- 1Dauphin Island Sea Lab, University Programs, Dauphin Island, AL, United States
- 2School of Marine and Environmental Sciences, University of South Alabama, Mobile, AL, United States
Human activity affects marine mammal stranding rates in two major ways; through human interaction (HI) that may lead to mortality and through search and response efforts that enable carcass detection. To better quantify the combined effects of these interacting human influences, we analyzed strandings for bottlenose dolphins (Tursiops truncatus) in the northern Gulf of Mexico (nGOM), an area of high cetacean strandings. To identify hotspots of human influence, we first determined the number of total and HI-related bottlenose dolphin strandings normalized to shoreline length in each nGOM U.S. state, which represent major response areas. To estimate the effects of response effort on stranding numbers (for HI and non-HI strandings), we used the Deepwater Horizon oil spill (DWHOS) as an established benchmark to compare periods of lower (pre-spill) and higher (post-spill) response effort. Strandings in Alabama waters were used as a case study to detail spatial and temporal variation due to human influences during the 39-year period of retrospective study. We found an increase in strandings from Louisiana through Alabama following the DWHOS. Non-oil related HI strandings increased in total number in AL, and they increased as a proportion of total strandings in Alabama (16%) and the Florida panhandle (12%). The increase in HI-related strandings in Alabama was driven by mortality of many types, but particularly fishery interactions and cases of apparent intentional harm. The Alabama case study clearly detected lower stranding numbers during periods of low or intermittent response coverage. Our findings are consistent with an overall increase in stranding numbers due to a combination of increased stranding occurrence and response effort following the DWHOS. Importantly, we provide evidence that HI-related standings increased independently from the DWHOS, with ongoing increases in at least one hotspot (Alabama). These findings provide a first step to parsing out different effects of human influences on stranding data for a common coastal cetacean. Our approach establishes baselines for future damage and recovery assessments, identifies areas where resources can be focused for management and education, and highlights the power of response and monitoring agencies to positively influence stranding datasets.
Introduction
Human activity can directly lead to stranding of live or dead aquatic animals. The most commonly reported anthropogenic drivers of marine mammal strandings (animals alive and in distress or dead washed ashore or floating) include various types of pollution, fisheries interactions, and boat strikes, which can have a range of effects that tend to be poorly defined even when animals are recovered dead (e.g., Desforges et al., 2018; Harvell et al., 1999; Laist et al., 2001; Nowacek et al., 2007; Read et al., 2006). Marine mammals may also depredate commercial and recreational fisheries species and become entangled in fishing line or caught incidentally in nets and drown (Zollett and Read, 2006; Read, 2008; Deming et al., 2020). In some locations, these human interactions include intentional or unintentional harassment and associated changes in natural behavior and feeding or cause purposeful injury to marine mammals (Spradlin et al., 2001; Samuels and Bejder, 2004; Vail, 2016; Collins et al., 2020). As a result, increased interaction with humans can increase the rate of marine mammal strandings, especially among coastal and estuarine species that are in close proximity to human activities such as fishing and tourism (Cunningham-Smith et al., 2006; Powell et al., 2018; Collins et al., 2020).
Stranding rates of marine mammals are also indirectly influenced by humans through search and response efforts. Across the globe, marine mammal stranding networks respond to live and dead stranded marine mammals, with varying levels of consistency and coverage (Chan et al., 2017; Simeone and Moore, 2018; Gómez-Hernández et al., 2022). The presence of a stranding network necessarily increases the likelihood that data will be collected on stranded animals to determine potential causes of death, including human interactions (HI). In the U.S., local stranding networks collect at least basic demographic data and submit them to a national database (NOAA National Marine Fisheries Service’s Marine Mammal Health and Stranding Response Program (MMHSRP)), which is one of a few such databases world-wide (Chan et al., 2017; Gómez-Hernández et al., 2022). Stranding data, however, have been historically limited by insufficient funding and infrastructure for comprehensive and enduring response, which can result in poor monitoring, incomplete documentation, and inconsistent data collection (Hofman, 1991; Wilkinson, 1991; Gómez-Hernández et al., 2022). These factors can result in underreporting despite the existence of a stranding response network (e.g., Kemper et al., 2005; Wells et al., 2015; Lloyd and Ross, 2015). Hence, variation in stranding response effort and potential effects on spatial or temporal demographics of stranding patterns must be considered to accurately quantify stranding numbers and clearly define hotspots of stranding activity.
How to best quantify monitoring efforts for demographics studies is an ongoing challenge in ecology (e.g., Symons et al., 2018; McBride-Kebert et al., 2019). Effects of effort on demographics data for live animals have been well studied in fisheries and some targeted marine mammal research (McCluskey and Lewison, 2008; McBride-Kebert et al., 2019; Tiongson et al., 2021), but these approaches typically cannot be applied to marine mammal stranding data. Stranding data typically rely on opportunistic public reporting rather than regular targeted monitoring of a given location. Furthermore, because marine mammals are not a commercial or recreational fishery in the U.S., many traditional metrics of effort such as fishing licenses, boats on the water, and catch reports are not options for effort assessment. Comparable data on public access or use are not broadly available for many locations where marine mammals strand to historically relate strandings to frequency of human presence. Despite these limitations, use of opportunistic, anecdotal, or traditional ecological knowledge has been successfully used and is growing in popularity to at least yield best estimates of long-term or large-scale patterns for some fisheries and marine mammal studies (e.g., Symons et al., 2018; Giovos et al., 2019; IJsseldijk et al., 2020). Moving forward, studies that apply stranding data will benefit from efforts to identify and test alternative metrics and approaches to account for monitoring or response efforts (e.g., Wells et al., 2015). Such approaches will be essential to the future of ecological monitoring, particularly for long-lived, difficult to study species and under changing climate regimes where understanding historical trends are critical to detecting and managing future change (Harvey et al., 2018; Symons et al., 2018).
The potential effect of response effort on marine mammal stranding numbers was recently evidenced following the Deepwater Horizon oil spill (DWHOS) in the northern Gulf of Mexico (nGOM). The DWHOS is recognized as a major source of HI-related mortality, responsible for the largest and longest-running cetacean unusual mortality event (UME) in the Gulf of Mexico in U.S. history, which included loss of more than 1,000 bottlenose dolphins (Litz et al., 2014; Table 1). During 2010, as part of NOAA’s Natural Resource Damage Assessment there was an increase in the number of observers monitoring and cleaning local beaches and increased funding for stranding network operations in the region (Wilkin et al., 2017). These increased resources led to greater stranding response through increased search effort and development of more formalized stranding response protocols for the nGOM, both of which may have influenced stranding numbers (Williams et al., 2011; Pitchford et al., 2018). The historical carcass recovery rate for cetacean species in the nGOM is estimated at 2%, largely due to poor detection among populations of unknown animals (Williams et al., 2011; Wells et al., 2015), but the region also includes known periods of marine mammal stranding response gaps, including little to no coverage in Louisiana, Mississippi, Alabama and parts of western Florida prior to the DWHOS (Worthy, 1998; Venn-Watson et al., 2015). These observations provide well-known and documented temporal and spatial inflection points with potential to help parse out the net effects of human influence on strandings in terms of mortality and response effort.
