- 1State Key Laboratory of Environmental Criteria and Risk Assessment, Chinese Research Academy of Environmental Sciences, Beijing, China
- 2College of Water Science, Beijing Normal University, Beijing, China
With the ban on brominated flame retardants (BFRs), organophosphate flame retardants (OPFRs) were used as substitutes generally as flame retardants, lubricants, plasticizers, and so on. Tris(2-chloroethyl) phosphate (TCEP) is a common OPFR and has been widely detected in different environmental compartments such as the atmosphere, dust, water, sediment, and soil. At elevated levels, TCEP can cause adverse health effects on humans and aquatic life. The present study aimed to use bibliometrics analysis of the important research about TCEP and assess the risk of TCEP to aquatic life through a comprehensive meta-data analysis of available secondary data on its toxic effects and exposure levels. The results showed that toxicity and environmental occurrence and concentration are research hot spots. Meanwhile, given the relatively high detection rates and high concentrations of TCEP in some freshwaters, the ecological risk of TCEP to freshwater organisms should not be ignored. This result of the present study could provide data support and references for the risk assessment and environmental management for such new emerging contaminants.
Introduction
The use of organophosphate esters (OPEs) as organophosphate flame retardants (OPFRs) has gradually increased after the ban of brominated flame retardants (BFRs) (Van Der Veen and De Boer, 2012; Wei et al., 2015; Mekni et al., 2020). Generally, OPEs are classified into three categories based on molecular structures, including alkyl OPEs, halogenated OPEs, and phenyl OPEs. As one of halogenated OPEs, tris(2-chloroethyl) phosphate (TCEP) (CAS:115-96-8) has been widely used in various commercial and industrial products such as electronic devices, paint, glue building materials, furniture, and baby toys (Bateman et al., 2017; Chokwe et al., 2020; Yan et al., 2021; Hu et al., 2022).
TCEP is generally manufactured by the reaction of phosphorus oxychloride with 2-chloroethanol or by the reaction of ethylene oxide with phosphorus oxychloride. Due to its physical and chemical properties, TCEP is mainly used as a flame retardant. In Europe, the use of organophosphorus flame retardants like TCEP has an upward trend with the ban of poly brominated diphenyl ethers (PBDEs). The amount of TCEP accounted for about 50% of the total amount of hard and soft polyurethane vinegar and about 30% of polyvinyl chloride (Reemtsma et al., 2008). In China, the consumption of halogenated organophosphate flame retardants like TCEP accounts for about 80% of total flame retardants. In addition, it can also be used as an additive for plasticizers, lubricating oil or gasoline, and as an extractant for rare metals (uranium, polonium, tantalum, etc.) (Van Der Veen and De Boer, 2012). The annual production and use of TCEP in China are tens of thousands of tons (Gao Xiaozhong and Wang, 2015).
TCEP is physically combined with materials surfaces as additives rather than combined by chemical reactions, which causes TCEP to be easily released into the environment. Compared with other OPEs, TCEP has higher water solubility (25°C, >7000 μg/L) and is difficult to biodegrade in the environment (Zhao et al., 2016). Research data showed that TCEP can be detected in surface water, sediment, atmosphere, soil, and other environmental media in many regions of China (Wang et al., 2014; Wang et al., 2014; Xing et al., 2018). In addition, several studies showed that TCEP has neurotoxicity, reproductive toxicity, immunotoxicity, and endocrine-disrupting effects (Noyes et al., 2015; Li et al., 2019; Sutha et al., 2022; Yan et al., 2022). Exposure to environmental concentrations of TCEP results in impaired structural development and reduced metabolic capacity in the gills of pelteobagrus fulvidraco (Zhao et al., 2021). Long-term exposure to TCEP leads to structural changes in zebrafish gonadal tissues and significantly alters the content of related enzymes (Sutha et al., 2022). TCEP has been added to the list of priority substances for the second batch by the China Ministry of Ecology and Environment; TCEP is highly harmful to the ecological system of freshwater and damages the healthy reproduction and nervous system of humans (Mee, 2022). Internationally, the EU implements authorization management for TCEP and United States restricts the use of TCEP in children’s products (U.S. Environmental Protection Agency, 2015; ECHA, 2018).
Due to the toxicity (carcinogenicity, nerve toxicity, endocrine disruption) and potential ecological risks, TCEP has been defined as a contaminant of emerging concern (CEC) (Chen et al., 2020). However, at present, most countries or regions still lack environmental standards and environmental management measures for TCEP. Research on ecological risk and environmental quality criteria is still required as there is no data support and scientific basis for environmental management of harmful CECs. However, ecological risk assessment of CECs based on traditional toxicity endpoints that predicted no effect concentration (PNEC) may not provide adequate protection for freshwater organisms (Jin et al., 2014). Because the concentration of CECs is lower than PNEC in the environment, it may cause neurotoxicity and reproduction toxicity rather than the obvious effect of death and development toxicity to freshwater organisms (Du et al., 2015; Liu et al., 2016). Therefore, it is necessary to calculate the ecological risk of TCEP for freshwater organisms by different toxicity endpoints.
In the present study, the scientific bibliometric analysis of TCEP-related articles was conducted using CiteSpace software to summarize the research hotspots, important research areas, and the toxic effects and toxicity endpoints of TCEP. The sensitive chronic toxicological endpoints were focused on. At the same time, based on the death, development, neural, and reproduction toxicity endpoints, the ecological risk to aquatic organisms was assessed according to the exposure to TCEP in the freshwater environment.
Materials and methods
Data collection and analysis methods for bibliometric of TCEP
The Web of Science (WOS) Core Collection SCI-expand database (https://www.webofscience.com/wos/woscc/basic-search, last accessed on 12 May 2022) was used to search for the literature related to TCEP. The WOS core data set is considered one of the most scientific and comprehensive literature databases, and it contains the most influential and relevant journals (Olawumi and Chan, 2018; Niu et al., 2021). The conditions for the text search were set as follows: 1) the search topic (TS) = [“Tris(2-chloroethyl) phosphate *” or “TCEP*”], 2) the type of the document was set to “article”, 3) the language of the article was set to English, and 4) the search data was set all years (from 1900 to 2022).
