- 1China-UK Low Carbon College, Shanghai Jiao Tong University, Shanghai, China
- 2School of Economics and Management, China University of Mining and Technology, Xuzhou, China
- 3School of International and Public Affairs, Shanghai Jiao Tong University, Shanghai, China
- 4Institute of Inner Mongolia, Shanghai Jiao Tong University, Huhhot, China
- 5School of Materials Science and Engineering, Shanghai Jiao Tong University, Shanghai, China
- 6School of Environmental Science and Engineering, Shanghai Jiao Tong University, Shanghai, China
Rare earth elements (REEs) are essential for promoting clean energy technologies and making high-performance materials because of their unique physical and chemical properties. In order to respond to climate change, the Chinese government has promised to achieve carbon neutrality before 2060. Under such a circumstance, the demand for REEs will increase significantly. However, several challenges exist in current REEs supply chain management in China. This policy brief discusses these challenges from a life cycle perspective, covering REEs mining, smelting, manufacturing, waste management, and recycling. Policy recommendations on future REEs supply chain management are then proposed, including adequate mining quota, a strategic REEs list, innovative high-tech applications, and circular economy.
1 Introduction
Rare earth elements (REEs) include scandium (Sc), yttrium (Y), and 15 kinds of lanthanide elements, including lanthanum (La), cerium (Ce), praseodymium (Pr), neodymium (Nd), promethium (Pm), samarium (Sm), europium (Eu), gadolinium (Gd), terbium (Tb), dysprosium (Dy), holmium (Ho), erbium (Er), thulium (Tm), ytterbium (Yb), and lutetium (Lu) (Zeng et al., 2020). These rare earth elements have outstanding optical, electrical, magnetic, and nuclear characteristics and can be composed with other materials to improve the quality and performance of various products. Currently, rare earth elements are mainly used in chemical, metallurgical, military, clean energy, and other high-tech industries (Wang X et al., 2017; LeeJason and Wen, 2018). The global rare earth reserves reached 0.13 Gt (gigatons) in 2017, among which China accounted for about 30% with a figure of 44 million tons (Das et al., 2018). Due to its rich reserve, China has become the largest REEs supplier in the world, supplying more than 60% of the global REEs production (Du and Graedel, 2011). However, with unsustainable and extensive mining activities during the past few decades, both the amount and the grade of rare earth ores have gradually reduced in China. As a result, rare earth elements were listed as strategic mineral resources by the Ministry of Natural Resources in 2016 (Ministry of Natural Resources, 2016). Meanwhile, in order to respond to climate change, many countries proposed to achieve carbon neutrality before 2050 and have begun their efforts to transit their energy systems towards renewable and clean energy systems, as well as through the rapid development of high-tech industry (Wei et al., 2021). With the future expansion on these emerging industries, the demand for rare earth elements will continue to increase, indicating new challenges to the global rare earth elements supply chain. Under such a circumstance, it would be urgent for China to manage its rare earth elements supply chain by considering these complicated realities. Based on the literature review and site investigations, this policy brief discusses the whole rare earth elements supply chain from a life cycle perspective so that valuable policy insights can be obtained. In the remaining part of this paper, Section 2 identifies the associated supply chain challenges in each life cycle stage; Section 3 proposes policy recommendations. Finally, Section 4 draws research conclusions.
2 Current challenges on rare earth elements supply chain in China
From a life cycle perspective, the rare earth elements supply chain can be divided into five stages in China, covering mining, smelting, manufacturing, waste management, and recycling (Figure 1). There are three major types of rare earth ores in China, including mixed monazite and bastnaesite in Bayan Obo ores of Inner Mongolia, bastnaesite ores in Sichuan and Shandong, and ionic clays in seven southern provinces. In particular, more than 80% of domestic rare earth elements were mined from Bayan Obo ores, the largest rare earth elements reserve in China (LeeJason and Wen, 2018). In the mining process. Rare earth elements are extracted from these natural reserves and beneficiated into high-grade concentrates through gravity separation, magnetic separation, electrostatic separation, and froth flotation. These concentrates are then refined and separated into rare earth oxides and other compounds by leaching, extraction, and precipitation in the smelting stage. During the manufacturing process, final REEs-containing products are produced. These final products will be consumed and become stocks serving the whole society. When these products reach the end of their life spans, they become scraps and will be recycled in the waste treatment plants or landfilled. To date, China has already built such a complete rare earth elements supply chain. But several challenges exist and should be identified for further improvement. We discuss these challenges along this supply chain.
