- 1Department of Biology and Ecology, Faculty of Science, University of Kragujevac, Kragujevac, Serbia
- 2Applied Ecology Research Center, Lucian Blaga University of Sibiu, Sibiu, Romania
- 3Department of Biology and Ecology, Faculty of Sciences and Mathematics, University of Niš, Niš, Serbia
This study examines the ecological sustainability of the Velika Morava River Basin (Serbia, Central Balkans) under modern conditions of multiple freshwater stressors, including climate change. The impact of stressors on the ecological services of the river basin is considered, including: drinking water, irrigation, recreation, tourism, ecotourism, and fishing. In order to assess the ecological sustainability of the river basin, a modification of the ESHIPPO model was performed. The essence of the modification is a change to the ES component, which, in the basic model, assesses the degree of ecological specialization of a taxon; and in the modified assessment of the ecological stability of the ecosystem (ESE). The structure of ichthyocenosis is used as the basic biological indicator for the assessment of ESE. The assessment of river basin sustainability was obtained as the difference between ESE and the impact of multiple factors, including: habitat change, invasive species, pollution, human population growth (social factors), and the over-exploitation of resources (HIPPO). The statistical analysis (SOM—Self Organizing Map) highlights the most reliable indicators of both biotic (ichthyocenosis structure elements) and the indicators that best detect the influence of HIPPO factors. The structure of the model is based on general and easily measurable indicators, which enables its application in any river basin in the world. The results of the model indicate that 80% of the studied basin is ecologically unsustainable and that its potential environmental services are greatly reduced.
Introduction
The ecological stability of river basins as aquatic macro-ecosystems in the era of the Anthropocene reflects both natural and anthropogenic influences, with their cumulative effects transmitted and accumulated downstream (Rockström et al., 2014a). Since a river basin, as a kind of “bloodstream”, permeates a land area of variable size, the ecological condition of the river basin largely reflects the state of the environment in that area.
Theoretically, the ecological stability and health of the main river basin should be a cumulative reflection of ecological stability of its tributarities and catchments. Fish communities within the river basin represent high levels of the food chain and, as such, are good indicators of long-term changes within the basin; and thus its overall stability and environmental sustainability (Canning and Death, 2018; Huang et al., 2022). The fish community structure dynamics of the Danube River basin, too, both in general and especially in its delta-sea interface area accurately reveal the present and historical impact of various natural and anthropogenic stressors on aquatic ecosystems (Simonović et al., 2010; Bănăduc et al., 2014, 2016, 2020, Simić et al., 2014a). Fish stocks offer traditional natural ecological river basin services, together with quality water for the local population (such as drinking water, crop irrigation water, and recreation) (Brugere et al., 2015).
River basin technological services, such as energy potential, river traffic, and technical water for industry and industrial agriculture, are factors that mainly reduce the quantity and quality of traditional environmental services. However, in the era of the Anthropocene, in which human populations have been growing, the need for increased use of traditional ecological services provided by aquatic ecosystems (fish resources, water resources) is constantly growing. Therefore, these activities are now unfavorable for preserving the ecological sustainability of river habitats and river basins as a whole (Petts et al., 2015).
Fish as indicators of aquatic habitat health are widely used through biotic ichthyological indices. This has been done in the USA (Karr, 1981, 1987) and, more recently, in EU countries (Jepsen and Pont, 2007; Pont et al., 2007). However, the universal application of ichthyological indices can still be hampered by regional and local differences in the structure of ichthyocenoses, complex definitions in terms of reference conditions, and different effective monitoring objectives (Stojković et al., 2011; Pitcher, 2015; Radinger et al., 2019). For the territory of Serbia, there has not been an application of the IBI index, however, the initial studies were conducted in Stojković et al. (2013).
The model that is the subject of this study is used in order to assess the overall ecological sustainability of a river basin. It generally starts from the hypothesis concerning the relationship between biodiversity, productivity, and ecosystem stability (Johnson et al., 1996). In this study, as an indicator of the ecological stability of aquatic lotic habitats, the time trend in terms of the basic characteristics of ichthyocenoses is used, such as species diversity, abundance, biomass, body length, and age class of dominant, frequent, autochthonous, and predatory fish species. The time trend was analyzed through the spatial dimension of the entire investigated river basin. The analyzed parameters of ichthyocenoses are, for the most part, easily accessible because they are globally presented in the state programs of fisheries management plans for inland waterways. In addition, scientific institutions primarily run management programs, so they are reliable data sources. These facts enable the global use of this model assuming that there are available data regarding the continuous state of the fish stocks.
The impact of multiple stressors on inland aquatic ecosystems was obtained by analyzing the intensity of the impact of basic factors influencing biodiversity and grouped according to the acronym HIPPO (Habitat alteration, Invasive species, Pollution, Population growth, and Overexploitation) (Brennan and Withgott, 2005). This analysis of the impact of HIPPO factors has proven to be very effective as an element of the ESHIPPO fish model in assessing the environmental sustainability and conservation priorities of commercially important fish and other aquatic species in inland waterways (Simić et al., 2007; Simić et al., 2014b; Simić et al., 2015).
In our new model, the ESE-HIPPOriver basin, the ecological sustainability of the river basin was obtained as the difference between the mathematically expressed value of the estimated ecological stability of the basin (ESE) as a complex aquatic macro-ecosystem and the total impact of multiple stressors (HIPPO factors). The model enables water and fish resource managers to identify the most endangered habitats in the basin area, target those with conservation measures, and adjust them to effectively eliminate or mitigate the impact of endangering factors (Arlinghaus et al., 2015).
Materials and methods
The new ESE-HIPPOriver basin model was designed on the example of the Morava River Basin (Serbia, Western Balkans) to assess the ecological sustainability of its river basins. The main characteristics of the Morava basin are shown in Figure 1 (Gavrilović and Dukić, 2002).
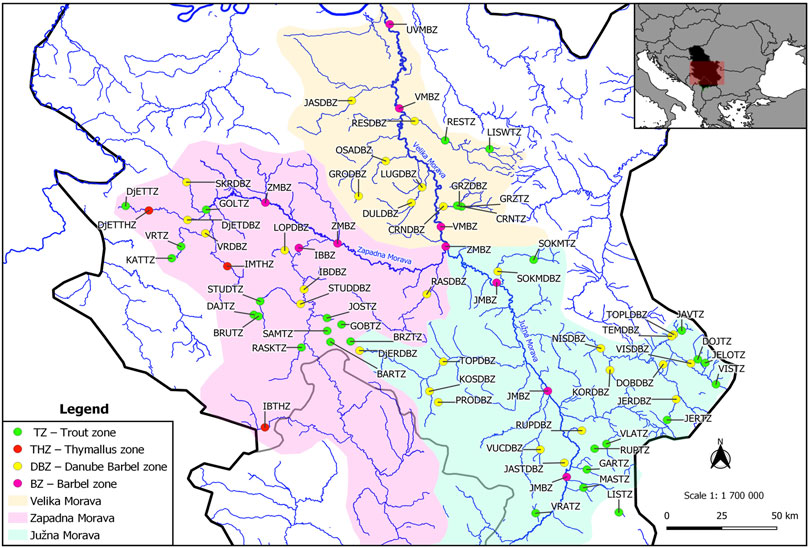
FIGURE 1. Map of analysed locations in the Velika Morava basin. Hydrological characteristics of the Velika Morava basin: The drainage basin of the Velika Morava is 6.126 km2, and of the whole Morava system is 38.207 km2 (of that, 1.237 km2 are in Bulgaria and 44 km2 are in the Republic of Macedonia). This drainage basin covers 42.38% the area of Serbia. The Zapadna Morava drains an area of 15,754 km2 (41.2% of the entire Velika Morava watershed). The Južna Morava has a drainage area of 15.696 km2, of which 1.237 km2 is in Bulgaria (through its right tributary Nišava) (Gavrilović and Dukić, 2002).
The primary data source for this study was hydrobiological and ichthyological research of the Morava river basin, conducted from 2001 to 2021. The research was conducted during the development of the fisheries management plans for the protection and the sustainable use of fish stocks (Supplementary Table S1) and it involves the monitoring of fish stocks that were done every third year in a given period. Locations for the conception of the ESE-HIPPOriver basin model are located on the lower, middle, and upper course of the main river (5) and the tributaries of second to forth order (classification according to Horton, 1945 and Strahler, 1957) (Figure 1).
In addition to ichthyological research conducted using standard procedures (Jepsen and Pont, 2007), basic physical and chemical parameters were measured at each site, and macrozoobenthos and periphyton samples were taken. Based on abiotic and biotic parameters (macroinvertebrates, diatoms), estimates of aquatic ecological status were obtained according to Water Framework Directive (WFD, 2000). In addition to these results, this paper also used data on the ecological status of waters in the Morava basin obtained from the RS Environmental Protection (http://www.sepa.gov.rs/). Based on all sources, the basic working database “Morava fishbase 2001–2021” was conceived and presented in Supplementary Table S2.
The implementation of the ESE-HIPPOriver basin model was carried out through an analysis of this database. In order to assess Ecological Stability of the Ecosystem values (an element of the ESE model), ichthyocenosis indicators were selected as indicated in Table 1. The evaluation of these indicators was performed using a three-point scale (1, 3, 5) according to the criteria shown in Table 2. In cases that there are lack of data for the evaluation of the indicators, alternative information are suggested in Table 1 (not evaluated for this study).
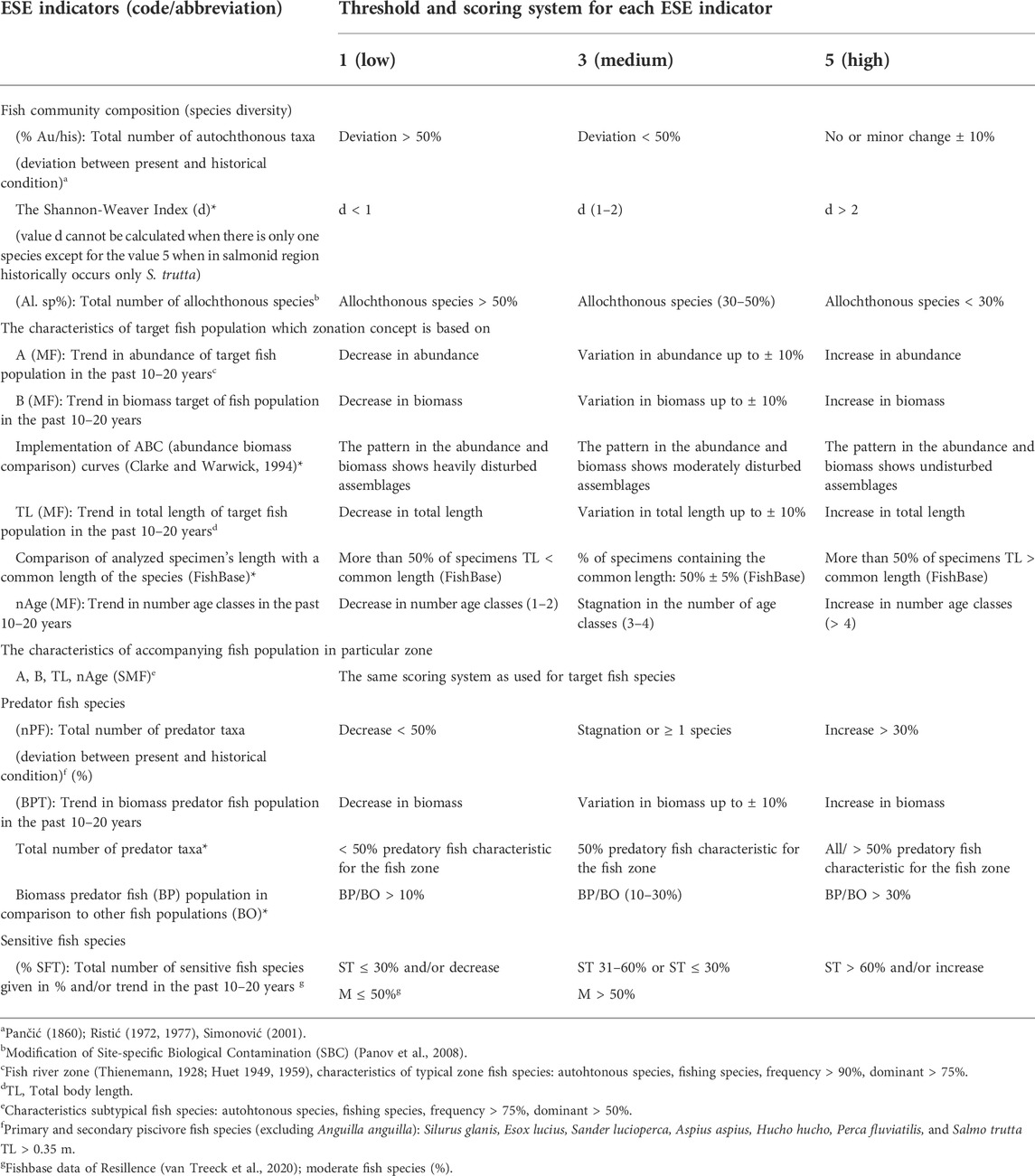
TABLE 1. ESE model indicators and criteria for their evaluation. In cases that there are lack of data for the evaluation of the indicators, alternative information for the evaluation are provided and marked with an asterisk.
