- 1College of Life and Environmental Sciences, Minzu University of China, Beijing, China
- 2Key Laboratory of Ecosystem Network Observation and Modeling, Institute of Geographic Sciences and Natural Resources Research, Chinese Academy of Science, Beijing, China
- 3College of Resources and Environment, University of Chinese Academy of Sciences, Beijing, China
- 4Center for Ecological Research, Northeast Forestry University, Harbin, China
Although it is important to determine how the global carbon (C) cycle is responding to climate change in our three-dimensional Earth, variation in the soil organic matter (SOM) and temperature sensitivity (Q10) of soil respiration along the altitudinal gradient remains unclear globally. This study explored spatial variability in SOM and Q10 and its regulatory mechanisms from a three-dimensional perspective. We collected SOM and Q10 datasets from published studies for 0–10 cm soil depth along elevational gradients of mountain ranges globally. We found that the SOM content in most mountains changed significantly with increasing altitude; unexpectedly, these changes with altitude significantly differed across different climate zones. Specifically, the SOM content increased with increasing altitude in tropical and subtropical regions, while it decreased with increasing altitude in temperate and plateau regions. Climate factors (mean annual temperature and mean annual precipitation) and soil properties (total N content, C/N ratio, and pH) together accounted for 83% of spatial variation in SOM with altitude. Q10 values, under both laboratory incubation and in situ studies, did not significantly correlate with altitude, and no apparent global patterns were detected (mean: 2.07); however, Q10 was regulated by variation in soil substrate quality along altitudinal gradients, to some extent. In the context of global climate change, this new information on SOM and Q10 in relation to the altitude at a global scale might enhance our understanding of soil C−climate feedbacks.
1 Introduction
Soil organic matter (SOM) is an important pool of carbon (C) in terrestrial ecosystems, being approximately 2 to 3-fold that of atmospheric and biotic C, respectively (Jackson et al., 2017). SOM supports many vital ecosystem services, from the provision of food and fiber, to regulating climate and water cycles, regenerating fertility, and supporting the immense biodiversity of soils (Lehmann and Kleber, 2015). CO2 emitted from SOM decomposition is the second-largest CO2 flux in the land–atmosphere interface and is influenced by various factors, including climate (Hawkes et al., 2017), temperature (Li et al., 2017a), moisture (Yan et al., 2018), substrate quality (Liu et al., 2021), substrate effectiveness (Li et al., 2022), and microbes (Karhu et al., 2014). Among these factors, temperature is the most important, regulating SOM decomposition (Davidson and Janssens, 2006), and thus, it is essential to understand SOM dynamics, especially with the background of global warming (Davidson and Janssens, 2006). It is reported that approximately 25% of the land surface of the Earth is covered by mountainous regions (Korner, 2007), are particularly vulnerable to climate warming as they combine a steep climatic gradient, and thus, are widely used to investigate soil C–climate feedbacks (Korner, 2007). Although many studies have investigated trends in C, nitrogen, and their stoichiometry along altitudinal gradients in individual mountain ranges, our understanding of changes in SOM along elevational gradients at the global scale is greatly limited. SOM stocks as a result of C input from primary productivity and output through microbial decomposition. Temperature and precipitation are major influences on vegetation productivity and important factors affecting SOM decomposition (Wang et al., 2016; Li et al., 2017a), which may also lead to inconsistent trends in SOM along the altitudinal gradient in different climatic zones around the globe, as changes in latitude and longitude also imply variability in temperature and precipitation (Tashi et al., 2016; Yin et al., 2021). Therefore, it is particularly important to investigate the global pattern in altitudinal SOM content and the factors influencing its decomposition, and this contributes to our understanding of the global C cycle.
The temperature sensitivity of SOM decomposition is often quantified using Q10, which is defined as the proportional increase in the CO2 emission rate for a 10°C increase in temperature, and the Q10 is considered to be a key parameter in depicting the magnitude and direction of soil C–climate feedbacks. Minor variation in Q10 can result in large differences in estimates of soil C dynamics (Todd-Brown et al., 2013). However, in most ecological models, researchers often consider Q10 as a fixed value to simplify them, rising uncertainty in C–climate model predictions (Jian et al., 2018). Therefore, it is important and urgent, to quantitatively analyze the spatial patterns (horizontal and elevational) of Q10 and its drivers to optimize terrestrial C cycle models (Jian et al., 2018). Spatial horizontal variation in Q10 has been widely demonstrated at regional and global scales (Liu et al., 2017; Li et al., 2020b; Li et al., 2020c). These studies have greatly advanced our current understanding of the response of SOM decomposition to temperature variations and underlying mechanisms at different scales. However, approximately 25% of the land surface of Earth is covered by mountains; thus, the three-dimensional zonality of terrestrial ecosystems is a common feature, globally (Sundqvist et al., 2013). Changes in altitude affect temperature and precipitation, similar to latitudinal variation in terrestrial ecosystems to some extent (Korner, 2007). However, the trend in the variation in Q10 with altitude at a global scale remains unclear, despite altitude potentially being an important source of spatial variability in Q10. Furthermore, studies on how Q10 varies with altitude have been sparse to date, and those that have been conducted have generally focused on a single mountain range in a given climate zone (Niklińska and Klimek, 2007; Zimmermann and Bird, 2012; Gutierrez-Giron et al., 2015; Wang et al., 2016; Li et al., 2017b). To our knowledge, there is no integration of relevant studies across multiple climate zones, which also hinders our understanding of Q10 variation at spatial scales. Therefore, exploring how Q10 responds to altitude changes can help us to more comprehensively understand the influencing factors and variability patterns of Q10, and thus provide a basis for building a more reasonable C-climate model, which is especially important in the context of global warming.
Accordingly, in this study, we collected data from 323 studies on the distribution of SOM along altitudinal gradients, and 18 laboratory studies and 36 in situ studies on Q10. The primary objectives of this study were to 1) explore variation in SOM content and Q10 along the altitudinal gradient at zonal and global scales and 2) elucidate the mechanism underlying this effect. The latitudinal pattern of SOM globally showed that terrestrial SOM levels increase progressively with latitude (Stockmann et al., 2015), due to the effects of vegetation and other environmental factors (Sundqvist et al., 2013). Similar to latitudinal patterns, changes in altitude are related to changes in climate factors, which, in turn, alter SOM (Sundqvist et al., 2013). Tropical and subtropical regions have favorable hydrothermal conditions and high vegetative productivity, which is highly favorable for soil microbial activity. Because microbes decompose SOM rapidly, SOM content is lower at lower altitudes in tropical and subtropical areas. However, temperature decreases with increasing altitude, limiting microbial activity; consequently, the rate of SOM decomposition decreases, and SOM from plants is more strongly sequestered in the soil in tropical and subtropical areas (Sundqvist et al., 2013). Therefore, SOM content increases with altitude. Nevertheless, in temperate and plateau regions, lower MAT and MAP limit soil microbial activity and inhibit the microbial decomposition of SOM, such that basal SOM values are relatively high in these regions. However, as the altitude increases, relatively poor hydrothermal conditions result in lower plant biomass and net primary production (Li et al., 2021a), especially in alpine regions (Sundqvist et al., 2013). Consequently, C return to soil is lowered, particularly when coupled with the slow rate of microbial decomposition of litter in nutrient-deficient, low-temperature, dry environments (Vitousek et al., 1994); thus, SOM gradually decreases with increasing altitude (Vitousek et al., 1994). Therefore, we hypothesized that it is difficult to establish a uniform association between SOM content and altitude on a global scale (Figures 1A,B); however, it is possible, to distinguish SOM in different climate zones to establish a potential association.