CiteSpace is a scientific bibliometric piece of software that provides citation analysis to obtain trends and research hotspots on research topics (Chen et al., 2012). The retrieved relevant literature was analyzed by CiteSpace for keyword co-occurrence, clustering, burst detection, and timeline analysis. Research content and hotspots in TCEP-related fields were systematically concluded by the Keyword co-occurrence and clustering analysis. Research progress and trends were concluded by keyword emergence and timeline analysis. The nodes in the clustering graph represent keywords, and the larger the node means that the node appears more frequently. The line between the nodes indicates that the nodes appear in the same or more articles and the thicker the line, the nodes are more relevant to each other. In addition, the color represents the time at which the node was located, and the labels in the clustering graph were named after the keywords that contribute the most to that cluster.
Toxicity and exposure data collection about TCEP
Toxicity and exposure data about TCEP to freshwater organisms were obtained through collection and screening from the USEPA ecotoxicology database (https://cfpub.epa.gov/ecotox/search.cfm), Web of Science (https://www.webofscience.com/), ELSEVIER (https://www.sciencedirect.com/), and CNKI (https://cnki.net) and published in the literature and government documents. Toxicity data were collected following these conditions: 1) the tested media should be freshwater; 2) the acute toxicity data should be including LC50 and EC50; 3) the chronic toxicity data of aquatic animals (including NOEC, LOEC, LC50, EC50, or MATC, etc.) should be available; and 4) the toxicity effects should be including reproduction, development, or neurotoxicity (Wang et al., 2020; Liu et al., 2022). The data generally included acute and chronic toxicity of TCEP to aquatic organisms like fish, water fleas, and so on. For acute toxicity data, selected measurement endpoints were the median lethal concentration (LC50) or median effect concentration (EC50) based on immobility for animals and biomass or growth for plants. For chronic toxicity data, no observed effects concentration (NOEC), maximum accept concentration (MATC), lowest observed effect concentration (LOEC), or x% effect concentration (ECx) was used.
Toxicity data for different effects of TCEP on aquatic organisms were divided into different categories by different endpoints. For acute toxicity, the effects of lethality, livability, and behavioral inhibition on aquatic organisms were mainly considered. Chronic toxicity data were divided into toxicity effects of development and growth inhibition to aquatic organisms, reproduction toxicity, neurotoxicity toxicity, and so on. Besides, the data for calculations needed to be filtered according to the following data priority selection: 1) the data obtained by the flow exposure test should be superior to the semi-static exposure test; 2) the data of toxicity of the full life cycle are superior to the part life cycle; 3) the most sensitive toxicity data should be chosen when there are more toxicity endpoints; and 4) for the data of the same species, chronic is superior to acute and NOEC is preferred, the second is LOEC, and then LC50 is superior to EC50.
Environmental concentrations of TCEP were collected from the published literature (Table 3). The exposure concentrations in freshwater were collected in China in recent 12 years (2011–2022) as well as in other countries to assess the ecological risks of TCEP to freshwater organisms.
Ecological risk assessment method
There were two approaches used to the ecological risk assessment of TCEP for freshwater organisms in this study. The first one is risk quotients (RQs), which are calculated as quotients of the environmental concentration of TCEP in surface waters divided by the predicted no effect concentration (PNEC) (Equation 1) (Liu et al., 2020). The preliminary risk assessment ranks of TCEP were classified as follows: if RQ < 0.1, the risk is insignificant; if 0.1 ≤ RQ < 1, the risk is low; if 1 ≤ RQ < 10, the risk is moderate; and if RQ ≥ 10, the risk is high (Bu et al., 2013; Yan et al., 2015).
CE is the environmental exposure concentration of TCEP in this equation. PNEC is derived from the most sensitive toxicity data with assessment factors (Afs) of 10, 20, or 100, which are calculated by test endpoints of NOEC, LOEC, EC50, or LC50 (Tarazona et al., 2010; Bu et al., 2013).
The second method of ecological risk assessment of TCEP to freshwater organisms is joint probability curves (JPCs), which is one of the most widely used and refined methods of probabilistic ecological risk assessment (Solomon et al., 2000). JPCs describe the probability that the exceeded frequency of exposure to environmental concentrations (y-axis) and the number of freshwater organisms would be affected (x-axis) (Zheng et al., 2017). On the curve of JPCs, each point has its meaning, which represents the probability that the proportion of freshwater organisms will be affected and the frequency with which that magnitude of the effect would be exceeded (Jin et al., 2014). The closer the curve is to the coordinate axis, the less the toxic ecological risk of pollutants to organisms in the freshwater ecosystem (Solomon et al., 2000). Besides, risks were categorized as de minimis, low, intermediate, or high by the criteria that risk values = exceedance probability × magnitude of the effect (Moore et al., 2010; Moore et al., 2014; Whitfield Aslund et al., 2017). There are four risk categories introduced as follows: 1) The risk was categorized as de minimis when the maximum risk value was <0.25%. 2) The risk was categorized as low when the maximum risk value was ≥0.25% and <2%. 3) The risk was categorized as intermediate when the maximum risk value was ≥2% and <10%. 4) The risk was categorized as high when the maximum risk value was >10% (Liu et al., 2020).
Besides, the different endpoint toxicity data (X) of TCEP for different effect endpoints were normalized (X = NOEC, LOEC/2, EC50/10 or LC50/100) in the risk assessment method of JPCs (Bu et al., 2013; Liu et al., 2020). In JPCs, concentration and toxicity data of TCEP were calculated by (Lg10) and verified by the normal distribution.
Statistical analysis
All the data were processed and tested for normality by SPSS 26.0, and the data were subjected to either the Kolmogorov–Smirnov test (K-S test) or the Shapiro-Wilk test (S-W test) to verify whether the data conform to the normal distribution. JPCs were plotted and adjusted by MATLAB R2017a.
Results and discussion
Bibliometric results and analysis
The total number of articles retrieved was 1,071 after CiteSpace removed the duplicate articles. The data from these articles were analyzed by the keyword co-occurrence, and the pivotal research themes of TCEP are presented by the visual clustering analysis (Figure 1). TCEP is known to be an OPFR that is often added to plastics as a plasticizer and flame retardant. Therefore, the highest frequency of “flame retardant” and “plasticizer” is to be expected. It is worth noting that the frequency of the keyword “toxicity” is also high and research about toxicity has formed important research areas (#10 neurotoxicity and #8 gene transcriptional analysis in Figure 1), indicating that TCEP is toxic and toxicity is a hot topic in TCEP research. There are several studies focused on representing possible human exposure to environments containing TCEP, such as “indoor dust”, “human exposure”, “air”, “water”, “house dust”, and “surface water”. This indicates that the effects of TCEP on humans are of great concern and TCEP has been detected in a variety of environmental media. Although the “risk assessment” appeared slightly later than the other keywords by a few years, this indicates that the ecological hazards of TCEP have been recognized, such as #14 surface water, #16 purification, and #18 reduction, which are related to risk assessment research, and a limited approach is being taken to assess and control the environmental behavior of TCEP.
