- 1Department of Biology, University of Naples Federico II, Naples, Italy
- 2Department of Chemical Sciences, University of Naples Federico II, Naples, Italy
- 3Centro Servizi Metrologici e Tecnologici Avanzati (CeSMA), Complesso Universitario di Monte Sant'Angelo, Naples, Italy
The increasing use and demand of rare earth elements in many emerging technologies is leading to a potentially higher input to the marine environment. This study compared for the first time the effect of lanthanum (La), cerium (Ce), neodymium (Nd), samarium (Sm), europium (Eu), gadolinium (Gd), dysprosium (Dy), and erbium (Er) to the microalga Phaeodactylum tricornutum Bohlin. The algal growth inhibition was investigated after 72 h of exposure. The median effect concentrations (EC50) ranged from 0.98 mg/L to 13.21 mg/L and elements were ranked as follows: Gd > Ce > Er > La > Eu > Nd > Dy > Sm. The comparison of predicted no effect concentrations (PNEC) for hazard and risk assessment with measured environmental concentrations showed that ecological risks deriving from REEs could be present, but limited to specific environments like estuarine waters. The results support evidence of actions to manage the REE impact in seawater environments, looking to improve the monitoring tailored to the different and dynamic nature of ecosystems.
Introduction
Rare earth elements (REEs) can have potential harmful effects on environmental and human health, and the related exposure is expected to increase in the near future due to their wide use in technology, medicine, agriculture and industry (Pagano et al., 2019). REEs are considered essential for a large number of applications including the alternative energy sector (Hurst, 2010; Adeel et al., 2019; Cardoso et al., 2019) including permanent catalysis (24%), magnets (23%), polishing (18%), metallurgy (8%), and batteries (8%) (Roskill Information Services Ltd). REEs are strategic resources, but they are not renewable and are considered to be close to “peaking” (Rustad, 2012; Balaram, 2019) with an application boom that would continue also in the near future (Balaram, 2019). The REEs supply chain is still linear and recycling barely reaches 1% of the world production (Rustad, 2012). In this way, REEs enter the environment via many ways, particularly during disposal of consumer and industrial products (e.g., landfills), discharges from mining and mineral processing, and wastewater from industrial processes that use REEs (Migaszewski and Gałuszka, 2015; Gwenzi et al., 2018; Balaram, 2019). Anthropogenic REEs contamination can be of great concern in hot spots (e.g., ore mine tailings and abandoned mines) (Pagano et al., 2015a). The general alteration of their biogeochemical cycles can transform them into potential emerging contaminants (Pagano et al., 2015a; Pagano et al., 2015b; Gravina et al., 2018; Gwenzi et al., 2018; Balaram, 2019; Galdiero et al., 2019; Pagano et al., 2019; Naccarato et al., 2020). There is still relatively little knowledge of the natural or anthropogenic cycles of REEs in the environment as well as their biological effects compared to cadmium (Cd), mercury (Hg), lead (Pb), chromium (Cr), and nickel (Ni) (Hirano and Suzuki, 1996). However, REEs and heavy metals (HMs) have similar environmental behaviours, can be accumulated by animals and plants and consequently they can pose potential risks in a one-health perspective (Pagano et al., 2015a; Gwenzi et al., 2018). Like HMs, REEs could generate reactive oxygen species (ROS), weaken, or inactivate the antioxidant defence and bind for the high affinity to vital macromolecules (Pagano et al., 2015a; Balali-Mood et al., 2021; Siciliano et al., 2021; Trapasso et al., 2021).
The increased mobilisation and concentration of REEs in water systems could have significant impacts primarily to aquatic organisms (Freitas et al., 2020). The REE-content in seawater can be determined by factors relating to different input sources (e.g., terrestrial, hydrothermal) and scavenging processes related to depth, salinity, and oxygen levels (Greaves et al., 1999; Nozaki, 2001). The distinctive character of the seawater REEs distribution is largely controlled by the uniform trivalent behaviour most REEs (except for Ce and Eu which vary with oxygen levels) and estuarine and oceanic scavenging processes (Nothdurft et al., 2004). In contrast, anthropogenic, strongly chelated, anionic REEs appear to have a conservative behaviour and a long environmental half-life (Kulaksız and Bau, 2007; Lawrence et al., 2009; Kulaksız and Bau, 2013). REEs anomalies due to anthropogenic activities were observed in seawaters in Plymouth Sound, UK (Karadaş et al., 2011), Ibaraki, Japan (Zhu, 2020) and Western Philippine (Goldstein and Jacobsen, 1988).
The biological effects and the toxicity of REEs is not fully understood, especially in saltwater. From the scarce literature on REE toxicity to aquatic organisms, only few data are available for marine organisms compared to freshwater ones (González et al., 2015; Freitas et al., 2020). Most studies demonstrated the ability of REEs to accumulate in marine species belonging to lower trophic levels, and consequently posing potential ecological risks (Pagano et al., 2015b; Ponnurangam et al., 2016; Gwenzi et al., 2018). Currently, no data about REEs exist about Phaeodactylum tricornutum Bohlin, that is, a cosmopolitan species found in transitional, marine-coastal and marine waters and considered as a reference model in monitoring seawater pollution and assessment of pure organic and inorganic chemicals (Libralato et al., 2016).