2.1 Mining
Firstly, the production capacity of rare earth elements is far higher than the mining quota in China. Although China has rich rare earth elements reserves, the large export amount with lower economic values will deplete such reserves shortly. Therefore, in order to protect the domestic rare earth elements supply chain and control the total mining amount, the Ministry of Natural Resources and the Ministry of the Industry and Information Technology jointly released annual quota for such rare earth elements in different provinces. Figure 2 shows the annual quotas and demands of rare earth elements in China from 2011 to 2020. However, with rapid technological development, such quota is very limited and cannot meet with the soaring demand from those downstream companies. For example, the mining and smelting quota for Inner Mongolia was 73,550 and 63,784 tons in 2020, respectively. However, such mining and smelting production capacity was 125,000 and 100,000 tons in Inner Mongolia in 2020, respectively (Chinese Society of Rare Earths, 2011). Several relevant studies found that the demands for several rare earth elements, such as Nd, exceeded the official supply with the development of the emerging clean technologies (Packey and Kingsnorth, 2016; LeeJason and Wen, 2018; Geng et al., 2021). In order to achieve carbon neutrality before 2060, the demands for such rare earth elements will continuously increase (Wang, 2018). Thus, it is necessary to set adequate mining quotas to satisfy these companies’ production capacities and meet with the increasing demands for rare earth elements. Additionally, such mining process results in various environmental damages, such as radioactive contamination, soil and water contamination, and eutrophication (Wang L et al., 2017). Also, many suppliers of rare earth elements paid less attention on such environmental impacts due to their backward awareness, higher treatment costs, and ineffective enforcement of relevant regulations by local governments. From a legal point of view, current regulations on rare earth mining do not address such environmental impacts efficiently. As such, stricter and more suitable environmental standards are still lacking.
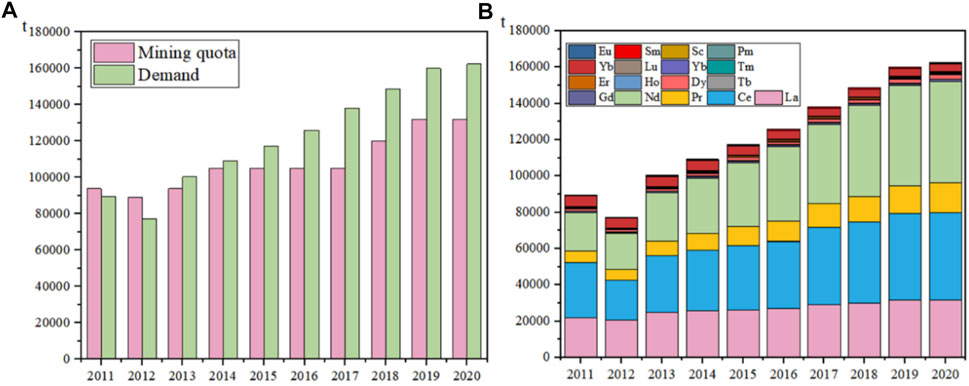
FIGURE 2. Annual mining quota and demand of rare earth elements in China from 2011 to 2020 (A); Annual demand for different rare earth elements in China from 2011 to 2020 (B). Data source: Chinese Society of Rare Earths Yearbooks.
2.2 Smelting and manufacturing
In the smelting process, the production amounts for different rare earth elements are significantly different. The amounts of several bulk rare earth elements, such as Nd, La, and Y, are hundreds of times that of other elements, such as Lu, Tm, and Er (Goodenough et al., 2018). Such differences are attributed by different applications of different rare earth elements (Figure 3). Renewable energy sector has a high demand for several rare earth elements (such as Nd, Y) since such elements are indispensable for making magnets. The annual demands for such rare earth elements always exceed their official supplies (Gong et al., 2017; Chen et al., 2018). However, the demands for rare earth elements in traditional industries (such as optical material) are lower, leading to oversupply, such as Eu (Wang L et al., 2020). In addition, since many rare earth elements are mined together, it is not easy to separate one specific rare earth element from the entire ore. For example, light rare earth elements are mainly extracted from iron tailings in Bayan Obo ores and become concentrates. It is extremely difficult to separate different elements from such concentrates. Also, since it is difficult for those companies to sell their rare earth elements surplus, they have to reserve such surplus by themselves, which increased their storage costs and influenced their monetary flows. Additionally, due to the lack of advanced technologies, several rare earth elements are mainly used for making primary products in China, such as rare earth-related oxides and compounds. For several heavy rare earth elements such as Tm, Yb, and Er, only their oxides are being circulated in the market. Their final products are mainly used as additives (Li et al., 2012). As such, Pm and Sc are the scarcest rare earth elements, but have not been applied in China (Wang L et al., 2020). However, these rare earth elements are key in several high-tech industries, especially under China’s ambitious carbon neutrality targets (Fishman and Graedel, 2019; Li et al., 2020; Wei et al., 2022). Thus, with the rapid development of emerging technologies, the demands for these REEs will grow.
