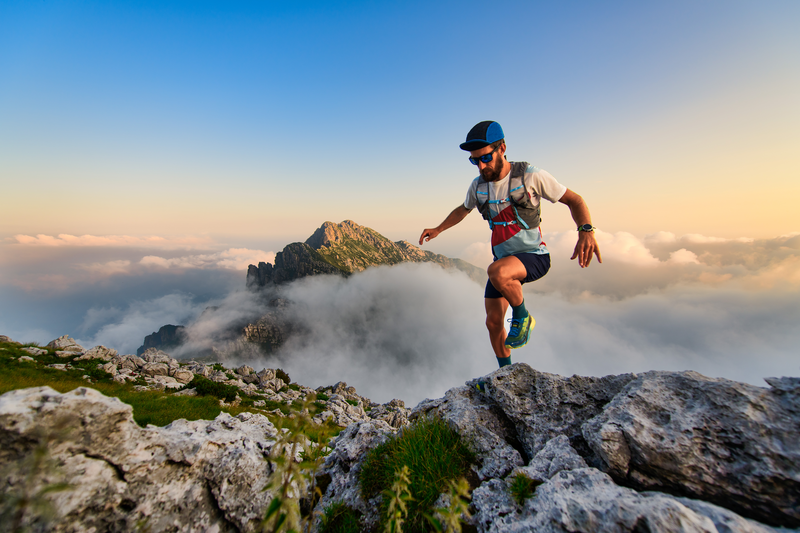
94% of researchers rate our articles as excellent or good
Learn more about the work of our research integrity team to safeguard the quality of each article we publish.
Find out more
ORIGINAL RESEARCH article
Front. Environ. Sci. , 29 September 2022
Sec. Drylands
Volume 10 - 2022 | https://doi.org/10.3389/fenvs.2022.960969
This article is part of the Research Topic Ecological Restoration in Drylands: Towards Land Degradation Neutrality View all 19 articles
Different branching architectures reflect the adaptation strategies of different plants and affect their resistance to wind erosion. This study presents field-based observations that demonstrate the relationship between the branching architecture of Artemisia ordosica and its resistance to wind erosion. This species is the dominant plant species in the semi-fixed and fixed dunes of the Mu Us Sandy land. The overall bifurcation ratio (OBR) of semi-fixed sandy land is higher than the fixed sandy land 0.27; Similarly, the total stepwise bifurcation ratio (SBR) is higher than the fixed sandy land about 0.74; The length of first levels of total branches is also higher than 8.07. The aerodynamic roughness was greater than the A. ordosica community in the fixed and semi-fixed sandy land than in the bare sandy land. The airflow fields in the cross-wind direction were strongly affected by the windward shape of the plants, which became gradually narrower from the base to the top, while in the leeward direction, the wind speed at different heights behind the plant returned to the incoming airflow velocity. The result confirms that the influence of the windward shape of the plant on the surrounding airflow field is much larger than the influence of plant thickness, porosity or other factors.
Psammophytes are adapted to growing in special habitat conditions. Their adaptation characteristics include resistance to wind erosion, sand burial, aridity and soil infertility, and these adaptations play an important role in maintaining the stability of the fragile ecosystem and in reconstructing damaged ecosystems (Zhou, 2001). Psammophytes influence the protection of surface soil in complex ways. Previous research has focused on the effect of plant groups rather than individual plants. However, the morphological characteristics of single plants have a great influence on sand resistance and fixation.
A plant can be regarded as a collection of modules. There are variety modules having different architecture characteristics due to their arrangement in space, so a plant presents diverse morphological structures (Harper, 1977; White, 1979; Barlow, 1989). The concept of the architecture characteristics of the plant, which in a narrow sense mainly refers to branching architecture, was advanced by Halle and others in 1978. Different branching architectures not only reflect the utilization of space, light and other resources by plants, but also indicate different adaptation strategies of plants at different growth stages. At the same time, branching architectures affects a plant’s resistance to wind erosion.