This study investigated the effects of lanthanum (La), cerium (Ce), neodymium (Nd), samarium (Sm), europium (Eu), gadolinium (Gd), dysprosium (Dy), and erbium (Er) on P. tricornutum growth explicitly providing the median and 10% effect concentrations (EC50 and EC10). The aims of this study were to: 1) generate ecotoxicological information regarding P. tricornutum poorly studied for the REE toxicity, 2) compare, if possible, REEs toxicity with HMs, and 3) weigh up the EC50 with concentrations already found in the seawater to determine the potential risk associated with these elements.
Materials and methods
Chemicals, testing solutions, and analytical characterization
Nitrate standard solutions of 8 REEs (100 mg/L) provided by Sigma-Aldrich (Saint Louis, United States of America) were used for conducting the toxicity trials [La(NO3)3 6H2O, purity 97%, Ce(NO3)3 6H2O, purity 97%; Nd(NO3)3 6H2O, purity 99.9%], Sm (NO3)3 6H2O, purity 99.9%, Eu(NO3)3 5H2O, purity 99.9%, Gd(NO3)3 6H2O, purity 99.9%, Dy(NO3)3 xH2O, purity 99.9% and Er(NO3)3 6H2O, purity 99.9%.Treatment REE solutions were prepared by diluting REE standard solutions into artificial seawater (ISO, 2016) at least 1 h prior to the exposure followed by the pH measurement (Mettler Toledo Five Easy, Milan, Italy).
Rare earth elements analytical concentrations were determined by inductively coupled plasma mass spectrometry (ICP-MS, Aurora M90 Bruker Daltonics Inc.). High-purity water (resistivity of 18.2 MΩ cm) was obtained from a Milli-Q unit (Millipore, United States). Nitric acid (HNO3, 69% v/v Ultratrace@ ppb-trace analysis grade) was provided by Scharlau (Barcelona, Spain). All samples analyzed in ICP-MS were prepared in HNO3 solution (2% v/v). The analysis was performed in Normal Sensitivity mode. Calibration curves for determining REEs ranged from 0.5 to 1,000 µg/L and were constructed daily by analysis of standard solutions prepared immediately before analysis. The internal standard was 115In for both calibration curve and sample analysis.
Phaeodactylum tricornutum bioassay
Axenic cultures of P. tricornutum microalgae were cultured at Hygiene Laboratory, Department of Biology at the University of Naples Federico II. Culture of P. tricornutum were routinely maintained in axenic artificial seawater medium supplemented with nutrients (ISO, 2016)at 22 ± 1°C and 4,800 lux on a 16:8 h light-dark cycle. Algal growth inhibition test (72 h) was performed according to ISO 10253:2016 (ISO, 2016) by using multiwell plates. For each concentrations four wells were filled with 2,250 µL of the respective solution (K2Cr2O7 positive control, spiked REE solution, sinthetic sea water as negative control), 125 μL of the 20-fold culture media and 125 μL of inocula of exponentially growing microalgae. One of the four replicates served as blanks the prepared well plates were then placed on a horizontal shaker with 50 rpm for 72 h at 22 ± 1°C under continuous light of 6,700 lux. Solutions spiked with REEs ranged between 0.3 mg/L and 5.0 mg/L (nominal concentrations).
After a 72 h of exposure, spectrophotometric measurement of samples at 670 nm (DR 5000 sc, Hach) allowed to determine algal cell density through linear regression equation that described the relationship between optical density and cell density. The growth rate compared to negative control was calculated according to ISO (2016).
Data analysis
After screening assays, testing concentrations were set up in order to allow the calculation of median, 20, 10 and 5% effect concentrations (EC50, EC20, EC10, and EC5) which were determined using linear and nonlinear regression. For each REE, the best-fitting model was selected based on the mean corrected coefficient of determination (R2) and by graphical interpretation of the model fit. Differences between treatments were assessed via one-way analysis of variance (ANOVA) after the verification of normality (Shapiro-Wilk’s test) and homoscedasticity (Levene’s test). Moreover, to further evaluate the risk of REEs, the estimated concentration causing an effect (EC50) was divided by an assessment factor (AF) to estimate a predicted no-effect concentration (PNEC). The ratio of measured environmental concentration (MEC) listed in Table 3 to the PNEC was calculated to estimate the risk quotient (RQ).
Results
REE nominal vs. analytical concentrations
Analytical concentrations used to calculate concentration-response curves are highlighted in Table 1. The ratio between analytical and nominal concentrations in most samples ranged from 0.8 to 1.2. Results are in accordance with previous studies (Pagano et al., 2016; Oral et al., 2017).