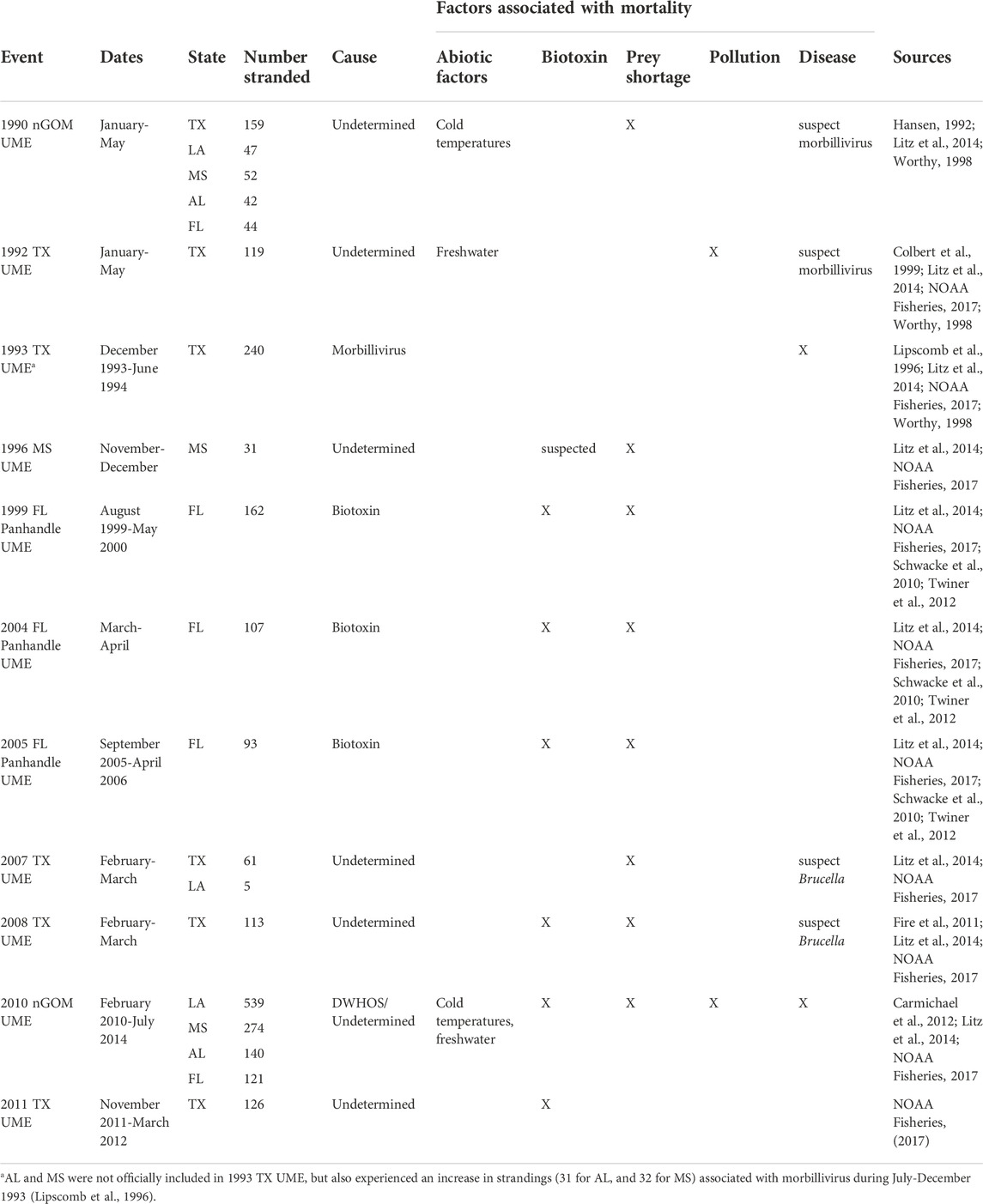
TABLE 1. Marine mammal stranding occurrences designated as unusual mortality events (UMEs) in nGOM states that occurred 1990–2016 [Texas = TX, Louisiana = LA, Mississippi = MS, Alabama = AL, Florida panhandle (Escambia-Jefferson counties)] = FL.
In this study, we sought to better quantify human influence on strandings rates of the bottlenose dolphin (Tursiops truncatus) in the nGOM in terms of human interactions with potential for contributions to mortality (HI) and response effort. T. truncatus was selected because it is prevalent in coastal waters globally, interactions with people are well documented, and it is the most commonly-stranded marine mammal in the nGOM, comprising approximately 85% of cetacean strandings in the region (Litz, 2015; Russell et al., 2022). We hypothesized that the proportion of HI-positive strandings would increase through time relative to increased stranding response effort, particularly following the DWHOS. We also hypothesized that hotspots of human influence would be detectable as areas with a high density of HI-positive cases due to increased HI events, regardless of effort. To test these hypotheses, we determined the number of total and HI-positive bottlenose dolphin strandings normalized to shoreline length in each nGOM state. To estimate the effects of response effort on stranding number (for total strandings and HI-positive strandings), we used the DWHOS as an established benchmark to compare periods of lower (pre-spill) and higher (post-spill) response effort. Strandings in Alabama waters were used as a case study to detail spatial and temporal variation due to human influences during the 39-year period of retrospective study.
Materials and methods
Publicly available demographic (Level A; NOAA, 2020) data for T. truncatus stranded in the nGOM from the Texas-Mexico border through Jefferson County, Florida were accessed from NOAA’s MMHSRP database as of 8 November 2017 for the years 1978 through 2016. For the state of Alabama case study, we additionally documented the names of designated responding organizations from Level A data for each stranding.
Human interaction-positive strandings
To determine the number of HI-positive strandings, data were categorized as HI-positive (definitive evidence of HI present) or HI-negative (no definitive evidence of HI, including cases where HI status could not be determined or the carcass was not entirely examined) based on documentation in the MMHSRP. The initial determination of HI status for stranded dolphins within each state is made in the field, typically by a primary responder or response team and corroborated by NOAA personnel when data are verified in the MMHSRP (e.g., Moore and Barco, 2013). Strandings prior to 2001, when HI status was first formally recorded in the MMHSRP database, were updated as HI-positive or HI-negative based on whether HI was mentioned in the “additional comments” section of the Level A data.
To determine the density of HI-positive strandings along the nGOM coast, the annual number of total and HI-positive strandings were standardized using the linear distance of shoreline, separated by state (Figure 1). The linear shoreline distance of each state (Texas, Louisiana, Mississippi, Alabama, Florida panhandle) was determined from the National Environmental Sensitivity Index Shoreline dataset (NOAA Office of Response and Restoration, 2017). Vector line data used from this dataset included all natural and manmade shoreline boundaries of the contiguous nGOM states where strandings could wash ashore, ending at the eastern boundary of Jefferson County, Florida where there is an historical break in stranding response that separates the panhandle from peninsular Florida (Litz et al., 2014). We analyzed HI data including and excluding data from animals stranded during the DWHOS UME that were classified as visibly oiled in the MMHSRP to remove bias associated with this acute event in the HI data.
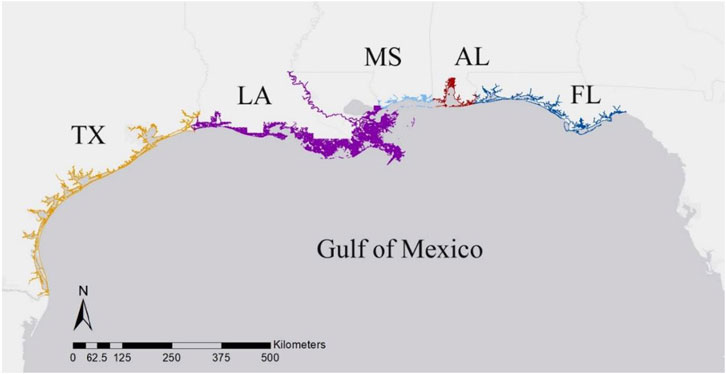
FIGURE 1. Linear coastline of each state in the northern Gulf of Mexico, where Texas = TX, Louisiana = LA, Mississippi = MS, Alabama = AL, and the Florida panhandle = FL.