With respect to the branching architecture of plants, woody plants and herbs growing in tropical or humid areas have been relatively well studied. Research both in China and abroad has mainly focused on the morphological characteristics of plant branches and the quantitative study of the meristem dynamic relationship (Sprugel et al., 1991; Ohsawa and Nitta., 1997; Nitta and Ohsawa. 1998; Sun and Chen., 1999). For woody plants, studies have often focused on the crown and trunk studying the development, morphology and growth dynamics of branching, and dynamic changes and mutual relations among other components of the branches. Studies of herbs have mainly addressed the adaptation strategies of clonal ramets as reflected in their placement mode in different habitats. However, studies of the branching architecture of psammophytes have been much less common. Existing research has primarily focused on the quantitative study of plant populations at the component level, as well as adaption strategies to different environments as reflected in different branching architectures (Anwar and Yin, 1997; Sun et al., 1997; He et al., 2005, He et al., 2006; Zhang et al., 2009). Few studies have concentrated on the influence of psammophyte branching architecture on resistance to wind erosion.
The Mu Us Sandy land is located in the transition zone between arid or semi-arid areas and sub-humid areas of northern China. The region is a typical multi-level ecotone in terms of geography and geology and is one of the areas most seriously affected by desertification in China (Wu and Ci., 1998). Due to these special features, the Mu Us Sandy land has a variety plant types, and shrubs are the dominant species. The psammophytic semi-shrub community, dominated by the species Artemisia ordosica, is the most developed type, playing a significant role in desert ecosystem reconstruction and rehabilitation. A. ordosica, which commonly appears at the southern edge of the Mu Us Sandy land, was selected as the subject of this study. This study investigates the resistance of A. ordosica to wind erosion through its architecture characteristics. The result of this study has important scientific and practical significance: quantitative determination of the mechanisms used by psammophytes to resist wind erosion, and the exploration of plant variety in the semi-fixed and fixed dunes of the Mu Us Sandy land.
The study was conducted during the 2016 windy season, in the site of an A. ordosica community for windbreak and sand-fixation at the southern edge of the Mu Us Sandy land in northern China (37°38′42”∼37°47′54″N, 108°50′54”∼108°58′00″E) (Figure 1). This location is in a semi-arid region with a temperate monsoon climate characterized by hot summers and mild winters. Desert shrubs and herbs comprise the primary plant communities in the research area. A. ordosica is the most developed type, and its communities occupy most of the sand dunes and sand ridges. The site receives approximately 400 mm annual average rainfall, mostly during the summer (www.cdc.cma.gov.cn). Annual pan evaporation exceeds 2,400 mm, which is over 6 times greater than annual average precipitation (www.cdc.cma.gov.cn). Thirty-year average annual wind speed is 3.2 m s−1. Aeolian sand activity occurs mainly between March to May, when wind speeds are greater than 4.0 m s−1 (the threshold velocity of sand), and this period accounts for 50.2% of total annual aeolian activity. Vegetation recovery began in the 1970s. Most drifting dune activity ceased after afforestation, and semi-fixed dunes and fixed dunes are now the prevailing landforms.
FIGURE 1. Location of the study area on the southern fringe of the Mu Us Sandy land, China (37°38′42”∼37°47′54″N, 108°50′54”∼108°58′00″E).
Two areas were chosen on the semi-fixed and fixed dunes, where A. ordosica is concentrated within the research area. Ten mature plants were selected as the research samples in each site. The plant selection criteria included similar size, good growth, no pest or man-made damage, and a distance far enough from other plants to reduce the impact on plant modules of intraspecific and interspecific competition for space resources. A steel tape (with a precision of 0.1 cm) was used to measure the height, diameter at chest height, branch lengths at all levels, the interval between branches and other parameters of each plant. A protractor was used to measure the inclination of the branches and a caliper was used to measure the base diameter of the branches at all levels. The branch sequence was defined on the basis of the Strahler method (Sun and Chen, 1999), which is used to determine the branch sequence from the outside to the inside of the canopy layer. The first external branch is the first level, the branch formed by intersection of the two first levels is the second level, and so forth. If different branch levels intersect, then the one that is higher is regarded as the branch level.