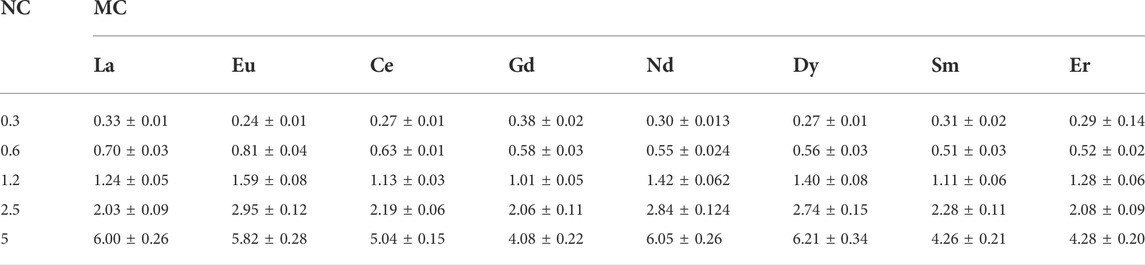
TABLE 1. Nominal and measured concentrations of rare earth elements and the relative standard deviations; NC = nominal concentrations; MC = measured concentrations (mg/L).
REE toxicity on P. tricornutum
In Figure 1, the results about the growth inhibition of P. tricornutum are reported for Ce, Dy, Eu, La, Er, Gd, Sm, and Nd. All equations and the relative standard errors are provided in Figure 1 and the determination of EC50, EC20, EC10, and EC5 are summarized in Table 2.
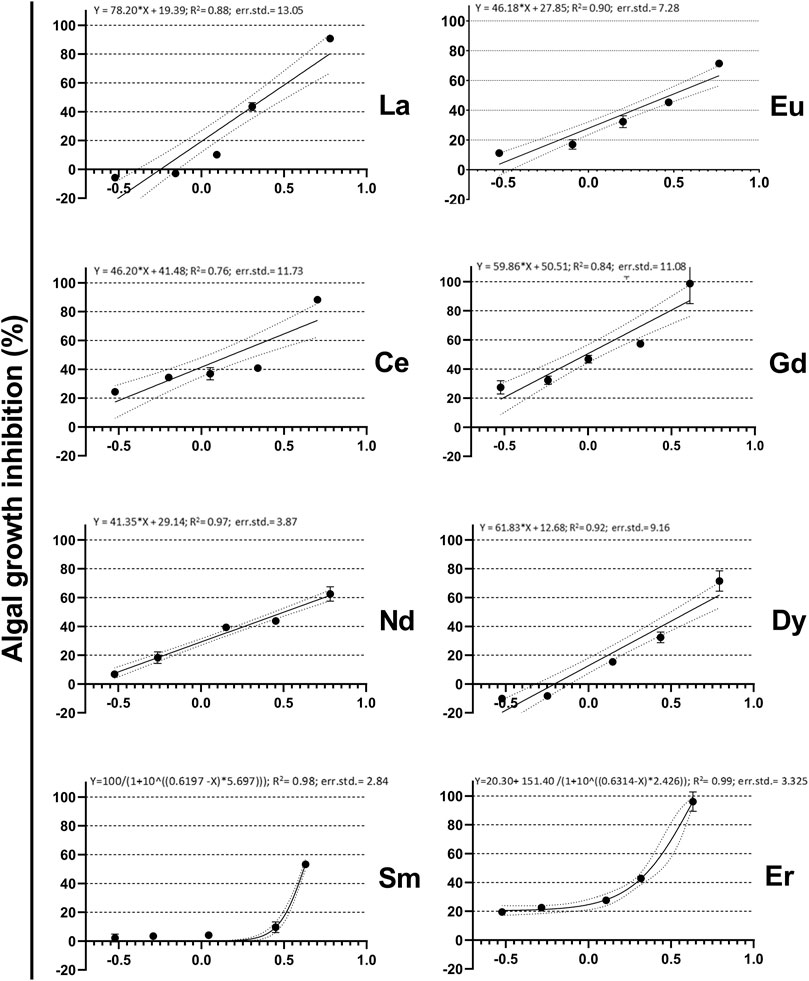
FIGURE 1. Concentration-response relationship of La, Eu, Ce, Gd, Nd, Dy, Sm, and Er exposed to P. tricornutum; x-axis log scaled concentrations are in mg/L. Solid line indicates the line of best fit, the dashed lines represent the 95% confidence intervals for linear regression (La, Eu, Ce, Gd, Nd, and Dy) and for nonlinear regression (Sm, and Er).