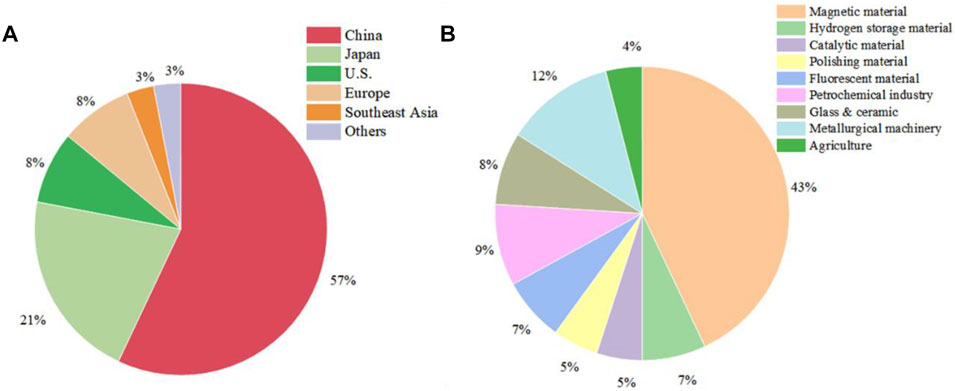
FIGURE 3. Leading suppliers of rare earth elements around the world (A); Main applications of rare earth elements in China (B). Data source: Chinese Society of Rare Earths Yearbooks
2.3 Waste management and recycling
When the REEs-containing final products reach the ends of their life spans, these products should be further treated before being discarded into the environment. It is rational to extend their life spans through repair, refurbish, remanufacturing, recycling, and reuse. However, waste collection and recycling system for rare earth elements is still ineffective in China, resulting in that most end-of-life REEs-containing final products flow into landfills directly without any treatment, especially for several light rare earth elements such as Eu, Gd, Sm. Currently, only Nd-Fe-B permanent magnet has been widely recycled in China because it has the highest demand and value among all the REEs (Jo, 2015). Theoretically, it is feasible to recycle such rare earth elements from these end-of-life REEs-containing final products (Rollat et al., 2016; Qiu and Suh, 2019). For example, by adding hydrochloric acid and oxalic acid, samarium can be recycled from Sm-Co magnet (Onoda and Kurioka, 2015). However, the recovery rate of Sm is still less than 1% in China due to the lack of policy support, backward awareness, a lack of economic incentives, and a lack of advanced recycling technologies (Xiao et al., 2022a; Ge et al., 2022).
3 Policy recommendations
First, since annual quota determines the real rare earth elements mining amount in China, it is essential to reallocate such annual quotas by considering the real needs from those downstream companies. This requires scientific evaluation on the real demands for various rare earth elements. Such evaluation should consider both domestic and international demands and avoid irrational allocation to those firms without reasonable production plans. Several studies have already been carried out in this field to evaluate the real demands for different rare earth elements in China. For example, Wang Q et al. (2020) and Xiao et al. (2022a) found the real demands for Eu and Y in China are less than their official supply, leading to enormous surplus. Ge et al. (2022) and Xiao et al. (2022b) found the real demands for Sm and Dy are gradually increasing in China, which may exceed their official supply in the future. Also, the Chinese government should set up appropriate environmental standards for rare earth mining. For example, heavy rare earth mining can lead to severe water pollution with a feature of excessive ammonium nitrogen, which is difficult to be removed for a long time (Packey and Kingsnorth, 2016). In order to mitigate such pollution, the Chinese government has updated national sewage treatment standards and installed on site detectors to measure mining waste water since 2019. Such standards should be updated regularly so that more parameters can be included. In addition, such environmental standards should be updated with the rapid development of innovative technologies so that these technologies can be promoted quickly. For example, ammonium-free mining technology has emerged and can replace the traditional mining process since it avoids using ammonium sulfate. Unfortunately, such ammonium-free process may result in adverse effects on human health since it uses highly concentrated magnesium (Schulze et al., 2017). Consequently, it is urgent for the Chinese government to prepare corresponding regulations to address this public health concern.