Wind profile observation was conducted in the two study areas mentioned above. At the same time, one bare sandy area with no vegetation cover, was selected within the study site as a control plot. Wind speed was measured continuously at 9 different heights in the center of the communities using anemometers connected to a data logging system. The wind cup heights of the measuring points were 5 cm, 25 cm, 50 cm, 75 cm, 100 cm, 150 cm, 200 cm, 300 cm and 400 cm. Wind speed was recorded with a 30 s frequency, and each group of data was recorded for 5 min, making a total of 10 measurements in each data group. We ensured that the atmosphere was relatively stable while the test was being conducted. Data collected below the threshold velocity of sand (4 m s−1) were selected for regression analysis (Wu, 2003). Wind profiles were drawn, and wind roughness was calculated.
An individual shrub on a gentle slope in the semi-fixed sandy terrain was selected for airflow visualization. There were 67 total measuring points located at the windward side, leeward side and crosswind side of the plant. Three-cup wind speed sensors for each measuring point were located at the base, the mean height, the top of the plant and at a height of 200 cm. Simultaneously, one control site was set at an open location on the windward side, and the wind velocity at a height of 200 cm was used as a control. Each point was continuously measured for 5 min. After taking the average, the data were standardized according to the values obtained during the corresponding period at the control site, so that the data from all of the measuring points were converted to the average wind speed during the same 5 min period. The airflow fields at the other three heights were then obtained.
Branching architecture was analyzed using the following indicators: bifurcation ratio, the ratio of branch diameters, and the fractal dimensions of branching. The ratio of branch diameter (RBD) is given by
where
where
where
where C is a constant proportionality coefficient. After transformation, the above equation can be written as follows:
There is a good visible linear relationship between SB and LB when plotted on double logarithm coordinates, and D, the fractal dimension, is the slope of the resulting line.
The collected wind speed data was analyzed by converting data to the average wind speed within 5 min from the beginning of observations. The standardized method adapted from Zhang et al., (2006) is as follows:
where
The average plant height of the sampled A. ordosica on the semi-fixed sandy land was 55 ± 0.4 cm with a porosity of 48%, and an average crown size of 150 ± 2 cm × 140 ± 2 cm. However, the average plant height on the fixed sandy land was 50 ± 0.3 cm with a porosity of 49%, and an average crown size of 130 ± 2 cm × 120 ± 2 cm. A. ordosica grows better on semi-fixed dunes, sandy land and soil covered with sand and grows particularly well on loose sand dunes. However, on fixed dunes, due to enhanced soil compaction, A. ordosica is less suited to the environmental conditions. Although the number of plants can be large, the plant sizes are generally small. The number of dry branches increases and growth declines gradually. The seeds are small and light, and it is difficult for seeds to be retained in sand dunes, thus A. ordosica has a relatively smaller distribution in mobile sandy environments.
Branching angles are not only important indicators of plant spatial distribution, but are also a factor in the plants’ erosion resistence. A. ordosica has a wide base and a gradually narrowing middle-upper part and is visualized as a hemisphere based on this morphology. The outside branches of the plant are weak branching angles, with branching angles between 35° and 50°. Partial branches may grow nearly horizontally in a creeping manner. This structure is beneficial for forming adventitious roots for clonal growth, generating new plants, and enhancing vegetation coverage of the covered sandy land. The interior branches of the plant are mostly middle branching angles, with branching angles between 50° and 70°, along with some strong branching angles between 70° and 85°. This structure means that the growth of each branch does not disturb the growth of the other branches, thereby maximizing the use of space. There was little difference in the inclinations of the highest level of branches on the windward, leeward and crosswind sides, which demonstrates that they were not influenced by the force of the wind. However, the angles of the penultimate levels of branches had different values (Table 1).