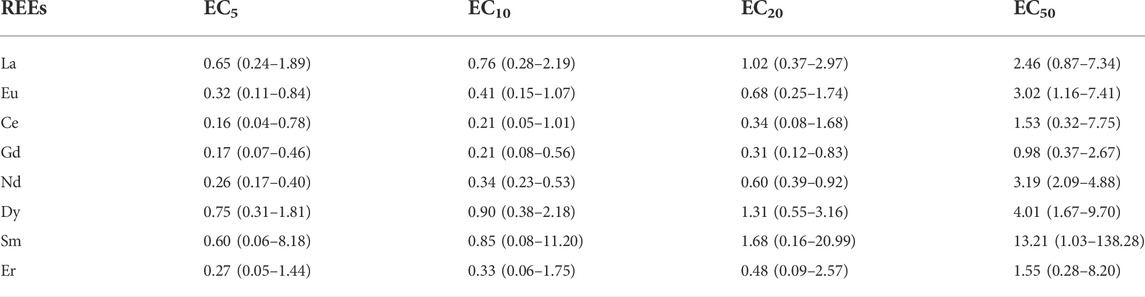
TABLE 2. EC5, EC10, EC20, and EC50 values for Ce, Dy, Eu; La and Nd on P. tricornutum; values are in mg/L; n.a. = not available; REEs = rare earth elements; EC = effective concentration; average EC values are provided ±95% confidence limit values in brackets (n = 3).
As a general overview, the algal growth always evidenced inhibitory effects at all the tested concentrations, except for La and Dy, which displayed biostimulation effect at the two lowest tested concentrations. Cells of P. tricornutum exposed to La and Dy concentrations between 0.3 and 0.7 mg/L after 72 h showed greater cell density than the control group. The elements that caused the highest growth inhibition at the lowest concentrations were Gd and Ce (27 and 24%, respectively).
Cell density of P. tricornutum at the highest concentrations exposed of Ce, La, Gd and Er showed the highest inhibition of growth with inhibitions of 88, 91, 98, and 96% respectively (Figure 1). In contrast, the highest concentrations of Eu, Nd, Dy, and Sm showed the lowest growth inhibition 72, 62, 71, and 53%, respectively (Figure 1).
The toxicity always showed a dose-response relationship. Both Dy and La displayed biostimulation at low concentrations, while the highest relative inhibition was observed at the highest concentration.
The EC50 values (±95% confidence limit values) obtained for all the tested elements were summarised in Table 2 including EC5, EC10 and E20. Based on the calculated EC50 values, the following toxicity relationship was established: Gd > Ce > Er > La > Eu > Nd > Dy > Sm.
Comparative risk assessment
About risk assessment, RQs for each REEs were estimated using values of MEC considering three different scenarios from the literature (coastal seawater, open seawater and estuarine seawater) (Wysocka and Vassileva, 2017; Zhu, 2020) and PNEC (Table 3). Wennmalm and Gunnarsson (2009) and Verlicchi et al. (2012) classified the environmental risk into different levels according to the RQs obtained. The classification follows: if RQ < 0.1 the environmental risk level is considered insignificant, an RQ from 0.1–1.0 is considered low, an RQ from 1.0–10 is considered moderate, and an RQ > 10 is considered high risk. For groups of relatively unknown sensitivity such as saltwater species and for acute laboratory studies, the assessment factor is between the range of 10–1,000 (Fairbrother, 2008). Because of the scarce data available about the toxicity of REEs to marine species, the factor of this study was set at 1,000.
In all scenarios, RQs were below 1 and none of the investigated REEs were classified as high risk. The estimated RQs varied from 0.0006 to 0.1803 with a mean value of 0.0346 in the coastal seawaters, from 0.0008 to 0.0403 in the open seawaters with a mean value of 0.0135 and from 0.0196 to 0.4606 in the estuarine waters with a mean value of 0.2154, indicating, in the latter case, a potential low risk posed by the REEs on the marine organisms. Only Ce posed low risks to aquatic organisms in the coastal seawaters, while all the other elements resulted in insignificant risk. Moreover, for open seawaters, all the REEs resulted in insignificant risks, including Ce, La and Nd, which are considered the most abundant REEs in the environment (Freitas et al., 2020; Adeel et al., 2019). Overall, of the 8 REEs, more than 60% exhibited potential ecotoxicological risks in the estuarine waters due to their relative high concentrations and/or toxicities. The results agreed with the previous study of (González et al., 2015) that REEs in the different exposure environmental conditions had ecological risks limited to some hotspots.
Discussion
To the best of our knowledge, this is the first study reporting a large set of REEs toxicity data towards P. tricornutum. Previous data are available only from Sun et al. (2019) on La but considering only nominal concentrations. The EC50 of La from this study (2.46 mg/L) is more than 4 times lower than that obtained by (Sun et al., 2019) (10.08 mg/L), being such difference attributable to the use in our paper of measured concentrations. However, the values obtained in both studies do not differ greatly and can therefore still be comparable in the effects on the growth. Moreover, to further evaluate the data obtained and interpret the toxicological relations for tested REEs, the results obtained were compared to those obtained by (Tai et al., 2010) on diatom Skeletonema costatum. From this study, the EC50 of Ce (1.53 mg/L) was lower than those obtained by Tai et al. (2010) (4.2 mg/L) and the Dy values obtained were very similar in value (4.01 and 4.6 mg/L).