A partially modified evaluation system as incorporated in the ESHIPPOfish model (Simić et al., 2014b) was used to assess the impact of HIPPO factors (H-habitat alternation, I-invasive species, P-pollution, P-population growth, and O-overexploitation). The HIPPO factor evaluation system for the ESE-HIPPO river basin model is presented in Table 2.
To visualize the effect of each HIPPO factor on fish communities, which is represented by several ESE elements, a Kohonen artificial neural network (i.e., self-organizing map—SOM; Kohonen, 1982, 2001) was applied. The SOM technique, an unsupervised learning algorithm, is commonly used in ecological studies as a powerful tool for the clustering and visualization of large data sets (Penczak et al., 2012; Stojković et al., 2013), and was thus found to be the most convenient tool for this study.
The input data matrix for the data analysis consisted of 21 ESE element (columns) and 76 samples (rows). The ESE values were log-transformed for the purposes of data normalization. As an output, a 6 × 6 two-dimensional map of hexagonal output units (neurons) was obtained, with the sites assigned to the neurons. Sites with a similar pattern of ESE elements were assigned to one particular neuron and its adjoining neurons, while sites that were significantly dissimilar in their ESE values were assigned to distant neurons, especially those belonging to two different clusters (Chon et al., 1996; Park et al., 2003, 2005; Penczak et al., 2012). The resolution of the SOM map was determined with respect to both rules proposed by Vesanto et al. (2000) and Park et al. (2003). Finally, to define the boundaries between clusters on the SOM map, the k-means method was used (Jain and Dubes, 1988). Moreover, the neurons were clustered according to their similarities using the unified distance matrix (U-matrix) method (Ultsch, 1993). Low intensity color areas on the U-matrix cover similar neurons, while high intensity areas divide neurons into different clusters (Park et al., 2005). In the next step of the SOM analysis, each HIPPO factor was included as a passive variable in the previously trained SOM map, which does not influence the ordination and clustering based on the active ones (Milošević et al., 2013). HIPPO factors were assigned to the SOM map as the mean values of each variable for each output neuron of the trained SOM, which was occupied by at least one input vector. We used the component planes technique to emphasize the relationship between metrics, disturbance variables, and the SOM map. The distribution of fish biomass data, metrics, and environmental and disturbance variables was presented in the form of a greyness gradient. A more detailed description of SOM methodology is presented in Stojković et al. (2013). The SOM analysis was conducted using the Matlab ver. 6.1.0.450 algorithm interface (http://www.cis.hut.fi/projects/som-toolbox).
The Kruskall–Wallis ANOVA and Mann–Whitney test were conducted post hoc to examine the differences between the mean values of the HIPPO factors among the clusters. The Kruskall–Wallis and Mann–Whitney tests were conducted in SPSS version 15.0 (SPSS Inc., Chicago, IL, United States).
To calculate the values of models and trends of ESE parameters, a programmed matrix was organized in the Microsoft Excel file (Supplementary Table S3). The Excel matrix is programmed to calculate ESE and HIPPO values for each investigated river basin (Rx1..n) according to the formulas:
ESERx1..n = ∑(AUFT + ALSP) + (AMF + BMF + TLMF + ACMF)+ (A,B,TL,AC SMF1,2,3 … n)+ (NPFS + BPFS) + SFT
HIPPORx1..n = ∑(H + I + P + P + O) or ∑(H + I + P + P + O + Clch1).
where 1 Clch-value was given points for climate change intensity if there is data for assessment, as follows:
1—direct effects of climate change on the aquatic ecosystem cannot be determined, but it can be indirectly assumed to have a weak impact (long-term average air temperature, frequency of “ecological droughts” or torrent).
3—moderate impact based on direct water temperature data.
5—strong impact based on direct water temperature data.
The level of ecological sustainability for each analyzed river “ES Rx1..n B” in a drainage is calculated based on the % of decrement of ∑ESE factors ∑HIPPO according to the formula:
ES Rx1..n B = % << ∑ESE/∑HIPPO or ∑(HIPPO + Clch).
and according to the scale provided in Table 3.
The total ecological sustainability for the basin (ESE-HIPPOriver basin) is assessed based on the percentage ratios of the length of river flows in relation to the total length of the basin and based on the obtained values of ESE-HIPPOriver basin according to the formula:
ESE-HIPPOriver basin = % (Total L km - L HS),
where total L km (total basin length) is calculated as ∑L Rx1..n.. Therefore L HS represents the total basin length where a high degree of environmental sustainability (HS) is estimated.
It is accepted that high sustainability (HS) is a condition that corresponds to the natural state of the ecosystem, i.e., the basin is ecologically sustainable if a high sustainability (HS) value is present at more than 50% of the length of the basin (Table 3). In both economic and practical terms, the length of the basin is also very important, in situations in which model-based sustainability is assessed as moderate, as it requires less extensive and expensive protection, conservation, or improvement measures.
Based on the frequency of parameter values expressed in points 1, 3, and 5, the Excel matrix calculated the ratios of decline, stagnation, or growth of the populations of typical and accompanying fish species indicator values and its facilitates the selected suitable graphical representations of results.
Results
Changes in the qualitative composition of the fish communities of the lotic habitats in the Morava basin were estimated based on available literature data from the earlier period (Pančić, 1860; Marković, 1962; Momirović, 1972; Ristić, 1972, 1977; Janković and Ćetković-Krpo, 1995; Simonović, 2001) and based on the results of our research over the past 20 years. The obtained results are shown in Table 4.
The data analysis from Table 4 informs the graph presented in Figure 2. In relation to the historical data, the total number of autochthonous fish species (47) in the Morava basin decreased by 21.3%. The loss of autochthonous species is greatest in the barbel zone (36.7%).
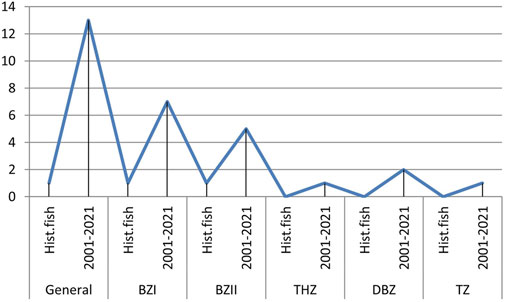
FIGURE 2. Number of autochthonous fish species in the Morava basin in the historical and current period calculated for the entire basin and by fish zones. BbzI—lower Barbus barbus zone; BbzII- Barbus barbus zone, middle and upper part; Thz-Thymallus thymallus zone, Dbz-Barbus balacanicus zone, TZ-Salmo trutta zone.
Figure 3 presents changes in the qualitative composition of the fish communities in the Morava basin due to an increase in the number of non-native fish species in the basin during the period from 2001 to 2021. Carassius gibelio (Pančić, 1860) is the oldest non-native species mentioned in the historical period. The real ratio of autochthonous and allochthonous fish species for the whole basin is 68.1: 31.9%, with the most prominent abundance of allochthonous species in the lower barbel zone.
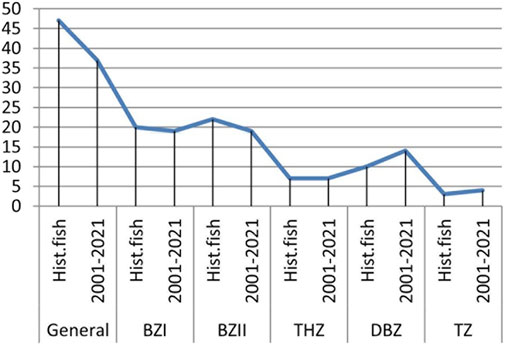
FIGURE 3. Presence of non-native fish species in the entire Morava basin and in fish zones (the meaning of abbreviations is the same as in Figure 2).
Figure 3 shows the presence of non-native fish species in the entire Morava basin and fish zones (the abbreviations are the same as in Figure 2).
The trained SOM map (Figures 4, 5), according to the U-matrix distances, distinguish two main Clusters, X and Y, which can be further divided into two sub-clusters labeled X1 and X2, and Y1 and Y2 (Figure 4). Cluster X is generally composed of samples from the Danube barbel zone (31), which also contains several samples from the trout (5), barbel (4), and grayling (3) zone. On the other side, Cluster Y mainly contains samples from the trout zone (31) and only a few samples from the Danube barbel zone (2). Furthermore, Cluster X differs from Cluster Y in terms of many elements of ESE (see Figure 5). However, the division into the subclusters also was the result of specific elements of ESE (autochthonous fish taxa, percentage of allochthonous species, percentage of sensitive fish taxa, standard length, abundance, biomass and age classes of accompanying fish species 2). In addition, Group X1 was characterized by high scores in terms for standard length, abundance, biomass, and age classes of accompanying fish species 3 in comparison to Group X2. On the other side, group Y1 was characterized by high scores for standard length, abundance, biomass, and age classes for accompanying fish species 1 in comparison to group X1.
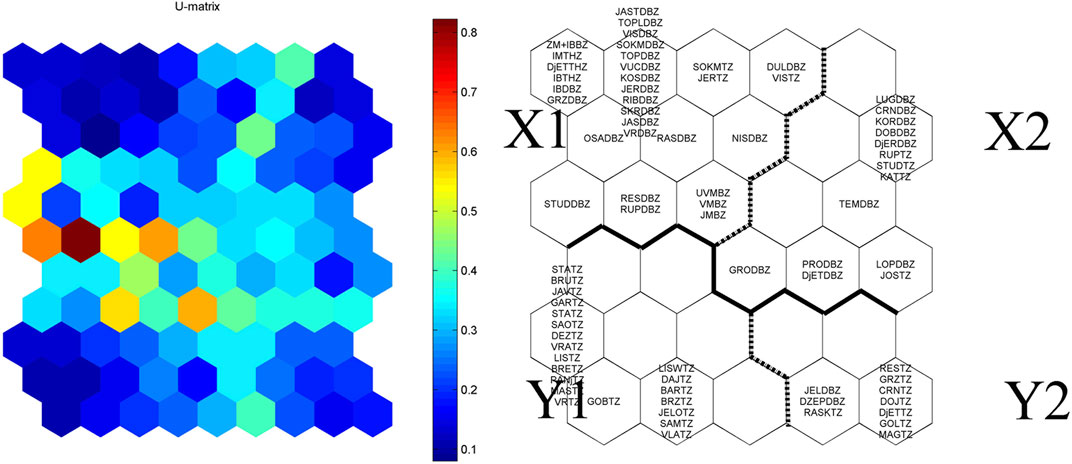
FIGURE 4. A SOM map formed by 30 hexagons representing neurons. Clusters of neurons (X1, X2, Y1, Y2) are distinguished on the basis of the U-matrix. The color intensity indicates the degree of dissimilarity.