For the state of Alabama case study, we further related temporal and spatial variation in HI strandings to HI type by classifying HI-positive strandings into five groups: fishery interaction, mutilation, boat collision, incidental take, and other. For these analyses, one visibly oiled animal that stranded during the DWHOS UME was included and categorized as “other.” Strandings were then plotted through time and mapped using ArcGIS 10.3 (ESRI, 2014) to detect local patterns relative to HI type.
Stranding response effort
Because stranding response efforts in the U.S. are state-specific, we accounted for response effort by state and normalized to shoreline distance as described above and further divided the data into pre-DWHOS (1978–2009) and post-DWHOS (2010–2016) coverage periods, representing known periods of lower and higher effort, respectively (Wilkin et al., 2017). We additionally analyzed data with and without respect to known UMEs to eliminate the effect of these acute events, particularly associated with the DWHOS.
We took advantage of additional data on stranding response effort in the state of Alabama to conduct a more detailed case study to extend our approach to account for interannual variation in response effort in this state. We compared annual stranding counts for all cetaceans (90% T. truncatus) and the occurrence and types of HI (100% T. truncatus) to the level of stranding response effort, indicated by the response organization in the MMHSRP and known relevant metrics of effort (i.e., whether there was a formal stranding agreement holder designated by NOAA NMFS, if response was opportunistic or conducted by trained volunteers or paid staff members, if responders included full-time designated personnel, and if dedicated state or federal funding was awarded; Supplementary Table S1).
Based on these metrics, we defined three distinct levels of stranding response effort in Alabama, including: 1) low effort (periods of minimal response when response was opportunistic and there was no formal stranding response designee or dedicated stranding response funding for the state; 1978–1986, 2007–2008), 2) moderate effort (fully volunteer response designees with limited dedicated funding; 1987–2006), and 3) high effort (full-time paid response designees with regular dedicated funding; 2009–2016). In some years, designees responded for part of a year or multiple groups responded to strandings, with different levels of effort. In these cases, the maximum estimated level of effort was assigned for the full year (Supplementary Table S1). Through time, the location of designated response organizations also varied from the eastern to the western sides of Mobile Bay, the major body of water in Alabama. Hence, we were additionally able to test possible effects of effort on the local geographic distribution of stranding numbers, assuming greater response effort may occur in areas nearer responding organizations.
Data analysis
To compare stranding numbers among states and between pre- and post-DWHOS periods for the entire nGOM, we performed Multivariate Analysis of Variance (MANOVA) with marginal p-values on annual stranding count data, normalized by linear shoreline distance (total, HI-positive, and non-oiled HI-positive data). A Levene’s test (Levene, 1960) was performed to test for homoskedasticity, and generalized least squares (GLS) models in R nlme v3.1-124 were applied to compensate for heteroskedasticity. Model selection was performed using a corrected Akaike information criterion estimator (AICc; Burnham and Anderson, 2004). We selected a GLS model with variance weighted by state for the total annual stranding data and weighted by state and coverage period (pre-v. post-DWHOS) for HI-positive and non-oiled HI-positive strandings, normalized by linear shoreline distance and reported as mean annual strandings per 100 km for pre- and post-DWHOS periods.
After performing the MANOVA on the selected GLS models, post-hoc two-sided Welch’s t-tests (Welch, 1947) and Westfall method of multiple comparisons (Westfall, 1997) were performed to determine differences between pre- and post-DWHOS periods (low v. high effort) within states and between states, respectively. All GLS models were selected in R using MuMIn v1.156, with post-hoc analyses performed in R car version 2.3 and R multcomp v1.4-6. To additionally determine if there were differences in the proportion of HI-positive and non-oiled HI-positive strandings (% of total) for all the states within the two (pre-v. post-DWHOS) periods, the test for equality of proportions with continuity correction was used for each respective category. We then used a chi-square test statistic and Holm-corrected pair-wise comparison of proportions post-hoc test with five groups. All analyses were performed in R v3.4.1, SingleCandle.
For the Alabama case study, regression analysis was used to detect changes in total cetacean strandings or HI-positive T. truncatus strandings through time relative to estimated level of response effort (low, moderate, high) using StatPlus: mac v6. Data were analyzed including and excluding UMEs to help distinguish changes in effort from acute events (e.g., Table 1). To detect differences in stranding distributions relative to the primary location of response agencies, stranding location data were divided geographically into 0.2-degree longitude bins, separated by location and level of effort, and a matrix was used to compare locations in R v3.4.1, SingleCandle.
Results
Total strandings in the northern Gulf of Mexico
There were a total of 8,667 T. truncatus strandings along the 112,669 linear km nGOM shoreline from 1978–2016 (Figure 1; Table 2). Texas had the highest number of total strandings along the second longest shoreline, and Alabama had the highest proportion (8%) of total HI-positive strandings along the shortest shoreline during the 39-year study period (Table 2). Accordingly, the mean number of strandings per 100 km differed by state (MANOVA; F4,185 = 52.69, p < 0.0001) and pre- or post-spill coverage period (MANOVA; F1,185 = 12.28, p < 0.01; Supplementary Tables S2, S3), with a significant interaction between state and coverage period (MANOVA; F4,185 = 5.00, p < 0.001).
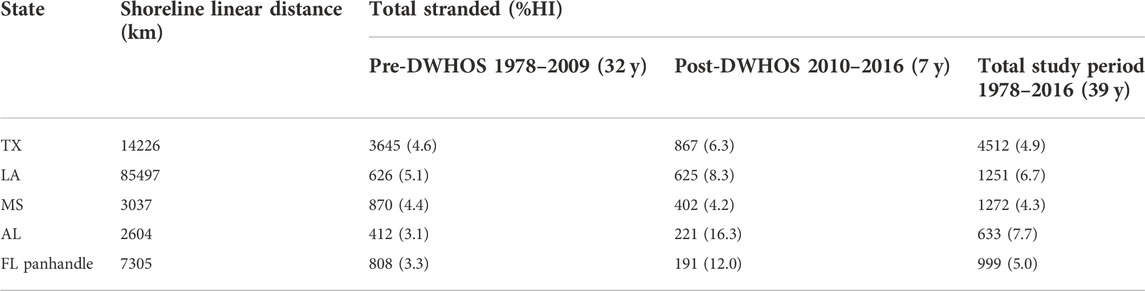
TABLE 2. The total number (and percent HI-positive) of T. truncatus strandings compared to linear shoreline distance of nGOM states (shown in Figure 1) during the 39-year study period, separated by time periods pre- (1978–2009) and post- (2010–2016) DWHOS. FL panhandle includes shoreline from the Alabama-Florida border through Jefferson County, FL.
Prior to the DWHOS, when response efforts were lower, Texas and Mississippi had greater mean annual strandings per 100 km of shoreline than other states (Westfall; p < 0.02 for all comparisons; Figure 2A). Significant increases in strandings in Mississippi and Alabama post-spill (Westfall; p < 0.01 for all comparisons) resulted in a higher number of strandings in these states compared to others during this 2010–2016 period (Westfall; p < 0.02). Although strandings increased in Louisiana post-spill, Louisiana had fewer annual strandings relative to shoreline length than other states during both periods (Westfall; p < 0.001 for all comparisons; Figure 2A).