Moreover, to understand better the sensitivity of P. tricornutum to metals, the EC50 values obtained were also compared to data available on the toxicity of heavy metals (Table 4). The ecotoxicity of HMs (e.g., Cd, Pb) is higher than that of REEs, when compared under similar experimental conditions and species. From the results presented in this study compared to those obtained by (Horvatić and Peršić, 2007), the element with the highest toxicity was Gd (0.98 mg/L), which differed around 4 orders of magnitude with the element with the highest toxicity mercury chloride (1.16·10−5 mg/L), had a similar toxicity to cobalt chloride (1.19 mg/L) and had a higher toxicity than cadmium nitrate (5.37 mg/L). Moreover, (Horvatić and Peršić, 2007), also found that low concentrations of Cd and Co had a stimulatory effect on the growth rate from concentrations between 0.02 and 1.25 mg/L for Co and 0.16–0.31 mg/L for Cd, which are in a similar range to those obtained for Dy and La, which presented a biostimulation effect from concentrations between 0.3 and 0.7 mg/L. Results displayed, indicate that HMs such as copper and mercury are more toxic than the tested REEs, nevertheless, the results obtained are in a similar range to the remaining HMs presented. Elements such as Hg, Pb, Co and Cr have shown to be the most toxic elements affecting the growth of P. tricornutum (Cabrita et al., 2016), this might be explained by the fact that the cells incorporate these elements and the internalization causes damages in terms of cell division and growth (Deng et al., 2013; Cabrita et al., 2014; Cabrita et al., 2016).
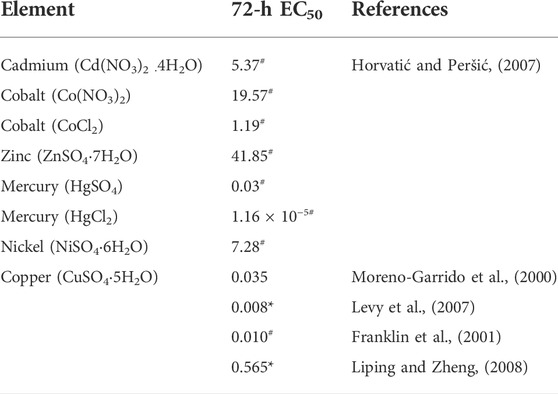
TABLE 4. Toxicity response of P. tricornutum to metals in 72-h growth inhibition bioassays. *Measured, #Nominal Concentrations.
The outcome of the environmental risk assessment of investigated REEs was different depending on the MECs of coastal, oceanic, and estuarine waters used to calculate the RQs. The evaluation results showed that RQ values were gradually increasing from ocean to estuary. The RQs were less than 0.1 in coastal and oceanic waters, except for Ce, which meant ecological environmental risk of REEs to marine species was not of concern. While for coastal and oceanic scenarios, hydrodynamics and significant dilution could explain the lower REE concentrations, it was notable that REEs concentrations were higher at estuarine waters, which could be attributed to the local sewage discharge and anthropic activities.
Either way, the RQs in the estuarine waters for REEs were always higher than 0.1. In this scenario, based on the RQ approach, the REEs assessed herein appear to be of raising marine concern, especially with regard to La and Ce, which could be explained based on the more frequent use of Ce and La that can promote their occurrence in environment as well as seawater. This work reinforces the urgent need for more data embracing other REEs and other species, in order to avoid under- or over- estimation when assessing the environmental risks of these metals.
Conclusion
Rare earth elements (Ce, La, Eu, Sm, Gd, Dy, Er, and Lu) effects to P. tricornutum growth inhibition were investigate. Results showed similar toxicity levels between light, medium and heavy REEs.
Toxicity can be compared to the effect of heavy metals on P. tricornutum. Although the differences in orders of magnitude between some elements, it can be observed that both groups of elements can have a bio-stimulatory effect but an inhibitory effect at higher concentrations. Further studies to evaluate the effects of low and constant exposure is suggested.
Further, this study presents a first insight on the effect of several REEs on the unicellular species P. tricornutum, which can be considered as a potential candidate to further study the effect of REEs on more complex matrices such as artificial sea water.
Based on P. tricornutum toxicity results, the presence of REEs in the marine environment does not seem to represent a widespread environmental risk except at some hotspots which may become more severe with the increase of REE application.
This approach could be used to further investigate more relevant endpoints, which could lead to indirect effects on the food chain and to study predictable patterns in bioavailability, bioaccumulation and ecotoxicity, as well as studies addressing the combined effect of several stressors such as REEs mixtures.
Data availability statement
The raw data supporting the conclusions of this article will be made available by the authors, without undue reservation.
Author contributions
AS: Conceptualization, Formal analysis, Data curation,Writing- original draft- review and editing. MS: Formal analysis. AP: Formal analysis. EP: Formal analysis, Data curation, Writing- original draft- review and editing. MTR: Formal analysis AB: Formal analysis, Data curation, Writing- original draft. LS: Formal analysis, Data curation. EG: Formal analysis. MTO: Funding acquisition. MG: Supervision, Funding acquisition- review and editing. GL: Writing—original draft, Supervision, Funding acquisition- review and editing.
Funding
This project has received funding from the European Union’s Horizon 2020 research and innovation programme under the Marie SkÅ‚odowska-Curie grant agreement N. 857989.
Conflict of interest
The authors declare that the research was conducted in the absence of any commercial or financial relationships that could be construed as a potential conflict of interest.