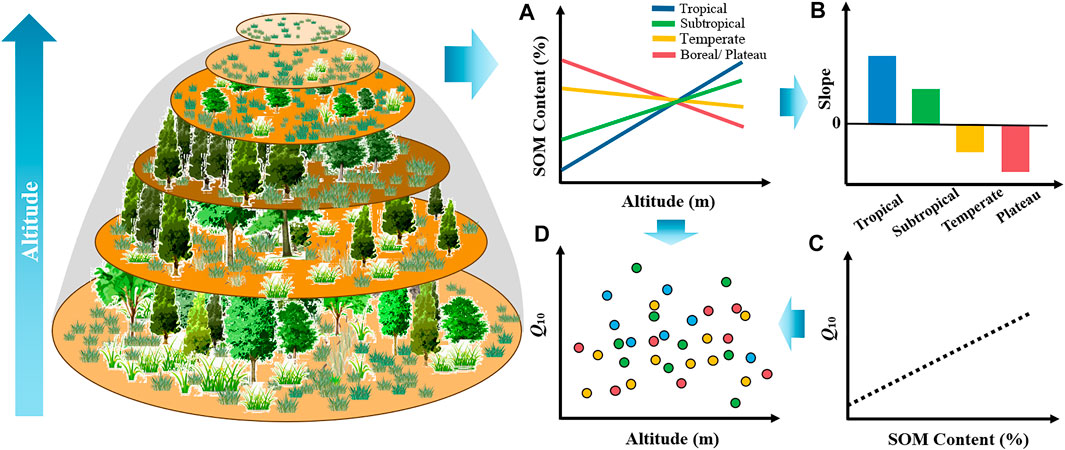
FIGURE 1. Hypothesized altitudinal variation of soil organic matter (SOM) content and Q10 values in different climatic zones. The left side represents a schematic diagram of the change of vegetation with increasing mountain altitude. (A) Trend of SOM content with altitude increases in different climatic zones; (B) slope of SOM content with increasing altitude in different climatic zones; (C) relationship between SOM content and Q10, where the black line shows the positive correlation between SOM and Q10; (D) variation in altitudinal Q10 with increasing altitude on a global scale.
Q10 is often considered as an intrinsic property of a given pool independent of the environmental conditions and this is how it is classically represented in models (Davidson and Janssens, 2006). Although Q10 is also influenced by environmental factors such as temperature and moisture, these environmental factors mainly affect Q10 by shaping microbial activity, traits (Li et al., 2021b), and substrate availability (Wang et al., 2016). Additionally, numerous studies have verified from different perspectives that substrate quality and availability control the responses of SOM decomposition to warming (Davidson and Janssens, 2006; Conant et al., 2008a; Conant et al., 2008b; Davidson et al., 2012; Wang et al., 2018; Liu et al., 2021), and the Arrhenius kinetics and Michelis–Menten kinetics were used to describe the effect of substrate quality and availability on Q10. Therefore, we suggest that Q10 along the altitudinal gradient is also controlled by the soil substrate quality (e.g., SOC, C/N, and pH). As mentioned earlier, we inferred that there is no uniform relationship between SOM and altitude on a global scale. Based on the relationship between soil substrate quality/quantity and Q10 (Figure 1C), we, therefore, hypothesized that the relationship between Q10 and altitude may be different in different climatic zones and there is no uniform relationship on a global scale (Figure 1D).
2 Material and methods
2.1 Literature survey and data extraction
We collected peer-reviewed articles on SOM and Q10 from the Web of Science (www.webofknowledge.com) and China National Knowledge Infrastructure (http://epub.cnki.net) from 1984 to 2020. In total, 323 articles describing the distribution of SOM along altitudinal gradients were obtained; especially, there were 20, 117, 145, and 41 articles for tropical, subtropical, temperate, and plateau regions, respectively (Supplementary Table S2). SOM content was selected for 10-cm depth soil. The original data were first extracted from reported tables or graphs using GetData Graph Digitizer (http://getdata-graph-digitizer.com). Simultaneously, other auxiliary data, such as geographic information (i.e., longitude, latitude, and altitude), climate data (i.e., mean annual temperature [MAT] and mean annual precipitation [MAP]), and soil chemical properties (i.e., SOM, SOC [SOM and SOC can be interconverted with a factor of 1.724], soil total N [TN], and C: N ratio [C/N]) were obtained from the published literature. Unfortunately, studies conducted in boreal regions were not found. The Qing–Tibet Plateau is called the “third pole of the world,” and has certain similarities with the boreal zone in terms of climate, especially regarding temperature. Therefore, the plateau was taken as being representative of the boreal zone in this study.
To avoid the influence of complex ecological factors in the field, we collected laboratory studies that used optimal conditions (without temperature and moisture limitations) in incubation experiments; that is, at an incubation temperature of 10–30°C and 50–70% field water holding capacity (Liu et al., 2017). The Q10 value was selected for 10-cm soil depth because this surface layer has the highest respiration activity of all soil layers (Liu et al., 2017). Most Q10 values were directly obtained from published studies. Only some Q10 values were indirectly obtained from respiration data using Eq. 1:
where SR is the measured soil respiration rate, T is the measured soil temperature, and coefficients α and β are fitted parameters. The Q10 value was determined using Eq. 2:
A total of 18 studies on the distribution of Q10 along altitudinal gradients were collected, of which, 1, 8, and 9 studies were conducted in tropical, subtropical, and temperate regions, respectively (Supplementary Table S3). Each study included at least three altitudinal levels. We also selected in situ studies on Q10 along altitude gradients. In total, 169 datasets were derived from 36 published studies, of which 3, 5, 18, and 10 studies were from tropical, subtropical, temperate, and plateau regions, respectively (Supplementary Table S4).
2.2 Data assembly
In total, 2,370 SOM datasets were obtained from 323 published studies with 20, 117, 145, and 41 studies for tropical, subtropical, temperate and plateau regions, corresponding to 185, 778, 1,079, and 328 datasets, respectively. Of these, only 1,909 datasets from 246 published studies contained SOM, geographical information, climate information, and soil chemical properties, with 11, 101, 112, and 22 studies for tropical, subtropical, temperate, and plateau regions, corresponding to 96, 706, 859, and 248 datasets, respectively. A total of 83 datasets from 18 published studies were selected for the Q10 analysis, of which 3, 28, and 52 datasets were from tropical, subtropical, and temperate regions, respectively. These studies contained information on climate and soil properties. Furthermore, 169 datasets of Q10 data were obtained from 36 published studies, including 12, 19, 92, and 46 datasets from tropical, subtropical, temperate, and plateau regions, respectively. The geographical distribution of the sites where SOM and Q10 were collected is shown in Figure 2.
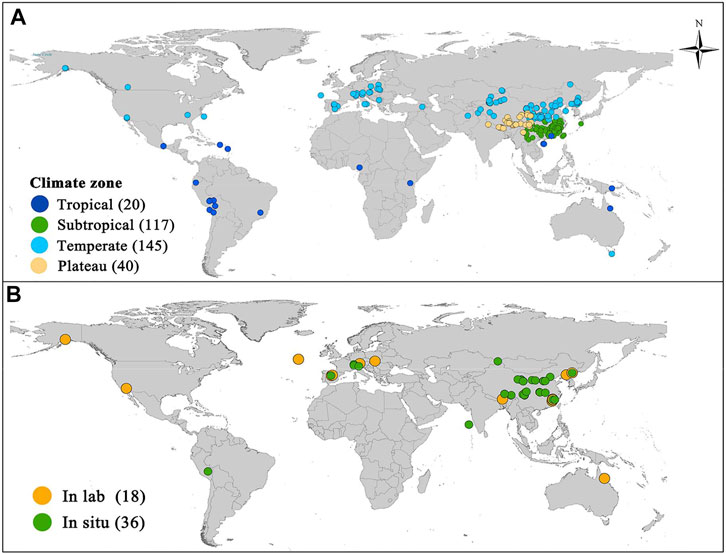
FIGURE 2. Geographical distributions of the study sites. The numbers in parentheses indicate observations of soil organic matter (A) and Q10 (B) values of soil respiration for each climatic zone. Some sites are very close to each other, resulting in corresponding symbols overlapping to some extent.