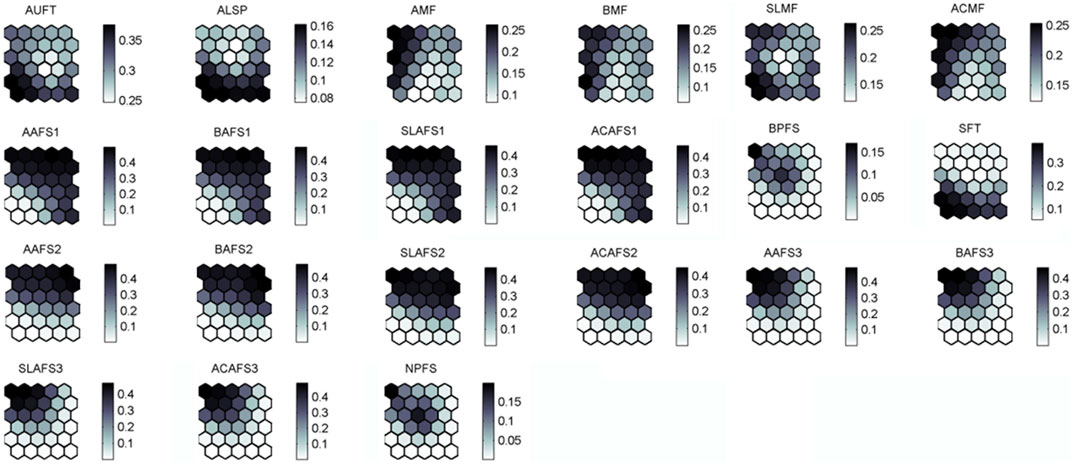
FIGURE 5. Visualization of a distribution patterns of 21 ESE elements in the previously trained SOM. The intensity of the gray color indicates species previously assigned scores.
In order to provide a more detailed presentation of the trend in terms of the indicator characteristics for various populations (A- abundance, B- biomass, TL - total body length, AC-age classes) of typical (main) fish species (Barbus barbus, Thymallus thymallus, Barbus balcanicus, and Salmo trutta) along the Morava basin in the period 2001–2021, Figures 6–13 graphically presented the results obtained with the % frequency of final scores (1, 3, 5) indicators.
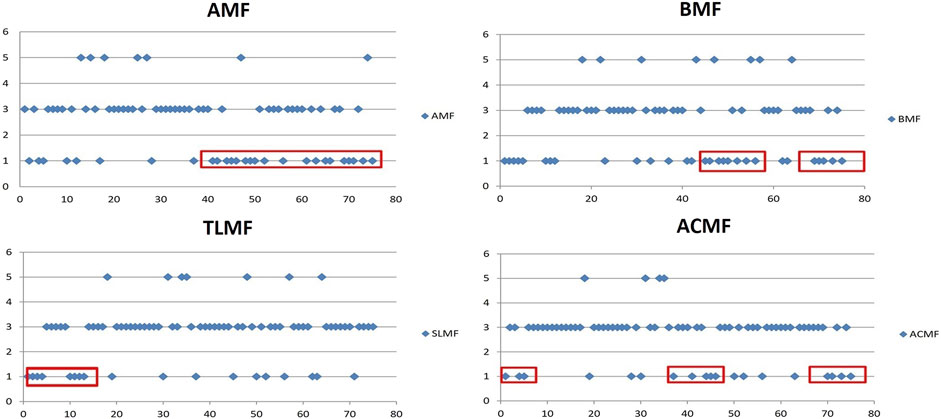
FIGURE 6. Trend of indicator characteristics of fish populations. Abbreviations: A, Abundance; B, Biomass; TL, Total Length; AC, Age Class. Value < 3 decrease, value > 3 increase. x, river fish zone (barbel 0–20, grayling 20–25, Danube barbel 25–55, and trout zone 55–80). AMF, Abundance of Main (typical) Fish species; BMF, Biomass of Main (typical) Fish species; TLMF, Total Length of Main (typical) Fish species; ACMF, Age Class of Main (typical) Fish species.
As shown in these figures, a small part of the basin has indicator values in the rise zone (indicator value 5), the largest part is in zone 3 (indicator value 3; stagnation), and a significant part is in the decline zone (indicator value 1). The abundance of typical fish species (AMF) significantly decreases in the Danube barbel, B. balcanicus and trout, S. trutta zone. In contrast, the biomass (BMF) decreases more significantly in the barbel zone, including B. barbus, Danube barbel, and trout. The value of the number of age classes (ACMF) has the same trend, while the total body length of fish (TLMF) decreases significantly in the barbel zone.
The analysis of the trend of indicator characteristics of the populations of each typical (main) fish species (Figures 6, 7) shows the largest decline in B. barbus and a moderate decline in S. trutta. Populations of T. thymallus are in the zone of stagnation, while the increase is observed only in B. balcanicus populations.
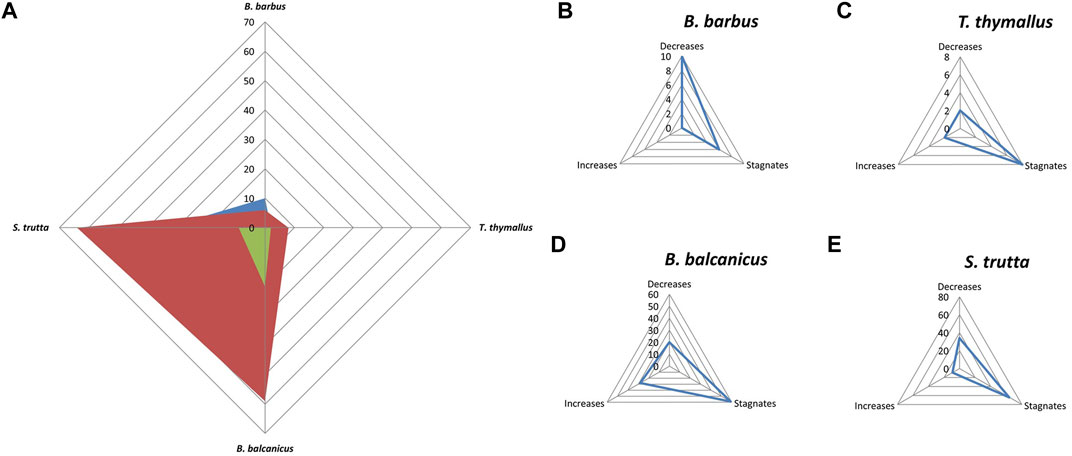
FIGURE 7. General trend of indicator characteristics of populations of typical fish species populations in the Morava basin.
The biomass of predatory fish species stagnates and decreases more than experiencing growth (Figure 8) in the barbel and grayling zones. Based on the monitored indicators, the population of Silurus glanis has the best characteristics in the lower part of the barbel zone (lower course of Velika Morava), Sander lucioperca in the middle and upper course of Velika Morava (barbel zone II), and Aspius aspius in the lower part of Južna Morava (barbel zone II). Esox lucius is found in barbel zones I and II, but with a more rapidly declining trend compared to other predators. Hucho hucho was introduced by restocking in all habitats and the population is currently growing only in the part of the course of the Ivanjička Moravica (IMTHZ) and covers the transition zones of Danube barbel and grayling.
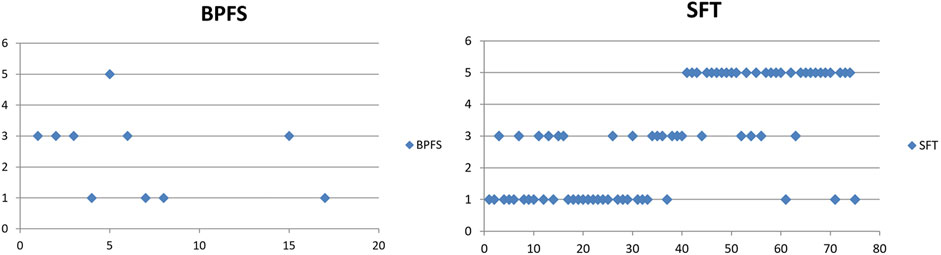
FIGURE 8. Trend of predatory fish species biomass (BPFS) and the presence of sensitive fish species (SFT) in the Morava basin for the period 2001–2021.
Figure 8 demonstrates that the presence of sensitive fish species decreases the most in the barbel zone and somewhat less in the zone of grayling and Danube barbel.
The analysis of the trend of indicator characteristics (A, B, TL, AC) of subtypical (accompanying) fish species by river basin zones are shown in Figures 9–11. In the barbel zone, C. nasus has the strongest indicator characteristics of populations in relation to other fish species (Figure 9).
Significant frequencies of Squalius cephalus and Alburnoides bipunctatus can be seen in the Danube barbel zone (Figure 10), as well as the overall declining trend of indicator characteristics of S. cephalus populations (Figure 10C), and increasing trend of A. bipuncatus (Figure 10B).
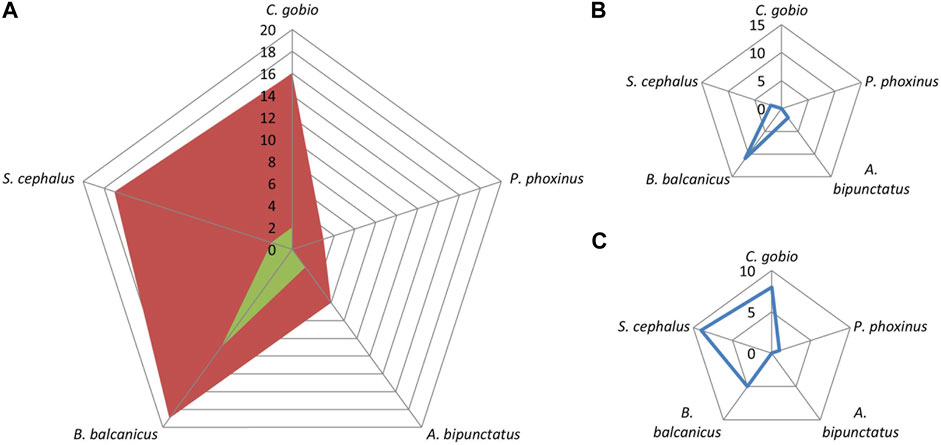
FIGURE 10. Frequency (A) and trend [increases—(B); decreases—(C)] of indicator characteristics of subtypic (accompanying) fish species in the Danube barbel zone.
In the brown trout zone (Figure 11), an expected significant frequency of Cottus gobio and B. balcanicus was detected (Figure 11A). Moreover, Phoxinus phoxinus has a declining trend (Figure 11C) compared to the increasing trend for B. balcanicus (Figure 11B).
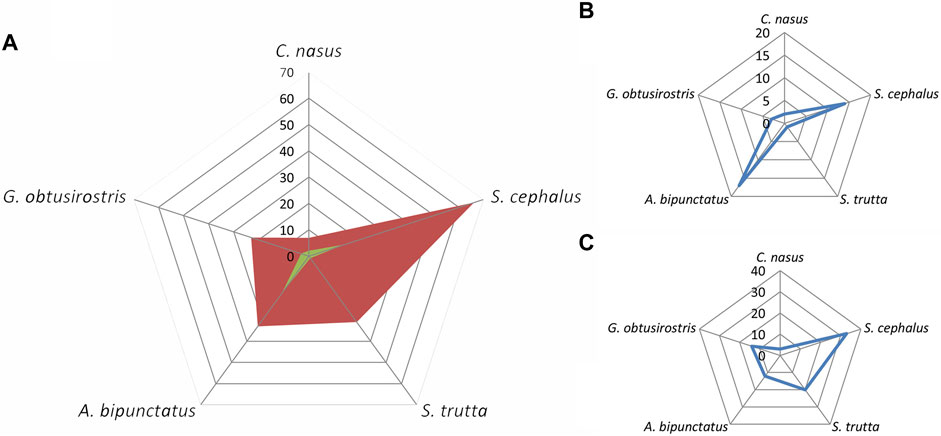
FIGURE 11. Frequency (A) and trend (increases—(B); decreases—(C)] of indicator characteristics of subtype (accompanying) fish species in the trout zone.
