- 1Department of Agronomy, Kansas State University, Manhattan, KS, United States
- 2Escuela Superior Politécnica Del Litoral, ESPOL Facultad de Ciencias de la Vida, Campus Gustavo Galindo, Guayaquil, Ecuador
- 3KU Leuven, Division of Soil and Water Management, Heverlee, Belgium
- 4Kansas Department of Agriculture Laboratory, Manhattan, KS, United States
Elevated cadmium (Cd) concentrations in cacao (Theobroma cacao L.) beans have concerned chocolate consumers worldwide because of the potential detrimental human health effects. Compost application on the soil surface could modify the labile Cd in soils and yet it could enhance Cd bound to humic and fulvic acids, forming an organo-metallic complex that could reduce the availability of Cd to plants. This study investigated the effect of surface compost applications at two rates, the chemistry and fractionation of Cd at two soil depths, and the relationship of these soil Cd pools with plant uptake. The research was carried out on four Ecuadorian cacao farms. The compost was applied at 6.25 Mg·ha−1 (low) and 12.5 Mg·ha−1 (high) per annum. There was also a control treatment with no compost application. Soil samples were collected at two depths, surface (0–5 cm) and below surface (5–20 cm). Leaf samples and cacao pots were collected from each treatment. Soil Cd was fractionated into five operational pools. Additionally, the Cd-bound to fulvic acids and humic acids in soils were extracted separately. The EDTA-extractable fraction showed the highest concentration of Cd at both depths. Cadmium bound to fulvic acids was higher in compost-applied soils than in the control (p < 0.05) in all farms. Leaf and bean-Cd were negative and significantly correlated with the Cd extracted by EDTA, NaOH, HNO3, and FA-Cd pools. The mobility of Cd in soils cultivated with cacao, based on plant uptake, was strongly associated with the soils’ chemical characteristics, especially pH and SOC. The surface application of organic matter facilitates the redistribution of Cd in soil fractions, mainly in EDTA-, NaOH-, and HNO3-extractable fractions, suggesting a reduction in Cd soil–plant transfer via adsorption or complexation processes. Apparently, the application of high-quality compost, i.e., high FA content, could aid in mitigating Cd contamination in cacao orchards. Experiments on perennial crops merit a longer evaluation time to better assess the changes in plant-Cd.
Introduction
Cadmium (Cd) is a non-essential trace element with unknown physiological activity in plants, animals, and humans (Kabata-Pendias, 2000). Cadmium is naturally present in soils with a concentration below 1.1 mg·kg−1 in non-polluted soils (Smolders and Mertens, 2013). This element is readily taken up by plants from the soil and translocated to edible parts. The main Cd exposure pathway to humans is the ingestion of Cd-contaminated food (Nordberg et al., 2002; Islam et al., 2015). Chronic Cd toxicity has been linked to kidneys, pulmonary, and cardiovascular diseases (Nordberg, 2009; Robertsa, 2014). It has also been identified as a factor for osteoporosis development (Buha et al., 2019). In the last few years, there is an increasing concern within the cacao supply chain about the high concentration of Cd in cacao beans and the direct transmission to chocolates. Due to the risk of Cd exposure, the European Union (EU) has implemented a regulation to limit the maximum permissible levels of Cd in chocolates and various cacao products (The European Commission, 2014). This regulation could limit the trade of cacao from Latin American and Caribbean countries to European markets if a feasible mitigation strategy is not found. Cacao (T. cacao L.) is one of the most important agricultural commodities in this region. Ecuador is one of the major players, with an annual cacao bean production of around 345,000 Mg in 2019/2020. However, in some areas, the Cd concentration in cacao beans could be above a selected threshold of 0.6 mg·kg−1 dry weight (DW), which may impair the commercialization of the commodity (Chavez et al., 2015; Barraza et al., 2017; Argüello et al., 2019). Soil chemical stabilization methods are considered the most cost-effective mitigation strategy in agricultural soils (McLaughlin et al., 1995; Chen et al., 2000; Liu et al., 2007). The use of organic amendments has been proposed as a plausible mitigation strategy to reduce Cd accumulation in cacao beans (Argüello et al., 2019; Ramtahal et al., 2019). Prediction models showed that a 2-fold increase in soil organic carbon (SOC) could reduce bean Cd level by a factor of 1.9 (Vanderschueren et al., 2021). In this context, organic amendments, i.e., composts, are among the most popular soil conditioners. The application of compost to the soil surface can change the mobility and bioavailability of trace elements by redistributing Cd in soluble and exchangeable organic matter, carbonates, and residual pools which are less available. These changes can reduce Cd availability, plant uptake, and leaching into groundwater (Walker et al., 2003; Chavez et al., 2015). Organic matter application can increase the content of fulvic and humic acids, consequently complexing Cd and restricting its mobility and plant uptake (Karlsson et al., 2005; Al Chami et al., 2013; Essington, 2015). Organic matter and Cd interactions are attributed to various processes, including adsorption, complexation, precipitation, redox reactions, and modification of soil properties (such as pH, cation exchange capacity, and salinity) as well as the nature of the organic matter, particularly the degree of humification (Staunton, 2002; Karaca, 2004; Walker et al., 2004; Bolan et al., 2014; Huang et al., 2016). Most of the researchers have reported the effect of organic amendments in Cd mitigation in seasonal crops whereas such evaluation in perennial crops, e.g., cacao, is very vague. Ramtahal et al. (2019, 2022) used lime and biochar to mitigate Cd contamination in cacao. The authors reported a lower leaf–Cd concentration when using commercially available biochar. Neither of those field studies identified the mechanism by which biochar reduced the Cd concentration in the soil and plants. In this study, we evaluated the effects of organic matter application, as compost, in the redistribution of Cd among organic and inorganic soil phases and the relationship between these changes and Cd uptake. The objective of the study was to determine the effect of surface compost applications in four cacao farms at two rates, on the chemistry and fractionation of Cd at two soil depths (0–5 and 5–20 cm), and the relationship of these soil Cd pools with plant uptake. In addition, the aging effect of compost application was analyzed by sampling farms 3 and 12 months after the amendment application.