2.3 Statistical analyses
We analyzed the relationship between SOM and altitude using 2,370 SOM datasets. However, as mentioned earlier, because only some of the SOM datasets contained both SOM data, geographic information, climatic information, and soil properties, we used only the 1,909 datasets containing such information in our analysis of the factors influencing SOM. The Kolmogorov–Smirnov test was used to evaluate data normality. When the data did not conform to a normal distribution, a logarithmic transformation or normalization process was followed, so that they better resembled a normal distribution. Differences in Q10 across climatic regions were analyzed using one way analysis of variance (ANOVA), with the least significant difference (LSD) for multiple comparisons. Regression analysis was performed to evaluate the association between SOM and Q10 with altitude, soil properties, and climate factors. We used a structural equation model (SEM) to quantify the relative importance of direct and indirect factors predicting SOM and Q10 values, respectively. Before implementing the SEM, we first normalized and standardized each variable (i.e., SOM, Q10, TN, C/N ratio, and pH) using min-max normalization transformation (actual value–min)/(max–min), which was performed on the entire dataset and for all climatic zones (Li et al., 2020c). To conduct the SEM, we first established a priori model based on our existing knowledge of the direct and indirect effects of climate (i.e., MAT and MAP) and soil properties (i.e., SOM, TN, C/N, and pH) on SOM and Q10 values. Through the stepwise exclusion of nonsignificant paths in the initial model, we selected a final model that best fitted our data. The adequacy of the model was assessed using the chi-square (χ2) test, goodness-of-fit (GFI) index, and root mean squared error of approximation (RMSEA) index (Liu et al., 2017; Li et al., 2020c). Favorable model fits were ascertained from no significant difference in the χ2 test (p > 0.05), high GFI (>0.9), and low RMSEA (<0.05).
Statistical analyses and correlations were performed using SPSS Statistics 13 (IBM, Chicago, IL, United States). SEM analysis was conducted using AMOS 21.0 software (IBM, Chicago, IL, United States). Graphs were plotted using Origin software (Version 8.5; Northampton, MA, United States) and ESRI ArcGIS software (Version 10.2; Redlands, CA, United States). A statistical probability of p < 0.05 determined significance.
3 Results
3.1 Variation in SOM with increasing altitude in different climatic regions globally
Within the SOM datasets we collected, 61.8% of these studies observed a significant relationship between SOM content and altitude (Supplementary Table S1); however, we observed no significant associations between altitude and SOM content on a global scale (p > 0.05; Figure 3E). Changes in SOM with altitude were significant among different climate zones. Specifically, SOM content increased with altitude in tropical (R2 = 0.38, p < 0.001; Figure 3A) and subtropical regions (R2 = 0.15, p < 0.001; Figure 3B), but decreased with altitude in temperate (R2 = 0.03, p < 0.001; Figure 3C) and plateau regions (R2 = 0.08, p < 0.001; Figure 3D). Moreover, on comparing the slope of SOM content with changes in altitude for different climate zones, the slope displayed a decreasing (positive to negative) trend from tropical to plateau regions (Figure 3F).
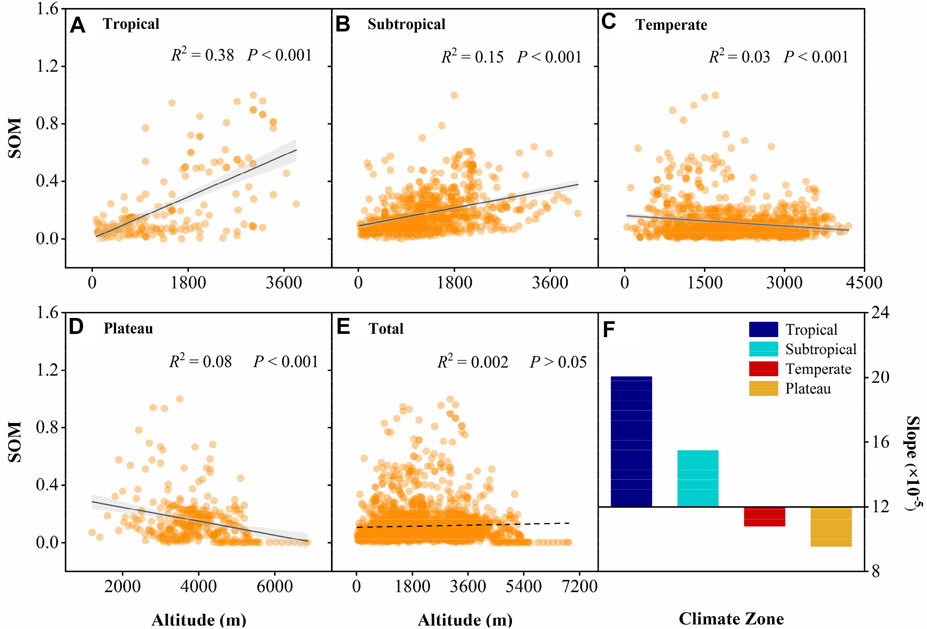
FIGURE 3. Relationship between soil organic matter content and altitude across climatic zones. (A–E) Trends in SOM content with altitude in different climatic zones, respectively. (F) Slopes of SOM content in comparison to altitude in different climatic zones. Tropical: n = 185, subtropical: n = 778, temperate: n = 1,079, plateau: n = 328, total: 2,370; SOM data in the figure were normalized by min-max normalization. Shaded areas indicate the 95% confidence interval.
3.2 Variation in Q10 with increasing altitude in different climatic regions globally
For the laboratory studies, Q10 values changed from 1.37 to 3.15 (mean 2.07) with an altitudinal gradient. The mean values were 2.02, 1.97, and 2.13 for tropical, subtropical, and temperate zones, respectively, and were not significantly different among zones (Supplementary Figure S1). We did not observe a significant relationship between Q10 values and altitude for the laboratory studies, either in different climatic zones or overall (p > 0.05; Figure 4). For in situ studies, Q10 values changed from 0.90 to 5.74 (mean 2.76) with an altitudinal gradient (Supplementary Figure S2A). We also did not observe a significant relationship between Q10 values and altitude in in situ studies on a global scale (p > 0.05; Supplementary Figure S2B), but the Q10 in different climate zones was significantly correlated with altitude except for tropical regions (p < 0.05; Supplementary Figures S2C–F) and was similar to the trend obtained for SOM content with altitude in corresponding climatic zones.