The branching angles of the penultimate levels of branches on the windward side were between 30° and 80°, with a relatively large range, and included weak branching angle types and middle branching angle types. Due to the more direct exposure to wind on the windward side, branching angles of the outside penultimate levels of branches gradually increased. However, the angles on branches on the internal penultimate levels were closer to the angles at the highest level, and the branching angles decreased. Branching angles of the branches on the leeward side, were weak branching angles. The branching angles on the crosswind side, which was less affected by the wind, were in between the angles on the windward and leeward sides.
The branch diameter ratio (RBD) is an indicator of the bearing capacity of different levels of branch. The larger the branch diameter, the greater the loading on branches at the next level. In general, if the plant has a large branch diameter, the bifurcation ratio is relatively large. The bifurcation ratio describes the branching capability of the branches, as well as the number of configurations among all branch levels. The value of the bifurcation ratio is closely related to the branching angle and the branch diameter ratio. A higher bifurcation ratio shows a higher level of a plant’s utilization of its spatial resources.
The average number of the highest level of branches of A. ordosica on the windward side was equal to the number on the leeward side, and both were less than that of the crosswind side. The branches on the windward and the leeward sides accounted for 27% of the total number of branches on each side, while the branches on the crosswind side accounted for 46% of the total number. The distribution of the branches in all four directions was similar, leading each individual plant to resemble a symmetrical semicircle. Wind had no significant effect on the distribution of the branches of A. ordosica.
The branches of A. ordosica were divided into 4-5 levels in the fixed sandy land and 3 levels in the semi-fixed sandy land. The index statistics for the branching architecture on the windward, leeward and crosswind sides, and for branches divided according to the azimuth of the highest levels of branches, are given in Table 2.
The overall branching ratio of A. ordosica on the semi-fixed sandy land was greater than that of plants on the fixed sandy land, but both were less than 5 and had low branching rates. The overall branching ratio on the leeward side was less than that on the windward and crosswind sides. The branching number of the highest level of branches was the greatest at each azimuth on the semi-fixed sandy land. However, in the fixed sandy land, the number of the highest level of branches was greatest on the windward and crosswind sides, while the number of second level branches was the greatest on the leeward side. The ratio of branch diameter in the semi-fixed sandy land was larger than that in the fixed sandy land, and the base diameter of the highest level of branches on the crosswind side was the largest. The branch diameter ratio of branches in all directions in the semi-fixed sandy land showed little difference, while in the fixed sandy land the ratio in the windward direction was larger than that on the leeward and crosswind sides. These characteristics of A. ordosica not only reflect the impact of blown sand activities, but also indicate a type of adaptive strategy that simultaneously plays an effective role in controlling wind erosion and desertification.
The fractal dimension of branching (D) is an important parameter for measuring the spatial distribution pattern of plant branches. If D is less than 1.4000, the plant has a weak fractal dimension (WD), indicating that the branching pattern of the plant is simple. If D is larger than 1.8000, the plant would have a strong fractal dimension (SD), showing that the branching pattern of the plant is complex. If D is between 1.4000 and 1.8000, the plant has a moderate fractal dimension (MD). After fitting each level of branch numbers (SB) and branch lengths (LB) of the sampled A. ;ordosica plants in the semi-fixed and fixed sandy lands, the straight lines and fractal dimensions are shown in Figure 2.
FIGURE 2. Linear fitting of the fractal dimension of branching of Artemisia ordosica in sandy land [(A) Semi-fixed sandy land; (B) Fixed sandy land].
A. ordosica exhibited different D values in the three directions. On fixed sandy land, A. ordosica was WD on the leeward and crosswind sides, and SD on the windward side. In semi-fixed sandy land, they were MD on the windward and the leeward side, and SD on the crosswind side. Because growth of A. ordosica was poor in the fixed sandy land, D was smaller than in the semi-fixed sandy land and the branching pattern was relatively simple. Because blown sand activities in the semi-fixed sandy land is stronger than in fixed sandy land, branches on the windward side were more strongly affected by in the fixed sandy land. However, in the semi-fixed sandy land, the branches on the windward and the crosswind side developed a more complex branching pattern, which enlarged the upwind projected area of the plant, so as to adapt to the blown sand.