Materials and methods
Location and sampling strategy
The research was carried out in four Ecuadorian cacao farms, three of them located in Manabí (farms 1–3) and one located in the Guayas (farm 4) province in Ecuador, both major cacao-producing provinces affected by high Cd in cacao beans (Argüello et al., 2019). The total soil Cd concentration ranged between 0.37 mg·kg−1 to 0.92 mg·kg−1. In addition, all cacao farms showed a clay soil texture. In all farms, the organic matter was applied at two rates in the form of commercial compost, produced from different plant raw materials (e.g., manure, leaves, twigs, and corncob). The compost had an organic matter content of 62% with a pH ≥ 6.16, EC = 5.13 mS·cm−1, and Cd concentration of 0.03 mg·kg−1. The compost was applied at two rates: 6.25 Mg·ha−1 (low) and 12.5 Mg·ha−1 (high). There was also a control treatment with no compost application. These compost rates were applied once in farms 1, 2, and 3 (Manabí) and twice in farm 4 (Guayas). The times of application were 3 (in Manabí) and 12 months (Guayas) before samples were collected. This sampling strategy was followed to estimate the aging effect of compost application. The treatments were established in plots of 21 trees which consisted of 16 border trees and five central trees where the samples were obtained. The compost was applied on the surface of the soils at a 1-m radius from the trunk. Leaf litter and twigs were removed from the soil surface before compost application. All treatments were applied in a completely randomized block design (CRBD) with three replicates per farm. Soil samples were collected at 3 and 12 months after the first compost application in the Manabí and Guayas farms, respectively. Four soil sub-samples were taken per tree at 70 cm from the main trunk. The soil samples were separated into two depths, surface (0–5 cm) and below surface (5–20 cm). Soil was placed in a plastic bucket and mixed thoroughly. Approximately 1 kg of the mixed soil was collected per treatment. Before chemical analysis, the soil samples were air-dried, ground, and sieved through a stainless-steel mesh (<2 mm). Cacao is a slow-growing perennial tree. From early leaf development to full maturity, the tree needs around 7 months (Gil et al., 2017). Therefore, to capture the soil influence in cacao tissue, leaves were collected at two-time intervals: 0 and 3 months after the soil sampling in the Manabí farms (i.e., 6 months after compost addition); and 0 and 10 months after soil sampling in the Guayas (i.e., 12 months after compost addition). At each time interval, four middle-aged leaves were collected from each of the central trees. In total, 20–30 leaves were collected from different branches around the canopy of the central trees. The leaf samples were washed with deionized water to remove any adhered particles, and oven-dried at 65°C for 72 h. Later, the dry leaves were ground using a blender and sieved through a stainless-steel mesh (<850 µm) and healthy and ripe cacao pods were collected from each treatment 12 months after the compost application. Cacao pods were opened and beans from each pod were thoroughly mixed. A sub-sample of this mixture was washed with deionized water and oven-dried at 65°C for 72 h. Finally, the unfermented and unpeeled dry samples were ground using a blender and stored for chemical elemental analysis.
Soil and amendment properties
Soil pH and electrical conductivity (EC) were measured with deionized water (1:5 soil/water ratio) using a pH/conductivity meter (Thermo Scientific Orion glass electrode, Beverly, MA), previously calibrated in buffer solutions (Thomas, 1996). Soil organic carbon (SOC) was determined by using the dry combustion method using an elemental analyzer vario MACRO Cube (Nelson and Sommers, 1996). Soil texture was determined by using the hydrometer method (Sikora and Moore-Kucera, 2014).
Total soil cadmium and sequential extraction procedure
Total soil Cd concentration was determined by acid digestion in aqua regia (3:1 HCl:HNO3 ratio) (Amacher, 1966). Soil Cd was fractionated into five pools, i.e., KNO3- (exchangeable), H2O- (sorbed), NaOH- (organic bound), EDTA- (carbonate bound), and HNO3- (residual), using a sequential extraction procedure developed by Sposito et al. (1982) and Mohamed et al. (2010) (Table 1). Briefly, 2 g of air-dried soil was mixed with 25 ml of 0.5M KNO3 and stirred for 16 h to obtain the KNO3 fraction. Then, the remaining soil was mixed with 25 ml of deionized water and shaken for 2 h to obtain the H2O fraction. This procedure was repeated thrice, and the extracts were combined. Later, the soil was mixed with 25 ml of 0.5M NaOH and shaken for 16 h to release the Cd bound to the NaOH fraction. After that, the soil was combined with 25 ml of 0.05M Na2-EDTA solution and shaken for 6 h to obtain EDTA. Finally, the remaining soil was mixed with 25 ml of 4M HNO3 and shaken for 16 h to extract residual Cd. At each of the extraction steps, the soils were centrifuged at 2,000 rpm for 30 min to obtain a clear supernatant which was decanted, filtered using Whatman Quantitative Filter Papers, Ashless, Grade 42, and stored in scintillation vials in a cold room at ∼4°C until analysis.
Humic and fulvic acid fractionation
Furthermore, fulvic acids (FA) and humic acids (HA) were extracted from 15 g of air-dried soil in a 1:10 soil to solution ratio (w/v) after the sequential addition of different reagents according to the International Humic Substance Society (Swift, 1996) with slight modifications (Pitumpe et al., 2018). The FA and HA fractionation procedures are summarized in Figure 1. Fulvic acid (FA1) was obtained by mixing the soil with 150 ml of 0.1M HCl, shaken for 1 h. The resulting supernatant was separated from the soil by centrifugation at 2,500 rpm for 30 min. The supernatant was decanted and stored in scintillation vials in a cold room at 5°C. Fulvic acid (FA2) was obtained from the remaining soil after extraction of FA1. Briefly, 150 ml of 0.1M NaOH was added to the soil residue and mixed for 4 h. The alkaline suspension was allowed to settle overnight, and the resulting supernatant was centrifuged at 2,500 rpm for 30 min. The soil residue was discarded, and the supernatant was acidified with 6M HCl with constant stirring at pH = 1. The suspension was allowed to stand for 16 h. Finally, FA2 was obtained after the separation of humic acid (precipitate) and fulvic acid (supernatant-FA2) fractions by centrifugation at 2,500 rpm for 30 min. The resulting supernatant (FA2) was decanted and stored in scintillation vials at 5°C. Lastly, the total Cd bound to fulvic acid was obtained after combining the solutions from FA1 and FA2. The humic acid (HA) fraction was obtained by re-dissolving the precipitate (resulting from FA procedures) with the addition of 40 ml of 0.1M KOH + 10 ml of 1M KOH + 3.351 g of KCl. The solution was shaken for 30 min and then acidified by adding 6M HCl constantly stirring at pH = 1. The acid suspension was allowed to settle overnight, and the resulting supernatant was collected after centrifuging at 2,500 rpm for 30 min. The supernatant was discarded, and the precipitate was dissolved in 40 ml of 0.1M NaOH. Lastly, the HA fraction was obtained by filtering the solution through 0.2 µm nylon filters (syringe), and the sealed filtrate was stored in 50 ml Falcon tubes in a cold room at 5°C.
Cadmium analysis
Total soil Cd concentration was quantified by an inductively coupled plasma optical emission spectrometer (ICP-OES, Perkin Elmer Optima 5300 DV). Cadmium concentrations in the different soil fractions (except H2O and EDTA) were quantified by Varian GTA 120 Graphite Tube Atomizer w/AA 240Z Zeeman Atomic Absorption Spectrometer (AAS). In addition, the EDTA and H2O fractions were confirmed by using an ICP-mass spectrometer (ICP-MS, Agilent 7500cx, Agilent Technologies). The total cadmium concentration in leaves and beans (except from farm 4) were determined after the digestion of 300 mg (beans) and 300 mg (leaves) in 6 ml (beans), and 5 ml (leaves) of concentrated nitric acid (HNO3) for about 6 h at 120°C (Thomas, 1966) and was quantified by the ICP-OES. However, in the leaves and beans from farm 4, the total Cd concentration was determined using an ICP-MS (Agilent 7700x) after microwave-assisted digestion (MARS 6 240/50, Matthews, NC). In this study, the practical quantification limit (PQL) for ICP-OES was 24 μg Cd L−1, for GFAAS was 0.6 μg Cd L−1, and for ICP-MS was 0.6 μg Cd L−1. Finally, certified references materials, NIST 2709a (soil), NIST 1573a (leaves), and cacao beans (FDA-CP36) were used for quality assurance–quality control (QA/QC) purposes, except for farm 4. Two standards, NIST 1573a tomato leaves and NIST 2384 baking chocolates for the tissue samples were included in some samples from farm 4. In addition, blanks, duplicates, and spiked samples were included in each digestion batch as part of the QA/QC protocol. The recoveries (±SD) of Cd calculated relative to the certified concentration (expressed as %) were 80.1 ± 0.05 for soil; 96.4 ± 0.03 (NIST 1573a), and 92.9 ± 3.70 (NIST 1573a farm 4) for leaves; 98.3 ± 0.01 (FDA-CP36) and 91.5 ± 10.2 (NIST 2384 farm 4) for cacao beans. In addition, soil and plant analyses contained duplicates in every 10 samples to evaluate the reproducibility. The coefficients of variation (CV) for each set of duplicated samples ranged from 1.29% to 2.80% (average 2.04%), from 2.09% to 2.75% (average 2.37%), and 7.58%–17.5% (average 11.5%) for Cd in the bean, leaf, and soil, respectively. The CV for each of the duplicate reference samples from sequential extraction procedures were between 7.32% and 17.1% and from 9.24% to 10.6% in the humus fractions.