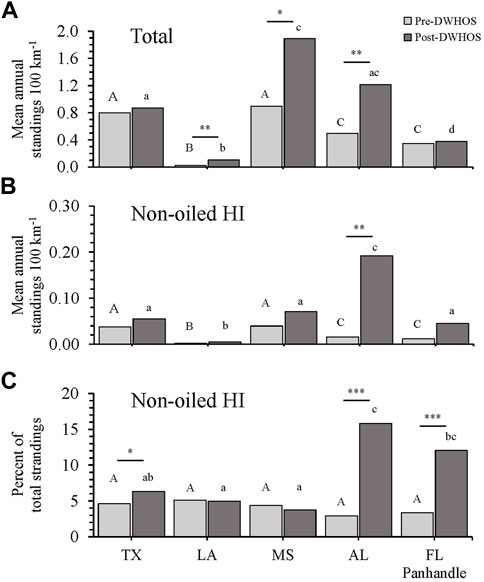
FIGURE 2. Total (A) and non-oiled HI-positive (B) mean annual strandings normalized to linear shoreline distance (100 km−1) and non-oiled HI-positive cases as a percentage of total strandings (C), separated by state and pre- (1978–2009) and post- (2010–2016) DWHOS coverage periods. Capital and lower-case letters indicate significant differences among locations for pre-DWHOS and post-DWHOS coverage periods, respectively. Asterisks indicate differences between coverage periods within each state, where *p < 0.05, **p < 0.01, ***p < 0.001.
Non-oiled human interaction-positive strandings in the northern Gulf of Mexico
Mean annual HI-positive strandings, excluding oiled animals, also differed by state (MANOVA; F4,175 = 23.75, p < 0.001) and pre- or post-spill period (MANOVA; F1,175 = 55.08, p < 0.001) and had a significant interaction between state and coverage period (MANOVA; F1,175 = 14.31, p < 0.001; Supplementary Tables S2, S3). As with total strandings, Texas and Mississippi had higher HI-positive strandings compared to other states prior to the DWHOS, when effort was lower (Westfall; p < 0.02 for all comparisons). Following the spill, there was a 13-fold increase in mean annual HI-positive strandings per 100 km in Alabama (t6.16 = −4.68, p = 0.0031). No other states had an increase in mean annual non-oiled HI-positive strandings between pre- and post-spill periods (Figure 2B).
When considering non-oiled HI-positive strandings as a proportion (%) of total strandings, there was no difference in HI prevalence among states during the pre-spill period (χ2 4 = 5.48, p = 0.24), but there was a difference among states during the post-spill period (χ2 4 = 45.73, p < 0.0001) (Figure 2C). Alabama had a higher proportion of HI-positive strandings than Louisiana (z = 4.45, p < 0.0001), Mississippi (z = 4.57, p < 0.0001), and Texas. Similarly, the Florida panhandle had a higher proportion of HI-positive strandings than Louisiana (z = 2.45, p = 0.0062) and Mississippi (z = 2.94, p = 0.0016). Texas (χ2 1 = 4.12, p = 0.042), Alabama (χ2 1 = 55.18, p < 0.001) and Florida panhandle (χ2 1 = 22.78, p < 0.001) showed significant relative increases in HI between pre- and post-spill periods, with Alabama and Florida having the highest percentage of HI-positive strandings (16% and 12%, respectively; Table 2 and Figure 2C).
Alabama case study
A total of 709 reported cetacean strandings occurred in Alabama from 1978 through 2016. Of the reported cetaceans, 633 strandings (89%) were identified as T. truncatus and 49 of those were documented as HI-positive. The total number of reported strandings increased through time at a rate of approximately 1 cetacean per year during the entire 39-year period of documented strandings (Figure 3A). During this same period, HI-positive strandings also increased through time, but at a lower rate due to low documented numbers of HI (≤ two per year) until 2010, when reports more than doubled in number during 2010 and 2011 (Figure 3B). Since 2012, HI-related strandings have increased exponentially, doubling in number during each of the last 2 years of this study (Figure 3B).
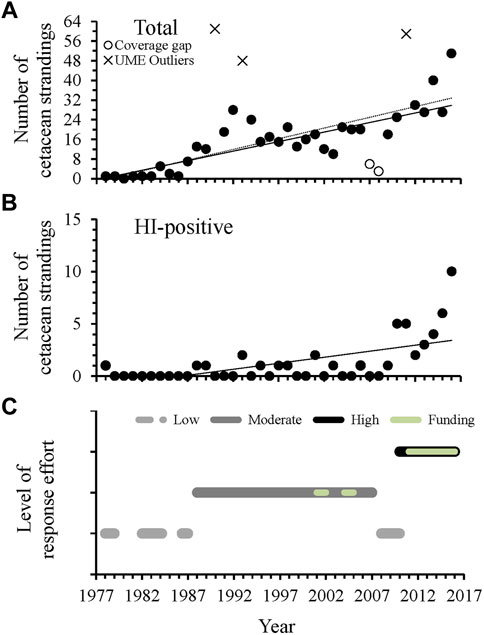
FIGURE 3. The total number of Alabama cetacean strandings (A) and HI-positive strandings, including a visibly oiled animal (n = 1 in 2010) (B) compared to estimated level of stranding effort and dedicated response funding (C) in Alabama waters through time. For total strandings, the solid black line (y = 0.78x - 1535.20 R2 = 0.57, F1,34 = 44.89, p < 0.001) excludes the documented UMEs that occurred in 1990, 1993 and 2011 (Table 1), which were outliers to the regression. The dotted black line (y = 0.87x - 1723.70, R2 = 0.71, F1,26 = 35.44, p < 0.001) additionally excludes data for 2007 and 2008 that coincided with a recent gap in stranding response and were outliers to the regression when UMEs were excluded. For HI-related strandings, y = 0.12x - 234.02, R2 = 0.38; Freg1,37 = 22.48, p < 0.001, with an exponential increase since 2012 (y = 1.96e0.39(x−2012), R2 = 0.99; Freg1,3 = 338.43, p < 0.001). Detailed metrics for level of effort assignments are provided in Supplementary Table S1.
Outliers to the regression comparing total stranding numbers through time were found during 1990, 1993, and 2011 (Figure 3A), consistent with periods of documented UMEs in the GOM even when UMEs were not specifically declared for AL waters (Table 1). Additional UMEs that occurred in the GOM during 1996 and 2010–2013 did not result in statistical outliers in AL. When data were reanalyzed excluding UME outliers, 2 years (2007, 2008) had lower than expected numbers of strandings and occurred during a period of known low stranding response effort (Figures 3A, C). Strandings during this period were comparable to those during the low coverage periods from 1978–1992 in Alabama, when response was limited and opportunistic (Supplementary Table S1). Stranding numbers were higher under moderate and high levels of effort from 1987–2007 and since 2010, respectively (Figure 3C). The geographic distribution of strandings was highly correlated between periods of moderate and high response effort (moderate compared to high effort: r = 0.94, p = 0.02), regardless of location of the response organization (Figure 4 and Supplementary Figure S1). When response effort was low, however, stranding locations were not correlated to stranding locations during moderate or high effort periods (r < 0.28, p > 0.64).