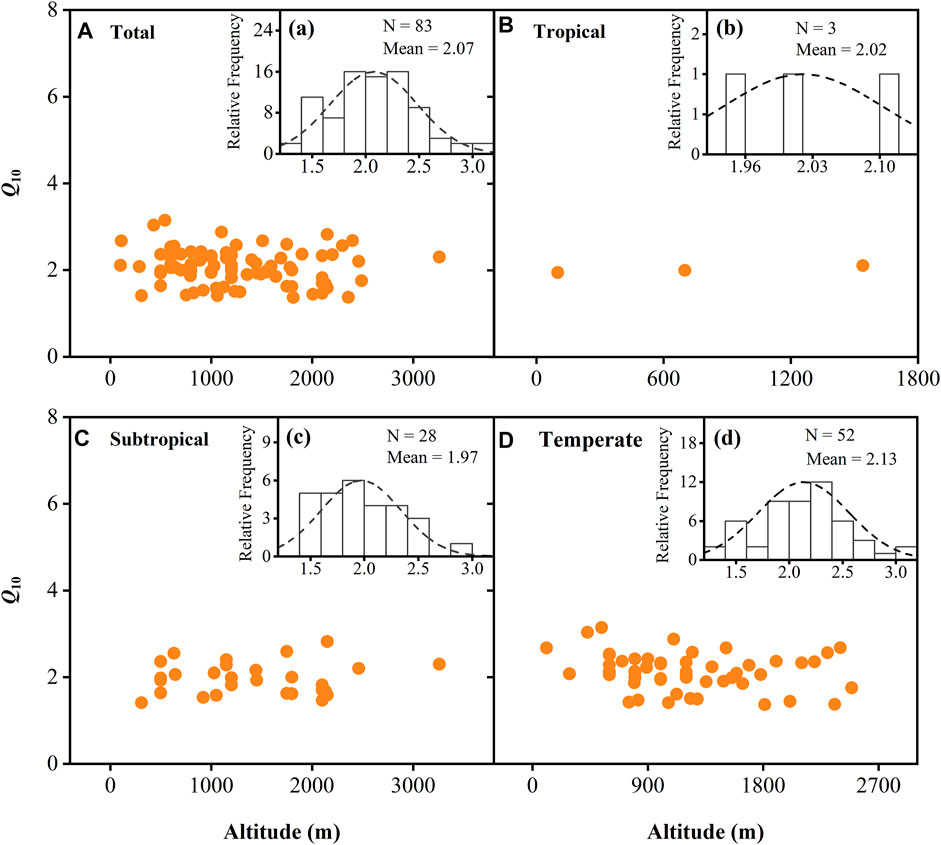
FIGURE 4. Variation in temperature sensitivity (Q10) of soil organic matter decomposition with altitude in different climatic zones. (A) Trends in Q10 values with altitude on a global scale; (B–D) Trends in Q10 content with altitude in tropical (B), subtropical (C), and temperate regions (D), respectively. (A–D) Frequency distribution of Q10 in the corresponding regions, respectively.
3.3 Drivers of SOM and Q10 variation along the altitudinal gradient
SOM content was significantly influenced by TN, with a clear linear increase in SOM with increasing TN (R2 = 0.65, p < 0.001; Supplementary Figure S3A). Moreover, SOM content significantly increased with increasing C/N (R2 = 0.08, p < 0.001; Supplementary Figure S3B) and MAP (R2 = 0.03, p < 0.001; Supplementary Figure S3E), but significantly decreased with falling pH (R2 = 0.07, p < 0.001; Supplementary Figure S3C); however, the associations between SOM and MAT were not significant (p > 0.05; Supplementary Figure S3D). SEM analysis revealed a strong association between climate and soil properties. Among all variables, MAT, TN, C/N, and pH had a direct, significant association with the SOM content, while MAP indirectly influenced spatial variation in SOM. These five variables together accounted for 83% of SOM variation (Figure 6A). Furthermore, TN had the most marked effect, followed by C/N and pH, as indicated by standardized total effects in predicting global SOM values (Figure 6B).
Q10 was significantly and negatively associated with the MAT (R2 = 0.08, p < 0.05; Figure 5E); however, the association between Q10 and MAP was not significant (p > 0.05; Figure 5F). Moreover, Q10 values significantly increased with increasing SOM content (R2 = 0.13, p < 0.001; Figure 5A), TN (R2 = 0.06, p < 0.05; Figure 5B), and C/N (R2 = 0.08, p < 0.01; Figure 5C). However, Q10 values were significantly affected by soil pH and linearly decreased with increasing pH (R2 = 0.05, p < 0.05; Figure 5D). SEM analysis revealed that soil properties (SOM, C/N ratio, and pH) could predict Q10 values, all of which had a significant direct effect on Q10 variability (Figure 6C). Climatic factors (including MAT and MAP) did not significantly and directly influence Q10; rather, they influenced Q10 indirectly by influencing soil properties. All variables accounted for 27% of Q10 variation; SOM was the primary influencing factor, accounting for 31% of the total variation, followed by pH and MAT (Figure 6D).
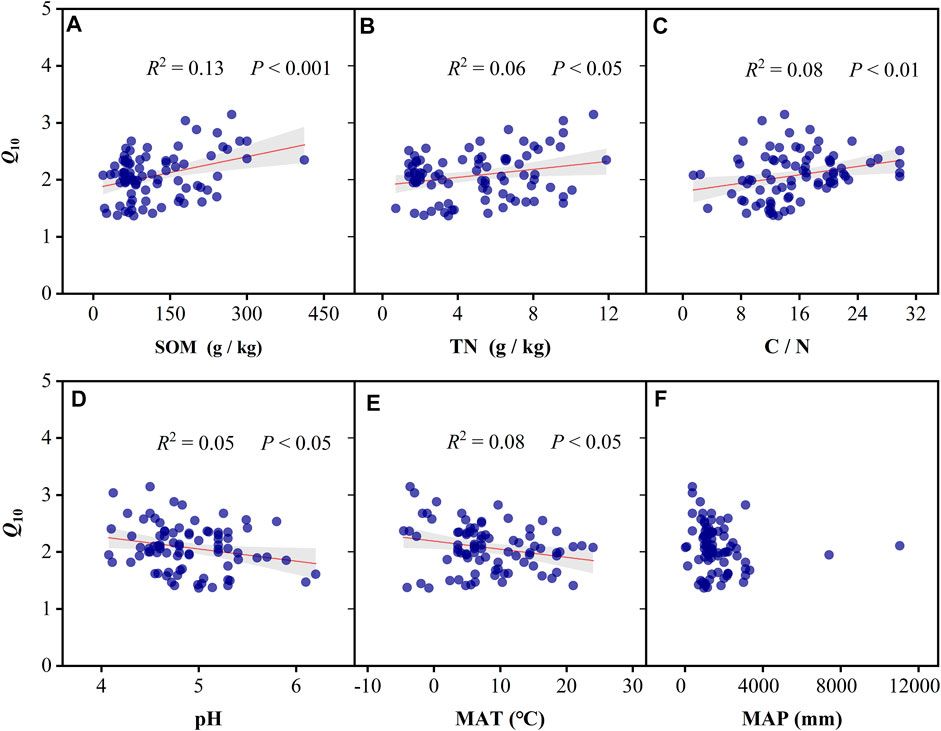
FIGURE 5. Q10 value of soil respiration in relation to soil properties and climatic factors on a global scale (n = 83). (A–F) Relationships between Q10 and SOM, TN, C/N, pH, MAT, and MAP, respectively. SOM, soil organic matter; TN, total nitrogen; C/N, ratio of carbon and nitrogen; MAT, mean annual temperature; MAP, mean annual precipitation. Shaded areas indicate the 95% confidence interval.