In the SOM network, from Cluster X to Cluster Y, the mean values of scores for all HIPPO factors decrease (Figure 12). The Kruskall–Wallis ANOVA test confirms significant differences among the clusters in all estimated HIPPO parameters, but the Mann–Whitney post hoc test reveals which clusters/subclusters are significantly different for each estimated parameter (Table 5).

FIGURE 12. Estimated HIPPO factors on the SOM map. The intensity of the gray color indicates the mean values of scores for each factor.
The influence of the HIPPO factor is also provided as a graphical representation of the frequency of given points awarded (Figure 13).
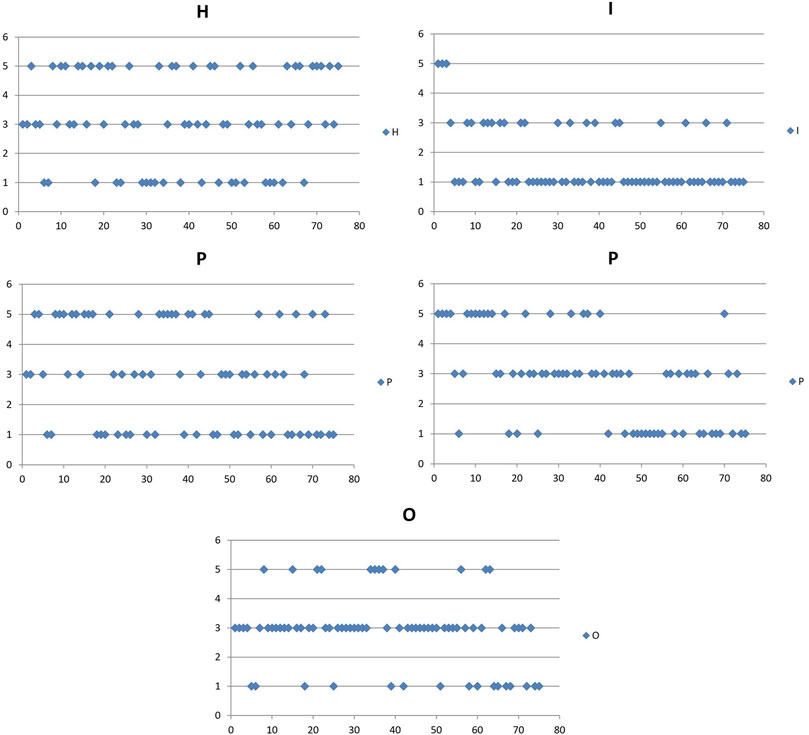
FIGURE 13. Graphic representation of the influence of HIPPO factors in the Morava basin. y - Degree of infuences of factors: 1- Low; 3-Moderate; 5 - High: x - river fish zone (barbel 0–20, grayling 20–25, Danube barbel 25–55 and trout zone 55–80).
Based on these analyses, we find that habitat changes have a significantly strong impact in the Danube barbel and trout zones, while the invasive species impact is the strongest in the lower barbel zone and more moderate in the Danube barbel and trout zones (in the trout zone due to genetic contamination). Pollution significantly impacts the barbel zone and part of the Danube barbel zone (lower parts of the fourth-order tributaries). The presence of humans and their permanent or seasonal activities are significant in the Danube barbel zone and some parts of the brown trout zone. According to these results, over-exploitation of fish resources has a predominantly moderate effect in all basin zones.
The final values produced by the ESE-HIPPOriver basin model for the Morava river basin are shown in Figure 14. If, for the purposes of sustainability reference values, it is considered that the theoretical assumption that aquatic ecosystems in their natural state are ecologically sustainable, then according to the model, the Morava river basin is sustainable along only 19.6 km of the length of the river. This percentage decreases if the climate change factor is introduced into the model (in this case, if the value is 1, which means that the factor impact is weak, primarily due to lack of data), and then the sustainability percentage decreases by about 5%. Analyzing the values produced by the ESE-HIPPOriver basin model by fish river zones (Figure 14), the highest level of sustainability was obtained for the grayling zone (50%), followed by brown trout (31.1%), slightly lower for the Danube barbel zone (21.8%), and lowest for the barbel zone (0%).
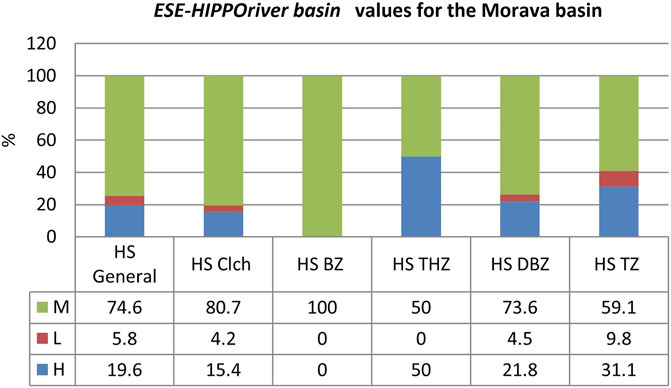
FIGURE 14. Final values of the ESE-HIPPOriver basin model for the Morava river basin (Serbia, Central Balkans). Abbreviations: H, high level of sustainability; M, medium level of sustainability; L, low level of sustainability. HS -% of the length of the catchment stream which has a high sustainability in relation to the total length. HS Clch -% HS under the influence of climate change. Th.zone - grayling zone, Thymallus thymallus zone; D.b.z—Danube barbel zone with: THZ - grayling zone, TZ ‐ trout zone, DBZ ‐ Danube barbel zone.
Discussion
Human activities in the Anthropocene era rapidly and efficiently reduce the natural ecological sustainability of inland water bodies in many ways, both directly and indirectly; including, for example, air pollution, soil pollution, deforestation, and accelerating climate change (Rockström et al., 2014b; Gozlan and Britton, 2015; Falkenmark et al., 2019). Long-term hydroecological and ichthyological research on streams in the Morava river basin (Serbia, Central Balkans) has shown that river health reflects the health of terrestrial ecosystems, air quality, and indirectly, the social status of human communities. In this study, the central indicator of the health of inland waters and the vast land area around them is the river basin. Here, the Morava basin study area covers 45% of the Serbian territory and therefore indicates the state of the environment of a significant part of the country.
The ESE-HIPPOriver basin model estimates the multiple impacts of stressors on the ecological sustainability of the river basin based on the fish communities. Fish are the top members of the food chain, so the structure and production of their populations reflects the ecological sustainability of the aquatic ecosystem (Arthington et al., 2004; Tejerina-Garro et al., 2005). For marine ecosystems, models assessing the sustainability of fish stocks and/or marine habitats are generally more developed than for inland waterways (Cooke et al., 2016). To assess the ecological status of inland waterways in the United States, IBIs were developed (Karr, 1981; 1987) and later modified for Europe and included in the WFD methodology (Jepsen and Pont, 2007; Pont et al., 2007). However, models assessing the ecological sustainability (stability, ecological efficiency, and production) of inland waterways and/or river basins are less well represented. In the context of our study, the work of Yang et al. (2021) is significant. The authors use elements of this approach to assess the structure of ichthyocenosis, such as alpha and beta diversity indices, and ABC (Abundance/Biomass Comparison) curves to assess community stability.
Based on field data, these authors presented the possible impacts of various factors on the fish community and river habitats, such as dams, pollution, and erosion. The ESE-HIPPOriver basin model is designed not just for scientific usage but also for practitioners (technicians, water and fish resources managers). For the latter, the developed system of three-point scoring of ichthyocenosis structure indicators (A- Abundance, B- Biomass, TL—Total body Length, and AC - Age Classes) significantly simplifies obtaining the final results of sustainability assessment, i.e., without the necessary use of complex mathematical and statistical methods. Therefore, in this work we compared the results obtained using SOM analysis and the results based on the simple addition of numerical values of indicators (points) in the active Excel matrix (Table 1; Supplementary Table S3) as a graphic representation.
The main specificity of the model on which the ecological sustainability of the basin is assessed, is the temporal and spatial trend of ichthyocenosis structure indicators. These indicators define the ecological stability of the ecosystem (ESE) as the first element of the model. The second set of indicators defines the impact of individual and cumulative effects of HIPPO factors, which reduce the ecological stability of ecosystems. The overall ecological stability of the basin estimated by the model results from the strength of the reduction of the ecological stability of the ecosystem by endangering factors.
However, one of the main problems of freshwater fisheries, the frequent lack of long-term catch statistics and other parameters important for the state of the fish stock, may cause the problem for the appropriate usage of the model. On the one hand, the concept of the model based on the trend of ichthyocenosis indicators should force users of the fisheries areas to introduce regular monitoring of fish stocks so that the results obtained on the basis of the model are reliable and useful. However, if such data are not available for any reason or the regular monitoring of fish stocks is not performed, the secondary parameters shown in Table 1 could be applied. These parameters refer to the results obtained on the basis of standard scientific methods of analysis of fish stocks.
The qualitative composition of fish in the Morava basin
There are not enough scientific and professional data to determine the exact historical qualitative composition of the Morava basin fish communities. From the oldest publication of Pančić (1860), the presence of fish species in the Morava basin can only be partially reconstructed. More precise information is provided in later publications (Ristić, 1972; Simonović, 2001; Stojković et al., 2013), with the useful data provided in professional publications on fishing and fishing waters of Serbia and the Morava basin (Marković, 1962; Momirović, 1972).
Based on the available data, it is possible to state that the loss of autochthonous species is about 20% in relation to the historical composition. Of the fishery-important species from the basin, the Crucian carp (Carassius carassius) and the tench (Tinca tinca) (Simić et al., 2013; Lujić et al., 2017) have disappeared or are very rare. There are also no new and reliable findings of species such as Alburnus chalcoides, Leucaspius delineatus, Leuciscus leuciscus, Pelecus cultratus, Zingel streber, Gymnocephalus baloni, Misgurnus fossilis, and Lota lota. In addition, the findings and catch of Acipenser ruthenus are extremely rare. Furthermore, the most significant changes and loss of autochthonous fish species are located in the lower parts of the Velika Morava.
Along with the loss of autochthonous species, the qualitative composition of the Morava basin ichthyofauna is changing with the expansion of allochthonous species, mainly from the Danube. The more substantial breach of autochthonous species occurred from 1960 to 1970 and 1990 to 2000 (Zorić et al., 2014). However, there is generally no detailed examination of non-native fish species in the Morava Basin. In Stojković et al. (2013), Lepomis gibbosus and Pseudoraspora parva are listed as frequent species. The results presented here confirm the frequency of these species, along with the frequent findings of the invasive Neogobius fluviatilis (Djikanović et al., 2013; Smederevac-Lalić et al., 2019). It is assumed that most allochthonous and allochthonous-invasive species in the lower course of the Velika Morava may also be found in the Danube. The Prussian carp (C. gibelio) is the only naturalized allochthonous species in the Morava basin previously mentioned in Pančić (1860).
Analysis of key population structure indicators of typical and accompanying fish species in the ichthyocenosis of the Morava basin and their significance for the ESE-HIPPOriver basin model
Results from this study are similar to those of Stojković et al. (2013). In their work, SOM analysis, which included the abiotic characteristics of habitats and fish communities in the Morava basin, detected three clusters. Clusters differed in their abiotic parameters and ichthyocenosis composition; and coincided with barbel, Danube barbel, and trout zones.