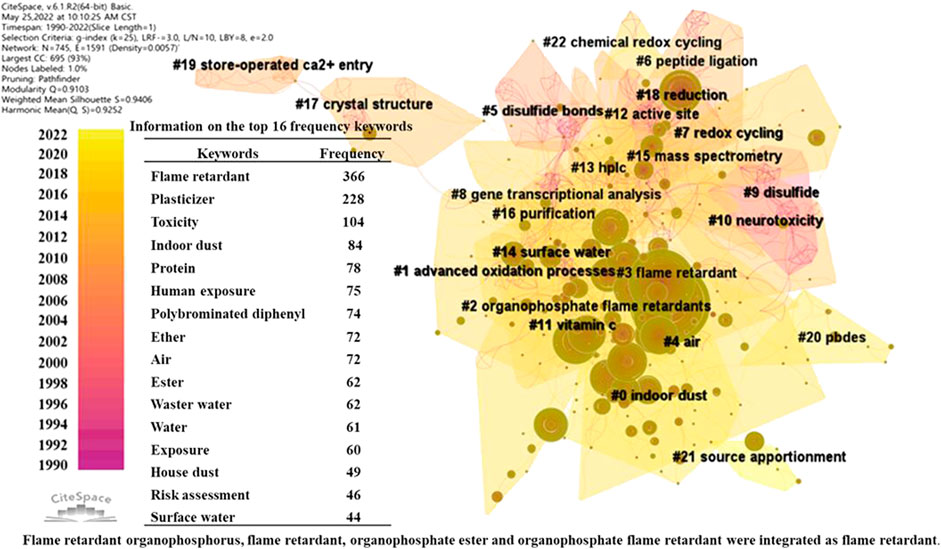
FIGURE 1. Visual cluster analysis of the keyword co-occurrence and the information on the top 16 frequency keywords.
Toxicity of TCEP to freshwater organisms
Acute toxicity
TCEP may cause the death of freshwater organisms when they are exposed to a high concentration of TCEP in a short time. The LC50 of several freshwater organisms caused by TCEP is listed in Table 1. Carassius auratus is the most sensitive species that has the lowest LC50, and Caenorhabditis elegans is the most tolerant species when it is exposed to TCEP. Several studies showed that although freshwater organisms were exposed to TCEP for a short time, their development, especially when they were in the early stages of life, would be affected. The nervous system of zebrafish embryos/larvae was significantly damaged when they were exposed to TCEP at 2,500 μg/L for 120 h (Li et al., 2019). Although the acute toxicity of TCEP is lower than that of other OPEs, it may induce the abnormal behavior of zebrafish larvae when they were exposed to TCEP after 2.5 h (Jarema et al., 2015). Besides, TCEP could be accumulated in tissues of the brain, intestine, liver, and muscle and could reach a steady state in 3 days, especially in the liver that has a higher concentration of TCEP compared to other tissues (Wang et al., 2017). In addition to fish, TCEP also causes acute toxicity to other organisms such as invertebrates. The theoretical population and cell viability were decreased when Tetrahymena thermophila were exposed to TCEP at 0.044 mg/L after 5 days, and the mechanism is that the expressions of genes of soluble N-ethylmaleimide sensitive factor attachment protein receptor (SNARE) were down-regulated (Hao et al., 2020). Thus, studies showed that TCEP could cause acute toxicity to freshwater organisms when they were exposed to TCEP at a high concentration and a short time, which could cause death or developmental defects to them.
Development and growth toxicity
TCEP may cause development and growth toxicity to freshwater organisms at a lower concentration than LC50, which may be hard to observe than the death of organisms. The development and growth toxicity of TCEP to several freshwater organisms are listed in Table 2. Developmental toxicity has an obvious difference when different freshwater organisms were exposed to TCEP. On one hand, TCEP could promote the development of Daphnia Magna at environmentally relevant or greater concentrations for a full life stage, in which the expression of genes associated with growth was significantly up-regulated and heart disease was reduced at the death stage.
Researchers have observed the developmental delay of Danio rerio embryos when the embryos were exposed to the highest concentrations of 14,250 and 28,500 μg/L of TCEP. Moreover, TCEP can also lead to spinal retardation and abnormal development (vertebral deformity, edema, immature spine, failure of gastrulation) (Wu et al., 2017). Other researchers found that shortened secondary gill lamellae and modest epithelial hyperplasia occurred in the 1 μg/L TCEP group, and telangiectasis, shortened secondary gill lamellae, severe epithelial hyperplasia, and epithelial lifting were observed in the gills of juvenile yellow catfish under 10 μg/L TCEP stress. Exposures to TCEP of 1,250 or 6,250 μg/L for 14 days caused shorter lengths of Japanese ricefish larvae (Sun et al., 2016a). The secondary gill lamellae were deformed in the 100 μg/L TCEP group. Genes related to antioxidant properties, osmoregulation, immunity, and apoptosis were differently expressed (Zhao et al., 2021).
In addition, it was also observed that the transcription of genes related to oxidative stress and lipid peroxidation was disrupted. The genes related to oxidative stress play integral roles in providing cellular protection during severe fatty acid β-oxidation. The disruption may lead to oxidative damage. The disruption of genes related to lipid peroxidation activation produces a rise in the expression of genes that promote fatty acid storage and finally led to obesity (Arukwe et al., 2016a).
Neurotoxicity
Neurotoxicity was mostly expressed in the abnormal behavior of freshwater organisms with the gene transcription occurrence abnormality related to their nervous system (Sun et al., 2016a; Sun et al., 2016b). Several studies showed that freshwater organisms observed neurotoxicity when they were exposed to TCEP. Some key genes and proteins of neurodevelopment, including mbp, a1-tubulin, and syn2a, were down-regulated when zebrafish embryos/larvae were exposed to TCEP at the concentration of 500 μg/L, which decreased their swimming ability (Li et al., 2019). It was found that in addition to the disruption of gene transcription, AChE activity was also influenced by TCEP, which affects the organization of the brain (Dishaw et al., 2014; Jarema et al., 2015; Sun et al., 2016a). In addition to fish, TCEP causes damage to dopaminergic neurons in Caenorhabditis Elegans at 750 mg/L and finally influences the behavior of Caenorhabditis elegans (Xu et al., 2017). Although freshwater organisms were exposed to a concentration lower than the lethal concentration, their nervous system was damaged and may induce abnormal behaviors.