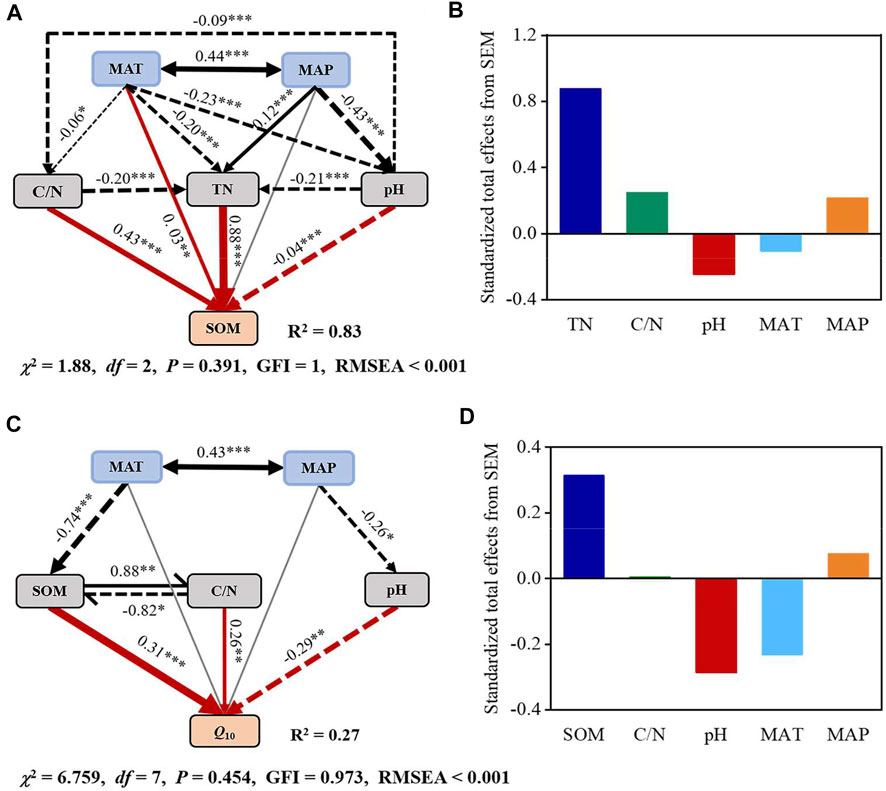
FIGURE 6. Structural equation model (SEM) on the direct and indirect effects of SOM (A) and Q10 (C) and standardized total effect (direct plus indirect effects) derived from SEM (B,D) on a global scale. Solid and dashed lines indicate positive and negative effects, respectively; the gray line indicates that the relationship is not significant at the 0.05 level; line thickness represents the magnitude of the path coefficient. The numbers adjacent to arrows are standardized path coefficients, indicating the effect size of the relationship. MAT, mean annual temperature; MAP, mean annual precipitation; SOM, soil organic carbon; TN, total nitrogen; C/N, ratio of carbon and nitrogen. Goodness-of-fit statistics are shown in the model. *p < 0.05, **p < 0.01, and ***p < 0.001.
4 Discussion
4.1 Trends in SOM with altitude depend on climatic zones
Soil C sequestration is the uptake of atmospheric CO2 by terrestrial ecosystems through plant photosynthesis and its conversion and long-term storage in the soil. However, differences in soil type, above-ground vegetation, and climate result in significant differences in soil C storage capacity across regions (Stockmann et al., 2015). Spatial patterns in SOM at different latitudes globally revealed that terrestrial SOM levels increase progressively with latitude and are affected by vegetation, temperature, and precipitation (Minasny et al., 2017). Similarly, changes in altitude are associated with changing climate factors, which, in turn, alter SOM. This study showed that changes in SOM with altitude were not consistent across different climatic zones and this is supported by a previous study (Yin et al., 2021). For instance, SOM content increased with increasing altitude in tropical and subtropical regions but decreased with increasing altitude in temperate and plateau regions. This finding contrasts with the conventional view that SOM content increases with altitude, mainly because changes in altitude also affect plant productivity and carbon inputs to the soil (Davidson et al., 2000), leading to different trends in SOM content with altitude in different climatic zones.
Suitable hydrothermal conditions at low elevations in the tropics and subtropics facilitate high net primary productivity in plants and favors microbial activity; consequently, microbial decomposition of SOM is faster and does not favor C sequestration, resulting in lower SOM content (Schimel et al., 1994; Sundqvist et al., 2013). However, as elevation increases, lower temperatures limit microbial activity, with the rate of microbial decomposition of SOM slowing and it being possible for organic matter from plants to be more strongly sequestered in soil; thus, SOM content increases with altitude (Dai and Huang, 2006; Sundqvist et al., 2013). Nevertheless, in temperate and plateau regions, lower MAT limits soil microbial activity and slows the decomposition of SOM, such that the basal SOM contents are relatively high in these regions (Dai and Huang, 2006). However, as altitude increases, relatively poor hydrothermal conditions result in lower plant biomass and productivity (Vitousek et al., 1994), especially in alpine regions. This phenomenon coupled with the slow rate of microbial decomposition of litter in nutrient-deficient soil at low temperature, results in lower quantities of organic matter returning to the soil. Moreover, there also is characterized by low diversity of the plant community and a harsh environment in high-altitude sites (Feng et al., 2021), the transfer strategy of microbes (e.g., r-/K-trait) may also play a key role (Dai et al., 2021; Feng et al., 2021). Thus, SOM content gradually decreases with increasing altitude (Davidson et al., 2000). Therefore, it is difficult to establish a uniform association between SOM content and altitude on a global scale; however, it is possible to identify differences in SOM among different climate zones to establish associations.
Soil properties (TN, C/N, and pH) and climate factors (MAT and MAP) explained the variation in SOM in the current study (Figure 6; Supplementary Figure S3). A significant correlation between soil N content and SOM content was previously reported (Spain, 1990). First, N-containing compounds and humus account for a large part of SOM, with N elements including amino acids and polypeptides being the primary components of humus (Spain, 1990); thus, soil TN reflects the size of SOM, to a large extent. Soil C/N tends to characterize the coupling of SOM and TN and is an important indicator of the potential of soil N mineralization and whether SOM decomposition is limited by soil N (Hu et al., 2001). In general, when the C/N ratio is lower, N is more available to microbes, making it more favorable for SOM decomposition. Conversely, the microbial function during SOM decomposition is limited by soil N (Hu et al., 2001), leading to the accumulation of SOM. Therefore, soil C/N has a significant positive effect on SOM. The soil pH affects the turnover and accumulation of SOM by influencing the soil microbial activity and community structure (Motavalli et al., 1995; Staddon et al., 1998).
Climate, especially temperature and precipitation, largely determines vegetation cover, quantity and quality of organic residues entering the soil, and the rate of SOM mineralization and litter decomposition; consequently, it also determines the turnover of SOM (Davidson et al., 2000). Many studies have reported a significant negative correlation between the MAT and SOM content (Ganuza and Almendros, 2003; Lemenih and Itanna, 2004). However, this study did not find any significant association between the MAT and SOM on a global scale. This phenomenon might be explained by the fact that lower temperatures imply lowered C input, despite favoring soil C storage (Yin et al., 2021). Therefore, the relationship between the MAT and SOM content over the altitudinal gradient depends on the climatic region in which it is located. Specifically, attention still needs to be paid to the effect of temperature on the balance of organic matter input and decomposition in the region where it is located. Moreover, these results indicate a positive correlation between SOM content and MAP, supporting previous studies (Dai and Huang, 2006; Homann et al., 2007). Generally, vegetation grows rapidly with higher net primary production in humid and warmer climate zones; consequently, there is a higher input litter to the soil. In addition, higher precipitation favors the decay and decomposition of falling litter, accelerating the input of external C to the soil, resulting in higher SOM content (Dai and Huang, 2006; Sundqvist et al., 2013).
4.2 No clear variation in Q10 along the altitudinal gradient at different scales
This study showed that Q10 ranged from 1.37–3.15 (mean 2.07) along the altitudinal gradient on a global scale, with no significant differences among climate zones. Some studies previously reported that Q10 increases gradually with altitude (Zimmermann and Bird, 2012; Wang et al., 2013); however, other studies reported that Q10 values show no discernible trend with altitude (Niklińska and Klimek, 2007; Xu et al., 2010; Xu et al., 2014; Wang et al., 2016). The contrasting results of these previous studies may be due to the fact that these studies focused only on separate mountain ranges in different climatic regions. Our study detected no consistent variation in Q10 along the altitudinal gradient on the global scale (Figure 4A). First, belowground ecological processes in different climatic zones were influenced by different climate factors (Colman and Schimel, 2013). Q10 is influenced by various factors such as climate (Li et al., 2020b) and soil properties (Liu et al., 2017), which further contribute to the complexity of Q10. Second, this study showed that SOM is the primary factor influencing spatial variation in Q10 along altitude (Figures 5, 6); however, trends in SOM content with altitude were inconsistent across climate zones (Figure 3); thus, environmental and soil properties jointly lead to the uncertainty in Q10 with altitude at a global scale.