When air flows over a surface covered by vegetation, the projected upwind shape of the plants plays a key role in their resistances to wind-blown sand. The shape of the plants can be expressed by differences in their morphological structures, including their height, width, and porosity. The width of the windward side at different heights of the plants under investigation is shown in Figure 3.
The average height of the plants was 0.53 m, and the average width on the windward side was 0.90 m. The widest part of the windward side was located within 0–20 cm of the base and gradually decreased with height. This hemispherical shape is conducive to sand accumulation at the base of the plant.
Aerodynamic roughness (z0) is defined as the height where surface wind velocity decreases to zero. It represents the aerodynamic characteristics of the surface and reflects the decreasing effects of wind speed on the ground surface and the influence of ground surface on wind airflow (Liu and Dong, 2003). The most common method for calculating aerodynamic roughness is using a least squares method with a logarithmic profile equation for the measured wind profile (Dong et al., 2001). Given stable and neutral atmospheric stratification, the velocity profile has a logarithmic relationship as follows:
where uz is the velocity at height z (m·s−1), and a and b are fitted constants. If uz = 0, then:
A. ordosica communities with similar plant heights, numbers and uniform distributions were selected on the fixed sandy land and semi-fixed sandy land. Coverage of the fixed sandy land with generated crusts was 35%, while coverage of the semi-fixed sandy land was 20%. According to wind velocity observations, four groups of wind speed, at a height of 2 m, were lower than the threshold velocity of the surface that was used to fit the wind profiles (Figure 4), and these wind speeds were then used to calculate the aerodynamic roughness (z0).
FIGURE 4. Wind profiles of the Artemisia ordosica community on sandy land under non-sand-driving wind [(A) Semi-fixed sandy land; (B) Fixed sandy land].
The logarithmic curve fitting result of wind profile is relatively accurate, and the fitting coefficient (R2) is close to 0.9. z0 of the A. ordosica community in the fixed sandy land was between 2.70 and 3.36 cm, and that in the semi-fixed sandy land was between 2 and 2.78 cm. Both were larger than the value in the bare sandy land, which was 0.04 cm (Qiu et al., 2004). Roughness of the fixed sandy land was enhanced, which supports positive succession of plants and soils.
An individual shrub, that was 55 cm high and located on a gentle slope in semi-fixed sandy land, was selected for airflow visualization. Three-cup wind speed sensors at each measuring point were located at heights of 5 cm, 30 cm and 55 cm, respectively. The results from the airflow fields at each of the three heights are shown in Figure 5. From the figure, it can be observed that the airflow fields change drastically because wind velocity in the cross-wind direction was affected by the windward shape of the plant, which became gradually narrower from the base to the top. Two wind acceleration regions formed on both sides of the plants. Those at the base were larger and affected a range of 2.50 m behind the plant, while at the mean of the range, 1.50 m behind the plant was affected, and at the top only a range of 0.50 m behind the plant was affected. The wind acceleration regions at the base and the mean were located at the post-lateral side of the plant and 1 m away from the center of the plant. However, the wind acceleration regions at the top were located at both sides of the plant and 2 m away from the center of the plant. Although the plant widths became gradually narrower from bottom to top, the wind speed in the windward direction at different heights behind the plants returned to the incoming airflow velocity approximately 2 m from the plant. This result confirms that the influence of the windward shape of a plant on the surrounding airflow field is much larger than the influence of plant thickness, porosity or other factors.
Research on the impact of the branching architecture of psammophytes on the prevention of wind erosion is still in its early stages. Although this study has presented a number of results, there are many issues that require further research.
The architecture of psammophytes is not only the result of their growth, development and adaptation, but is also a restrictive condition that affects their further development at certain architectural growth stages. Not only does the difference in architecture influence resistances to wind erosion, but sand airflow also plays a role in formation of the architecture. Elimination of other environmental factors is the key to analyzing the effect on wind erosion control of the architecture under field conditions. However, a good method has still not been developed to achieve this goal. Pot experiments can simulate environmental conditions to a certain extent, but there are still significant shortcomings with this method. One limitation is that cultivation requires an extended period of time. Also, differences have been reported between pot experiments and field studies because the experiments do not entirely represent the field conditions. Therefore, better methods to overcome this problem need to be developed through future studies.