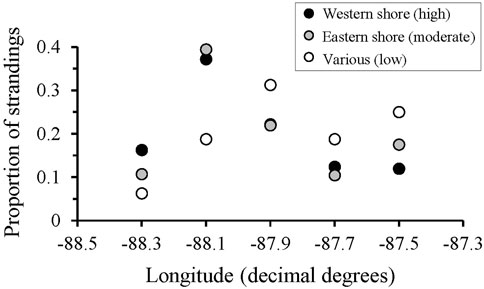
FIGURE 4. The proportion of Tursiops truncatus strandings that occurred along the Alabama coastline, grouped into 0.2-degrees longitude binds, separated by location of the major response agency and associated level of response effort (locations and effort metrics are detailed in Supplementary Table S1).
HI-positive strandings in Alabama were most frequently reported along Dauphin Island, Fort Morgan and the nGOM coastlines (Figure 5A) and included strandings in all categories throughout Alabama waters. Most HI-positive strandings were categorized as fishery interactions, which were documented since 1993 and increased in recent years (Figure 5B). Other types of strandings (categorized as ‘other’) included visibly oiled (n = 1), shot with a field arrow (n = 1), trapped behind a barrier (n = 1), rope entanglement of the fluke that could not be confirmed as a fishery interaction or not (n = 1), and blunt trauma from an unknown source (n = 1; Figure 6). Since 2010, five individuals were also documented to simultaneously exhibit two types of HI (Figure 5A, multicolored circles and Figure 5B, hashed bars).
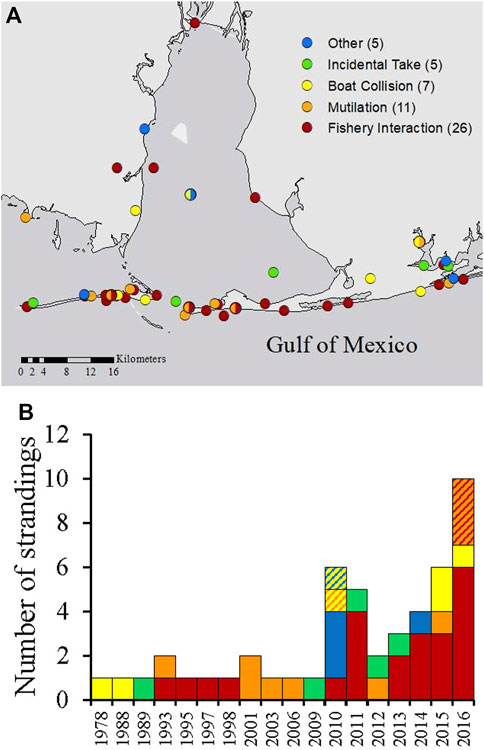
FIGURE 5. Location (A) and total annual numbers (B) of HI-positive strandings in Alabama, separated by HI type. Strandings that included more than one type of HI (n = 5) are indicated by multicolored circles (A) and muticolored hashed bars (B).
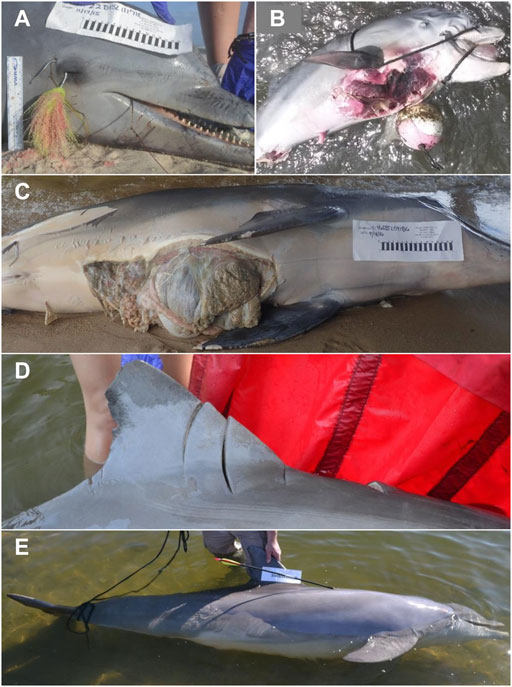
FIGURE 6. Examples of HI-positive dolphin stranding cases in Alabama, including fishery interaction [hook and line, (A); crab pot entanglement, (B); mutilation (ventrum sliced; (C); boat collision (acute trauma; (D); purposeful harm (shot with arrow; (E)].
Discussion
Human interactions with marine mammals pose a threat of increasing global concern for conservation (Obusan et al., 2016; Avila et al., 2018; Liu et al., 2019). Our study supports the idea that human activities also can provide for better response to and documentation of strandings, if effort is directed toward monitoring and reporting of stranded animals (see also Wilkin et al., 2017; Moore et al., 2018; Pitchford et al., 2018). We found that Alabama is a hotspot of HI-positive strandings along the nGOM coast, having the highest density and percentage of HI-positive strandings since 2010, not related to the DWHOS. This increase has included more HI-positive reports associated with many types of mortality, but particularly fishery interactions and cases of apparent intentional harm. Higher numbers of non-oiled HI-positive strandings in 2010-2011 provide quantitative evidence that greater effort associated with DWHOS Natural Resource Damage Assessment response, which provided for more vessels on the water and personnel monitoring beaches (Wilkin et al., 2017), likely contributed to increased reporting of strandings. Better awareness from local citizens also likely contributed to increased reporting during this period (Wilkin et al., 2017). The subsequent drop in HI-positive strandings in Alabama in 2012 is consistent with a return to pre-DWHOS (baseline) levels, further suggesting that the exponential increase in HI-positive strandings since 2012 is primarily due to increased stranding occurrence rather than effort alone; a critical finding that was only possible because of our assignment of effort levels to Alabama data. Of note, the higher proportion of HI-positive reports in Alabama cannot be attributed to greater capability for HI detection compared to other states because the ALMMSN at DISL was the newest stranding network on the nGOM coast and unlike most neighboring states did not have a full-time veterinarian on staff during the study period. Identifying areas of high HI-positive strandings provides an opportunity to direct resources toward efforts to change behavior and reduce negative interactions in areas of greatest need, while increasing the positive aspects of human participation in monitoring and stranding response. For Alabama, this analysis also afforded the unique opportunity to quantify the additional effects of effort on stranding numbers and detect a meaningful increase in HI-positive stranding occurrence in recent years.
Gulf-wide, our findings indicate a regionally specific increase in strandings along the nGOM coast from Louisiana through Alabama, with the greatest number of HI-positive strandings to the east. While the overall increase in stranding numbers is likely due to a combination of increased stranding occurrence and response effort following the DWHOS, we provide evidence that HI-positive standings increased independently from the DWHOS and in locations of the nGOM most distant from the spill site. Ongoing or residual effects of the DWHOS are possible throughout the nGOM, and HI-positive cases in particular may remain higher than historical numbers due to increased awareness and better-trained and prepared investigators. The additional resources provided to stranding networks throughout the Gulf of Mexico during and following the DWHOS, have helped build long-term capacity for enhanced response through funding for trained personnel and equipment as well as development and coast-wide use of standardized comprehensive protocols that include HI assessment (Wilkin et al., 2017). Furthermore, the Alabama case study suggests stranding numbers documented during the early years of stranding response (prior to 1990) may be artificially low due to poor, intermittent coverage, as evidenced by the similarity to stranding numbers during known periods of low coverage in recent years. As a result, the historical baseline for total annual stranding numbers may not be accurately definable for the nGOM, and small differences between pre- and post-spill periods may not be highly meaningful. Also, because HI status can most readily be determined in fresh carcasses by properly-trained personnel, these numbers likely represent a minimum count of HI-positive strandings. Therefore, the dramatic increases observed in total strandings in Alabama and Mississippi and HI-positive strandings in Alabama and the Florida panhandle warrant additional attention from managers and stranding networks as they continue to assess dolphin population recovery and plan for future conservation throughout the nGOM. These data will be particularly important to determine relationships between HI and causes of mortality, given that many animals may experience HI throughout their life, with evidence detectable at stranding, but HI may not be the proximate cause of death.