Publisher’s note
All claims expressed in this article are solely those of the authors and do not necessarily represent those of their affiliated organizations, or those of the publisher, the editors and the reviewers. Any product that may be evaluated in this article, or claim that may be made by its manufacturer, is not guaranteed or endorsed by the publisher.
References
Adeel, M., Lee, J. Y., Zain, M., Rizwan, M., Nawab, A., Ahmad, M., et al. (2019). Cryptic footprints of rare Earth elements on natural resources and living organisms. Environ. Int. 127, 785–800. doi:10.1016/j.envint.2019.03.022
Balali-Mood, M., Naseri, K., Tahergorabi, Z., Khazdair, M. R., and Sadeghi, M. (2021). Toxic mechanisms of five heavy metals: mercury, lead, chromium, cadmium, and arsenic. Front. Pharmacol. 12, 643972. doi:10.3389/fphar.2021.643972
Balaram, V. (2019). Rare Earth elements: A review of applications, occurrence, exploration, analysis, recycling, and environmental impact. Geosci. Front. 10 (4), 1285–1303. doi:10.1016/j.gsf.2018.12.005
Cabrita, M. T., Raimundo, J., Pereira, P., and Vale, C. (2014). Immobilised Phaeodactylum tricornutum as biomonitor of trace element availability in the water column during dredging. Environ. Sci. Pollut. Res. 21 (5), 3572–3581. doi:10.1007/s11356-013-2362-x
Cabrita, M. T., Gameiro, C., Utkin, A. B., Duarte, B., Caçador, I., and Cartaxana, P. (2016). Photosynthetic pigment laser-induced fluorescence indicators for the detection of changes associated with trace element stress in the diatom model species Phaeodactylum tricornutum. Environ. Monit. Assess. 188 (5), 285–313. doi:10.1007/s10661-016-5293-4
Cardoso, C. E., Almeida, J. C., Lopes, C. B., Trindade, T., Vale, C., and Pereira, E. (2019). Recovery of rare Earth elements by carbon-based nanomaterials—a review. Nanomaterials 9 (6), 814. doi:10.3390/nano9060814
Deng, C., Zhang, D., Pan, X., Chang, F., and Wang, S. (2013). Toxic effects of mercury on PSI and PSII activities, membrane potential and transthylakoid proton gradient in Microsorium pteropus. J. Photochem. Photobiol. B Biol. 127, 1–7. doi:10.1016/j.jphotobiol.2013.07.012
Fairbrother, A. (2008). Risk management safety factor. Encycl. Ecol. Ecotoxicol. 4, 3062–3068. doi:10.1016/B978-008045405-4.00426-2
Franklin, N. M., Stauber, J. L., and Lim, R. P. (2001). Development of flow cytometry‐based algal bioassays for assessing toxicity of copper in natural waters. Environ. Toxicol. Chem. 20 (1), 160–170. doi:10.1002/etc.5620200118
Freitas, R., Costa, S., Cardoso, C. E., Morais, T., Moleiro, P., Matias, A. C., et al. (2020). Toxicological effects of the rare Earth element neodymium in Mytilus galloprovincialis. Chemosphere 244, 125457. doi:10.1016/j.chemosphere.2019.125457
Galdiero, E., Carotenuto, R., Siciliano, A., Libralato, G., Race, M., Lofrano, G., et al. (2019). Cerium and erbium effects on Daphnia magna generations: A multiple endpoints approach. Environ. Pollut. 254, 112985. doi:10.1016/j.envpol.2019.112985
Goldstein, S. J., and Jacobsen, S. B. (1988). Rare Earth elements in river waters. Earth Planet. Sci. Lett. 89 (1), 35–47. doi:10.1016/0012-821x(88)90031-3
González, V., Vignati, D. A., Pons, M.-N., Montarges-Pelletier, E., Bojic, C., and Giamberini, L. (2015). Lanthanide ecotoxicity: First attempt to measure environmental risk for aquatic organisms. Environ. Pollut. 199, 139–147. doi:10.1016/j.envpol.2015.01.020
Gravina, M., Pagano, G., Oral, R., Guida, M., Toscanesi, M., Siciliano, A., et al. (2018). Heavy rare Earth elements affect Sphaerechinus granularis sea urchin early life stages by multiple toxicity endpoints. Bull. Environ. Contam. Toxicol. 100 (5), 641–646. doi:10.1007/s00128-018-2309-5
Greaves, M., Elderfield, H., and Sholkovitz, E. (1999). Aeolian sources of rare Earth elements to the Western Pacific Ocean. Mar. Chem. 68 (1-2), 31–38. doi:10.1016/s0304-4203(99)00063-8
Gwenzi, W., Mangori, L., Danha, C., Chaukura, N., Dunjana, N., and Sanganyado, E. (2018). Sources, behaviour, and environmental and human health risks of high-technology rare Earth elements as emerging contaminants. Sci. Total Environ. 636, 299–313. doi:10.1016/j.scitotenv.2018.04.235
Hirano, S., and Suzuki, K. T. (1996). Exposure, metabolism, and toxicity of rare earths and related compounds. Environ. health Perspect. 104, 85–95. doi:10.2307/3432699
Horvatić, J., and Peršić, V. (2007). The effect of Ni2+, Co2+, Zn2+, Cd2+ and Hg2+ on the growth rate of marine diatom Phaeodactylum tricornutum Bohlin: microplate growth inhibition test. Bull. Environ. Contam. Toxicol. 79 (5), 494–498. doi:10.1007/s00128-007-9291-7
Hurst, C. A. (2010). China's ace in the hole rare earth elements. Leavenworth: FOREIGN MILITARY STUDIES OFFICE (ARMY) FORT LEAVENWORTH KS.