The classification of psammophyte architecture is the basis for researching the mechanisms of resistane to wind erosion. He et al., 2005 divided desert plants into four types according to their architecture index, based on statistics relating to branching architecture, including all levels of branching angles, branch length, branch diameter ratios and other indicators. However, they did not consider the effects of environmental conditions on the differences in architecture. To describe the architecture characteristics fully, one needs to describe, for instance, the change in psammophyte architecture with growth stage, the external space-time difference, the climate characteristics and the geographical differences in soils to establish a psammophyte architecture index system to enable comprehensive classification of psammophytes.
The original contributions presented in the study are included in the article/Supplementary Material, further inquiries can be directed to the corresponding author.
ZQ: Writing - original draft, Data curation, Methodology, Conceptualization. ZL: Co-first Author, Writing - review & editing. LH: Writing - review & editing. LL: corresponding author, Writing - review & editing, Conceptualization, Methodology. XH: Methodology. GZ: Writing - review. YL: Writing - review, Data curation. LG: Methodology. YY: Methodology. YZ: Writing - review & editing. GH: Methodology. All authors contributed to the article and approved the submitted version.
This research was supported by a grant from the National Natural Science Foundation of China (No. 31960361) and Natural Science Foundation of Inner Mongolia Autonomous Region (No. 2022MS04010). The authors express their sincere appreciation to their supporters. All errors and omissions are the responsibility of the authors.
The authors declare that the research was conducted in the absence of any commercial or financial relationships that could be construed as a potential conflict of interest.
All claims expressed in this article are solely those of the authors and do not necessarily represent those of their affiliated organizations, or those of the publisher, the editors and the reviewers. Any product that may be evaluated in this article, or claim that may be made by its manufacturer, is not guaranteed or endorsed by the publisher.
The Supplementary Material for this article can be found online at: https://www.frontiersin.org/articles/10.3389/fenvs.2022.960969/full#supplementary-material
Anwar, M., and Yin, L. K. (1997). The study on biomass of tamarix. Environ. Prot. Xinjiang 1, 46–50.
Barlow, P. W. (1989). Meristems, metamers and modules and the development of shoot and root systems. Botanical J. Linn. Soc. 100, 255–279. doi:10.1111/j.1095-8339.1989.tb01721.x
Dong, Z. B., Wang, X. M., Zhao, A. G., Liu, L. Y., and Liu, X. W. (2001). Aerodynamic roughness of fixed sandy beds. J. Geophys. Res. 6, 11001–11011. doi:10.1029/2001jb900009
He, M. Z., Wang, H., and Zhang, J. G. (2005). Classification of the branching architectures of the desert plants in minqin county. Acta Bot. boreal.–occident. Sin. 9, 1827–1832. doi:10.1360/biodiv.050022
He, M. Z., Zhang, J. G., and Wang, H. (2006). Analysis of branching architecture factors of desert plants. J. desert Res. 4, 625–630. doi:10.3321/j.issn:1000-694X.2006.04.023
Liu, X. P., and Dong, Z. B. (2003). Review of aerodynamic roughness length. J. desert Res. 4, 337–346.