This study provides the first regional-scale analysis of anthropogenic drivers of bottlenose dolphin stranding reports in the nGOM and localized data in the state of Alabama. Our findings of this study highlight the need for high quality regional and local data collection and analysis of patterns in marine mammal stranding events. Had we not normalized data by area of shoreline for each state or considered the effects of effort through time, it is likely the substantial increase in HI-related strandings in an area of relatively small shoreline like Alabama or the Florida panhandle (compared to all of Florida) would be overlooked. Of note, the types of HI-positive strandings in Alabama represent the wide range of HI types, which are often described but rarely quantified in publications, for bottlenose dolphin strandings in U.S. waters (Samuels and Bejder, 2004; Byrd et al., 2014; Vail, 2016; Powell et al., 2018; Maze-Foley et al., 2019; Collins et al., 2020), further emphasizing the broad nature of HI in this local area. Our analytical approach provides a method for other researchers to similarly evaluate anthropogenic impacts, including historical assessment of response effort, on marine mammal strandings and identify areas of high occurrence for other species and geographical locations. The HI-positive stranding increases documented here were serendipitously detectable in part due to the increased effort enabled by DWHOS response. These results evidence the benefits of sufficient funding to stranding networks as well as potential importance of some human influences on stranding numbers. Stranding networks and managers will benefit from taking advantage of the increased information derived from post-DWHOS stranding response efforts to better define the net effects of human influences on dolphin strandings and work collaboratively toward solutions to human-caused mortality in areas of high occurrence (Balmer, 2007).
Data availability statement
The datasets presented in this study can be found in online repositories. The names of the repository/repositories and accession number(s) can be found below: The data analyzed in this study were obtained from the NOAA’s MMHSRP and Office of Response and Restoration. Requests to access the MMHSRP dataset should be directed to MMHSRP NationalDB@noaa.gov. The Office of Response and Restoration’s National Environmental Sensitivity Index Shoreline data are available at https://response.restoration.noaa.gov/esi_download.
Ethics statement
Ethical review and approval was not required for the animal study because work was conducted on dead animals under a NOAA NMFS stranding agreement to the DISL.
Author contributions
RC, MH, NW, and MR contributed conception and design of the study; MH and NW organized the dataset; RC, MH, and NW performed the statistical analysis; RC, MH, NW, and MR designed figures and tables; RC and MH wrote the first draft of the manuscript; RC, MH, and NW wrote sections of the manuscript. All authors contributed to manuscript revision, read, and approved the submitted version.
Funding
The preparation of this manuscript by RC, MH, NW, and MR was funded through a grant from the National Fish and Wildlife Foundation (NFWF #45720). Partial funding was also provided by Deepwater Horizon natural resource damage settlement funds provided by the Alabama Trustee Implementation Group with funding support and participation of the Alabama Department of Conservation and Natural Resources. MH was also funded by the Dauphin Island Sea Lab and the University of South Alabama. The statements, findings, conclusions, and opinions are those of the authors and nothing contained herein constitutes an endorsement in any respect by NFWF.
Acknowledgments
We thank Natalie Santini and Amber Simpson for database management and quality assurance or assistance compiling data for some figures and tables. We thank NOAA National Marine Fisheries Service and members of the Southeast United States Marine Mammal Stranding Network, particularly the Alabama Marine Mammal Stranding Network, the Alabama Department of Conservation and Natural Resources, the Institute for Marine Mammal Studies, Spring Hill College/Marterra Foundation, and volunteers who diligently and tirelessly responded to marine mammal strandings on the northern Gulf of Mexico coast during the 39-years considered in this study.
Conflict of interest
The authors declare that the research was conducted in the absence of any commercial or financial relationships that could be construed as a potential conflict of interest.
Publisher’s note
All claims expressed in this article are solely those of the authors and do not necessarily represent those of their affiliated organizations, or those of the publisher, the editors and the reviewers. Any product that may be evaluated in this article, or claim that may be made by its manufacturer, is not guaranteed or endorsed by the publisher.
Supplementary material
The Supplementary Material for this article can be found online at: https://www.frontiersin.org/articles/10.3389/fenvs.2022.951329/full#supplementary-material
References
Avila, I. C., Kashner, K., and Dormann, C. F. (2018). Current global risks to marine mammals: Taking stock of the threats. Biol. Conserv. 221, 44–58. doi:10.1016/j.biocon.2018/02.021
Balmer, B. C. (2007). Seasonal abundance, site-fidelity, and utilization areas of bottlenose dolphins in St. Joseph Bay, Florida. Thesis Submitt. to Univ. North Carolina Wilmingt. Available at: http://dl.uncw.edu/etd/2007-1/balmerb/brianbalmer.pdf.
Burnham, K. P., and Anderson, D. R. (2004). Model selection and multimodel inference: A practical information-theoretic approach. Second. New York, NY: Springer New York. doi:10.1007/b97636
Byrd, B. L., Hohn, A. A., Lovewell, G. N., Altman, K. M., Barco, S. G., Friedlaender, A., et al. (2014). Strandings as indicators of marine mammal biodiversity and human interactions off the coast of North Carolina. Fish. Bull. Wash. D. C). 112, 1–23. doi:10.7755/fb.112.1.1
Carmichael, R. H., Graham, W. M., Aven, A., Worthy, G., and Howden, S. (2012). Were multiple stressors a “perfect storm” for northern gulf of MeMexicoottlenose dolphins (tursiops truncatus) in 2011? PLoS One 7, e41155–e41159. doi:10.1371/journal.pone.0041155
Chan, D. K. P., Tsui, H. C. L., and Kot, B. C. W. (2017). Database documentation of marine mammal stranding and mortality: Current status review and future prospects. Dis. Aquat. Organ. 126, 247–256. doi:10.3354/dao03179
Colbert, A., Scott, G. I., Fulton, M. H., Wirth, E. F., Daugomah, J. W., Key, P. B., et al. (1999). Investigation of unusual mortalities of bottlenose dolphins along the mid-Texas coastal bay ecosystem during 1992. Seattle, Washington: NOAA Techincal Report NMFS 147, 1–23.