Data analysis
All statistical analyses were performed using the JMP Pro version 15.1 software. One-way analysis of variance (ANOVA) was conducted to determine significant differences of Cd concentration among the fractions (KNO3, H2O, NaOH, EDTA, and HNO3) extracted by sequential extraction and humus fractions (FA and HA). Differences between the treatments were analyzed by using Dunnett’s post-hoc test at a p < 0.05 significance level. A multiple correlation analysis was performed to select the soil fractions that best corresponded to the Cd concentration in cacao leaves and beans. Data were checked to comply with ANOVA assumptions.
Results
Soil properties affected by organic matter application
Soil pH and soil organic carbon (SOC) were the properties affected by the application of compost. Treatments significantly increased (p < 0.05) the soil pH at farm 4 on surface and below surface depths, where the soil pH was on average 0.2 units higher in the low organic rate treatment than in the control. Soil organic carbon was mainly affected at farm 3 (p < 0.05), where the high-compost rate increased SOC by a factor ∼1.4 at both soil sampling depths. There was an increasing trend in SOC with the higher rate of compost in sites 1 and 4 (Table 2). The low rate was, on average, 1.4-fold more elevated than the control, and the high rate showed a 1.56- and 1.25-fold increase with respect to the control in farms 1 and 4, respectively. Despite these apparent differences, the compost application did not result in statistical differences, likely due to the high natural variability of the soils.
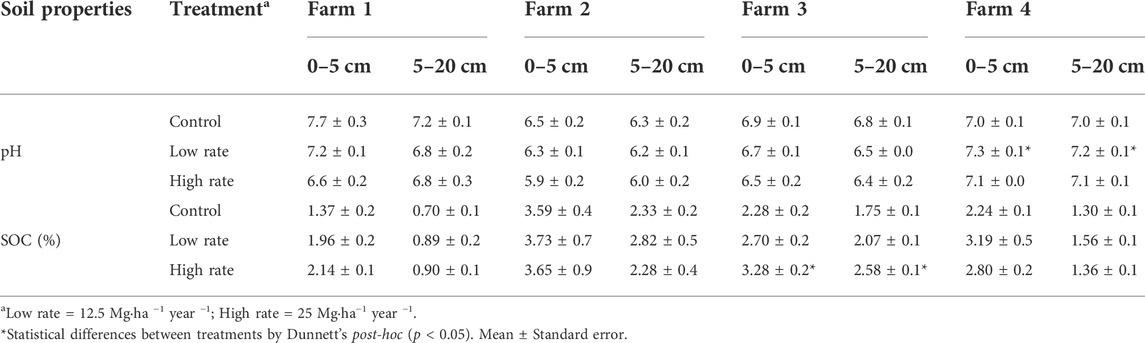
TABLE 2. Effect of organic matter application on the selected soil properties in the different field trials.
Cadmium pools affected by organic matter application
In general, the surface application of compost affected the Cd distribution in different soil fractions. Cadmium concentration showed a descending sequence order to EDTA > HNO3 > NaOH >> KNO3/H2O in all farms at the two soil depths (Figures 2, 3). For instance, the compost-amended soils showed a trend of reducing the Cd bound to the KNO3/H2O fraction. The greatest reductions were observed at farms 1 and 4. In farm 1, the Cd concentration in the low-rate treatment showed a 1.54-fold decrease compared to the control (p < 0.05) in the surface layer. In addition, the high-rate treatment showed a 1.44-fold reduction as compared to the control in below-surface depths (Figure 2). A similar trend was observed in farm 4. The compost applied at the high rate showed a 1.13- and 1.11-fold decrease as compared to the control at both depths, respectively (Figure 3). Cadmium bound to the NaOH fraction was higher (p < 0.05) in compost-amended soils at farm 4 at both depths. For example, the high rate increased NaOH-bound fraction by factor ∼3 compared to the control at surface and below-surface depths. A similar increase was observed in farms 1 and 4 in the below-surface soil depth. Cadmium bound to EDTA increased by factors 4 and 3.8 at farm 1 for the low and high-compost rates, respectively. Likewise, Cd-EDTA was 1.6- and 1.8-folds greater at the low and high rates, respectively at farm 4 (Figure 3). Finally, the concentration of Cd bound to the HNO3 fraction did not differ between the treatments in all farms at the two soil depths (Figure 3). Our results showed that the compost treatment tended to increase the Cd bound to the NaOH and EDTA fractions, mainly on below-surface soil depths. It appeared that the greatest effect was observed on the treatments with high compost rates where Cd associated with the NaOH fraction was 3.32-fold greater than that of the control. It is possible that, depending on organic matter mineralization and stabilization, humic substances can move down to lower soil depths, and subsequently modify the Cd associated with those soil fractions (Figure 4).
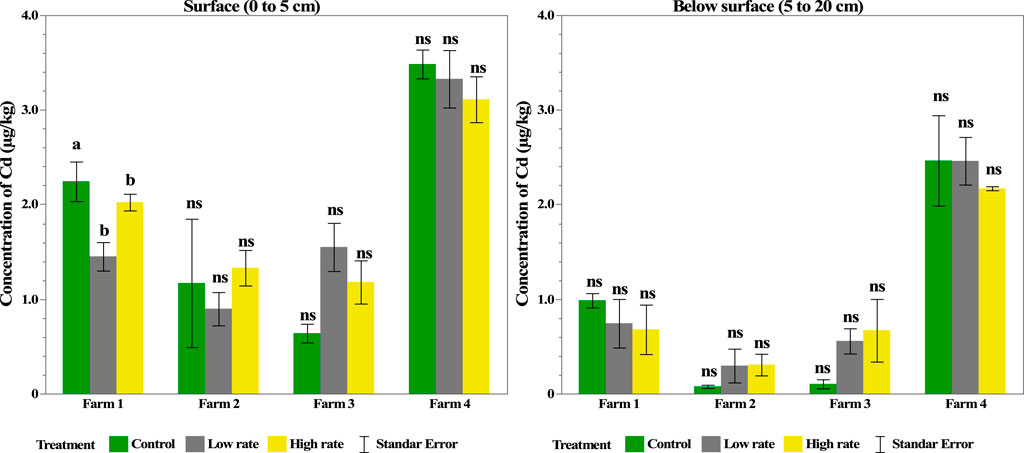
FIGURE 2. Cadmium concentrations bound to the KNO3/H2O fraction in the soil influenced by the compost applied at the surface and below-surface soil depth. Letters on the top of the bar indicate the statistical differences between treatments by using Dunnett’s post-hoc (p < 0.05). Ns: no statistical differences.