Reproduction toxicity
TCEP also has reproduction toxicity. For example, TCEP had significant effects on D. Magna reproduction. According to the observation of researchers, four pathways related to genetic information processing were significantly up-regulated (Hao et al., 2020) and can also lead to the delay of eggs of Caenorhabditis elegans, and TCEP of 250 mg/L leads to about 0.5 days delay compared with the control group. Maximum spawning also decreased with the increase of exposure concentrations of TCEP (Xu et al., 2017). Zebrafish after 14 days of exposure to TCEP increased both 17β-estradiol (E2) and testosterone (T) concentrations in H295R cells (Liu et al., 2012). The in vivo metabolism of salmon was affected after 7 days of exposure to 0.04 mg/L TCEP and significantly affected the expression of neuro- and inter-renal steroidogenesis in juvenile salmon (Arukwe et al., 2016a; Arukwe et al., 2016b; Arukwe et al., 2018).
Exposure levels and risk assessment
More average concentrations of TCEP in the surface water of multiple countries are shown in Table 3. In China, the concentration of TCEP was from 4.05 ng/L to 552.63 ng/L. The maximum concentration was 552.63 ng/L, which was detected in Nanjing surface water, and the minimum was 4.05 ng/L, detected in the Yangtze River (Zha et al., 2018; Wang et al., 2022). The most urban surface water concentration of TCEP was higher than in rivers (Shi et al., 2016; Zhang S. et al., 2019; Niu et al., 2019). The concentration of TCEP in Taihu Lake in the area close to the city was higher than that in the central lake; the reason is that maybe the central lake was further away from the pollution sources of TCEP (Wang et al., 2018). Besides, the concentration of TCEP was different in other countries. The concentrations of TCEP in Nalón (3.6 ng/L), Arga (2.6 ng/L), and five great lakes (18 ng/L) were lower than those in Sydney (265.3 ng/L) and Shihwa Lake (226.6 ng/L). The difference in concentration distribution of TCEP was maybe due to the difference in production and demand, treatment and discharge, and the time cycle of use.
In order to show the risk of each sampling site, the risk assessment was conducted by RQ for them, and the results are listed in Table 3. The results showed that the risk of death of freshwater organisms in the environmental concentration of TCEP was insignificant and that the RQ1 value was lower than 0.1 in each sampling site. The risk of development was between insignificant and moderate with the RQ2 from 0.00468 to 1.17581. According to the results, in addition to Taihu (Yixing), surface water in Nanjing, Yunzao river in Shanghai, and Sydney had low neurotoxicity risk, and other sampling sites were insignificant. It is worth noting that reproductive toxicity had four types of risks in these freshwater sampling sites. Especially, the risk was high in surface water in Nanjing and the reproductive risk had the most moderate risk in all toxicity effects. This showed that the reproductive effect was maybe the most sensitive endpoint of toxicity effects.
The results of JPCs shown in Figure 2 were calculated by different toxicity effects. The x-axis of the JPC represents the intensity of effects, and the y-axis represents their probability. The risk of survival posed by TCEP to freshwater organisms was de minimis, which is the reason why the curve of JPC was on the coordinate axis. The total risk value of the development risk was 11.96%, which was a high potential risk for TCEP to the development and growth of freshwater organisms. These percent exceedance potential risks of freshwater were 72.86, 15.30, and 1.021% when the affected proportion percent of species was 10, 15, and 20%, respectively. For the neurotoxicity risk, its total risk value was 9.20%, which was an intermediate risk. When the proportion percent of affected species was 10 and 15%, there were rivers of 33.58 and 1.624% that may cause neurotoxicity to freshwater organisms. The highest risk in all toxicity effects was reproduction, and its total risk value was 19.26%. When the proportion percent of affected species was 15, 20, and 25%, there were rivers of 92.26, 38.97, and 4.01%, respectively, that may cause reproductive system damage to freshwater organisms.
According to the results, the survival risk of TCEP to freshwater organisms was insignificant. The risk of neurotoxicity was lower but not negligible. However, TCEP had significant developmental toxicity and reproduction toxicity ecological risks. Daphnia magna is the most sensitive species to TCEP development and reproduction toxicity; upon exposure to 940 ng/L for 62 days, their body length and genes of reproduction were significantly affected (Li W. et al., 2020). These lower toxicity data may cause overall toxicity reduction and improve the risk value.
Conclusions
With the occurrence and concentration of TCEP in the environment detected, its frequent detectability in the environment and the risks of these substances to freshwater organisms are gradually appearing. According to the results of bibliometric, the research about TCEP was hot and “Toxicity” and “Risk assessment” were important research areas. There were many toxicity effects of TCEP on freshwater organisms through the collection of research that were classified as survival, neuro system, development, and reproduction toxicity effects. TCEP has obvious toxic effects on freshwater organisms, which not only affect the neuro system of freshwater organisms in freshwater but also have obvious development and reproduction toxicity effects on freshwater organisms, especially reproduction toxicity, which was the most sensitive toxicity endpoint. The different toxicity effect endpoints of the environmental assessment risk of TCEP were calculated by two methods, which were RQs and JPCs. From the results of this study, the survival risk was insignificant when freshwater organisms were exposed to the environmental concentration of TCEP. However, TCEP posed a significantly higher risk to reproduction than other toxicity endpoints, such as development and neuro system. This mechanism may be that the synthetic pathway of many genes and proteins associated with the neuro and reproductive systems was disturbed and damaged their tissue, which caused developmental disorders and abnormal behavior and reduced reproductive capacity of freshwater organisms. It should be taken seriously that although many freshwater organisms could not die when they were exposed to the environmental concentration of TCEP, the development, nervous system, and reproduction toxicities significantly exist. The number of different species was limited in the collected research. Therefore, further research needs to add the diversity of species to improve the representation of risk assessment. This study can help to protect freshwater organisms and control the environmental risk of TCEP and provide data support and references for the risk assessment and environmental management for such new emerging contaminants.
Data availability statement
Some or all data and models that support the findings of this study are available from the corresponding author upon reasonable request.
Author contributions
This work is the result of collaboration among YQ, DL, CF, NL, JW, ZY, and YB. All authors have tributed, reviewed, and improved the manuscript. All authors have revised the final manuscript. All authors have read and agreed to the published version of the manuscript.