Our analysis revealed several unfavorable phenomena in the structure of populations of typical and accompanying fish species in the basin. In the barbel zone, the population of B. barbus as a typical species for this zone declined. At the same time, the population of Chondrostoma nasus remains stable and dominates this zone. According to van Treeck et al. (2020), the general sensitivity of B. barbus is higher (High) compared to C. nasus (Moderate). However, the sensitivity of both species to the spawning substrate is high. The estimated susceptibility is undoubtedly important for the population status of these species in our research. In the barbel zone, the influence of various endangering factors is present, but the exploitation of gravel and sand from the riverbed is the dominant phenomenon here, which reduces the suitable places for spawning B. barbus. In contrast, C. nasus has an advantage in terms of spawning because it migrates upstream and can still finds suitable places for spawning at the mouths of tributaries. For a successful spawning, C. nasus especially requires access to the mouths of tributaries of the Zapadna Morava and Ibar [Ribnica (RIBDBZ), Studenica (STUDDBZ), and Lopatnica (LOPDBZ)].
The grayling zone is the shortest in the Morava basin since its significant shortening occurred after 1970, when the grayling population disappeared from the river Studenica (left tributary of the Ibar River) (Janković, 2010). Marić et al. (2011) have shown that the populations of T. thymallus in the Ibar are the only autochthonous ones for the Morava basin. Therefore, they are especially important in terms of conservation issues. According to these authors, T. thymallus populations from Veliki Rzav (IBTHZ) and Ivanjička Moravica (IMTHZ) are allochthonous and originate from the waterways of Slovenia. Thymallus thymallus populations stagnate in all habitats except the river Djetinja (DjETTZ), where a slight increase has been recorded. However, there are no data on the genetic structure of grayling from the Djetinja river, which was also introduced by restocking.
The Danube barbel zone in the Morava basin is very heterogeneous, so, in addition to B. balcanicus as a typical species, there is also the frequent occurrence of S. cephalus, and brown trout (S. trutta). In this zone, range expansion and population growth are evident for Alburnoides bipunctatus, which, according to the classification system of van Treeck at al. (2020), is considered a resistant species.
In the trout zone, the typical species S. trutta has a smaller decrease in the indicator characteristics of the populations. At the same time, an increased presence of B. balcanicus is noted. Additionally, in this zone, the indicator characteristics of P. phoxinus populations are significantly declining.
Impact of multiple stressors on the ecological sustainability of the Morava basin
The ESE-HIPPOriver basin model allows the assessment of single and cumulative stressors on river basins. SOM analysis and graphical presentation of the influence of HIPPO factors on the Morava river basin show the unequal intensity of various factors’ influences on different river basin zones. Habitat changes and/or destruction vary in shape and extent throughout the basin. In the barbel zone, which includes the largest rivers in basins, regulation of the riverbed and land reclamation, as well as excavation of sand and gravel, are the main factors that change and degrade the river habitat. At the beginning of the 1960s, Velika Morava River flow was regulated to prevent floods, and the river was shortened by about 80 km. This greatly reduced the flood zone and lost natural spawning places for a large number of phytophilous fish species (Janković et al., 1972; Šorić, 1996; Vlahović et al., 2006). The consequences of this are the extinction of fish species such as T. tinca (Lujić et al., 2017) and C. carassius, and a significant population decline for Cyprinus carpio and Abramis brama. The flow of the Morava has been regulated for the past 15 years due to the construction of highways along the Južna and Zapadna Morava.
The exploitation of gravel from the Morava riverbed is an ongoing process that has accelerated since 2000. The increased need for this material is due to the economic improvement of Serbia, especially in the construction sector. The consequences of sand and gravel exploitation on river habitats and fish has been moderately studied, so Gavriletea (2017) and Koehnken and Rintoul (2018) pointed out that sand exploitation in the Missouri River (Kansas, USA) increases the population of lotic fish species and decreases rheophilic populations such as sturgeon. Similar results are reported by Paukert et al. (2008) and Freedman et al. (2013) for US rivers. However, they also point out the higher occurrence of non-native species in river areas affected by sand and gravel exploitation. Mingist and Gebremedhin (2016) in Ethiopian rivers (Arno-Garno, Pib) point to severe negative impacts of sediment mining on local fish populations due to the destruction of hatcheries and disruption of migratory routes. The study was not supported by quantitative data, but they were obtained by surveying quarry workers. Our research shows a quantitative increase in the number and biomass of resistant allochthonous fish species in areas where sand exploitation has been conducted, such as C. gibelio and L. gibbosus.
In the Morava basin, habitat fragmentation due to the construction of dams is most pronounced in the Danube barbel zone (tributaries of the third and fourth order). In the past 70 years, 225 accumulations of various purposes and capacities have been built. The smallest number of built dams (one) are on the main streams of the Velika, Zapadna, and Južna Morava in the barbel zone. In contrast, the number of barriers due to the construction of small hydropower plants in the trout zone has increased sharply in the last 10 years, and 46 derivation-type small hydropower plants have been built. The consequences of small hydropower plants on trout populations are the greatest. This is a significant cause of the declining trend of populations of this species in the Morava basin, which was obtained as a result of this study. Numerous studies both worldwide and in Serbia have demonstrated the negative consequences of dams on rivers for fish biodiversity and river ecosystems in general (Radojković et al., 2019; Ganassin et al., 2021; Khatun and Pal, 2021; Sousa et al., 2021). During our research, the impact of hydro-accumulations (especially large ones) on the downstream parts of rivers is stated, which can be called the “habitat inversion effect”. It is reflected in the permanent discharge of cold water from the accumulation downstream and the formation of areas with average colder water in relation to the average temperature of the river. These colder parts of the river are inhabited by trouts (S. trutta, Salmo macedonicus) and in some rivers form stable populations. Examples are the Sokobanjska Moravica River (SOKMTZ) under the Bovan reservoir, where the population was formed by stocking, and the Nišava River (NISDBZ) where, due to the influence of the Zavoj reservoir, the trout population is formed spontaneously from tributaries.
The influence of allochthonous and allochthonous-invasive species is most significant in the barbel zone in the lower part of the Velika Morava. This is because most non-native species that inhabit the Danube can be found in this part of the basin (Simonović et al., 2010). There are no stable populations of non-native species in other basin zones; however, they are located in reservoirs. Therefore, there is a real danger that some rheophilic invasive species, such as N. fluviatilis, will eventually settle in parts of rivers upstream of reservoirs. The penetration of invasive fish species into new habitats by Panov et al. (2008) is called biological contamination, and the penetration of allochthonous haplotypes of grayling and brown trout into the trout zone of the Morava basin can be described as genetic contamination.
The harmful effect of allochthonous haplotypes on indigenous populations of brown trout and grayling in the territory of Serbia, especially on their adaptive value and autochthonous genetic diversity, is indicated by previous studies (Marić et al., 2006, 2011, 2022; Tošić et al., 2016; Simonović et al., 2017; Kanjuh et al., 2021). Results show that around 7% of rivers are genetically contaminated in the Morava basin. In addition, trout populations in the Morava basin have autochthonous Danubian haplotypes contaminated with Atlantic and Adriatic haplotypes. Allochthonous haplotypes were mainly introduced by the stocking practices that took place intensively after the Second World War. During that period, scientific knowledge about the influence of foreign genes on the genetic diversity of indigenous species was not developed, so the material for restocking the rivers of the Morava basin, especially trout zones, was brought from more or less remote geographical areas (Lake Ohrid and rivers from Slovenia and Bosnia and Herzegovina). After that period, the rainbow trout (Oncorhynchus mykiss) was also used for restocking (Janković and Raspopović, 1960).
Pollution as a stressor is present in various forms and intensities in the entire Morava basin. The influence of this factor is most pronounced in the Danube barbel and the barbel zones. Organic pollution from human settlements and industry dominates in the Danube barbel zone, while mixed pollution (organic and toxic) is mainly present in the barbel zone. In addition to municipal and industrial wastewater pollution, the Morava basin is also significantly affected by water leached from agricultural areas because 2/3 of the basin is used for agricultural purposes. The process of eutrophication is manifested not only in the reservoirs of the basin but also in the river flows, especially in the Danube barbel and barbel zones. The phenomenon of “algae bloom” due to the excessive development of Cyanobacteria is represented in the reservoirs of the basin (Djordjević et al., 2015; Simić et al., 2017), but this phenomenon and its impact on fish has not been studied in the rivers of the basin. Results from the Australian rivers (Bowling and Baker, 1996; Bowling et al., 2013) have demonstrated that new cyanobacterial blooms in slow-flowing rivers occur during dry years and emphasize the fact that blooms are intensifying due to climate change. In the future, scientific research should be dedicated to the process of eutrophication and flowering of cyanobacteria, especially in the lower slow-flowing part of the Velika Morava.
In researching the impact of heavy metals on fish in the Morava basin (Milošković et al., 2016, 2022), barbel, bream, and catfish stand out as the species in which the highest concentration of heavy metals have been found. This study found an increased concentration of heavy metals in fish from Velika and Zapadna Morava. The study by Jovanović et al. (2018), based on genotoxicity tests, estimates that the total contamination by pollutants of the largest rivers of the Morava basin (Velika, Zapadna, and Južna Morava) is significantly correlated with results obtained through the use of the ESE-HIPPOriver basin model. Thus, the most substantial impact of pollution is found in Velika Morava, the lower part of the Južna, and the middle course of the Zapadna Morava (Figures 12, 13). The model detects that pollution as a factor has the strongest impact in the Morava basin on tributaries of the fourth order in zones that can be marked as the lower barbel zone. This zone has the highest percentage of habitats with a low level of environmental sustainability. During the research on the entire Morava basin, a significant presence of plastic waste was ascertained. Research on the impact of plastics, especially microplastics in marine aquatic ecosystems, is very relevant today but is relatively less well represented among freshwater studies (Parker et al., 2021). Moreover, no studies have been conducted on the impact of microplastics in the Morava basin.
The intensity of pollution as a factor for the ecological sustainability of the Morava basin is correlated with the population density. About two million people live in the basin area; the highest population density is in the Velika Morava basin (101 people/km2); slightly lower in the Zapadna Morava basin (87 people/km2), and the lowest in the Južna Morava basin (78 people/km2) (Vlahović et al., 2006). The general demographic trend in the basin area is depopulation. In contrast, in the last 10 years, economic growth in all sectors of the economy has increased (Manić and Lutovac, 2022). Agriculture is intensifying in areas that coincide with the barbel and lower Danube barbel zones, while the electric power industry and tourism coincide with the trout zone. The ESE-HIPPOriver basin detects a greater influence on the population through the quantity and quality of human activities than the peak of the average population density. Such an impact is indicative of the trout zone being located in the mountainous basin area. In the mountainous areas of the basin, the population density is the lowest, and the impact comes from mass tourism and the construction of small hydropower plants. Hydropower plants are automated and do not require large increases in the number of people for their operation, but the effect of their work on mountain river basins is very strong (Zhong and Power, 1996; Bunn and Arthington, 2002; Kemp, 2015). Studies of the impact of mass tourism on mountainous areas are mainly based on the global impact on the environment or the social aspect of the population and less on aquatic ecosystems, fish resources and fisheries (Demirović et al., 2017; Miller 2022). This study shows a strong influence of mass tourism in the Morava basin on visually attractive rivers, such as Lisinе waterfall (LISWTZ).
