carvalho, Aarhus University, DenmarkReviewed by:
Segun Ayejuyo, University of Lagos, NigeriaThaise Machado Senez-Mello, Fluminense Federal University, Brazil
Copyright © 2022 Dai, Chen, Li, Xia, Wang and Huang. This is an open-access article distributed under the terms of the Creative Commons Attribution License (CC BY). The use, distribution or reproduction in other forums is permitted, provided the original author(s) and the copyright owner(s) are credited and that the original publication in this journal is cited, in accordance with accepted academic practice. No use, distribution or reproduction is permitted which does not comply with these terms.
*Correspondence: Qinghui Huang, cWhodWFuZ0B0b25namkuZWR1LmNu