Funding
This research was funded by the Beijing Natural Science Foundation (Grant No. 8222077) and the National Key Research and Development Program of China (Grant No. 2021YFC3200104).
Conflict of interest
The authors declare that the research was conducted in the absence of any commercial or financial relationships that could be construed as a potential conflict of interest.
Publisher’s note
All claims expressed in this article are solely those of the authors and do not necessarily represent those of their affiliated organizations or those of the publisher, the editors, and the reviewers. Any product that may be evaluated in this article or claim that may be made by its manufacturer is not guaranteed or endorsed by the publisher.
References
Arukwe, A., Carteny, C. C., and Eggen, T. (2016a). Lipid peroxidation and oxidative stress responses in juvenile salmon exposed to waterborne levels of the organophosphate compounds tris(2-butoxyethyl)- and tris(2-chloroethyl) phosphates. J. Toxicol. Environ. Health A 79 (13-15), 515–525. doi:10.1080/15287394.2016.1171978
Arukwe, A., Carteny, C. C., Eggen, T., and Möder, M. (2018). Novel aspects of uptake patterns, metabolite formation and toxicological responses in salmon exposed to the organophosphate esters—tris(2-butoxyethyl)- and tris(2-chloroethyl) phosphate. Aquat. Toxicol. 196, 146–153. doi:10.1016/j.aquatox.2018.01.014
Arukwe, A., Carteny, C. C., Möder, M., Bonini, A., Maubach, M. A., and Eggen, T. (2016b). Differential modulation of neuro- and interrenal steroidogenesis of juvenile salmon by the organophosphates - tris(2-butoxyethyl)- and tris(2-cloroethyl) phosphate. Environ. Res. 148, 63–71. doi:10.1016/j.envres.2016.03.020
Awonaike, B., Lei, Y. D., and Wania, F. J. E. S. W. R. (2021). Precipitation-induced transport and phase partitioning of organophosphate esters (OPEs) in urban and rural watersheds. Environ. Sci. Water Res. Technol. 7 (11), 2106–2115. doi:10.1039/D1EW00329A
Bateman, M. E., Strong, A. L., Mclachlan, J. A., Burow, M. E., and Bunnell, B. A. (2017). The effects of endocrine disruptors on adipogenesis and osteogenesis in mesenchymal stem cells: A review. Front. Endocrinol. 7, 171. doi:10.3389/fendo.2016.00171
Bu, Q., Wang, B., Huang, J., Deng, S., and Yu, G. (2013). Pharmaceuticals and personal care products in the aquatic environment in China: A review. J. Hazard. Mat. 262, 189–211. doi:10.1016/j.jhazmat.2013.08.040
Chen, C., Hu, Z., Liu, S., and Tseng, H. J. E. O. O. B. T. (2012). Emerging trends in regenerative medicine: A scientometric analysis in CiteSpace. Expert Opin. Biol. Ther. 12 (5), 593–608. doi:10.1517/14712598.2012.674507
Chen, P., Zhong, Y., Chen, K., Guo, C., Gong, J., Wang, D., et al. (2020). The impact of discharge reduction activities on the occurrence of contaminants of emerging concern in surface water from the Pearl River. Environ. Sci. Pollut. Res. 27 (24), 30378–30389. doi:10.1007/s11356-020-09295-x
Chokwe, T. B., Abafe, O. A., Mbelu, S. P., Okonkwo, J. O., and Sibali, L. L. (2020). A review of sources, fate, levels, toxicity, exposure and transformations of organophosphorus flame-retardants and plasticizers in the environment. Emerg. Contam. 6, 345–366. doi:10.1016/j.emcon.2020.08.004
Chokwe, T., and Mporetji, S. J. W. S. (2019). Organophosphorus flame retardants in surface and effluent water samples from the vaal river catchment, south Africa: Levels and risk to aquatic life. Water sa. 45 (3), 469–476. doi:10.17159/wsa/2019.v45.i3.6744
Choo, G., Cho, H-S., Park, K., Lee, J-W., Kim, P., and Oh, J-E. (2018). Tissue-specific distribution and bioaccumulation potential of organophosphate flame retardants in crucian carp. Environ. Pollut. 239, 161–168. doi:10.1016/j.envpol.2018.03.104
Cristale, J., García Vázquez, A., Barata, C., and Lacorte, S. (2013a). Priority and emerging flame retardants in rivers: Occurrence in water and sediment, Daphnia magna toxicity and risk assessment. Environ. Int. 59, 232–243. doi:10.1016/j.envint.2013.06.011
Cristale, J., Katsoyiannis, A., Sweetman, A. J., Jones, K. C., and Lacorte, S. (2013b). Occurrence and risk assessment of organophosphorus and brominated flame retardants in the River Aire (UK). Environ. Pollut. 179, 194–200. doi:10.1016/j.envpol.2013.04.001
Dishaw, L. V., Hunter, D. L., Padnos, B., Padilla, S., and Stapleton, H. M. (2014). Developmental exposure to organophosphate flame retardants elicits overt toxicity and alters behavior in early life stage zebrafish (Danio rerio). Toxicol. Sci. 142 (2), 445–454. doi:10.1093/toxsci/kfu194
Du, Z., Wang, G., Gao, S., and Wang, Z. (2015). Aryl organophosphate flame retardants induced cardiotoxicity during zebrafish embryogenesis: By disturbing expression of the transcriptional regulators. Aquat. Toxicol. 161, 25–32. doi:10.1016/j.aquatox.2015.01.027
ECHA (2018). Screening report: An assessment of whether the use of TCEP, TCPP and TDCP in articles should be restricted. Helsink: European Chemicals Agency. AvaliableAt: https://echa.europa.eu/completed-activities-on-restriction.