ISO (2016). 10253:2016 water quality -- marine Al gal growth inhibition test with Skeletonema sp. and Phaeodactylum tricornutum. Geneva: ISO.
Karadaş, C., Kara, D., and Fisher, A. (2011). Determination of rare Earth elements in seawater by inductively coupled plasma mass spectrometry with off-line column preconcentration using 2, 6-diacetylpyridine functionalized Amberlite XAD-4. Anal. Chim. acta 689 (2), 184–189. doi:10.1016/j.aca.2011.01.049
Kulaksız, S., and Bau, M. (2007). Contrasting behaviour of anthropogenic gadolinium and natural rare Earth elements in estuaries and the gadolinium input into the North Sea. Earth Planet. Sci. Lett. 260 (1-2), 361–371. doi:10.1016/j.epsl.2007.06.016
Kulaksız, S., and Bau, M. (2013). Anthropogenic dissolved and colloid/nanoparticle-bound samarium, lanthanum and gadolinium in the Rhine River and the impending destruction of the natural rare Earth element distribution in rivers. Earth Planet. Sci. Lett. 362, 43–50. doi:10.1016/j.epsl.2012.11.033
Lawrence, M. G., Ort, C., and Keller, J. (2009). Detection of anthropogenic gadolinium in treated wastewater in South East Queensland, Australia. Water Res. 43 (14), 3534–3540. doi:10.1016/j.watres.2009.04.033
Levy, J. L., Stauber, J. L., and Jolley, D. F. (2007). Sensitivity of marine microalgae to copper: the effect of biotic factors on copper adsorption and toxicity. Sci. Total Environ. 387 (1-3), 141–154. doi:10.1016/j.scitotenv.2007.07.016
Libralato, G., Gentile, E., and Ghirardini, A. V. (2016). Wastewater effects on Phaeodactylum tricornutum (Bohlin): Setting up a classification system. Ecol. Indic. 60, 31–37. doi:10.1016/j.ecolind.2015.06.014
Liping, W., and Zheng, B. (2008). Toxic effects of fluoranthene and copper on marine diatom Phaeodactylum tricornutum. J. Environ. Sci. 20 (11), 1363–1372. doi:10.1016/s1001-0742(08)62234-2
Migaszewski, Z. M., and Gałuszka, A. (2015). The characteristics, occurrence, and geochemical behavior of rare Earth elements in the environment: a review. Crit. Rev. Environ. Sci. Technol. 45 (5), 429–471. doi:10.1080/10643389.2013.866622
Moreno-Garrido, I., Lubián, L. M., and Soares, A. M. (2000). Influence of cellular density on determination of EC50 in microalgal growth inhibition tests. Ecotoxicol. Environ. Saf. 47 (2), 112–116. doi:10.1006/eesa.2000.1953
Naccarato, A., Tassone, A., Cavaliere, F., Elliani, R., Pirrone, N., Sprovieri, F., et al. (2020). Agrochemical treatments as a source of heavy metals and rare Earth elements in agricultural soils and bioaccumulation in ground beetles. Sci. Total Environ. 749, 141438. doi:10.1016/j.scitotenv.2020.141438
Nothdurft, L. D., Webb, G. E., and Kamber, B. S. (2004). Rare Earth element geochemistry of late devonian reefal carbonates, canning basin, western Australia: confirmation of a seawater REE proxy in ancient limestones. Geochimica Cosmochimica Acta 68 (2), 263–283. doi:10.1016/S0016-7037(03)00422-8
Nozaki, Y. (2001). “Rare earth elements and their isotopes in the ocean,” in Encyclopedia of ocean sciences. Editor J. H. Steele (Oxford: Academic Press), 2354–2366.