Collins, M. K., Carmichael, R. H., Rotstein, D. S., Byrd, J. H., and Deming, A. C. (2020). Suspected broadhead arrow injuries in two common bottlenose dolphins (Tursiops truncatus) along the Alabama Coast. Mar. Mamm. Sci. 36, 721–727. doi:10.1111/mms.12667
Cunningham-Smith, P., Colbert, D. E., Wells, R. S., and Speakman, T. (2006). Evaluation of human interactions with a provisioned wild bottlenose dolphin (Tursiops truncatus) near Sarasota Bay, Florida, and efforts to curtail the interactions. Aquat. Mamm. 32, 346–356. doi:10.1578/AM.32.3.2006.346
Deming, A. C., Wingers, N. L., Moore, D. P., Rotstein, D., Wells, R. S., Ewing, R., et al. (2020). Health impacts and recovery from prolonged freshwater exposure in a common bottlenose dolphin (Tursiops truncatus). Front. Vet. Sci. 7, 235. doi:10.3389/fvets.2020.00235
Desforges, J.-P., Hall, A., McConnell, B., Rosing-Asvid, A., Barber, J. L., Brownlow, A., et al. (2018). Predicting global killer whale population collapse from PCB pollution. Science 361, 1373–1376. doi:10.1126/science.aat1953
Fire, S. E., Wang, Z., Byrd, M., Whitehead, H. R., Paternoster, J., and Morton, S. L. (2011). Co-occurrence of multiple classes of harmful algal toxins in bottlenose dolphins (Tursiops truncatus) stranding during an unusual mortality event in Texas, USA. Harmful Algae 10, 330–336. doi:10.1016/j.hal.2010.12.001
Giovos, I., Stoilas, V. O., Al-Mabruk, S. A., Doumpas, N., Marakis, P., Maximiadi, M., et al. (2019). Integrating local ecological knowledge, citizen science and long-term historical data for endangered species conservation: Additional records of angel sharks (Chondrichthyes: Squatinidae) in the Mediterranean Sea. Aquat. Conserv. 29, 881–890. doi:10.1002/aqc.3089
Gómez-Hernández, G., Norzagaray-López, O., Lubinsky-Jinich, D., Heckel, G., Schramm, Y., and Seingier, G. (2022). From beach users to first responders: The role of civil society in response actions to marine mammal stranding events. Ocean. Coast. Manag. 219, 106073. doi:10.1016/j.ocecoaman.2022.106073
Hansen, L. J. (1992). Report on investiagtion of 1990 Gulf of Mexico bottlenose dolphin strandings. Miami: National Marine Fisheries Service, Southeast Fisheries Science Center. doi:10.5962/bhl.title.4016
Harvell, C. D., Kim, K., Burkholder, J. M., Colwell, R. R., Epstein, P. R., Grimes, D. J., et al. (1999). Emerging marine diseases — climate links and anthropogenic factors. Science 285, 1505–1510. doi:10.1126/science.285.5433.1505
Harvey, G. K., Nelson, T. A., Paquet, P. C., Ferster, C. J., and Fox, C. H. (2018). Comparing citizen science reports and systematic surveys of marine mammal distributions and densities. Biol. Conserv. 226, 92–100. doi:10.1016/j.biocon.2018.07.024
Hofman, R. J. (1991). History, goals, and achievements of the regional marine mammal stranding networks in the United States. Miami, Florida: Second Mar. Mammal Stranding Workshop, 7–16.
Ijsseldijk, L. L., ten Doeschate, M. T., Brownlow, A., Davison, N. J., Deaville, R., Galatius, A., et al. (2020). Spatiotemporal mortality and demographic trends in a small cetacean: Strandings to inform conservation management. Biol. Conserv. 249, 108733. doi:10.1016/j.biocon.2020.108733
Kemper, C. M., Flaherty, A., Gibbs, S. E., Hill, M., Long, M., and Byard, R. W. (2005). Cetacean captures, strandings and mortalities in South Australia 1881-2000, with special reference to human interactions. Aust. Mammal. 27, 37–47. doi:10.1071/am05037
Laist, D. W., Knowlton, A. R., Mead, J. G., Collet, A. S., and Podesta, M. (2001). Collisions between ships and whales. Mar. Mamm. Sci. 17, 35–75. doi:10.1111/j.1748-7692.2001.tb00980.x
Levene, H. (1960). “Robust tests for equality of variances,” in Contributions to probability and statistics: Essays in honor of harold hotelling. Editors I. Olkin, S. G. Ghurye, W. Hoeffding, W. G. Madow, and H. B. Mann (Stanford University Press), 278–292.
Lipscomb, T. P., Kennedy, S., Moffett, D., Krafft, A., Klaunberg, B. A., Lichy, J. H., et al. (1996). Morbilliviral epizootic in bottlenose dolphins of the gulf of Mexico. J. Vet. Diagn. Invest. 8, 283–290. doi:10.1177/104063879600800302
Litz, J. A., Baran, M. A., Bowen-Stevens, S. R., Carmichael, R. H., Colegrove, K. M., Garrison, L. P., et al. (2014). Review of historical unusual mortality events (UMEs) in the Gulf of Mexico (1990-2009): Providing context for the multi-year northern Gulf of Mexico cetacean UME declared in 2010. Dis. Aquat. Organ. 112, 161–175. doi:10.3354/dao02807
Litz, J. (2015). “Overview of cetacean stranding data from the gulf of Mexico: 2000-2014,” in Gulf of Mexico marine mammal research and monitoring meeting (New Orleans, LA).
Liu, M., Lin, M., Zhang, P., Xue, T., and Li, S. (2019). An overview of cetacean stranding around Hainan Island in the South China Sea, 1978–2016: Implications for research, conservation and management. Mar. Policy 101, 147–153. doi:10.1016/j.marpol.2018.04.029
Lloyd, H. B., and Ross, G. A. (2015). Long-term trends in cetacean incidents in new South wales, Australia. Aust. Zool. 37, 492–500. doi:10.7882/AZ.2015.015
Maze-Foley, K., Byrd, B. L., Horstman, S. C., and Powell, J. R. (2019). Analysis of stranding data to support estimates of mortality and serious injury in common bottlenose dolphin (Tursiops truncatus truncatus) stock assessments for the Atlantic Ocean and Gulf of Mexico. Miami, Florida: NOAA Technical Memorandum NMFS-SEFSC-742, 42. doi:10.25923/jt1x-jv75
McBride-Kebert, S., Taylor, J. S., Lyn, H., Moore, F. R., Sacco, D. F., Kar, B., et al. (2019). Controlling for survey effort is worth the effort: Comparing bottlenose dolphin (Tursiops truncatus) habitat use between standardized and opportunistic photographic-identification surveys. Aquat. Mamm. 45, 21–29. doi:10.1578/AM.45.1.2019.21
McCluskey, S. M., and Lewison, R. L. (2008). Quantifying fishing effort: A synthesis of current methods and their applications. Fish. Fish. (Oxf). 9, 188–200. doi:10.1111/j.1467-2979.2008.00283.x
Moore, K. T., and Barco, S. G. (2013). Handbook for recognizing, evaluating, and documenting human interaction in stranded cetaceans and pinnipeds. La Jolla, California: U. S. Department of Commerce, NOAA Technical Memorandum, NOAA-TM-NMFS- SWFSC-510, 102p.
Moore, M. J., Mattila, D., Landry, S., Coughran, D., Lyman, E., Smith, J., et al. (2018). “Whale entanglement response and diagnosis,” in CRC handbook of marine mammal medicine. Editors F. M. D. Gulland, L. A. Dierauf, and K. L. Whitman. Third Edition (Boca Raton, FL, USA: CRC Press), 37–45.
NOAA (2020). “Examiner’s guide, 2020 revision,” in Marine mammal stranding report – level A (NOAA Form 89-864, OMB No. 0648-0178) (National Oceanic and Atmospheric Administration). Available at: https://media.fisheries.noaa.gov/dam-migration/examiners_guide_2023.pdf (Accessed May 19, 2022).
NOAA Fisheries (2017). Marine mammal unusual mortality events. Available at: https://web.archive.org/web/20171113014901/http://www.nmfs.noaa.gov/pr/health/mmume/events.html (Accessed May 19, 2022).