Second, rare earth element-specific management should be initiated since different rare earth elements have different features and application fields. Several countries only listed several key rare earth elements in their strategic mineral resource plans. For example, in 2022, the United States updated its strategic mineral resource plan (USDI, 2022), in which 15 kinds of key rare earth elements were listed (except Pm and Sc). However, China listed all the rare earth elements as strategic mineral resources. It would be crucial to further highlight several key rare earth elements in the national strategic mineral plan, such as Nd, Dy, Pm and Sc. These elements can play a key role in promoting renewable energy development and other emerging sectors. Future demands for these key rare earth elements may rise very quickly and should be carefully managed so that sustainable supply can be achieved. For those rare earth elements with surplus, since the demands for such elements are still low, they should be stored with careful plan, rather than relying on those firms. The Chinese government may establish several regional storage centers for such rare earth elements by collecting them from those firms. Such a measure can also help stabilize the prices of such rare earth elements.
In addition, since most of rare earth elements are mainly used for making primary products in China, it is important to encourage research and development (R&D) activities, especially under the ambitious 2060 carbon neutrality target. These activities can help incubate more advanced technologies so that more value-added products can be manufactured within China, such as permanent magnets, hydrogen storage materials. A government-industrial partnership should be created to facilitate such R&D efforts so that adequate funds can be received. Both universities and research institutes should actively engage in such R&D efforts so that they can understand the real industrial needs and focus on seeking potential solutions. Moreover, intellectual rights should be seriously protected so that the promotion of such innovative technologies can be achieved smoothly.
Finally, it is essential to improve the overall resource efficiency of rare earth elements by promoting circular economy. The total recycling rate of such rare earth elements-containing end-of-life products is less than 1% in China (Sprecher et al., 2015; Morimoto et al., 2021). Recently, Ge et al. (2022) found that if the recycling rate reaches 40% in China, approximately 1728 tons of Sm can be recovered from in-use stocks, which can meet domestic demand for 2 years. However, several barriers exist for the implementation of circular economy in these REEs-related businesses, such as the lack of recycling awareness, backward recycling technologies, ineffective collection system for such REEs-containing end-of-life products, and a lack of economic incentives. Therefore, more efforts should be made to solve these problems. For instance, the Chinese government may establish a national information center on rare earth elements products so that information and data on these end-of-life products can be shared by more stakeholders. Similarly, recycling technologies can be shared through this information center so that technology transfer can be achieved quickly. In addition, the Chinese government may provide financial subsides for such recycling activities so that more firms would like to engage in such efforts. As such, capacity-building activities should be initiated so that more stakeholders can improve their recycling awareness and actively participate in such recycling efforts.
4 Conclusion
Rare earth elements are essential to help China achieve its ambitious carbon neutrality target. However, several challenges exist. This study discusses these challenges along REEs supply chain, including mining, smelting, manufacturing, waste management, and recycling. The first challenge is that mining quota is not adequate in China, which cannot meet with the soaring demands. The second challenge is that each rare earth element has different features, resulting in various supply problems, including both supply shortage and surplus problem. The third challenge is that current applications of most rare earth elements are mainly used to make primary products, instead of high-tech products. Finally, the recycling system of rare earth elements is ineffective, leading to that the current recycling rate of rare earth elements is still below 1%. By considering the Chinese realities, several policy recommendations are proposed to improve the overall rare earth resource efficiency, especially under the consideration of achieving carbon neutrality, including adequate mining quota, appropriate strategic rare earth elements list, the application of circular economy.
Author contributions
ZG designed this study and other authors jointly wrote this paper.
Funding
This study is supported by the National Key R&D Program of China (No. 2019YFC1908501), the Natural Science Foundation of China (Nos. 72088101, 71904125, and 71810107001).
Conflict of interest
The authors declare that the research was conducted in the absence of any commercial or financial relationships that could be construed as a potential conflict of interest.
Publisher’s note
All claims expressed in this article are solely those of the authors and do not necessarily represent those of their affiliated organizations, or those of the publisher, the editors and the reviewers. Any product that may be evaluated in this article, or claim that may be made by its manufacturer, is not guaranteed or endorsed by the publisher.