Moreover, our evaluation of in situ studies also supported the uncertainty in Q10 with altitude at a global scale (Supplementary Figure S2B). Specifically, Q10 increased with altitude in subtropical regions and decreased with altitude in temperate and plateau regions. This finding was consistent with the trend obtained for SOM content with altitude. Although CO2 released from SOM decomposition is only a partial source of CO2 from soil respiration, microbial respiration displays a stronger response to changing temperature than root respiration (Lin et al., 2010). Thus, this phenomenon might also reflect uncertainty in the global-scale variation of Q10 along the altitudinal gradient, to some extent and further corroborates our finding that variation in Q10 is regulated by the substrate quality of the soil.
The SEM analysis revealed that spatial variation in Q10 with an altitude gradient was directly influenced by soil properties (i.e., SOM, C/N, and soil pH) and indirectly influenced by climate factors (MAT and MAP) (Figure 6C). Together, these factors accounted for 27% of spatial variation in Q10. Q10 decreased with increasing MAT (Figure 5E), supporting previous studies (Zheng et al., 2009; Xu et al., 2015; Liu et al., 2017; Li et al., 2020a; Li et al., 2020b; Li et al., 2020c). The negative relationship between Q10 and MAT may be explained by the relative increase of enzyme activity diminishing as temperature increases (Schipper et al., 2014). Moreover, the macromolecular rate theory indicates that the net increase in soil respiration is relatively lower at higher temperatures (Schipper et al., 2014), causing Q10 to decline with increasing temperature. Additionally, temperature effects on soil substrate and microbial properties (e.g., K-/r-traits) may also have an impact on Q10 (Liu et al., 2017; Li et al., 2021b). The current study showed that areas with higher SOM and C/N also had higher Q10 values (Figure 5). This phenomenon was attributed to SOM being the primary substrate for soil respiration, with the quantity/quality of substrate strongly influencing the composition of the microbial community (Knorr et al., 2005; Tan et al., 2020). Furthermore, soils with higher SOM content have higher decomposable C content and microbial biomass (Colman and Schimel, 2013). Therefore, ecosystems with higher SOM content generally have a greater potential for CO2 efflux and higher Q10 (Zheng et al., 2009; Li et al., 2022).
N is an important factor for the life-sustaining activities of soil microorganisms and it is a limiting factor for microbial SOM decomposition (Hu et al., 2001). Consequently, it strongly influences the activity of soil microbes. Thus, higher N content enhances the decomposition rate of SOM. Moreover, soil C and N content was closely coupled, accumulating synergistically (Gärdenäs et al., 2011). Accordingly, soil N content also revealed a significant positive correlation with Q10 (Figure 5B). Overall, these findings advance our current understanding of the regional variation in Q10 along altitudinal gradients and how it may be driven by global warming. Since trends in SOM and Q10 with altitude vary across different climate zones, future studies should incorporate this variation.
4.3 Limitations and outlook
This study is the first to demonstrate variation in SOM content and Q10 along the altitudinal gradients across different climate zones at a global scale and the mechanism underlying this effect; however, some limitations existed. First, few studies have been conducted on soil respiration C dynamics along altitudinal gradients, with existing studies primarily focusing on temperate regions; thus, other regions require more focused studies, especially in the tropical and boreal zones. Second, the existing literature provides insufficient data on the factors influencing Q10, with many important factors (including soil texture and soil microbes) not being accounted for (Colman and Schimel, 2013). However, changes in altitude generate variations in biotic and abiotic factors over a smaller latitudinal and longitudinal range. This phenomenon made it difficult to obtain missing data from the International Soil Reference and Information Center (ISRIC) database. As a result, there was insufficient data on Q10 variation in the SEM analysis. Therefore, these effects require more comprehensive evaluation in future studies, by screening similar soil properties to improve the predictive capability of geographic patterns in soil C–climate feedback. Furthermore, because of the complexity of the field environment, we focused on Q10 experiments in a laboratory setting (i.e., heterotrophic respiration). However, autotrophic respiration of plants is an important part of soil respiration, with changes in temperature impacting this. Moreover, the contributions of autotrophic and heterotrophic respiration to total soil respiration may vary with the climate zone and ecosystem, and the underlying regulatory factors may also differ, which should be considered in future studies.
5 Conclusion
This global synthesis is the first to provide information on altitudinal patterns and regulatory mechanisms of SOM and Q10, through a comprehensive assessment at the global and regional levels. Unexpectedly, altitude-dependent trends of SOM and Q10 differed in different climate zones. However, a positive correlation between SOM and Q10 and C/N–Q10 indicates that SOM content is the primary predictor of Q10 for elevational soil respiration on a global scale. Furthermore, based on the negative relationship between the MAT and Q10, this suggests a greater potential for soil C loss in colder regions and C-stock-rich soil in the north, in contrast to warmer regions, in response to global warming. Overall, variation in SOM and Q10 along altitudinal gradients differed across climate zones, advancing our understanding of geographical variations and regulatory mechanisms of Q10 at local to global spatial scales. Our findings could be applied to improve the predictive accuracy of soil C−climate feedback models on a global three-dimensional scale.
Data availability statement
The original contributions presented in the study are included in the article/Supplementary Material; further inquiries can be directed to the corresponding authors.
Author contributions
NH and CX designed this study; CL collected the dataset, performed statistical analyses, prepared figures and tables, and drafted the manuscript with inputs from NH, CX, and ML; NH and LX reviewed and revised the manuscript and all authors contributed to revisions.
Funding
This work was supported by the Natural Science Foundation of China (31988102, 32171544, and 42141004).
Conflict of interest
The authors declare that the research was conducted in the absence of any commercial or financial relationships that could be construed as a potential conflict of interest.
Publisher’s note
All claims expressed in this article are solely those of the authors and do not necessarily represent those of their affiliated organizations, or those of the publisher, the editors, and the reviewers. Any product that may be evaluated in this article, or claim that may be made by its manufacturer, is not guaranteed or endorsed by the publisher.