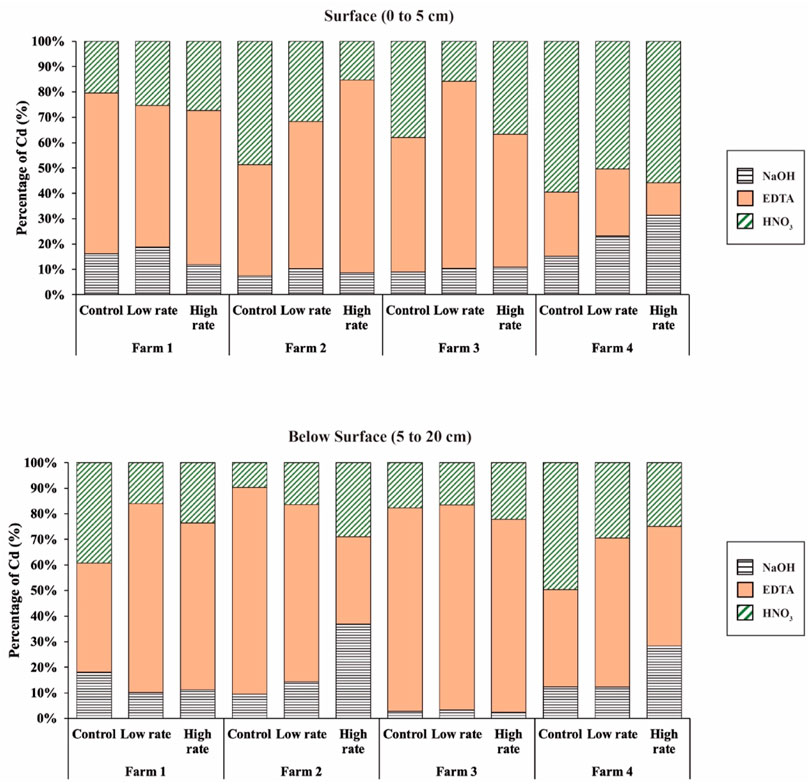
FIGURE 3. Soil-Cd expressed in percentage from different soil fractions at two depths in four field trials located in Ecuadorian cacao sites.
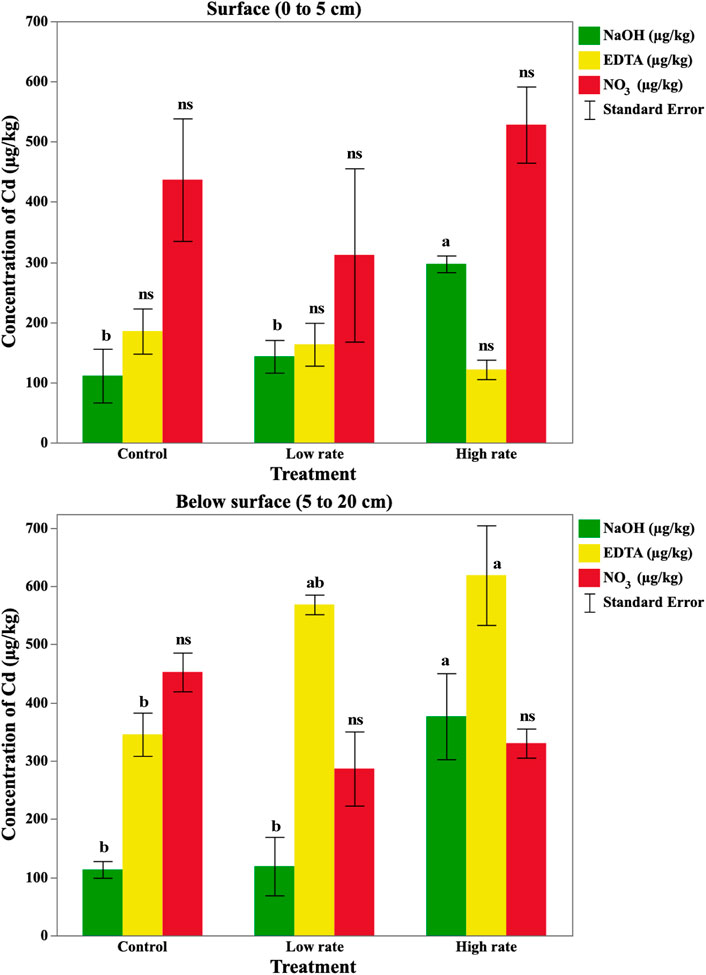
FIGURE 4. Cadmium concentrations in the soil fractions influenced by the compost applied at the surface and below-surface soil depths of farm 4. Letters on the top of the bar indicate the statistical differences between treatments by using Dunnett’s post-hoc (p < 0.05). Ns: no statistical differences.
Cadmium distribution among alkali-extractable soil organic carbon fractions
Soil Cd bound to humic acids (Cd-HA) differed (p < 0.05) between treatments at both depths (Table 3). On the 0–5 cm depth, the application of compost affected the concentration of Cd bound to HA. The greatest effect was observed at farm 1, where Cd in HA was nearly 6-folds greater when the compost was applied at a high rate as contrasted with unamended soils. Similarly, at farms 2 and 3, HA-Cd concentrations were nearly double in the high compost rate treatment as compared to the control. Furthermore, in farm 4, Cd-HA was 1.38-fold higher than control but only when compost was applied at the higher rate. On the 5–20 cm depth, the highest Cd-HA concentration was observed in farm 1, where the compost applied at the high rate was nearly 7-fold greater than the control. Moreover, at farms 3 and 4, the Cd-HA concentration in the high-rate treatment was on average 1.8-fold higher than the control. Compost application affected the concentration of Cd bound to FA (p < 0.05). On farm 1, the high-rate treatment showed a 1.4–fold increase compared to the control on the 0–5 cm depth (Table 3). Similarly, the compost applications resulted in significantly higher Cd-FA at farms 2, 3, and 4 in the 5–20 cm depth where the high-rate treatment increased the Cd-FA concentrations by factors of 2.2, 1.9, and 3.3-fold greater than the control, respectively (Table 3). Finally, in farm 4, the Cd-HA concentration was less variable within the replicates (CV < 15%) at both depths suggesting a potentially high degree of stabilization.
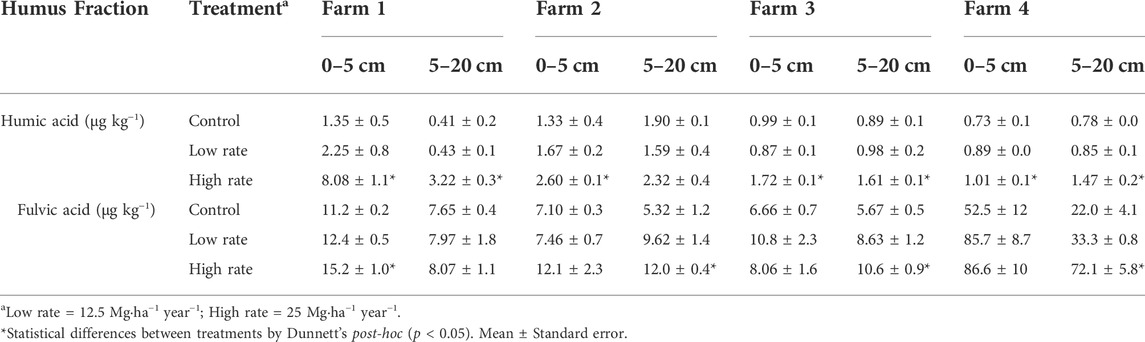
TABLE 3. Effect of compost application at two rates on Cd distribution among soil humus fractions at two depths in four cacao sites.