References
Chen, W., Nie, Z., Wang, Z., Gong, X., Sun, B., Gao, F., et al. (2018). Substance flow analysis of neodymium based on the generalized entropy in China. Resour. Conserv. Recycl. 133, 438–443. doi:10.1016/j.resconrec.2018.02.019
Chinese Society of Rare Earths (2011). Chinese society of rare earths yearbook. Beijing, China: Metallurgical Industry Press–2020.
Das, S., Gaustad, G., Sekar, A., and Williams, E. (2018). Techno-economic analysis of supercritical extraction of rare earth elements from coal ash. J. Clean. Prod. 189, 539–551. doi:10.1016/j.jclepro.2018.03.252
Du, X., and Graedel, T. E. (2011). Global in-use stocks of the rare earth elements: A first estimate. Environ. Sci. Technol. 45, 4096–4101. doi:10.1021/es102836s
Fishman, T., and Graedel, T. E. (2019). Impact of the establishment of US offshore wind power on neodymium flows. Nat. Sustain. 2, 332–338. doi:10.1038/s41893-019-0252-z
Ge, Z., Geng, Y., Wei, W., and Zhong, C. (2022). Assessing samarium resource efficiency in China: A dynamic material flow analysis. Resour. Policy 76, 102638. doi:10.1016/j.resourpol.2022.102638
Geng, J., Hao, H., Sun, X., Xun, D., Liu, Z., Zhao, F., et al. (2021). Static material flow analysis of neodymium in China. J. Ind. Ecol. 25, 114–124. doi:10.1111/jiec.13058
Gong, X., Wang, Z., Chen, W., Sun, B., Gao, F., Nie, Z., et al. (2017). Substance flow analysis of rare Earth lanthanum in China. Mater. Sci. Forum 898, 2455–2463. doi:10.4028/www.scientific.net/msf.898.2455
Goodenough, K. M., Wall, F., and Merriman, D. (2018). The rare earth element: Demand, global resources, and challenges for resourcing future generations. Nat. Resour. Res. 27, 201–216. doi:10.1007/s11053-017-9336-5
Jo, J. (2015). The Study on activation of resource recycling through flow analysis of neodymium-based rare Earth magnets. J. Korea Soc. Waste Manag. 32, 500–508. doi:10.9786/kswm.2015.32.5.500
LeeJason, C. K., and Wen, Z. (2018). Pathways for greening the supply of rare earth elements in China. Nat. Sustain. 1, 598–605. doi:10.1038/s41893-018-0154-5
Li, C., Kang, S., Zhang, Q., and Sharma, C. (2012). Effectiveness of rare earth elements constrain on different materials: A case study in central asia. Environ. Earth Sci. 67, 1415–1421. doi:10.1007/s12665-012-1586-2
Li, J., Peng, K., Wang, P., Meng, J., Wei, W., and Yang, Q. (2020). Critical rare earth elements mismatch global wind-power ambitions. One Earth 3, 116–125. doi:10.1016/j.oneear.2020.06.009
Ministry of Natural Resources (2016). National plan for mineral resources 2016-2020. http://www.mnr.gov.cn/dt/ywbb/201810/t2018.1030_2285197.html.
Morimoto, S., Kuroki, H., Narita, H., and Ishigaki, A. (2021). Scenario assessment of neodymium recycling in Japan based on substance flow analysis and future demand forecast. J. Mat. Cycles Waste Manag. 23, 2120–2132. doi:10.1007/s10163-021-01277-6
Onoda, H., and Kurioka, Y. (2015). Recovery of samarium from cobalt–samarium solution using phosphoric acid. J. Environ. Chem. Eng. 3, 2825–2828. doi:10.1016/j.jece.2015.10.012
Packey, D., and Kingsnorth, D. (2016). The impact of unregulated ionic clay rare Earth mining in China. Resour. Policy 48, 112–116. doi:10.1016/j.resourpol.2016.03.003
Qiu, Y., and Suh, S. (2019). Economic Feasibility of recycling rare Earth oxides from end-of-life lighting technologies. Resour. Conserv. Recycl. 150, 104432. doi:10.1016/j.resconrec.2019.104432
Rollat, A., Guyonnet, D., Planchon, M., and Tuduri, J. (2016). Prospective analysis of the flows of certain rare earths in Europe at the 2020 horizon. Waste Manag. 49, 427–436. doi:10.1016/j.wasman.2016.01.011
Schulze, R., Lartigue-Peyrou, F., Ding, J., Schebek, L., and Buchert, M. (2017). Developing a life cycle inventory for rare earth oxides from ion-adsorption deposits: Key impacts and further research needs. J. Sustain. Metall. 3, 753–771. doi:10.1007/s40831-017-0139-z
Sprecher, B., Daigo, I., Murakami, S., Kleijn, R., Vos, M., Kramer, G., et al. (2015). Framework for resilience in material supply chains, with a case study from the 2010 Rare Earth Crisis. Environ. Sci. Technol. 49, 6740–6750. doi:10.1021/acs.est.5b00206
Usdi (2022). Critical mineral resources of the United States—economic and environmental geology and prospects for future supply. United States: USGS.