Nitta, I., and Ohsawa, M. (1998). Bud structure and shoot architecture of canopy and understorey evergreen broad-leaved trees at their northern limit in east asia. Ann. Bot. 81, 115–129. doi:10.1006/anbo.1997.0545
Ohsawa, M. M., and Nitta, I. (1997). Patterning of subtropical ⁄ warm-temperate evergreen broad-leaved forests in east asian mountains with special reference to shoot phenology. Tropics 6, 317–334. doi:10.3759/tropics.6.317
Qiu, G. Y., In-Bok, L., Shimizu, H., Gao, Y., and Ding, G. D. (2004). Principles of sand dune fixation with straw checkerboard technology and its effects on the environment. J. Arid Environ. 56, 449–464. doi:10.1016/s0140-1963(03)00066-1
Sprugel, D. G., Hinckley, T. M., and Schaap, W. (1991). The theory and practice of branch autonomy. Annu. Rev. Ecol. Syst. 22, 309–334. doi:10.1146/annurev.es.22.110191.001521
Steingraeber, D. A., and Waller, D. M. (1986). Non-stationarity of tree branching patterns and bifurcation ratios. Proc. R. Soc. Lond. B 228, 187–194. doi:10.1098/rspb.1986.0050
Sun, J. M., li, G. T., Qin, F. C., Sun, F., Zhang, D. Y., Jia, S. Y., et al. (1997). The prediction research of aboveground biomass of artificial Haloxylon ammodendron forestry. Inn. Mong. For. Sci. Technol. 3, 9–11.
Sun, S. C., and Chen, L. Z. (1999). The architectural variation of Quercus liaotungensis in different habitats. Acta Ecol. Sin. 3, 359–364. doi:10.3321/j.issn:1000-0933.1999.03.012
White, J. (1979). The plant as a metapopulation. Annu. Rev. Ecol. Syst. 10, 109–145. doi:10.1146/annurev.es.10.110179.000545
Whitney, G. G. (1976). The bifurcation ratio as an indicator of adaptive strategy in woody plant species. Bull. Torrey Botanical Club 103, 67–72. doi:10.2307/2484833
Wu, B., and Ci, L. J. (1998). The developing situation and expanding causes of desertification in Mu Us Sandy Land since the 1950s. Quat. Sci. 2, 165–172.
Zhang, C. L., Zou, X. Y., Cheng, H., Yang, S., Pan, X. H., and Wang, H. T. (2006). Near surface air flow field across the shelterbelt of shapotou section, Baotou-Lanzhou line. J. Basic Sci. Eng. 3, 353–360. doi:10.3969/j.issn.1005-0930.2006.03.006
Zhang, D. K., Wang, J. H., Ma, Q. L., Liu, H. J., and Liu, Y. J. (2009). Study on branch module characteristics of Artemisia ordosica and Artemisia arenaria. Grassl. Turf 1, 43–46. doi:10.13817/j.cnki.cyycp.2009.01.001
Keywords: branching architecture, wind erosion, growing locations, psammophyte, Mu Us sandland
Citation: Qu Z, Li Z, Hu L, Liu L, Hu X, Zhang G, Lv Y, Guo L, Yang Y, Yang Z and Han G (2022) The branching architecture of artemisia ordosica and its resistance to wind erosion. Front. Environ. Sci. 10:960969. doi: 10.3389/fenvs.2022.960969
Received: 03 June 2022; Accepted: 02 September 2022;
Published: 29 September 2022.
Edited by:
Guang-Lei Gao, Beijing Forestry University, ChinaReviewed by:
Rende Wang, Hebei Academy of sciences, ChinaCopyright © 2022 Qu, Li, Hu, Liu, Hu, Zhang, Lv, Guo, Yang, Yang and Han. This is an open-access article distributed under the terms of the Creative Commons Attribution License (CC BY). The use, distribution or reproduction in other forums is permitted, provided the original author(s) and the copyright owner(s) are credited and that the original publication in this journal is cited, in accordance with accepted academic practice. No use, distribution or reproduction is permitted which does not comply with these terms.
*Correspondence: Lianyou Liu, TGlhbnlvdS5MaXVAYm51LmVkdS5jbg==
Disclaimer: All claims expressed in this article are solely those of the authors and do not necessarily represent those of their affiliated organizations, or those of the publisher, the editors and the reviewers. Any product that may be evaluated in this article or claim that may be made by its manufacturer is not guaranteed or endorsed by the publisher.
Research integrity at Frontiers
Learn more about the work of our research integrity team to safeguard the quality of each article we publish.