Identify the plant-available cadmium soil pool to cacao plants
A multiple correlation analysis was performed to study the relationship between the Cd associated with each soil Cd pool/fraction, sequential extraction fractions (KNO3/H2O, NaOH, EDTA, and HNO3) and humus fractions (FA and HA), physical–chemical parameters of the soils, and the Cd concentration in cacao leaves and beans. All data from the four farms were merged. The correlation analysis was distinguished by soil depths (surface or below surface) (Tables 4, 5). The two-leaf and one-bean sampling intervals were included to pinpoint when the tissue-Cd better reflects the changes in Cd pools in the soils. In general, soil and plant tissue parameters were more related to the below surface than to the surface soil depths. Leaf-Cd was negative and significantly correlated with the Cd in KNO3/H2O, -NaOH, -EDTA, and -HNO3 extractable pools at one or both soil sampling depths. In addition, leaf–Cd was more correlated with the Cd bound to FA than HA (p < 0.05). Soil pH showed a significant negative correlation with leaf–Cd at both soil depths. This negative relationship was more evident at later leaf samplings, e.g., 3 or 10 months after the soil was collected (Tables 4, 5). Finally, the bean–Cd concentration was negative affected by NaOH, and -HNO3 fraction, and FA and SOC (%) as well. The negative coefficient indicates that Cd bound to different soil fractions impaired the uptake of Cd by the cacao plant. Again, this effect was more notable at the second leaf sampling time, e.g., 3 and 10 months (leaves) and 12 months (beans) after the soil was collected. The aging effect of organic soil amendment may affect the absorption of Cd into beans and leaves 10 months after application. Surprisingly, humic acids showed a positive correlation with the leaf and bean Cd concentrations.
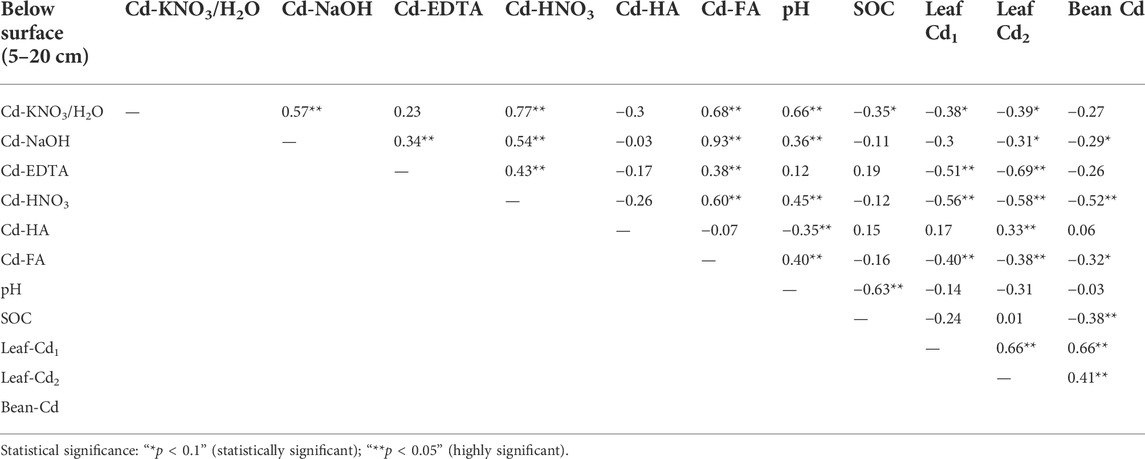
TABLE 5. Correlation matrix between the plant and soil parameters at the below surface (5–20 cm) soil depth.
Discussions
Cadmium distribution among the chemical fractions decreased in the following order: EDTA > HNO3 > NaOH >> KNO3/H2O in all farms. Similar results were found by Sposito et al. (1982), where the application of soil-composted sludge showed that the lowest concentration of Cd was bound to the KNO3/H2O fraction whereas the highest was bound to the EDTA fraction compared to NaOH or HNO3. These results suggest that the superficial organic matter application could reduce the amounts of Cd bound to available fractions and increase the contents of Cd bound to the HNO3, NaOH, and EDTA fractions (Sposito et al., 1982). In the absence of carbonates in soil, as the soils in the current study, EDTA extraction could extract metals specifically bound to organic matter and Fe/Al oxyhydroxides (Chrastný et al., 2008; Manouchehri and Bermond, 2009; Zhou et al., 2016). This is possible because EDTA can enhance the dissolution of Fe/Al oxyhydroxides via complexation or ligand-promoted dissolution (Essington, 2015). There is an apparent contradiction in the literature regarding the effect of organic matter on metal mobility, which may be due to the tendency of metals to bind with organic components in both the solid and solution phases of the soil (Rieuwerts et al., 1998). For example, metals may be complex with low-molecular weight organic carbon compounds in the soil solution phase, thereby increasing their mobility. However, it should be noted here that the most biologically active species are usually the free, unassociated metal ions; strong organo-metallic complexes in the soil solution would not be readily available for plant uptake (Essington, 2015). Finally, metals may be complexed with organic carbon compounds in the solid phase, and depending on the binding strength, restricting both mobility and availability of the metals (Esteves Da Silva and Oliveira, 2002).
The transformation of available-Cd to insoluble fractions can also be explained by soil pH changes (Wang et al., 2009). Increasing pH can increase Cd adsorption via multiple mechanisms. First, high pH causes an increase in the surface-negative charge, thereby increasing Cd2+ adsorption, especially in soils high in variable-charged minerals, such as the soils in Ecuador. Secondly, high pH favors the Cd hydrolysis forming Cd hydroxide (e.g., CdOH+) that are favorably adsorbed over metal cations; and thirdly, precipitation of Cd2+ as Cd(OH)2 (Sparks, 2003; Bolan et al., 2014; Palansooriya et al., 2020). Our results showed that compost application increased the soil pH on an average by 0.2 units compared to the control at both sampled depths. A similar effect was reported by Walker et al. (2004), where using an organic amendments in a pot experiment at two rates (24.3 and 23.3 g·kg−1) increased the soil pH by 0.84 units higher than the control. In addition, Li et al. (2008) reported that organic amendments such as pig manure and peat-increased soil pH by 1.31- and 1.18-folds compared to the control, respectively. On the other hand, as mentioned before, the SOC could form strong (i.e., inner-sphere) organo-metallic complexes with the trace metals (e.g., Zn, Cd, Cu, and Pb), reducing the bioavailability for the plants (Udom et al., 2004). It is possible that the concentrations of the available soil–Cd decreased with increasing soil pH observed in our study as an effect of amendment applications, suggesting that this could be one mechanism by which these amendments reduced Cd-bioavailability. Argüello et al. (2019) and Ramtahal et al. (2019) found that the most significant soil properties explaining Cd concentration in tissues were the soil pH and SOC in conjunction with total soil Cd, and oxalate extractable Mn. However, in their studies, the authors did not differentiate Cd distribution in different soil pools via sequential or humic substance (FA and HA) extraction procedures. Our results indicated that the soil parameters that had a significant relationship with Cd in cacao plant tissues (leaves—beans) were pH, SOC (%), and KNO3/H2O-, NaOH bound- and fulvic acid bound-Cd.