Supplementary material
The Supplementary Material for this article can be found online at: https://www.frontiersin.org/articles/10.3389/fenvs.2022.959292/full#supplementary-material
References
Colman, B. P., and Schimel, J. P. (2013). Drivers of microbial respiration and net N mineralization at the continental scale. Soil Biol. Biochem. 60, 65–76. doi:10.1016/j.soilbio.2013.01.003
Conant, R. T., Drijber, R. A., Haddix, M. L., Parton, W. J., Paul, E. A., Plante, A. F., et al. (2008a). Sensitivity of organic matter decomposition to warming varies with its quality. Glob. Chang. Biol. 14, 868–877. doi:10.1111/j.1365-2486.2008.01541.x
Conant, R. T., Steinweg, J. M., Haddix, M. L., Paul, E. A., Plante, A. F., and Six, J. (2008b). Experimental warming shows that decomposition temperature sensitivity increases with soil organic matter recalcitrance. Ecology 89, 2384–2391. doi:10.1890/08-0137.1
Dai, W. H., and Huang, Y. (2006). Relation of soil organic matter concentration to climate and altitude in zonal soils of China. CATENA 65, 87–94. doi:10.1016/j.catena.2005.10.006
Dai, Z., Zang, H., Chen, J., Fu, Y., Wang, X., Liu, H., et al. (2021). Metagenomic insights into soil microbial communities involved in carbon cycling along an elevation climosequences. Environ. Microbiol. 23, 4631–4645. doi:10.1111/1462-2920.15655
Davidson, E. A., and Janssens, I. A. (2006). Temperature sensitivity of soil carbon decomposition and feedbacks to climate change. Nature 440, 165–173. doi:10.1038/nature04514
Davidson, E. A., Samanta, S., Caramori, S. S., and Savage, K. (2012). The Dual Arrhenius and Michaelis-Menten kinetics model for decomposition of soil organic matter at hourly to seasonal time scales. Glob. Chang. Biol. 18, 371–384. doi:10.1111/j.1365-2486.2011.02546.x
Davidson, E. A., Trumbore, S. E., and Amundson, R. (2000). Soil warming and organic carbon content. Nature 408, 789–790. doi:10.1038/35048672
Feng, J., Zeng, X.-M., Zhang, Q., Zhou, X.-Q., Liu, Y.-R., and Huang, Q. (2021). Soil microbial trait-based strategies drive metabolic efficiency along an altitude gradient. ISME Commun. 1, 71. doi:10.1038/s43705-021-00076-2
Gärdenäs, A., Ågren, G., and Nilsson, I. (2011). Preface. Soil Biol. Biochem. 43, 701–717. doi:10.1016/j.soilbio.2011.01.025
Ganuza, A., and Almendros, G. (2003). Organic carbon storage in soils of the Basque country (Spain): The effect of climate, vegetation type and edaphic variables. Biol. Fertil. Soils 37, 154–162. doi:10.1007/s00374-003-0579-4
Gutierrez-Giron, A., Diaz-Pines, E., Rubio, A., and Gavilan, R. G. (2015). Both altitude and vegetation affect temperature sensitivity of soil organic matter decomposition in Mediterranean high mountain soils. Geoderma 237, 1–8. doi:10.1016/j.geoderma.2014.08.005
Hawkes, C. V., Waring, B. G., Rocca, J. D., and Kivlin, S. N. (2017). Historical climate controls soil respiration responses to current soil moisture. Proc. Natl. Acad. Sci. U. S. A. 114, 6322–6327. doi:10.1073/pnas.1620811114
Homann, P. S., Kapchinske, J. S., and Boyce, A. (2007). Relations of mineral-soil C and N to climate and texture: Regional differences within the conterminous USA. Biogeochemistry 85, 303–316. doi:10.1007/s10533-007-9139-6
Hu, S., Chapin, F. S., Firestone, M. K., Field, C. B., and Chiariello, N. R. (2001). Nitrogen limitation of microbial decomposition in a grassland under elevated CO2. Nature 409, 188–191. doi:10.1038/35051576
Jackson, R. B., Lajtha, K., Crow, S. E., Hugelius, G., Kramer, M. G., and Piñeiro, G. (2017). The Ecology of soil carbon: Pools, vulnerabilities, and biotic and abiotic controls. Annu. Rev. Ecol. Evol. Syst. 48, 419–445. doi:10.1146/annurev-ecolsys-112414-054234
Jian, J., Steele, M. K., Thomas, R. Q., Day, S. D., and Hodges, S. C. (2018). Constraining estimates of global soil respiration by quantifying sources of variability. Glob. Chang. Biol. 24, 4143–4159. doi:10.1111/gcb.14301
Karhu, K., Auffret, M. D., Dungait, J. A. J., Hopkins, D. W., Prosser, J. I., Singh, B. K., et al. (2014). Temperature sensitivity of soil respiration rates enhanced by microbial community response. Nature 513, 81–84. doi:10.1038/nature13604
Knorr, W., Prentice, I. C., House, J. I., and Holland, E. A. (2005). Long-term sensitivity of soil carbon turnover to warming. Nature 433, 298–301. doi:10.1038/nature03226
Korner, C. (2007). The use of 'altitude' in ecological research. Trends Ecol. Evol. 22, 569–574. doi:10.1016/j.tree.2007.09.006
Lehmann, J., and Kleber, M. (2015). The contentious nature of soil organic matter. Nature 528, 60–68. doi:10.1038/nature16069
Lemenih, M., and Itanna, F. (2004). Soil carbon stocks and turnovers in various vegetation types and arable lands along an elevation gradient in southern Ethiopia. Geoderma 123, 177–188. doi:10.1016/j.geoderma.2004.02.004
Li, C., Liu, L., Zheng, L., Yu, Y., Mushinski, R. M., Zhou, Y., et al. (2021a). Greater soil water and nitrogen availability increase C : N ratios of root exudates in a temperate steppe. Soil Biol. Biochem. 161, 108384. doi:10.1016/j.soilbio.2021.108384
Li, C., Xiao, C. W., Guenet, B., Li, M. X., Xu, L., and He, N. P. (2022). Short-term effects of labile organic C addition on soil microbial response to temperature in a temperate steppe. Soil Biol. Biochem. 167, 108589. doi:10.1016/j.soilbio.2022.108589
Li, H., Yang, S., Semenov, M. V., Yao, F., Ye, J., Bu, R., et al. (2021b). Temperature sensitivity of SOM decomposition is linked with a K-selected microbial community. Glob. Chang. Biol. 27, 2763–2779. doi:10.1111/gcb.15593
Li, J., Baath, E., Pei, J., Fang, C., and Nie, M. (2020a). Temperature adaptation of soil microbial respiration in alpine, boreal and tropical soils: An application of the square root (Ratkowsky) model. Glob. Chang. Biol. 27, 1281–1292. doi:10.1111/gcb.15476
Li, J., He, N. P., Xu, L., Chai, H., Liu, Y., Wang, D. L., et al. (2017a). Asymmetric responses of soil heterotrophic respiration to rising and decreasing temperatures. Soil Biol. Biochem. 106, 18–27. doi:10.1016/j.soilbio.2016.12.002
Li, J., Nie, M., Pendall, E., Reich, P. B., Pei, J., Noh, N. J., et al. (2020b). Biogeographic variation in temperature sensitivity of decomposition in forest soils. Glob. Chang. Biol. 26, 1873–1885. doi:10.1111/gcb.14838
Li, J., Pei, J. M., Pendall, E., Fang, C. M., and Nie, M. (2020c). Spatial heterogeneity of temperature sensitivity of soil respiration: A global analysis of field observations. Soil Biol. Biochem. 141, 107675. doi:10.1016/j.soilbio.2019.107675
Li, Q., Cheng, X. L., Luo, Y. Q., Xu, Z. K., Xu, L., Ruan, H. H., et al. (2017b). Consistent temperature sensitivity of labile soil organic carbon mineralization along an elevation gradient in the Wuyi Mountains, China. Appl. Soil Ecol. 117, 32–37. doi:10.1016/j.apsoil.2017.04.018
Lin, G., Ehleringer, J. R., Rygiewicz, P. L. T., Johnson, M. G., and Tingey, D. T. (2010). Elevated CO2 and temperature impacts on different components of soil CO2 efflux in Douglas-fir terracosms. Glob. Chang. Biol. 5, 157–168. doi:10.1046/j.1365-2486.1999.00211.x