The excessive exploitation of resources in this study mostly refers to the data on the exploitation of fish resources in the Morava basin. In contrast, the use of water resources for water supply and irrigation is shown as a total effect. Until Second World War, fishing in the Morava basin was carried out in the form of commercial fishing on the Velika Morava and recreational fishing in the rest of the basin. Following this period, fishing was legally limited to recreational fishing, which is still the only form of fishing in the entire Morava basin. There are no data on fishing pressure in the Morava basin before the 1960s; however, if we compare the number of recreational fishermen from before 2003 of about 17,000 and after 2003 at around 28,000 (Janković et al., 1972; Supplementary Table S1), a significant increase is evident, theoretically leading to higher fishing pressure. However, these data cannot be taken as relevant because of the lack of accurate fishing statistics on annual catches for recreational fishermen by fisheries managers, which is a chronic problem for freshwater fisheries in Serbia and most of the world (Cooke et al., 2016; Burgin, 2017). Based on the analysis of indirect data such as biomass trends, the total length of fish stocks, age structure, and sensitivity of fish species to fishing (Supplementary Table S3), the model indicates moderate fishing pressure in the entire Morava basin. If this factor is viewed independent of other factors, it could be considered relatively acceptable and sustainable if we suppose that it is analyzed in conjunction with the generally declining values of indicators A, B, TL, and AC populations of fish species, the intensity of HIPPO factors, and climate change. In that case, it acts as an unfavorable factor that reduces the overall environmental sustainability of the basin. This cumulative effect of factors, including recreational and commercial fishing on river ecosystems, is also indicated in the previous work (Arlinghaus, 2005; Cooke and Cowx, 2006; Lewin et al., 2006; Carpenter et al., 2011). There is a weaker impact of recreational fishing in the trout zone than in the Danube barbel zone, and especially the barbel zone. We believe that the “catch and release” fishing regime in the Morava basin, the only permitted form of fishing in the trout zone, which has been around since 2013, has also contributed to this trend (Simić et al., 2016; Supplementary Table S1).
Excessive exploitation of fish stocks in the Morava basin is also affected by some provisions of the law (the Law on Protection and Sustainable Use of Fish Stocks, Official Gazette of RS, No. 128/2014) that define the organization of fishing. Namely, in the territory of Serbia, the large fisheries areas are managed by just one fisheries stakeholder. Such a system makes it difficult to manage (provide a sufficient number of fish keepers, control the prescribed fishing quotas) and effectively combat poaching, as a significant factor in over-exploitation, especially the fish species of commercial importance (catfish, pike, perch) (Mickiewicz et al., 2020). Therefore, we believe that poaching is a significant factor in disrupting the sustainability of inland water ecosystems. This problem is unfairly neglected in relation to illegal, unreported, and unregulated (IUU) seafood on in marine contexts (Vince et al., 2021; Bartolo, 2022). Also, recreational fishermen buy a single fishing license that is valid for the entire territory of Serbia. This enables the fishing waters with the best and most attractive fish stock to be visited and engaged in recreational fishing by most fishermen. Additionally, it significantly increases the allowable fishing pressure, which cannot be controlled. In the Morava basin, this phenomenon was constant at the largest reactors, especially the Velika and Zapadna Morava.
In this paper, due to the lack of long-term data, only the general impact of climate change on the ecological sustainability of the Morava River Basin is presented. The Republic Hydrometeorological service of Serbia (https://www.hidmet.gov.rs) reports shows an increase in the average air temperature for Serbia from 1981 to 2021, i.e., the climate is becoming warmer and with less precipitation on average (web: http://www.hidmet.gov.rs). This climate data was used in the model and quantified for the whole basin as existing but with a low impact (Impact Level 1). The obtained values of basin sustainability are about 5% lower than those without climate change factors, and more in the Danube barbel and trout zone. This result indicates the need for more extensive research in the future into the impact of climate change on the Morava basin. This research aims to assess the sensitivity of the basin to climate change in order to take mitigation measures (Harrod, 2015; Marković et al., 2017; Morales-Marin et al., 2019; Jarić et al., 2020).
Conclusion
River basins are the most widespread form of inland waterway but, despite their undoubted ecological and economic importance, comprehensive data on the state and sustainability are still significantly under-represented in relation to marine ecosystems.
The ESE-HIPPOriver basin model provides an assessment of the ecological stability of the lotic parts of the basin based on the trends of key indicators of fish populations within ichthyocenoses and the impact of multiple modern anthropogenic stressors. Our results essentially represent the degree of weakening of the ecological stability of the basin under the influence of multiple stressors in time and space.
The Morava basin occupies about 45% of the territory of Serbia and, according to the model, 80% of the basin has a low or medium level of sustainability in relation to the high level of sustainability, which is considered to be the natural state. The model indicates that the parts of the basin that belong to the trout zone and the Danube barbel zone are more sensitive and that, in a higher percentage and in a shorter period of time, they decline into a state of low sustainability. On the other hand, large river basins belonging to the barbel zone have greater resistance to multiple stressors and can maintain a moderate level of sustainability for a long time.
In terms of basin environmental services, only parts with a high level of sustainability also have economic viability. From these areas, it is possible to sustainably use healthy drinking water (without expensive purification treatments), healthy water for irrigation and fish farming, and healthy fish (fish without contamination of meat with various toxic substances). Also, these areas provide conditions for quality recreation and ecotourism.
Our model can assess the sustainability of each part of the basin and the basin as a whole. In this way, protection and/or conservation measures can be taken according to their priority, i.e. the first of all of the parts of the basin that are most endangered or most sensitive but under threat, or immediately on the entire basin.
In order to apply this model, it is necessary to establish continuous monitoring of indicators of ichthyocenosis structure and the assessment of stressor type and intensity. This necessity strongly relates to the managers of companies that use inland water resources to modernize and improve their management in order to preserve, restore or improve environmental services of inland waterways.
Data availability statement
The original contributions presented in the study are included in the article/Supplementary Materials, further inquiries can be directed to the corresponding authors.
Ethics statement
The animal study was reviewed and approved by the Branka Ognjanović, Faculty of Science, University of Kragujevac, Serbia; Ana Petrović, Faculty of Science, University of Kragujevac, Serbia; Miloš Matić, Faculty of Science, University of Kragujevac, Serbia; Vitomir Anđelković, Faculty of Science, University of Kragujevac, Serbia; Milanko Šekler, Veterinary Specialized Institute Kraljevo, Kraljevo, Serbia; Miloš Radaković, Institute for Nature conservation of Serbia.
Author contributions
VS wrote the original draft, AP, TV, SS, DB, AC-B, writing—review and editing. MS-P performed statistical analysis. All authors have read and agreed to the published version of the manuscript. All authors listed have made a substantial, direct, and intellectual contribution to the work and approved it for publication.
Funding
VS, AP, TV, and SS were supported by the Serbian Ministry of Education, Science and Technological Development (Agreement No. 451-03-68/2022-14/200122). MS-P. was supported by the Ministry of Education, Science and Technological Development, Republic of Serbia (Contract Number 451-03-68/2022-14/200124).
Conflict of interest
The authors declare that the research was conducted in the absence of any commercial or financial relationships that could be construed as a potential conflict of interest.
Publisher’s note
All claims expressed in this article are solely those of the authors and do not necessarily represent those of their affiliated organizations, or those of the publisher, the editors and the reviewers. Any product that may be evaluated in this article, or claim that may be made by its manufacturer, is not guaranteed or endorsed by the publisher.
Acknowledgments
The authors thank to Grant S. McCall (Center for Human-Environmental Research, New Orleans, LA, USA) for his English language checking of the manuscript. The authors also express their gratitude to Ecotur Sibiu Environmental Association (Sibiu, Romania), which paid the publication fee for this research paper.
Supplementary material
The Supplementary Material for this article can be found online at: https://www.frontiersin.org/articles/10.3389/fenvs.2022.952692/full#supplementary-material
References
Arlinghaus, R. (2005). A conceptual framework to identify and understand conflicts in recreational fisheries systems, with implications for sustainable management. Aquat. Resour. Cult. Dev. 1, 145–174. doi:10.1079/ARC200511
Arlinghaus, R., Lorenzen, K., Johnson, B. M., Cooke, S. J., and Cowx, I. G. (2015). “Management of freshwater fisheries,” in Freshwater fisheries ecology. Editor J. F. Craig (New Jersey, United States: John Wiley & Sons), 557–579. doi:10.1002/9781118394380.ch44
Arthington, A. H., Lorenzen, K., Pusey, B. J., Abell, R., Halls, A. S., Winemiller, K. O., et al. (2004). “River fisheries: Ecological basis for management and conservation,” in Proceedings of the second international symposium on the management of large rivers for fisheries (Bangkok: FAO), 21–60.
Bănăduc, D., Bănăduc, A., Lenhardt, M., and Guti, G. (2014). "Porţile de Fier/iron gates" Gorges area (Danube) fish fauna. Transylv. Rev. Syst. Ecol. Res. 16, 171–196. doi:10.1515/trser-2015-0041
Bănăduc, D., Joy, M., Olosutean, H., Afanasyev, S., and Curtean-Bănăduc, A. (2020). Natural and anthropogenic driving forces as key elements in the lower Danube basin-south-eastern carpathians-north-western black sea coast area lakes: A broken stepping stones for fish in a climatic change scenario? Environ. Sci. Eur. 32, 73. doi:10.1186/s12302-020-00348-z
Bănăduc, D., Rey, S., Trichkova, T., Lenhardt, M., and Curtean-Bănăduc, A. (2016). The lower Danube River-Danube delta-north west black sea: A pivotal area of major interest for the past, present and future of its fish fauna - a short review. Sci. Total Environ. 545-546, 137–151. doi:10.1016/j.scitotenv.2015.12.058
Bartolo, I. (2022). Guide to illegal, unreported and unregulated fishing (IUU). Edinburgh, United Kingdom: Sea Fish Industry Authority.
Bowling, L., and Baker, P. (1996). Major cyanobacterial bloom in the Barwon-Darling River, Australia, in 1991, and underlying limnological conditions. Mar. Freshw. Res. 47, 643. doi:10.1071/MF9960643
Bowling, L. C., Merrick, C., Swann, J., Green, D., Smith, G., Neilan, B. A., et al. (2013). Effects of hydrology and river management on the distribution, abundance and persistence of cyanobacterial blooms in the Murray River, Australia. Harmful Algae 30, 27–36. doi:10.1016/j.hal.2013.08.002
Brennan, S., and Withgott, J. (2005). “Biodiversity and conservation biology,” in Environment: The science behind the stories (San Francisco: Pearson-Bewamin Cummings).
Brugere, C., Lymer, D., and Bartley, D. M. (2015). Ecosystem services in freshwater fish production systems and aquatic ecosystems: Recognizing, demonstrating and capturing their value in food production and water management decisions. Geneva: TEEB Agriculture & Food, 1–22.
Bunn, S. E., and Arthington, A. H. (2002). Basic principles and ecological consequences of altered flow regimes for aquatic biodiversity. Environ. Manag. 30, 492–507. doi:10.1007/s00267-002-2737-0
Burgin, S. (2017). Indirect consequences of recreational fishing in freshwater ecosystems: An exploration from an Australian perspective. Sustainability 9, 280. doi:10.3390/su9020280
Canning, A., and Death, R. (2018). “Ecosystem health indicatorsin - freshwater environments,” in Encyclopedia of ecology. Editor B. Fath (Elsevier B.V. All), 46–60.
Carpenter, S. R., Stanley, E. H., and Vander Zanden, M. J. (2011). State of the world's freshwater ecosystems: Physical, chemical, and biological changes. Annu. Rev. Environ. Resour. 36, 75–99. doi:10.1146/annurev-environ-021810-094524
Chon, T.-S., Park, Y. S., Moon, K. H., and Cha, E. Y. (1996). Patternizing communities by using an artificial neural network. Ecol. Model. 90, 69–78. doi:10.1016/0304-3800(95)00148-4
Clarke, K. R., and Warwick, R. M. (1994). Change in marine communities: An approach to statistical analysis and interpretation. Swindon, United Kingdom: Natural Environment Research Council UK.