In addition, the complexation of organic C with soil Cd could result in an overall decrease in the mobility of the contaminant. The results from this study showed that the SOC (%) in the compost-treated soils were on average 1.4-times higher than the control. The cadmium bound to humic and fulvic acids were significantly enhanced because of the application of composts in contrast with the control in all farms, at both sampling depths. The cadmium bound to FA was, on average, 13-fold greater compared to Cd-HA. In general, FA has a lower molecular weight and more polar structure than HA. Some studies suggest that FA performs better than HA, because FA has a higher capacity or surface-site density than HA due to its higher number of reactive groups (mainly acidic carboxyl groups) for metal complexation (Donisa et al., 2003; Popovic et al., 2011; Essington, 2015; Rashid et al., 2018; Liu et al., 2022). The cadmium concentration in cacao leaves and beans showed a negative correlation with the Cd bound to FA (p < 0.05). This suggests that Cd-FA complexation in soils tended to decrease the plant’s Cd uptake. Similar results were reported by Esringü et al. (2021), where soil FA application resulted in Cd stabilization which limited its availability in the root zone reducing Cd in Tagetes eracta and Zinnia elegans crops by factor ∼14. A positive correlation between HA and plant Cd (leaves and beans) could be because of the relatively low enhancement of soil HA levels upon compost addition. The increased levels might not have been high enough to negate the potential enhancement of soil Cd mobility (Bolan et al., 2014; Rashid et al., 2018). Furthermore, it should be noted that an apparent contradiction also exists regarding the effect of FA on metal availability. Bloomfield, (1981) stated that the metal salts of FA are readily soluble. However, research has shown that, metal-binding sites on soluble humic substances, such as FA, could range from a very strong inner-sphere and polydentate to weak outer sphere-binding sites (Brown et al., 1999; Otto et al., 2001; Esteves Da Silva and Oliveira, 2002). In addition, metal-binding constants to FA increase with increasing pH (Saar and Weber, 1980; Buckley et al., 1985), likely due to decreasing the proton competition. It has been suggested that, when the concentration of FA far exceeds the metal concentration in soils, FA–metal complexations are dominated by strong and inner-sphere complexations (Essington, 2015), consequently restricting their plant availability. Similar to our study, previous studies also suggested a link between soil organic matter content and the fixation of metals in the soil’s solid phase (Elliott et al., 1986; Maskall et al., 1995, 1996). In contrast, some other studies have found no such association (Abd-Elfattah and Wada, 1981; Scokart et al., 1983).
Conclusion
Cadmium contamination in cacao beans merits an in-depth study; particularly in evaluating soil amendments applied to affect major soil properties. The surface application of the composts resulted in the redistribution of soil Cd from readily available into less available phases, i.e., NaOH, EDTA, and HNO3 fractions. The compost quality is critical for Cd-binding in amended soils. Our results suggest that the amount of fulvic acids present in the compost, and the equilibration time between the amendment and the soil, played a key role in modifying the Cd partitioning in organic and inorganic phases. The most significant effects in soils occurred after 12 months of compost application. Furthermore, time also affected the layer at which the effect was observed. For instance, in the oldest farm, the soil changes were observed at the 5–20 cm layer. The application of organic matter can increase the Cd bound to fulvic acids possibly forming strong metal–fulvate complexes thus affecting Cd availability and the potential plant uptake. Plant-Cd uptake was strongly associated to the soil’s chemical characteristics such as soil pH, and SOC. The cadmium bound to HNO3, and mainly in fulvic acids were directly linked to plant-Cd. The Cd bound to HNO3 and fulvic acid showed a significant and negative correlation with Cd in cacao tissues (leaves and beans), indicating that the Cd bound to these fractions in the soil might have reduce the uptake of Cd by the cacao plant. Moreover, the soil properties and Cd fractions at the 5–20 cm layer showed a greater impact to plant-Cd as compared to the 0–5 cm layer. Fulvic acids play a more critical role in reducing labile Cd as compared to humic acids maybe because of the greater quantity, stability, and retention capacity of the former organic acid. Further studies are needed to fully understand the long-term effects of organic amendments for mitigating Cd in the cacao crop. Compost maturity and higher fulvic and humic acid contents may be key for lowering plant Cd in field conditions.
Data availability statement
The raw data supporting the conclusion of this article will be made available by the authors, without undue reservation.
Author contributions
All authors listed have made a substantial, direct, and intellectual contribution to the work and approved it for publication.
Funding
All sources of funding received for the research are submitted.
Acknowledgments
The authors would like to thank the USDA—Trade and Foreign Agricultural Affairs—Foreign Agricultural Service, the Borlaug Fellowship Program 2019, Grant Number FX19BF-10777R044. The authors report that this contribution no. 22-302-J of the Kansas Agricultural Experiment Station. The authors also acknowledge the help of the Escuela Superior Politécnica del Litoral Soil and Plant nutrition research group and Kansas State University Soil and Environmental Chemistry research group. The authors thank Prof. Erik Smolders and the Division Soil and Water Management at KU Leuven for providing analytical service for some of the samples.
Conflict of interest
The authors declare that the research was conducted in the absence of any commercial or financial relationships that could be construed as a potential conflict of interest.
Publisher’s note
All claims expressed in this article are solely those of the authors and do not necessarily represent those of their affiliated organizations, or those of the publisher, the editors, and the reviewers. Any product that may be evaluated in this article, or claim that may be made by its manufacturer, is not guaranteed or endorsed by the publisher.
References
Abd-Elfattah, A., and Wada, K. (1981). Adsorption of lead, copper, zinc, cobalt, and cadmium by soils that differ in cation-exchange materials. J. Soil Sci. 32, 271–283. doi:10.1111/j.1365-2389.1981.tb01706.x
Al Chami, Z., Cavoski, I., Mondelli, D., and Miano, T. (2013). Effect of compost and manure amendments on zinc soil speciation, plant content, and translocation in an artificially contaminated soil. Environ. Sci. Pollut. Res. 20, 4766–4776. doi:10.1007/s11356-012-1439-2
Amacher, M. (1966). “NIckel, cadmium, and lead,” in Methods of soil analysis. Part 3, Chemical methods. Editor J. Bartels (Madison, Wisconsin, USA: Soil Science Society of America, Inc.), 742–763.
Argüello, D., Chavez, E., Lauryssen, F., Vanderschueren, R., Smolders, E., and Montalvo, D. (2019). Soil properties and agronomic factors affecting cadmium concentrations in cacao beans: A nationwide survey in Ecuador. Sci. Total Environ. 649, 120–127. doi:10.1016/j.scitotenv.2018.08.292
Barraza, F., Schreck, E., Lévêque, T., Uzu, G., López, F., Ruales, J., et al. (2017). Cadmium bioaccumulation and gastric bioaccessibility in cacao: A field study in areas impacted by oil activities in Ecuador. Environ. Pollut. 229, 950–963. doi:10.1016/j.envpol.2017.07.080
Bloomfield, C. (1981). “The translocation of metals in soils,” in The chemistry of soil processes. Editors D. J. Greenland, and M. H. B. Hayes (Chichester: John Wiley & Sons), 463–504.