Oral, R., Pagano, G., Siciliano, A., Gravina, M., Palumbo, A., Castellano, I., et al. (2017). Heavy rare Earth elements affect early life stages in Paracentrotus lividus and Arbacia lixula sea urchins. Environ. Res. 154, 240–246. doi:10.1016/j.envres.2017.01.011
Pagano, G., Aliberti, F., Guida, M., Oral, R., Siciliano, A., Trifuoggi, M., et al. (2015a). Rare Earth elements in human and animal health: state of art and research priorities. Environ. Res. 142, 215–220. doi:10.1016/j.envres.2015.06.039
Pagano, G., Guida, M., Tommasi, F., and Oral, R. (2015b). Health effects and toxicity mechanisms of rare Earth elements—knowledge gaps and research prospects. Ecotoxicol. Environ. Saf. 115, 40–48. doi:10.1016/j.ecoenv.2015.01.030
Pagano, G., Guida, M., Siciliano, A., Oral, R., Koçbaş, F., Palumbo, A., et al. (2016). Comparative toxicities of selected rare Earth elements: Sea urchin embryogenesis and fertilization damage with redox and cytogenetic effects. Environ. Res. 147, 453–460. doi:10.1016/j.envres.2016.02.031
Pagano, G., Thomas, P. J., Di Nunzio, A., and Trifuoggi, M. (2019). Human exposures to rare Earth elements: Present knowledge and research prospects. Environ. Res. 171, 493–500. doi:10.1016/j.envres.2019.02.004
Ponnurangam, A., Bau, M., Brenner, M., and Koschinsky, A. (2016). Mussel shells of Mytilus edulis as bioarchives of the distribution of rare Earth elements and yttrium in seawater and the potential impact of pH and temperature on their partitioning behavior. Biogeosciences 13 (3), 751–760. doi:10.5194/bg-13-751-2016
Roskill Information Services Ltd (2015). Rare earths global industry, markets and outlook London: Roskill Information Services.
Rustad, J. R. (2012). Peak nothing: Recent trends in mineral resource production. Environ. Sci. Technol. 46 (3), 1903–1906. doi:10.1021/es203065g
Siciliano, A., Guida, M., Serafini, S., Micillo, M., Galdiero, E., Carfagna, S., et al. (2021). Long-term multi-endpoint exposure of the microalga Raphidocelis subcapitata to lanthanum and cerium. Sci. Total Environ. 790, 148229. doi:10.1016/j.scitotenv.2021.148229
Sun, D., He, N., Chen, Q., and Duan, S. (2019). Effects of lanthanum on the photosystem II energy fluxes and antioxidant system of Chlorella Vulgaris and Phaeodactylum Tricornutum. Int. J. Environ. Res. Public Health 16 (12), 2242. doi:10.3390/ijerph16122242
Tai, P., Zhao, Q., Su, D., Li, P., and Stagnitti, F. (2010). Biological toxicity of lanthanide elements on algae. Chemosphere 80 (9), 1031–1035. doi:10.1016/j.chemosphere.2010.05.030
Trapasso, G., Chiesa, S., Freitas, R., and Pereira, E. (2021). What do we know about the ecotoxicological implications of the rare Earth element gadolinium in aquatic ecosystems? Sci. Total Environ. 781, 146273. doi:10.1016/j.scitotenv.2021.146273
Verlicchi, P., Al Aukidy, M., and Zambello, E. (2012). Occurrence of pharmaceutical compounds in urban wastewater: removal, mass load and environmental risk after a secondary treatment—a review. Sci. total Environ. 429, 123–155. doi:10.1016/j.scitotenv.2012.04.028
Wennmalm, Å., and Gunnarsson, B. (2009). Pharmaceutical management through environmental product labeling in Sweden. Environ. Int. 35 (5), 775–777. doi:10.1016/j.envint.2008.12.008
Wysocka, I., and Vassileva, E. (2017). Method validation for high resolution sector field inductively coupled plasma mass spectrometry determination of the emerging contaminants in the open ocean: Rare Earth elements as a case study. Spectrochim. Acta Part B At. Spectrosc. 128, 1–10. doi:10.1016/j.sab.2016.12.004
Keywords: rare earth elements, phaeodactylum tricornutum, algal growth, emerging contaminants, risk assessment
Citation: Siciliano A, Sabatino M, Paone A, Padilla Suarez EG, Toscanesi M, Brouziotis AA, Gambino E, Saviano L, Trifuoggi M, Guida M and Libralato G (2022) A first attempt to evaluate the toxicity to Phaeodactylum tricornutum Bohlin exposed to rare earth elements. Front. Environ. Sci. 10:957943. doi: 10.3389/fenvs.2022.957943
Received: 31 May 2022; Accepted: 27 July 2022;
Published: 29 August 2022.
Edited by:
Mallavarapu Megharaj, The University of Newcastle, AustraliaReviewed by:
Dawei Li, Jinan University, ChinaHung-Yun Lin, National Taiwan Ocean University, Taiwan
Copyright © 2022 Siciliano, Sabatino, Paone, Padilla Suarez, Toscanesi, Brouziotis, Gambino, Saviano, Trifuoggi, Guida and Libralato. This is an open-access article distributed under the terms of the Creative Commons Attribution License (CC BY). The use, distribution or reproduction in other forums is permitted, provided the original author(s) and the copyright owner(s) are credited and that the original publication in this journal is cited, in accordance with accepted academic practice. No use, distribution or reproduction is permitted which does not comply with these terms.
*Correspondence: Edith Guadalupe Padilla Suarez, ZWRpdGgucGFkaWxsYUB1bmluYS5jb20=
†These authors have contributed equally to this work and share first authorship