Gao Xiaozhong, X. Y., and Wang, Z. (2015). Progress in environment exposure, transport and transform of organophosphorus flame retardants. Asian J. Ecotoxicol. 10 (2), 56–58. doi:10.7524/AJE.1673-5897.20150103001
Hao, H., Yuan, S., Cheng, S., Sun, Q., Giesy, J. P., and Liu, C. (2020). Effects of tris (2-chloroethyl) phosphate (TCEP) on growth, reproduction and gene transcription in the protozoan Tetrahymena thermophila. Aquat. Toxicol. 222, 105477. doi:10.1016/j.aquatox.2020.105477
He, M-J., Lu, J-F., and Wei, S-Q. (2019). Organophosphate esters in biota, water, and air from an agricultural area of Chongqing, Western China: Concentrations, composition profiles, partition and human exposure. Environ. Pollut. 244, 388–397. doi:10.1016/j.envpol.2018.10.085
Hu, F., Zhao, Y., Dong, F., Wang, H., Zheng, M., Zhang, W., et al. (2022). Insights into the mechanisms of tris(2-chloroethyl) phosphate-induced growth inhibition in juvenile yellow catfish Pelteobagrus fulvidraco. Aquat. Toxicol. 247, 106170. doi:10.1016/j.aquatox.2022.106170
Jarema, K. A., Hunter, D. L., Shaffer, R. M., Behl, M., and Padilla, S. (2015). Acute and developmental behavioral effects of flame retardants and related chemicals in zebrafish. Neurotoxicol. Teratol. 52, 194–209. doi:10.1016/j.ntt.2015.08.010
Jin, X., Wang, Y., Jin, W., Rao, K., Giesy, J. P., Hollert, H., et al. (2014). Ecological risk of nonylphenol in China surface waters based on reproductive fitness. Environ. Sci. Technol. 48 (2), 1256–1262. doi:10.1021/es403781z
Kovacevic, V., Simpson, A. J., and Simpson, M. J. (2018). Investigation of Daphnia magna sub-lethal exposure to organophosphate esters in the presence of dissolved organic matter using 1H NMR-based metabolomics. Metabolites 8 (2), 34. doi:10.3390/metabo8020034
Lee, S., Jeong, W., Kannan, K., and Moon, H-B. (2016). Occurrence and exposure assessment of organophosphate flame retardants (OPFRs) through the consumption of drinking water in Korea. Water Res. 103, 182–188. doi:10.1016/j.watres.2016.07.034
Li, R., Wang, H., Mi, C., Feng, C., Zhang, L., Yang, L., et al. (2019). The adverse effect of TCIPP and TCEP on neurodevelopment of zebrafish embryos/larvae. Chemosphere 220, 811–817. doi:10.1016/j.chemosphere.2018.12.198
Li, W., Yuan, S., Sun, Q., and Liu, C. (2020a). Toxicity of tris(2-chloroethyl) phosphate in Daphnia magna after lifetime exposure: Changes in growth, reproduction, survival and gene transcription. Ecotoxicol. Environ. Saf. 200, 110769. doi:10.1016/j.ecoenv.2020.110769
Li, Y., Yao, C., Zheng, Q., Yang, W., Niu, X., Zhang, Y., et al. (2020b). Occurrence and ecological implications of organophosphate triesters and diester degradation products in wastewater, river water, and tap water. Environ. Pollut. 259, 113810. doi:10.1016/j.envpol.2019.113810
Liu, N., Jin, X., Feng, C., Wang, Z., Wu, F., Johnson, A. C., et al. (2020). Ecological risk assessment of fifty pharmaceuticals and personal care products (PPCPs) in Chinese surface waters: A proposed multiple-level system. Environ. Int. 136, 105454. doi:10.1016/j.envint.2019.105454
Liu, N., Wang, Y., Yang, Q., Lv, Y., Jin, X., Giesy, J. P., et al. (2016). Probabilistic assessment of risks of diethylhexyl phthalate (DEHP) in surface waters of China on reproduction of fish. Environ. Pollut. 213, 482–488. doi:10.1016/j.envpol.2016.03.005
Liu, X., Ji, K., and Choi, K. (2012). Endocrine disruption potentials of organophosphate flame retardants and related mechanisms in H295R and MVLN cell lines and in zebrafish. Aquat. Toxicol. 114-115, 173–181. doi:10.1016/j.aquatox.2012.02.019
Liu, X., Tu, M., Wang, S., Wang, Y., Wang, J., Hou, Y., et al. (2022). Research on freshwater water quality criteria, sediment quality criteria and ecological risk assessment of triclosan in China. Sci. Total Environ. 816, 151616. doi:10.1016/j.scitotenv.2021.151616
Liu, Y., Song, N., Guo, R., Xu, H., Zhang, Q., Han, Z., et al. (2018). Occurrence and partitioning behavior of organophosphate esters in surface water and sediment of a shallow Chinese freshwater lake (Taihu Lake): Implication for eco-toxicity risk. Chemosphere 202, 255–263. doi:10.1016/j.chemosphere.2018.03.108
Mee, (2022). "Ministry of ecology and environment of the People's republic of China," in Technical guideline for deriving water quality criteria for freshwater organisms HJ831-2022. Ministry of Ecology and Environment. Beijing: PRC. Avaliable at: https://www.mee.gov.cn/xxgk2018/xxgk/xxgk01/202011/t20201102_805937.html.