Bolan, N., Kunhikrishnan, A., Thangarajan, R., Kumpiene, J., Park, J., Makino, T., et al. (2014). Remediation of heavy metal(loid)s contaminated soils - to mobilize or to immobilize? J. Hazard. Mat. 266, 141–166. doi:10.1016/j.jhazmat.2013.12.018
Brown, G. K., MacCarthy, P., and Leenheer, J. A. (1999). Simultaneous determination of Ca, Cu, Ni, Zn and Cd binding strengths with fulvic acid fractions by Schubert’s method. Anal. Chim. Acta X. 402, 169–181. doi:10.1016/S0003-2670(99)00530-9
Buckley, J. A., Yoshida, G. A., Wells, N. R., and Aquino, R. T. (1985). Toxicities of total and chelex-labile cadmium to salmon in solutions of natural water and diluted sewage with potentially different cadmium complexing capacities. Water Res. 19, 1549–1554. doi:10.1016/0043-1354(85)90400-2
Buha, A., Jugdaohsingh, R., Matovic, V., Bulat, Z., Antonijevic, B., Kerns, J. G., et al. (2019). Bone mineral health is sensitively related to environmental cadmium exposure- experimental and human data. Environ. Res. 176, 108539. doi:10.1016/j.envres.2019.108539
Chavez, E., He, Z. L., Stoffella, P. J., Mylavarapu, R. S., Li, Y. C., Moyano, B., et al. (2015). Concentration of cadmium in cacao beans and its relationship with soil cadmium in southern Ecuador. Sci. Total Environ. 533, 205–214. doi:10.1016/j.scitotenv.2015.06.106
Chen, Z. S., Lee, G. J., and Liu, J. C. (2000). The effects of chemical remediation treatments on the extractability and speciation of cadmium and lead in contaminated soils. Chemosphere 41, 235–242. doi:10.1016/S0045-6535(99)00416-6
Chrastný, V., Komárek, M., Jrovcová, E., and Štíchová, J. (2008). A critical evaluation of the 0.05 M EDTA extraction of Pb from forest soils. Int. J. Environ. Anal. Chem. 88, 385–396. doi:10.1080/03067310701744283
Donisa, C., Mocanu, R., and Steinnes, E. (2003). Distribution of some major and minor elements between fulvic and humic acid fractions in natural soils. Geoderma 111, 75–84. doi:10.1016/S0016-7061(02)00254-9
Elliott, H. A., Liberati, M. R., and Huang, C. P. (1986). Competitive adsorption of heavy metals by soils. J. Environ. Qual. 15, 214–219. doi:10.2134/jeq1986.00472425001500030002x
Esringü, A., Turan, M., and Cangönül, A. (2021). Remediation of Pb and Cd polluted soils with fulvic acid. Forests 12, 1608–1613. doi:10.3390/F12111608
Essington, M. E. (2015). “Organic matter in soil,” in Soil and water chemistry : An integrative approach (Bosa Roca: CRC Press), 155–205.
Esteves Da Silva, J. C. G., and Oliveira, C. J. S. (2002). Metal ion complexation properties of fulvic acids extracted from composted sewage sludge as compared to a soil fulvic acid. Water Res. 36, 3404–3409. doi:10.1016/S0043-1354(02)00023-4
Gil, J. P., Leiva, E. I., and Ramirez, R. (2017). Phenology of cocoa tree in a tropical moist forest. Cientifica 45, 240–252. doi:10.15361/1984-5529.2017v45n3p240-252
Huang, M., Zhu, Y., Li, Z., Huang, B., Luo, N., Liu, C., et al. (2016). Compost as a soil amendment to remediate heavy metal-contaminated agricultural soil: Mechanisms, efficacy, problems, and strategies. Water Air Soil Pollut. 227, 359. doi:10.1007/s11270-016-3068-8
Islam, M. S., Ahmed, M. K., Habibullah-Al-Mamun, M., and Raknuzzaman, M. (2015). The concentration, source and potential human health risk of heavy metals in the commonly consumed foods in Bangladesh. Ecotoxicol. Environ. Saf. 122, 462–469. doi:10.1016/j.ecoenv.2015.09.022
Kabata-Pendias, A. (2000). “IV. Soil,” in Trace elements in soils and plants trace elements in soils and plants (Boca Raton: CRC Press), 38–39. doi:10.1201/9781420039900
Karaca, A. (2004). Effect of organic wastes on the extractability of cadmium, copper, nickel, and zinc in soil. Geoderma 122, 297–303. doi:10.1016/j.geoderma.2004.01.016
Karlsson, T., Persson, P., and Skyllberg, U. (2005). Extended X-ray absorption fine structure spectroscopy evidence for the complexation of cadmium by reduced sulfur groups in natural organic matter. Environ. Sci. Technol. 39, 3048–3055. doi:10.1021/es048585a
Li, P., Wang, X., Zhang, T., Zhou, D., and He, Y. (2008). Effects of several amendments on rice growth and uptake of copper and cadmium from a contaminated soil. J. Environ. Sci. 20, 449–455. doi:10.1016/S1001-0742(08)62078-1
Liu, H. J., Zhang, J. L., Christie, P., and Zhang, F. S. (2007). Influence of external zinc and phosphorus supply on Cd uptake by rice (Oryza sativa L.) seedlings with root surface iron plaque. Plant Soil 300, 105–115. doi:10.1007/s11104-007-9393-3
Liu, H., Zhang, T., Tong, Y., Zhu, Q., Huang, D., and Zeng, X. (2022). Effect of humic and calcareous substance amendments on the availability of cadmium in paddy soil and its accumulation in rice. Ecotoxicol. Environ. Saf. 231, 113186. doi:10.1016/j.ecoenv.2022.113186
Manouchehri, N., and Bermond, A. (2009). EDTA in soil science: A review of its application in soil trace metal investigations. Terr. Aquat. Environ. Technol. 3, 1–15. http://www.globalsciencebooks.info/Online/GSBOnline/images/0906/TAET_3(1&2)/TAET_3(1)1-15o.pdf.
Maskall, J., Whitehead, K., Gee, C., and Thornton, I. (1996). Long-term migration of metals at historical smelting sites. Appl. Geochem. J. Int. Assoc. Geochem. Cosmochem. 11, 43–51. doi:10.1016/0883-2927(95)00079-8
Maskall, J., Whitehead, K., and Thornton, I. (1995). Heavy metal migration in soils and rocks at historical smelting sites. Environ. Geochem. Health 17, 127–138. doi:10.1007/BF00126081
McLaughlin, M. J., Maier, N. A., Freeman, K., Tiller, K. G., Williams, C. M. J., and Smart, M. K. (1995). Effect of potassic and phosphatic fertilizer type, fertilizer Cd concentration and zinc rate on cadmium uptake by potatoes. Fertilizer Res. 40, 63–70. doi:10.1007/BF00749863
Mohamed, I., Ahamadou, B., Li, M., Gong, C., Cai, P., Liang, W., et al. (2010). Fractionation of copper and cadmium and their binding with soil organic matter in a contaminated soil amended with organic materials. J. Soils Sediments 10, 973–982. doi:10.1007/s11368-010-0199-1
Nelson, D., and Sommers, L. (1996). “Total carbon, organic carbon and organic matter,” in Methods of soil analysis. Part 3. Chemical Methods (Madison, WI: SSSA and ASA), 961–1010.