Liu, Y., He, N., Zhu, J., Xu, L., Yu, G., Niu, S., et al. (2017). Regional variation in the temperature sensitivity of soil organic matter decomposition in China's forests and grasslands. Glob. Chang. Biol. 23, 3393–3402. doi:10.1111/gcb.13613
Liu, Y., Xu, L., Zheng, S., Chen, Z., Cao, Y. Q., Wen, X. F., et al. (2021). Temperature sensitivity of soil microbial respiration in soils with lower substrate availability is enhanced more by labile carbon input. Soil Biol. Biochem. 154, 108148. doi:10.1016/j.soilbio.2021.108148
Minasny, B., Malone, B. P., McBratney, A. B., Angers, D. A., Arrouays, D., Chambers, A., et al. (2017). Soil carbon 4 per mille. Geoderma 292, 59–86. doi:10.1016/j.geoderma.2017.01.002
Motavalli, P. P., Palm, C. A., Parton, W. J., Elliott, E. T., and Frey, S. D., (1995). Soil pH and organic C dynamics in tropical forest soils: Evidence from laboratory and simulation studies. Soil Biol. Biochem. 27, 1589–1599. doi:10.1016/0038-0717(95)00082-p
Niklińska, M., and Klimek, B. (2007). Effect of temperature on the respiration rate of forest soil organic layer along an elevation gradient in the Polish Carpathians. Biol. Fertil. Soils 43, 511–518. doi:10.1007/s00374-006-0129-y
Schimel, D. S., Braswell, B. H., Holland, E. A., Mckeown, R., Ojima, D. S., Painter, T. H., et al. (1994). Climatic, edaphic, and biotic controls over storage and turnover of carbon in soils. Glob. Biogeochem. Cycles 8, 279–293. doi:10.1029/94GB00993
Schipper, L. A., Hobbs, J. K., Rutledge, S., and Arcus, V. L. (2014). Thermodynamic theory explains the temperature optima of soil microbial processes and high Q10 values at low temperatures. Glob. Chang. Biol. 20, 3578–3586. doi:10.1111/gcb.12596
Spain, A. (1990). Influence of environmental conditions and some soil chemical properties on the carbon and nitrogen contents of some tropical Australian rainforest soils. Soil Res. 28, 825–839. doi:10.1071/sr9900825
Staddon, W. J., Trevors, J. T., Duchesne, L. C., and Colombo, C. A. (1998). Soil microbial diversity and community structure across a climatic gradient in Western Canada. Biodivers. Conserv. 7, 1081–1092. doi:10.1023/A:1008813232395
Stockmann, U., Padarian, J., McBratney, A., Minasny, B., de Brogniez, D., Montanarella, L., et al. (2015). Global soil organic carbon assessment. Glob. Food Secur. 6, 9–16. doi:10.1016/j.gfs.2015.07.001
Sundqvist, M. K., Sanders, N. J., and Wardle, D. A. (2013). Community and ecosystem responses to elevational gradients: Processes, mechanisms, and insights for global change. Annu. Rev. Ecol. Evol. Syst. 44, 261–280. doi:10.1146/annurev-ecolsys-110512-135750
Tan, X. P., Machmuller, M. B., Huang, F., He, J. H., Chen, J., Cotrufo, M. F., et al. (2020). Temperature sensitivity of ecoenzyme kinetics driving litter decomposition: The effects of nitrogen enrichment, litter chemistry, and decomposer community. Soil Biol. Biochem. 148, 107878. doi:10.1016/j.soilbio.2020.107878
Tashi, S., Singh, B., Keitel, C., and Adams, M. (2016). Soil carbon and nitrogen stocks in forests along an altitudinal gradient in the eastern Himalayas and a meta-analysis of global data. Glob. Chang. Biol. 22, 2255–2268. doi:10.1111/gcb.13234
Todd-Brown, K. E. O., Randerson, J. T., Post, W. M., Hoffman, F. M., Tarnocai, C., Schuur, E. A. G., et al. (2013). Causes of variation in soil carbon simulations from CMIP5 Earth system models and comparison with observations. Biogeosciences 10, 1717–1736. doi:10.5194/bg-10-1717-2013
Vitousek, P. M., Turner, D. R., Parton, W. J., and Sanford, R. L. (1994). Litter decomposition on the mauna loa environmental matrix, hawai'i: Patterns, mechanisms, and models. Ecology 75, 418–429. doi:10.2307/1939545
Wang, D., He, N., P., Wang, Q., Lu, Y., L., Wang, Q., F., Xu, Z., W., et al. (2016). Effects of temperature and moisture on soil organic matter decomposition along elevation gradients on the Changbai Mountains, Northeast China. Pedosphere 26, 399–407. doi:10.1016/S1002-0160(15)60052-2
Wang, Q., Liu, S., and Tian, P. (2018). Carbon quality and soil microbial property control the latitudinal pattern in temperature sensitivity of soil microbial respiration across Chinese forest ecosystems. Glob. Chang. Biol. 24, 2841–2849. doi:10.1111/gcb.14105
Wang, X., Zhao, X., and Gao, L. (2013). Climatic response of Betula ermanii along an altitudinal gradient in the northern slope of Changbai Mountain, China. Dendrobiology 70, 99–107. doi:10.12657/denbio.070.011
Xu, W., Li, W., Jiang, P., Wang, H., and Bai, E. (2014). Distinct temperature sensitivity of soil carbon decomposition in forest organic layer and mineral soil. Sci. Rep. 4, 6512. doi:10.1038/srep06512
Xu, X., Zhou, Y., Ruan, H. H., Luo, Y. Q., and Wang, J. S. (2010). Temperature sensitivity increases with soil organic carbon recalcitrance along an elevational gradient in the Wuyi Mountains, China. Soil Biol. Biochem. 42, 1811–1815. doi:10.1016/j.soilbio.2010.06.021
Xu, Z., Tang, S., Xiong, L., Yang, W., Yin, H., Tu, L., et al. (2015). Temperature sensitivity of soil respiration in China’s forest ecosystems: Patterns and controls. Appl. Soil Ecol. 93, 105–110. doi:10.1016/j.apsoil.2015.04.008
Yan, Z., Bond-Lamberty, B., Todd-Brown, K. E., Bailey, V. L., Li, S., Liu, C., et al. (2018). A moisture function of soil heterotrophic respiration that incorporates microscale processes. Nat. Commun. 9, 2562. doi:10.1038/s41467-018-04971-6
Yin, S., Wang, C., and Zhou, Z. (2021). Globally altitudinal trends in soil carbon and nitrogen storages. CATENA 210, 105870. doi:10.1016/j.catena.2021.105870
Zheng, Z. M., Yu, G. R., Fu, Y. L., Wang, Y. S., Sun, X. M., and Wang, Y. H. (2009). Temperature sensitivity of soil respiration is affected by prevailing climatic conditions and soil organic carbon content: A trans-China based case study. Soil Biol. Biochem. 41, 1531–1540. doi:10.1016/j.soilbio.2009.04.013
Keywords: soil organic matter, organic carbon, soil respiration, altitude gradient, temperature sensitivity
Citation: Li C, Xiao C, Li M, Xu L and He N (2022) A global synthesis of patterns in soil organic matter and temperature sensitivity along the altitudinal gradient. Front. Environ. Sci. 10:959292. doi: 10.3389/fenvs.2022.959292
Received: 07 July 2022; Accepted: 16 August 2022;
Published: 08 September 2022.
Edited by:
Dima Chen, China Three Gorges University, ChinaReviewed by:
Li Ji, Helmholtz Association of German Research Centres (HZ), GermanyYuhui Meng, China Three Gorges University, China
Copyright © 2022 Li, Xiao, Li, Xu and He. This is an open-access article distributed under the terms of the Creative Commons Attribution License (CC BY). The use, distribution or reproduction in other forums is permitted, provided the original author(s) and the copyright owner(s) are credited and that the original publication in this journal is cited, in accordance with accepted academic practice. No use, distribution or reproduction is permitted which does not comply with these terms.
*Correspondence: Chunwang Xiao, Y3d4aWFvQG11Yy5lZHUuY24=; Mingxu Li, bWluZ3h1bGlAaWdzbnJyLmFjLmNu