Mekni, S., Barhoumi, B., Touil, S., Driss, M. R., and Eljarrat, E. (2020). Occurrence of halogenated and organophosphate flame retardants in sediments and eels (Anguilla anguilla) from bizerte lagoon, Tunisia. Front. Environ. Sci. 8, 67. doi:10.3389/fenvs.2020.00067
Moore, D. R. J., Thompson, R. P., Rodney, S. I., Fischer, D., Ramanarayanan, T., and Hall, T. (2010). Refined aquatic risk assessment for aldicarb. Integr. Environ. Assess. Manag. 6 (1), 1–118. doi:10.1897/IEAM_2009-024.1
Moore, D. R. J., Teed, R. S., Greer, C. D., Solomon, K. R., and Giesy, J. P. (2014). Refined avian risk assessment for chlorpyrifos in the United States. Rev. Environ. Contam. Toxicol. 231, 163–217. doi:10.1007/978-3-319-03865-0_6
Niu, L., Zhao, X., Wu, F., Tang, Z., Lv, H., Wang, J., et al. (2021). Hotpots and trends of covalent organic frameworks (COFs) in the environmental and energy field: Bibliometric analysis. Sci. Total Environ. 783, 146838. doi:10.1016/j.scitotenv.2021.146838
Niu, Z., Zhang, Z., Li, J., He, J., and Zhang, Y. (2019). Threats of organophosphate esters (OPEs) in surface water to ecological system in Haihe River of China based on species sensitivity distribution model and assessment factor model. Environ. Sci. Pollut. Res. 26 (11), 10854–10866. doi:10.1007/s11356-019-04461-2
Noyes, P. D., Haggard, D. E., Gonnerman, G. D., and Tanguay, R. L. (2015). Advanced morphological — behavioral test platform reveals neurodevelopmental defects in embryonic zebrafish exposed to comprehensive suite of halogenated and organophosphate flame retardants. Toxicol. Sci. 145 (1), 177–195. doi:10.1093/toxsci/kfv044
Olawumi, T. O., and Chan, D. W. M. (2018). A scientometric review of global research on sustainability and sustainable development. J. Clean. Prod. 183, 231–250. doi:10.1016/j.jclepro.2018.02.162
Reemtsma, T., Quintana, J. B., Rodil, R., Garcı´a-López, M., and Rodrı´Guez, I. (2008). Organophosphorus flame retardants and plasticizers in water and air I. Occurrence and fate. TrAC Trends Anal. Chem. 27 (9), 727–737. doi:10.1016/j.trac.2008.07.002
Shi, Y., Gao, L., Li, W., Wang, Y., Liu, J., and Cai, Y. (2016). Occurrence, distribution and seasonal variation of organophosphate flame retardants and plasticizers in urban surface water in Beijing, China. Environ. Pollut. 209, 1–10. doi:10.1016/j.envpol.2015.11.008
Solomon, K., Giesy, J., and Jones, P. (2000). Probabilistic risk assessment of agrochemicals in the environment. Crop Prot. 19 (8), 649–655. doi:10.1016/S0261-2194(00)00086-7
Sun, L., Tan, H., Peng, T., Wang, S., Xu, W., Qian, H., et al. (2016a). Developmental neurotoxicity of organophosphate flame retardants in early life stages of Japanese medaka (Oryzias latipes). Environ. Toxicol. Chem. 35 (12), 2931–2940. doi:10.1002/etc.3477
Sun, L., Xu, W., Peng, T., Chen, H., Ren, L., Tan, H., et al. (2016b). Developmental exposure of zebrafish larvae to organophosphate flame retardants causes neurotoxicity. Neurotoxicol. Teratol. 55, 16–22. doi:10.1016/j.ntt.2016.03.003
Sutha, J., Anila, P. A., Gayathri, M., and Ramesh, M. (2022). Long term exposure to tris (2-chloroethyl) phosphate (TCEP) causes alterations in reproductive hormones, vitellogenin, antioxidant enzymes, and histology of gonads in zebrafish (Danio rerio): In vivo and computational analysis. Comp. Biochem. Physiology Part C Toxicol. Pharmacol. 254, 109263. doi:10.1016/j.cbpc.2021.109263
Sutha, J., Anila, P. A., Umamaheswari, S., Ramesh, M., Narayanasamy, A., Poopal, R-K., et al. (2020). Biochemical responses of a freshwater fish Cirrhinus mrigala exposed to tris(2-chloroethyl) phosphate (TCEP). Environ. Sci. Pollut. Res. 27 (27), 34369–34387. doi:10.1007/s11356-020-09527-0
Tarazona, J. V., Escher, B. I., Giltrow, E., Sumpter, J., and Knacker, T. (2010). Targeting the environmental risk assessment of pharmaceuticals: Facts and fantasies. Integr. Environ. Assess. Manag. 6 (S1), 1–613. doi:10.1897/IEAM_2009-052.1
Teo, T. L. L., Mcdonald, J. A., Coleman, H. M., and Khan, S. J. (2015). Analysis of organophosphate flame retardants and plasticisers in water by isotope dilution gas chromatography–electron ionisation tandem mass spectrometry. Talanta 143, 114–120. doi:10.1016/j.talanta.2015.04.091
Tsuji, S., Tonogai, Y., Ito, Y., and Kanoh, S. J. J. H. C. (1986). The influence of rearing temperatures on the toxicity of various environmental pollutants for killifish(Oryzias latipes). Eisei kagaku 32 (1), 46–53. doi:10.1248/jhs1956.32.46
U.S. Environmental Protection Agency (2015). TSCA work plan Chemical problem formulation and initial assessment, chlorinated phosphate ester cluster flame retardants. Washington, DC: United States Environmental Protection Agency. Avaliable At: https://www.epa.gov/assessing-and-managing-chemicals-under-tsca/tsca-work-plan-chemical.
Van Der Veen, I., and De Boer, J. (2012). Phosphorus flame retardants: Properties, production, environmental occurrence, toxicity and analysis. Chemosphere 88 (10), 1119–1153. doi:10.1016/j.chemosphere.2012.03.067
Venier, M., Dove, A., Romanak, K., Backus, S., and Hites, R. (2014). Flame retardants and legacy chemicals in great lakes’ water. Environ. Sci. Technol. 48 (16), 9563–9572. doi:10.1021/es501509r
Verbruggen, E. M. J., Rila, J. P., Traas, T. P., Posthuma-Doodeman, C. J. A. M., and Posthumus, R. (2005). Environmental risk limits for several phosphate esters, with possible application as flame retardant. RIVM report 60501024.