Nordberg, G. F. (2009). Historical perspectives on cadmium toxicology. Toxicol. Appl. Pharmacol. 238, 192–200. doi:10.1016/j.taap.2009.03.015
Nordberg, G., Jin, T., Bernard, A., Fierens, S., Buchet, J. P., Ye, T., et al. (2002). Low bone density and renal dysfunction following environmental cadmium exposure in China. AMBIO A J. Hum. Environ. 31, 478–481. doi:10.1579/0044-7447-31.6.478
Otto, W. H., Robert Carper, W., and Larive, C. K. (2001). Measurement of cadmium(II) and calcium(II) complexation by fulvic acids using 113Cd NMR. Environ. Sci. Technol. 35, 1463–1468. doi:10.1021/es991372e
Palansooriya, K. N., Shaheen, S. M., Chen, S. S., Tsang, D. C. W., Hashimoto, Y., Hou, D., et al. (2020). Soil amendments for immobilization of potentially toxic elements in contaminated soils: A critical review. Environ. Int. 134, 105046. doi:10.1016/j.envint.2019.105046
Pitumpe, P., Hettiarachchi, G., Rice, C., Dynes, J., Maurmann, L., Wang, J., et al. (2018). Sub-micron level investigation reveals the inaccessibility of stabilized carbon in soil microaggregates. Sci. Rep. 8, 16810–16813. doi:10.1038/s41598-018-34981-9
Popovic, O., Almås, A., Manojlovic, M., Muratović, S., and Singh, B. (2011). Chemical speciation and bioavailability of Cd, Cu, Pb and Zn in Western Balkan soils. Acta Agric. Scand. Sect. B - Soil & Plant Sci. 61, 730–738. doi:10.1080/09064710.2011.554424
Ramtahal, G., Umaharan, P., Hanuman, A., Davis, C., and Ali, L. (2019). The effectiveness of soil amendments, biochar and lime, in mitigating cadmium bioaccumulation in Theobroma cacao L. Sci. Total Environ. 693, 133563. doi:10.1016/j.scitotenv.2019.07.369
Ramtahal, G., Umaharan, P., Davis, C., Roberts, C., Hanuman, A., and Ali, L. (2022). Mitigation of cadmium uptake in theobroma cacao L: Efficacy of soil application methods of hydrated lime and biochar. Plant Soil. doi:10.1007/s11104-022-05422-4
Rashid, I., Murtaza, G., Zahir, Z. A., and Farooq, M. (2018). Effect of humic and fulvic acid transformation on cadmium availability to wheat cultivars in sewage sludge amended soil. Environ. Sci. Pollut. Res. 25, 16071–16079. doi:10.1007/s11356-018-1821-9
Rieuwerts, J. S., Thornton, I., Farago, M. E., and Ashmore, M. R. (1998). Factors influencing metal bioavailability in soils: Preliminary investigations for the development of a critical loads approach for metals. Chem. Speciat. Bioavailab. 10, 61–75. 10 no.2. doi:10.3184/095422998782775835
Robertsa, T. (2014). “Cadmium and phosphorous fertilizers: The issues and the science,” in Procedia engineering (Elsevier B.V.), 52–59. doi:10.1016/j.proeng.2014.09.012
Saar, R. A., and Weber, J. H. (1980). Comparison of spectrofluorometry and ion-selective electrode potentiometry for determination of complexes between fulvic acid and heavy-metal ions. Anal. Chem. 52, 2095–2100. doi:10.1021/ac50063a025
Scokart, P. O., Meeus-Verdinne, K., and De Borger, R. (1983). Mobility of heavy metals in polluted soils near zinc smelters. Water Air Soil Pollut. 20, 451–463. doi:10.1007/BF00208519
Sikora, L. J., and Moore-Kucera, J. (2014). Soil test methods from the southeastern United States. Athens, GA: University of Georgia, College of Agriculture, Experiment Stations, 180. Available at: http://www.clemson.edu/agsrvlb/sera6/MethodsManualFinalSERA6.pdf.
Smolders, E., and Mertens, J. (2013). “Cadmium BT - heavy metals in soils: Trace metals and metalloids in soils and their bioavailability,” in, ed. B. J. Alloway (Dordrecht: Springer Netherlands), 283–311. doi:10.1007/978-94-007-4470-7_10
Sparks, D. L. (2003). “Sorption phenomena on soils,” in Environmental soil chemistry (Academic Press), 99–139. Available at: https://www.sciencedirect.com/science/article/pii/B9780126564457500097.
Sposito, G., Lund, J., and Chang, A. (1982). Trace metal chemistry in arid-zone field soils amended with sewage sludge: I. Fractionation of Ni, Cu, Zn, Cd, and Pb in solid phases. SOIL Sci. Soc. Am. J. 46, 260–264. doi:10.2136/sssaj1982.03615995004600020009x
Staunton, S. (2002). Direct and indirect effects of organic matter on metal immobilisation in soil. Dev. Soil Sci. 28, 79–97. doi:10.1016/S0166-2481(02)80045-9
Swift, R. S. (1996). “Organic matter characterization,” in Methods of soil analysis. Part 3. Chemical methods. Soil sci. Soc. Am. Book series: 5. Editor D. L. Sparks, P. A. Helmke, R. H. Loeppert, P. N. Soltanpour, M. A. Tabatabai, C. T. Johnstonet al. (Madison, WI: Soil Sci. Soc. Am.), 1018–1020.
The European Commission (2014). Statement on tolerable weekly intake for cadmium. EFSA J. 9. doi:10.2903/j.efsa.2011.1975
Thomas, G. (1966). “Chemical methods,” in Methods of soil analysis. Part 3. Chemical methods (Madison, WI: SSSA), 739–765.
Thomas, G. (1996). “Soil pH and soil acidity,” in Methods of soil analysis. Part 3. Chemical methods (Madison, WI: SSSA), 475–490.
Udom, B. E., Mbagwu, J. S. C., Adesodun, J. K., and Agbim, N. N. (2004). Distributions of zinc, copper, cadmium and lead in a tropical ultisol after long-term disposal of sewage sludge. Environ. Int. 30, 467–470. doi:10.1016/j.envint.2003.09.004
Vanderschueren, R., Argüello, D., Blommaert, H., Montalvo, D., Barraza, F., Maurice, L., et al. (2021). Mitigating the level of cadmium in cacao products: Reviewing the transfer of cadmium from soil to chocolate bar. Sci. Total Environ. 781, 146779. doi:10.1016/j.scitotenv.2021.146779
Walker, D. J., Clemente, R., Roig, A., and Bernal, M. P. (2003). The effects of soil amendments on heavy metal bioavailability in two contaminated Mediterranean soils. Environ. Pollut. 122, 303–312. doi:10.1016/S0269-7491(02)00287-7
Walker, D. J., Clemente, R., and Bernal, M. P. (2004). Contrasting effects of manure and compost on soil pH, heavy metal availability and growth of Chenopodium album L. in a soil contaminated by pyritic mine waste. Chemosphere 57, 215–224. doi:10.1016/j.chemosphere.2004.05.020
Wang, J., Zhang, C. B., and Jin, Z. X. (2009). The distribution and phytoavailability of heavy metal fractions in rhizosphere soils of Paulowniu fortunei (seem) Hems near a Pb/Zn smelter in Guangdong, PR China. Geoderma 148, 299–306. doi:10.1016/j.geoderma.2008.10.015
Keywords: Cacao, cadmium, organic matter, fulvic acid (FA), humic acid (HA)
Citation: Gutiérrez E, Chávez E, Gamage KHH, Argüello D, Galkaduwa MB and Hettiarachchi GM (2022) Cadmium fractionation in soils affected by organic matter application: Transfer of cadmium to cacao (Theobroma cacao L.) tissues. Front. Environ. Sci. 10:954521. doi: 10.3389/fenvs.2022.954521
Received: 27 May 2022; Accepted: 08 August 2022;
Published: 19 September 2022.
Edited by:
Guanghui Yu, Tianjin University, ChinaCopyright © 2022 Gutiérrez, Chávez, Gamage, Argüello, Galkaduwa and Hettiarachchi. This is an open-access article distributed under the terms of the Creative Commons Attribution License (CC BY). The use, distribution or reproduction in other forums is permitted, provided the original author(s) and the copyright owner(s) are credited and that the original publication in this journal is cited, in accordance with accepted academic practice. No use, distribution or reproduction is permitted which does not comply with these terms.
*Correspondence: Ganga M. Hettiarachchi, ganga@ksu.edu