Cooke, S. J., Allison, E. H., Beard, T. D., Arlinghaus, R., Arthington, A. H., Bartley, D. M., et al. (2016). On the sustainability of inland fisheries: Finding a future for the forgotten. Ambio 45, 753–764. doi:10.1007/s13280-016-0787-4
Cooke, S. J., and Cowx, I. G. (2006). Contrasting recreational and commercial fishing: Searching for common issues to promote unified conservation of fisheries resources and aquatic environments. Biol. Conserv. 128, 93–108. doi:10.1016/j.biocon.2005.09.019
Demirović, D., Radovanović, M., Petrović, M., Cimbaljević, M., Vuksanović, N., Vuković, D., et al. (2017). Environmental and community stability of a mountain destination: An analysis of residents' perception. Sustainability 10, 70. doi:10.3390/su10010070
Djikanovic, V., Markovic, G., and Skoric, S. (2013). New record of Neogobius fluviatilis (pallas, 1814) (gobiidae) in the Danube River basin (Serbia). Arch. Biol. Sci. Belgra 65, 1469–1472. doi:10.2298/ABS1304469D
Djordjević, N., Simić, S., and Ćirić, A. (2015). First identification of the cylindrospermopsin (CYN)-producing cyanobacterium Cylindrospermopsis raciborskii (woloszyńska) seenayya & subba raju in Serbia. Fresenius Environ. Bull. 24, 3736–3742.
Falkenmark, M., Wang-Erlandsson, L., and Rockström, J. (2019). Understanding of water resilience in the Anthropocene. J. Hydrology X 2, 100009. doi:10.1016/j.hydroa.2018.100009
Freedman, J. A., Carline, R. F., and Stauffer, J. R. (2013). Gravel dredging alters diversity and structure of riverine fish assemblages. Freshw. Biol. 58, 261–274. doi:10.1111/fwb.12056
Ganassin, M. J. M., Muñoz-Mas, R., de Oliveira, F. J. M., Muniz, C. M., Dos Santos, N. C. L., García-Berthou, E., et al. (2021). Effects of reservoir cascades on diversity, distribution, and abundance of fish assemblages in three Neotropical basins. Sci. Total Environ. 778, 146246. doi:10.1016/j.scitotenv.2021.146246
Gavriletea, M. (2017). Environmental impacts of sand exploitation. Analysis of sand market. Sustainability 9, 1118. doi:10.3390/su9071118
Gavrilović, L. J., and Dukić, D. (2002). Reke Srbije. Beograd: Zavod za udzbenike i nastavna sredstva.
Gozlan, R. E., and Britton, J. R. (2015). “Sustainable freshwater fisheries,” in Freshwater fisheries ecology. Editor J. F. Craig (New Jersey, United States: John Wiley & Sons), 616–621. doi:10.1002/9781118394380.ch47
Harrod, C. (2015). “Climate change and freshwater fisheries,” in Freshwater fisheries ecology. Editor J. F. Craig (New Jersey, United States: John Wiley & Sons), 641–694. doi:10.1002/9781118394380.ch50
Horton, R. E. (1945). Erosional development of streams and their drainage basins; hydrophysical approach to quantitative morphology. Geol. Soc. Am. Bull. 56, 275–370. doi:10.1130/0016-7606(1945)56[275:edosat]2.0.co;2
Huang, X., Xu, J., Liu, B., Guan, X., and Li, J. (2022). Assessment of aquatic ecosystem health with indices of biotic integrity (IBIs) in the ganjiang river system, China. Water 14, 278. doi:10.3390/w14030278
Huet, M. (1949). Aperçu des relations entre la pente et les populations piscicoles des eaux courantes. Schweiz. z. Hydrol. 11, 332–351. doi:10.1007/bf02503356
Huet, M. (1959). Profiles and biology of Western European streams as related to fish management. Trans. Am. Fish. Soc. 88, 155–163. doi:10.1577/1548-8659(1959)88[155:pabowe]2.0.co;2
Jain, A. K., and Dubes, R. C. (1988). Algorithms for clustering data. Upper Saddle River, NJ: Prentice-Hall.
Janković, D., and Ćetković-Krpo, J. (1995). “Diverzitet slatkovodnih riba (Osteichthyes) i kolousta (Cephalaspidomorpha) Jugoslavije sa pregledom vrsta od medjunarodnog znacaja,” in Biodiverzitet jugoslavije. Editors V. Stevanović, and V. Vasić (Belgrade: Bioloski faultet i Ekolibri), 425–445.
Janković, D., Raspopović, M., and Mitrović-Tutundžić, V. (1972). Qualitative and quantitative study of fish fauna in the Velika Morava river. Ekologiia 7, 227–236.
Janković, D., and Raspopović, M. (1960). Ohridska pastrmka (Salmo letnica typicus K) pod izmenjenim ulovima u Vlasinskom baražnom jezeru. Arh. bioloških nauka XII, 117–122.
Jankovic, D. (2010). Serbia: Part of the south zone of the range of distribution of the European grayling (Thymallus thymallus L.). Arch. Biol. Sci. Belgra 62, 115–121. doi:10.2298/ABS1001115J
Jarić, I., Bellard, C., Courchamp, F., Kalinkat, G., Meinard, Y., Roberts, D. L., et al. (2020). Societal attention toward extinction threats: A comparison between climate change and biological invasions. Sci. Rep. 10, 11085. doi:10.1038/s41598-020-67931-5
Jepsen, N., and Pont, D. (2015). Intercalibration of fish-based methods to evaluate river ecological quality. Rep. E. U. intercalibration pilot Exerc. doi:10.13140/RG.2.1.5148.5608
Johnson, K. H., Vogt, K. A., Clark, H. J., Schmitz, O. J., Vogt, D. J., and Vogt, J. D. (1996). Biodiversity and the productivity and stability of ecosystems. Trends Ecol. Evol. 11, 372–377. doi:10.1016/0169-5347(96)10040-9
Jovanović, J., Kolarević, S., Milošković, A., Radojković, N., Simić, V., Dojčinović, B., et al. (2018). Evaluation of genotoxic potential in the Velika Morava river basin in vitro and in situ. Sci. Total Environ. 621, 1289–1299. doi:10.1016/j.scitotenv.2017.10.099
Kanjuh, T., Tomić, S., Marić, A., Jurlina, D. Š., Nikolić, V., and Simonović, P. (2021). Trout Salmo spp. (Salmoniformes: Salmonidae) molecular diversity in streams on the southern slopes of the stara planina mts. in Serbia. Acta Zool. Bulg. 73, 425–429.
Karr, J. R. (1981). Assessment of biotic integrity using fish communities. Fisheries 6, 21–27. doi:10.1577/1548-8446(1981)006<0021:aobiuf>2.0.co;2
Karr, J. R. (1987). Biological monitoring and environmental assessment: A conceptual framework. Environ. Manag. 11, 249–256. doi:10.1007/BF01867203
Kemp, P. S. (2015). “Impoundments, barriers and abstractions,” in Freshwater fisheries ecology. Editor J. F. Craig (New Jersey, United States: John Wiley & Sons), 717–769. doi:10.1002/9781118394380.ch52
Khatun, R., and Pal, S. (2021). Effects of hydrological modification on fish habitability in riparian flood plain river basin. Ecol. Inf. 65, 101398. doi:10.1016/j.ecoinf.2021.101398
Koehnken, L., and Rintoul, M. (2018). Impacts of sand mining on ecosystem structure, process and biodiversity in Rivers. Gland, Switzerland: WWF, 1–162.
Kohonen, T. (1982). Self-organized formation of topologically correct feature maps. Biol. Cybern. 43, 59–69. doi:10.1007/bf00337288
Kohonen, T. (2001). Self-organizing maps. Third extended edn. Berlin: Springer, 502. doi:10.1007/978-3-642-56927-2Self-organizing maps
Lewin, W.-C., Arlinghaus, R., and Mehner, T. (2006). Documented and potential biological impacts of recreational fishing: Insights for management and conservation. Rev. Fish. Sci. 14, 305–367. doi:10.1080/10641260600886455
Lujić, J., Kohlmann, K., Kersten, P., Marinović, Z., Ćirković, M., Simić, V., et al. (2017). Phylogeographic identification of tench Tinca tinca (L., 1758) (Actinopterygii: Cyprinidae) from the Northern Balkans and adjacent regions and its implications for conservation. Zool. Stud. 56, e3. doi:10.6620/ZS.2017.56-03
Manić, E., and Lutovac, M. (2022). “Natural resources and manufacturing sector,” in The geography of Serbia. Editors E. Manić, V. Nikitović, and P. Djurović (Cham): Springer), 207–220.
Marić, S., Razpet, A., Nikolić, V., and Simonović, P. (2011). Genetic differentiation of European grayling (Thymallus thymallus) populations in Serbia, based on mitochondrial and nuclear DNA analyses. Genet. Sel. Evol. 43, 2. doi:10.1186/1297-9686-43-2
Marić, S., Stanković, D., Sušnik Bajec, S., Vukić, J., Šanda, R., Stefanov, T., et al. (2022). Perils of Brown trout (Salmo spp.) mitigation-driven translocations: A case study from the vlasina plateau, southeast Serbia. Biol. Invasions 24, 999–1016. doi:10.1007/s10530-021-02688-0
Marić, S., Sušnik, S., Simonović, P., and Snoj, A. (2006). Phylogeographic study of Brown trout from Serbia, based on mitochondrial DNA control region analysis. Genet. Sel. Evol. 38 (4), 411. doi:10.1186/1297-9686-38-4-411
Markovic, D., Carrizo, S. F., Kärcher, O., Walz, A., David, J. N. W., and David, J. N. W. (2017). Vulnerability of European freshwater catchments to climate change. Glob. Change Biol. 23, 3567–3580. doi:10.1111/gcb.13657
Mickiewicz, M., Wołos, A., and Trella, M. (2020). Characteristics of illegal inland fishing according to the opinions of Polish fisheries guards. Fish. Aquat. Life 28, 125–132. doi:10.2478/aopf-2020-0015
Miller, K. M. (2022). Disentangling tourism impacts on small-scale fishing pressure. Mar. Policy 137, 104960. doi:10.1016/j.marpol.2022.104960
Milošević, D., Simić, V., Stojković, M., Čerba, D., Mančev, D., Petrović, A., et al. (2013). Spatio-temporal pattern of the chironomidae community: Toward the use of non-biting midges in bioassessment programs. Aquat. Ecol. 47, 37–55. doi:10.1007/s10452-012-9423-y
Milošković, A., Dojčinović, B., Kovačević, S., Radojković, N., Radenković, M., Milošević, D., et al. (2016). Spatial monitoring of heavy metals in the inland waters of Serbia: A multispecies approach based on commercial fish. Environ. Sci. Pollut. Res. 23, 9918–9933. doi:10.1007/s11356-016-6207-2
Milošković, A., Piperac, M. S., Kojadinović, N., Radenković, M., Đuretanović, S., Čerba, D., et al. (2022). Potentially toxic elements in invasive fish species Prussian carp (Carassius gibelio) from different freshwater ecosystems and human exposure assessment. Environ. Sci. Pollut. Res., 1–13. doi:10.21203/rs.3.rs-851568/v1
Mingist, M., and Gebremedhin, S. (2016). Could sand mining be a major threat for the declining endemicLabeobarbusspecies of Lake Tana, Ethiopia? Singap. J. Trop. Geogr. 37, 195–208. doi:10.1111/sjtg.12150
Morales-Marín, L. A., Rokaya, P., Sanyal, P. R., Sereda, J., and Lindenschmidt, K. E. (2019). Changes in streamflow and water temperature affect fish habitat in the Athabasca River basin in the context of climate change. Ecol. Model. 407, 108718. doi:10.1016/j.ecolmodel.2019.108718
Panov, V. E., Alexandrov, B., Arbaciauskas, K., Binimelis, R., Gordon, H., Copp, G. H., et al. (2008). Interim protocols for risk assessment of aquatic invasive species introductions via European inland waterways. Mumbai, Maharashtra: Invasions Module (Aquatic Group.
Park, Y.-S., Céréghino, R., Compin, A., and Lek, S. (2003). Applications of artificial neural networks for patterning and predicting aquatic insect species richness in running waters. Ecol. Model. 160, 265–280. doi:10.1016/S0304-3800(02)00258-2
Park, Y., Oberdorff, T., and Lek, S. (2005). “Patterning riverine fish assemblages using an unsupervised neural network,” in Modelling community structure in freshwater ecosystems. Editors S. Lek, M. Scardi, P. Verdonschot, J. P. Descy, and Y. S. Park (Berlin): Springer), 43–53.