Wang, G., Shi, H., Du, Z., Chen, H., Peng, J., and Gao, S. (2017). Bioaccumulation mechanism of organophosphate esters in adult zebrafish (Danio rerio). Environ. Pollut. 229, 177–187. doi:10.1016/j.envpol.2017.05.075
Wang, T., Xu, C., Song, N., Zhang, S., Bu, Y., Xiong, L., et al. (2022). Seasonal variation and health risk assessment of organophosphate esters in surface and drinking water in Nanjing, China. Int. J. Environ. Sci. Technol, 1–12. doi:10.1007/s13762-022-03987-2
Wang, X., Fan, B., Fan, M., Belanger, S., Li, J., Chen, J., et al. (2020). Development and use of interspecies correlation estimation models in China for potential application in water quality criteria. Chemosphere 240, 124848. doi:10.1016/j.chemosphere.2019.124848
Wang, X., He, Y., Lin, L., Zeng, F., and Luan, T. (2014). Application of fully automatic hollow fiber liquid phase microextraction to assess the distribution of organophosphate esters in the Pearl River Estuaries. Sci. Total Environ. 470-471, 263–269. doi:10.1016/j.scitotenv.2013.09.069
Wang, X., Zhu, L., Zhong, W., and Yang, L. (2018). Partition and source identification of organophosphate esters in the water and sediment of Taihu Lake, China. J. Hazard. Mat. 360, 43–50. doi:10.1016/j.jhazmat.2018.07.082
Wei, G-L., Li, D-Q., Zhuo, M-N., Liao, Y-S., Xie, Z-Y., Guo, T-L., et al. (2015). Organophosphorus flame retardants and plasticizers: Sources, occurrence, toxicity and human exposure. Environ. Pollut. 196, 29–46. doi:10.1016/j.envpol.2014.09.012
Whitfield Aslund, M., Breton, R. L., Padilla, L., Winchell, M., Wooding, K. L., Moore, D. R. J., et al. (2017). Ecological risk assessment for Pacific salmon exposed to dimethoate in California. Environ. Toxicol. Chem. 36 (2), 532–543. doi:10.1002/etc.3563
Wu, Y., Su, G., Tang, S., Liu, W., Ma, Z., Zheng, X., et al. (2017). The combination of in silico and in vivo approaches for the investigation of disrupting effects of tris (2-chloroethyl) phosphate (TCEP) toward core receptors of zebrafish. Chemosphere 168, 122–130. doi:10.1016/j.chemosphere.2016.10.038
Xing, L., Tao, M., Zhang, Q., Kong, M., Sun, J., Jia, S., et al. (2020). Occurrence, spatial distribution and risk assessment of organophosphate esters in surface water from the lower Yangtze River Basin. Sci. Total Environ. 734, 139380. doi:10.1016/j.scitotenv.2020.139380
Xing, L., Zhang, Q., Sun, X., Zhu, H., Zhang, S., and Xu, H. (2018). Occurrence, distribution and risk assessment of organophosphate esters in surface water and sediment from a shallow freshwater Lake, China. Sci. Total Environ. 636, 632–640. doi:10.1016/j.scitotenv.2018.04.320
Xu, L., Zhang, B., Hu, Q., Liu, Y., Shang, T., Zeng, X., et al. (2021). Occurrence and spatio-seasonal distribution of organophosphate tri- and di-esters in surface water from Dongting Lake and their potential biological risk. Environ. Pollut. 282, 117031. doi:10.1016/j.envpol.2021.117031
Xu, T., Li, P., Wu, S., Lei, L., and He, D. (2017). Tris(2-chloroethyl) phosphate (TCEP) and tris(2-chloropropyl) phosphate (TCPP) induce locomotor deficits and dopaminergic degeneration in Caenorhabditis elegans. Toxicol. Res. 6 (1), 63–72. doi:10.1039/c6tx00306k
Yan, Z., Feng, C., Jin, X., Liu, D., Hong, Y., Qiao, Y., et al. (2021). In vitro metabolic kinetics of cresyl diphenyl phosphate (CDP) in liver microsomes of crucian carp (Carassius carassius). Environ. Pollut. 274, 116586. doi:10.1016/j.envpol.2021.116586
Yan, Z., Feng, C., Jin, X., Wang, F., Liu, C., Li, N., et al. (2022). Organophosphate esters cause thyroid dysfunction via multiple signaling pathways in zebrafish brain. Environ. Sci. Ecotechnology 12, 100198. doi:10.1016/j.ese.2022.100198
Yan, Z., Wang, W., Zhou, J., Yi, X., Zhang, J., Wang, X., et al. (2015). Screening of high phytotoxicity priority pollutants and their ecological risk assessment in China’s surface waters. Chemosphere 128, 28–35. doi:10.1016/j.chemosphere.2015.01.015
Yin, H., Liu, L., Liu, Q., Song, J., Fang, S., and Liu, X. (2022). Levels and distribution of organophosphate esters (OPEs) in typical megacity wetland park landscape water bodies in southwest China. Arch. Environ. Contam. Toxicol. 82 (1), 11–20. doi:10.1007/s00244-021-00899-z
Yuan, L., Li, J., Zha, J., and Wang, Z. (2016). Targeting neurotrophic factors and their receptors, but not cholinesterase or neurotransmitter, in the neurotoxicity of TDCPP in Chinese rare minnow adults (Gobiocypris rarus). Environ. Pollut. 208, 670–677. doi:10.1016/j.envpol.2015.10.045
Zha, D., Li, Y., Yang, C., and Yao, C. (2018). Assessment of organophosphate flame retardants in surface water and sediment from a freshwater environment (Yangtze River, China). Environ. Monit. Assess. 190 (4), 222. doi:10.1007/s10661-018-6587-5
Zhang, S., Ireland, D., Sipes, N. S., Behl, M., and Collins, E-M. S. (2019a). Screening for neurotoxic potential of 15 flame retardants using freshwater planarians. Neurotoxicol. Teratol. 73, 54–66. doi:10.1016/j.ntt.2019.03.003
Zhang, Z., Shao, H., Wu, M., Zhang, J., Li, D., Li, J., et al. (2019b). Occurrence, distribution, and potential sources of organophosphate esters in urban and rural surface water in Shanghai, China. Arch. Environ. Contam. Toxicol. 77 (1), 115–126. doi:10.1007/s00244-019-00633-w
Zhao, F., Wang, J., Fang, Y., Ding, J., Yang, H., Li, L., et al. (2016). Effects of tris(1, 3-dichloro-2-propyl)phosphate on pathomorphology and gene/protein expression related to thyroid disruption in rats. Toxicol. Res. 5 (3), 921–930. doi:10.1039/c5tx00374a
Zhao, Y., Yin, L., Dong, F., Zhang, W., and Hu, F. (2021). Effects of tris (2-chloroethyl) phosphate (TCEP) on survival, growth, histological changes and gene expressions in juvenile yellow catfish Pelteobagrus fulvidraco. Environ. Toxicol. Pharmacol. 87, 103699. doi:10.1016/j.etap.2021.103699
Keywords: tris(2-chloroethyl) phosphate, ecological risk assessment, toxicity effects, aquatic organisms, bibliometrics
Citation: Qiao Y, Liu D, Feng C, Liu N, Wang J, Yan Z and Bai Y (2022) Ecological risk assessment for tris(2-chloroethyl) phosphate to freshwater organisms. Front. Environ. Sci. 10:963918. doi: 10.3389/fenvs.2022.963918
Received: 08 June 2022; Accepted: 29 July 2022;
Published: 01 September 2022.
Edited by:
Wei He, China University of Geosciences, ChinaReviewed by:
Xiangzhen Kong, Nanjing Institute of Geography and Limnology (CAS), ChinaNing Qin, University of Science and Technology Beijing, China
Copyright © 2022 Qiao, Liu, Feng, Liu, Wang, Yan and Bai. This is an open-access article distributed under the terms of the Creative Commons Attribution License (CC BY). The use, distribution or reproduction in other forums is permitted, provided the original author(s) and the copyright owner(s) are credited and that the original publication in this journal is cited, in accordance with accepted academic practice. No use, distribution or reproduction is permitted which does not comply with these terms.
*Correspondence: Chenglian Feng, fengcl@craes.org.cn