NOAA Office of Response and Restoration (2017). National enironmental sensitivity Index shoreline: GULF/ATLANTIC esi, pacific esi: ESIL (ESI shoreline types - lines). Available at: http://response.restoration.noaa.gov/esi_download.
Nowacek, D. P., Thorne, L. H., Johnston, D. W., and Tyack, P. L. (2007). Responses of cetaceans to anthropogenic noise. Mamm. Rev. 37, 81–115. doi:10.1111/j.1365-2907.2007.00104.x
Obusan, M. C. M., Rivera, W. L., Siringan, M. A. T., and Aragones, L. V. (2016). Stranding events in the Philippines provide evidence for impacts of human interactions on cetaceans. Ocean. Coast. Manag. 134, 41–51. doi:10.1016/j.ocecoaman.2016.09.021
Pitchford, J. L., Garcia, M., Pulis, E. E., Ambert, A. M., Heaton, A. J., and Solangi, M. (2018). Gauging the influence of increased search effort on reporting rates of bottlenose dolphin (Tursiops truncatus) strandings following the deepwater horizon oil spill. PLoS One 13, e0199214–e0199219. doi:10.1371/journal.pone.0199214
Powell, J. R., Machernis, A. F., Engleby, L. K., Farmer, N. A., and Spradlin, T. R. (2018). Sixteen years later an updated evaluation of the impacts of chronic human interactions with bottlenose dolphins (Tursiops truncatus truncatus) at Panama City, Florida, USA. J. Cetacean Res. Manag. 19, 79–93.
Read, A. J., Drinker, P., and Northridge, S. (2006). Bycatch of marine mammals in U.S. And global fisheries. Conserv. Biol. 20, 163–169. doi:10.1111/j.1523-1739.2006.00338.x
Read, A. J. (2008). The looming crisis: Interactions between marine mammals and fisheries. J. Mammal. 89, 541–548. doi:10.1644/07-MAMM-S-315R1.1
Russell, M. L., Bloodgood, J. C. G., and Carmichael, R. H. (2022). Spatial, temporal and demographic patterns of cetacean strandings in the northcentral Gulf of Mexico. J. Cetacean Res. Manage. 23, 171–182.
Samuels, A., and Bejder, L. (2004). Chronic interaction between humans and free-ranging bottlenose dolphins near Panama City Beach, Florida, USA. J. Cetacean Res. Manag. 6, 69–77.
Schwacke, L. H., Twiner, M. J., De Guise, S., Balmer, B. C., Wells, R. S., Townsend, F. I., et al. (2010). Eosinophilia and biotoxin exposure in bottlenose dolphins (Tursiops truncatus) from a coastal area impacted by repeated mortality events. Environ. Res. 110, 548–555. doi:10.1016/j.envres.2010.05.003
Simeone, C. A., and Moore, K. M. T. (2018). “Appendix 5: International stranding networks,” in CRC handbook of marine mammal medicine. Editors F. M. D. Gulland, A. Dierauf, Leslie, and K. L. Whitman. Third Edition (Boca Raton, FL, USA: Taylor & Francis Group, LLC), 1035–1085.
Spradlin, T. R., Barre, L. M., Lewandowski, J. K., and Nitta, E. T. (2001). Too close for comfort: Concern about the growing trend in public interactions with wild marine mammals. Mar. Mammal. Soc. Newsl. 9, 1–6.
Symons, J., Sprogis, K. R., and Bejder, L. (2018). Implications of survey effort on estimating demographic parameters of a long-lived marine top predator. Ecol. Evol. 8, 10470–10481. doi:10.1002/ece3.4512
Tiongson, A. J. C., Utzurrum, J. A., and De la Paz, M. E. L. (2021). Patterns of research effort and extinction risk of marine mammals in the Philippines. Front. Mar. Sci. 8, 607020. doi:10.3389/fmars.2021.607020
Twiner, M. J., Flewelling, L. J., Fire, S. E., Bowen-stevens, S. R., Gaydos, J. K., Johnson, C. K., et al. (2012). Comparative analysis of three brevetoxin-associated bottlenose dolphin (Tursiops truncatus) mortality events in the Florida panhandle region (USA). PLoS One 7, 429744–e43019. doi:10.1371/journal.pone.0042974
Vail, C. S. (2016). An overview of increasing incidents of bottlenose dolphin harassment in the gulf of Mexico and possible solutions. Front. Mar. Sci. 3, 1–7. doi:10.3389/fmars.2016.00110
Venn-Watson, S., Garrison, L., Litz, J., Fougeres, E., Mase, B., Rappucci, G., et al. (2015). Demographic clusters identified within the northern Gulf of Mexico common bottlenose dolphin (Tursiops truncates) unusual mortality event: January 2010 - june 2013. PLoS One 10, 01172488–e117313. doi:10.1371/journal.pone.0117248
Welch, B. L. (1947). The generalization of ‘student’s’ problem when several different population varlances are involved. Biometrika 34, 28–35. doi:10.1093/biomet/34.1-2.28
Wells, R. S., Allen, J. B., Lovewell, G., Gorzelany, J., Delynn, R. E., Fauquier, D. A., et al. (2015). Carcass-recovery rates for resident bottlenose dolphins in Sarasota Bay, Florida. Mar. Mamm. Sci. 31, 355–368. doi:10.1111/mms.12142
Westfall, P. H. (1997). Multiple testing of general contrasts using logical constraints and correlations. J. Am. Stat. Assoc. 92, 299–306. doi:10.1080/01621459.1997.10473627
Wilkin, S. M., Rowles, T. K., Stratton, E., Adimey, N., Field, C. L., Wissmann, S., et al. (2017). Marine mammal response operations during the Deepwater Horizon oil spill. Endanger. Species Res. 33, 107–118. doi:10.3354/esr00811
Wilkinson, D. M. (1991). Report to Assistant Administrator for Fisheries: Program review of the marine mammal stranding networks. Silver Spring, MD.
Williams, R., Gero, S., Bejder, L., Calambokidis, J., Kraus, S. D., Lusseau, D., et al. (2011). Underestimating the damage: Interpreting cetacean carcass recoveries in the context of the Deepwater Horizon/BP incident. Conserv. Lett. 4, 228–233. doi:10.1111/j.1755-263X.2011.00168.x
Worthy, G. A. J. (1998). “Patterns of bottlenose dolphin, Tursiops truncatus, strandings in Texas,” in NOAA tech. Rep. NMFS, 47–55.
Keywords: human interaction, mortality, search effort, deepwater horizon oil spill, marine mammals
Citation: Carmichael RH, Hodanbosi MR, Russell ML and Wingers NL (2022) Human influence on bottlenose dolphin (Tursiops truncatus) strandings in the northern Gulf of Mexico. Front. Environ. Sci. 10:951329. doi: 10.3389/fenvs.2022.951329
Received: 23 May 2022; Accepted: 31 October 2022;
Published: 17 November 2022.
Edited by:
Brian Joseph Roberts, Louisiana Universities Marine Consortium, United StatesReviewed by:
Christina Toms, Chicago Zoological Society, United StatesHolley Muraco, Mississippi Aquarium, United States
Copyright © 2022 Carmichael, Hodanbosi, Russell and Wingers. This is an open-access article distributed under the terms of the Creative Commons Attribution License (CC BY). The use, distribution or reproduction in other forums is permitted, provided the original author(s) and the copyright owner(s) are credited and that the original publication in this journal is cited, in accordance with accepted academic practice. No use, distribution or reproduction is permitted which does not comply with these terms.
*Correspondence: Ruth H. Carmichael, rcarmichael@disl.org