Parker, B., Andreou, D., Green, I. D., Britton, J. R., and Britton, R. (2021). Microplastics in freshwater fishes: Occurrence, impacts and future perspectives. Fish. Fish. 22, 467–488. doi:10.1111/faf.12528
Paukert, C., Schloesser, J., Fischer, J., Eitzmann, J., Pitts, K., and Thornbrugh, D. (2008). Effect of instream sand dredging on fish communities in the Kansas river USA: Current and historical perspectives. J. Freshw. Ecol. 23, 623–633. doi:10.1080/02705060.2008.9664250
Penczak, T., Głowacki, Ł., Kruk, A., and Galicka, W. (2012). Implementation of a self-organizing map for investigation of impoundment impact on fish assemblages in a large, lowland river: Long-term study. Ecol. Model. 227, 64–71. doi:10.1016/j.ecolmodel.2011.12.006
Petts, G., Gosselin, M.-P., and Gray, J. (2015). “The dynamics of rivers in relation to fishes and fisheries,” in The dynamics of rivers in relation to fishes and fisheriesFreshwater Fisheries Ecology. Editor J. F. Craig (New Jersey, United States: John Wiley & Sons), 9–30. doi:10.1002/9781118394380.ch3
Pitcher, T. J. (2015). “Assessment and modelling in freshwater fisheries,” in Freshwater fisheries ecology. Editor J. F. Craig (New Jersey, United States: John Wiley & Sons), 483–499. doi:10.1002/9781118394380.ch38
Pont, D., Hugueny, B., and Rogers, C. (2007). Development of a fish-based index for the assessment of river health in Europe: The European Fish Index. Fish. Manage 14, 427–439. doi:10.1111/j.1365-2400.2007.00577.x
Radinger, J., Britton, J. R., Carlson, S. M., Magurran, A. E., Alcaraz‐Hernández, J. D., Almodóvar, A., et al. (2019). Effective monitoring of freshwater fish. Fish. Fish. 20, faf.12373. doi:10.1111/faf.12373
Radojković, N., Marinović, Z., Milošković, A., Radenković, M., Đuretanović, S., Lujić, J., et al. (2019). Effects of stream damming on morphological variability of fish: Case study on large spot barbell. Barbus Balc. Turk. J. Fish. Aquat. Sci. 19, 231–239. doi:10.4194/1303-2712-v19_03_0610.4194/1303-2712-v19_3_06
Ristić, M. (1972). Rasprostranjenost riba u vodama Srbije", u Lov i ribolov u Srbiji. Beograd): B Djuričić Borba, 201–210.
Rockström, J., Falkenmark, M., Allan, T., Folke, C., Gordon, L., Jägerskog, A., et al. (2014a). The unfolding water drama in the Anthropocene: Towards a resilience‐based perspective on water for global sustainability. Ecohydrol. 7, 1249–1261. doi:10.1002/eco.1562
Rockström, J., Falkenmark, M., Folke, C., Lannerstad, M., Barron, J., Enfors, E., et al. (2014b). Water resilience for human prosperity. Cambridge, United Kingdom: Cambridge University Press.
Simić, S. B., Đorđević, N. B., and Milošević, D. (2017). The relationship between the dominance of Cyanobacteria species and environmental variables in different seasons and after extreme precipitation. fal 190, 1–11. doi:10.1127/fal/2017/0975
Simić, V., Lujić, J., Kostić, D., Ćirković, M., Bjelić-Čabrilo, O., Simić, S., et al. (2013). Diversity characteristics of the fish species important for fishery in the waters of Serbia. Bulg. J. Agr. Sci. 19, 77–87.
Simić, V., Maguire, I., Rajković, M., and Petrović, A. (2015). Conservation strategy for the endangered crayfish species of the family astacidae: The ESHIPPO crayfish model. Hydrobiologia 760, 1–13. doi:10.1007/s10750-015-2295-0
Simić, V. M., Simić, S. B., Stojković Piperac, M., Petrović, A., and Milošević, D. (2014b). Commercial fish species of inland waters: A model for sustainability assessment and management. Sci. Total Environ. 497-498, 642–650. doi:10.1016/j.scitotenv.2014.07.092
Simić, V., Petrović, A., Erg, B., Dimović, D., Makovinska, J., Karadžić, B., et al. (2014a). “Indicative status assessment, biodiversity conservation and protected areas within the Sava River Basin,” in The sava river: The handbook of environmental chemisrty. Editors M. Milačič, J. Ščančar, and M. Paunović (Berlin/Heidelberg, Germany: Springer), 490.
Simić, V., Simić, S., Paunović, M., and Cakić, P. (2007). Model of the assessment of the critical risk of extinction and the priorities of protection of endangered aquatic species at the national level. Biodivers. Conserv. 16, 2471–2493. doi:10.1007/s10531-006-9130-x
Simić, V., Simić, S., Paunović, M., Radojković, N., Petrović, A., Talevski, T., et al. (2016). The Alburnus benthopelagic fish species of the Western Balkan Peninsula: An assessment of their sustainable use. Sci. Total Environ. 540, 410–417. doi:10.1016/j.scitotenv.2015.07.030
Simonović, P. (2001). Ribe Srbije. Beograd: Zavod za zaštitu prirode Srbije i Biološki fakultet Univerziteta u Beogradu.
Simonović, P., Simić, V., Nikolić, V., and Marić, S. (2010). "Various aspects of water status of the Danube River and its tributaries (the Sava, Tisa and Velika Morava) in Serbia analyzed after the structure of fish communities assessed from the samples taken during the JDS2 expedition", in eds. P. Paunović, V. Simonović, and S. Simić, Danube in Serbia – joint Danube survey 2. (Ministry of agriculture, forestry and water management – directorate for water, University of Kragujevac, Faculty of Science, Institute for Biology and Ecology, University of Belgrade, Institute for Biological Research “Siniša Stanković”, Belgrade), 225–241.
Simonović, P., Tošić, A., Škraba Jurlina, D., Nikolić, V., Piria, M., Tomljanović, T., et al. (2017). Diversity of Brown trout Salmo cf. trutta in the River Danube basin of Western Balkans as assessed from the structure of their mitochondrial control region haplotypes. J. Ichthyol. 57, 603–616. doi:10.1134/S0032945217040154
Smederevac-Lalić, M., Regner, S., Lenhardt, M., Nikolić, D., Cvijanović, G., Jaćimović, D., et al. (2019). Review of allochthonous fish species with the marine origin in Serbian freshwater system. Stud. Mar. 32, 33–46. doi:10.5281/zenodo.3274548
Šorić, V. (1996). Ihtiofauna reke Gruže, pritoke Zapadne Morave (Dunavski sliv) I. Reproduktivni potencijal vrsta Leuciscus cephalus, Alburnus alburnus i Rutilus rutilus. Ichtyologia 28, 1–14.
Sousa, R. G. C., de Freitas, H. C. P., Zacardi, D. M., and Faria-Junior, C. H. (2021). Effects of river dams on the fish guilds in the northwest region of the Brazilian Amazon. Fish. Res. 243, 106091. doi:10.1016/j.fishres.2021.106091
Stojković, M., Milosević, Dj., and Simić, V. (2011). Ichthyological integral indices, the history of development and possible application on rivers in Serbia. Biol. Nyssana 2, 59–66.
Stojković, M., Simic, V., Milosevic, D., Mancev, D., and Penczak, T. (2013). Visualization of fish community distribution patterns using the self-organizing map: A case study of the great Morava river system (Serbia). Ecol. Model. 248, 20–29. doi:10.1016/j.ecolmodel.2012.09.014
Strahler, A. N. (1957). Quantitative analysis of watershed geomorphology. Trans. AGU 38, 913. doi:10.1029/tr038i006p00913
Tejerina-Garro, F. L., Maldonado, M., Ibañez, C., Pont, D., Roset, N., Oberdorff, T., et al. (2005). Effects of natural and anthropogenic environmental changes on riverine fish assemblages: A framework for ecological assessment of rivers. Braz. Arch. Biol. Technol. 48, 91–108. doi:10.1590/S1516-89132005000100013
Thienemann, A. (1928). Der Sauerstoff in eutrophen und oligotrophen Seen: Ein Beitrag jursestypenlehre. Die Binnengewasser IV. Stuttgart: E. Echweizerbart.
Tošić, A., Škraba, D., Nikolić, V., Čanak Atlagić, J., Mrdak, D., Simonović, P., et al. (2016). Haplotype diversity of Brown trout Salmo trutta (L.) in the broader Iron Gate area. Turk. J. Zool. 40, 655–662. doi:10.3906/zoo-1510-54
Ultsch, A. (1993). “Self-organizing neural networks for visualisation and classification,” in Information and classification. Editors O. Opitz, B. Lausen, and R. Klar (Berlin): Springer), 307–313. doi:10.1007/978-3-642-50974-2_31
van Treeck, R., Van Wichelen, J., and Wolter, C. (2020). Fish species sensitivity classification for environmental impact assessment, conservation and restoration planning. Sci. Total Environ. 708, 135173. doi:10.1016/j.scitotenv.2019.135173
Vesanto, J., Himberg, J., Alhoniemi, E., and Parhankangas, J. (2000). Technical report A57. Helsinki: Neural Network Research Centre, Helsinki University of Technology. Available at: http://www.cis.hut./projects/somtoolbox/.SOM toolbox for Matlab 5
Vince, J., Hardesty, B. D., and Wilcox, C. (2021). Progress and challenges in eliminating illegal fishing. Fish. Fish. 22, 518–531. doi:10.1111/faf.12532
Vlahović, P., Veselinović, A., Remetić, S., Stepić, M., Gavrilović, L. J., Gavrilović, D., et al. (2006). Morava. Beograd: Zavod za udžbenike i nastavna sredstva.
WFD (2000). Water Framework directive - directive of European parliament and of the council 2000/60/EC – establishing a Framework for community action in the field of water policy. Luxembourg: European Union, the European Parliament and Council.
Yang, J., Yan, D., Yang, Q., Gong, S., Shi, Z., Qiu, Q., et al. (2021). Fish species composition, distribution and community structure in the fuhe river basin, jiangxi province, China. Glob. Ecol. Conservation 27, e01559. doi:10.1016/j.gecco.2021.e01559
Zhong, Y., and Power, G. (1996). Environmental impacts of hydroelectric projects on fish resources in China. Regul. Rivers Res. Mgmt. 12, 81–98. doi:10.1002/(sici)1099-1646(199601)12:1<81::aid-rrr378>3.0.co;2-9
Keywords: ecological sustainability, river basin, ichthyocenosis, ESHIPPOfish model, multiple freshwater stressors
Citation: Simić V, Bănăduc D, Curtean-Bănăduc A, Petrović A, Veličković T, Stojković-Piperac M and Simić S (2022) Assessment of the ecological sustainability of river basins based on the modified the ESHIPPOfish model on the example of the Velika Morava basin (Serbia, Central Balkans). Front. Environ. Sci. 10:952692. doi: 10.3389/fenvs.2022.952692
Received: 25 May 2022; Accepted: 27 June 2022;
Published: 22 July 2022.
Edited by:
Jun Hou, Hohai University, ChinaReviewed by:
Xiaogang Wang, Nanjing Hydraulic Research Institute, ChinaXiaotao Shi, China Three Gorges University, China
Copyright © 2022 Simić, Bănăduc, Curtean-Bănăduc, Petrović, Veličković, Stojković-Piperac and Simić. This is an open-access article distributed under the terms of the Creative Commons Attribution License (CC BY). The use, distribution or reproduction in other forums is permitted, provided the original author(s) and the copyright owner(s) are credited and that the original publication in this journal is cited, in accordance with accepted academic practice. No use, distribution or reproduction is permitted which does not comply with these terms.
*Correspondence: Vladica Simić, vladica.simic@pmf.kg.ac.rs; Ana Petrović, ana.petrovic@pmf.kg.ac.rs