Wang, L., Huang, X., Yu, Y., Zhao, L., Wang, C., Feng, Z., et al. (2017). Towards cleaner production of rare earth elements from bastnaesite in China. J. Clean. Prod. 165, 231–242. doi:10.1016/j.jclepro.2017.07.107
Wang, L., Wang, P., Chen, W., Wang, Q., and Lu, H. (2020). Environmental impacts of scandium oxide production from rare earths tailings of Bayan Obo Mine. J. Clean. Prod. 270, 122464. doi:10.1016/j.jclepro.2020.122464
Wang, Q., Wang, P., Qiu, Y., Dai, T., and Chen, W. (2020). Byproduct surplus: Lighting the depreciative europium in China's rare Earth boom. Environ. Sci. Technol. 54, 14686–14693. doi:10.1021/acs.est.0c02870
Wang, S. (2018). Study on present situations, problems and strategies of rare Earth industry under there form of supply-side. China Min. Mag. 27, 6–11.
Wang, X., Wei, W., Ge, J., Wu, B., Bu, W., Li, J., et al. (2017). Embodied rare earths flow between industrial sectors in China: A complex network approach. Resour. Conserv. Recycl. 125, 363–374. doi:10.1016/j.resconrec.2017.07.006
Wei, W., Ge, Z., Geng, Y., Jiang, M., Chen, Z., Wu, W., et al. (2022). Toward carbon neutrality: Uncovering constraints on critical minerals in the Chinese power system. Fundam. Res. 2, 367–374. doi:10.1016/j.fmre.2022.02.006
Wei, W., Li, J., Chen, B., Wang, M., Zhang, P., Guan, D., et al. (2021). Embodied greenhouse gas emissions from building China’s large-scale power transmission infrastructure. Nat. Sustain. 4, 739–747. doi:10.1038/s41893-021-00704-8
Xiao, S., Geng, Y., Pan, H., Gao, Z., and Yao, T. (2022b). Uncovering the key features of dysprosium flows and stocks in China. Environ. Sci. Technol. 56, 8682–8690. doi:10.1021/acs.est.1c07724
Xiao, S., Geng, Y., Rui, X., Su, C., and Yao, T. (2022a). Behind of the criticality for rare earth elements: Surplus of China’s yttrium. Resour. Policy 76, 102624. doi:10.1016/j.resourpol.2022.102624
Keywords: rare earth elements, supply chain management, carbon neutrality, policy implication, high-tech industry
Citation: Ge Z, Geng Y, Dong F, Liang J and Zhong C (2022) Towards carbon neutrality: Improving resource efficiency of the rare earth elements in China. Front. Environ. Sci. 10:962724. doi: 10.3389/fenvs.2022.962724
Received: 06 June 2022; Accepted: 04 July 2022;
Published: 16 August 2022.
Edited by:
Jiashuo Li, Shandong University, ChinaReviewed by:
Haoqi Qian, Fudan University, ChinaYadong Yu, East China University of Science and Technology, China
Copyright © 2022 Ge, Geng, Dong, Liang and Zhong. This is an open-access article distributed under the terms of the Creative Commons Attribution License (CC BY). The use, distribution or reproduction in other forums is permitted, provided the original author(s) and the copyright owner(s) are credited and that the original publication in this journal is cited, in accordance with accepted academic practice. No use, distribution or reproduction is permitted which does not comply with these terms.
*Correspondence: Fanli Dong, dongfanli@sjtu.edu.cn; Yong Geng, ygeng@sjtu.edu.cn.