- 1College of Resources and Environmental Sciences, National Academy of Agriculture Green Development, China Agricultural University, Beijing, China
- 2College of Horticulture, Fujian Agriculture and Forestry University, Fuzhou, China
- 3Institute of Soil and Environmental Sciences, University of Agriculture Faisalabad, Faisalabad, Pakistan
- 4Department of Agronomy, Ghazi University, Dera Ghazi Khan, Pakistan
- 5Department of Agronomy, University of New England, Armidale, NSW, Australia
- 6Botany and Microbiology Department, College of Science, King Saud University, Riyadh, Saudi Arabia
- 7Faculty of Agriculture (Saba Basha), Alexandria University, Alexandria, Egypt
- 8Plant Protection and Biomolecular Diagnosis Department, Arid Lands Cultivation Research Institute, City of Scientific Research and Technological Applications, Borg El-Arab, Alexandria, Egypt
Phosphorus (P) constraint can be alleviated by increasing C inputs, which can help to improve crop production and P fertilizer use efficiency. However, the effects of different manures on soil microbial biomass P (MBP) and P fractions as well as C fractions in calcareous soils remain poorly understood. Soil MBP pool involves the P mineralization and immobilization processes, potentially changing P fractions and P availability. Therefore, the effects of different manures on soil microbial biomass (MBP, MBC) pool, P, and C fractions and crop P utilization were evaluated in greenhouse experiments with maize plantation. Treatments included no manure (control), poultry manure (PM), cow manure (CM), goat manure (GM), mixed manure (MM), and three inorganic P (Pi) rates; P0: 0 mg kg-1, P50: 50 mg kg-1, and P100: P100 mg kg-1 (P2O5). For plant growth comparison, crop physiological growth indices, shoot P contents and total P uptake were increased by PM and P100 as compared to other treatments. The PM with P100 significantly increased the plant growth by inducing P uptake of ∼18% compared with control. The results exhibited that Pi (P100) combined with manure (PM) significantly (p < 0.05) increased the soil physicochemical properties, that is, 683.76 mg kg-1 total P, 21.5 mg kg-1 Olsen P, 4.26 g kg-1 SOC, 2.41 g kg-1 POC, as well as microbial biomass C and P increased by 152.84 mg kg-1 and 36.83 mg kg-1, respectively. Consequently, we concluded that PM with Pi (P100) application builds up soil microbial biomass, which is more beneficial for promoting P utilization for maize.
Introduction
Phosphorus deficiency is the world’s second most serious soil fertility issue and a major constraint to agricultural productivity (Gichangi et al., 2011). In Pakistan, soils have low organic matter levels, ranging from 2.6 to 6.4 mg C g−1, and extracted phosphate (NaHCO3), about 6 μg P g−1 (Khan and Joergensen 2009). Low P availability in soil (pH 8.2–9.3) is mainly due to the rapid formation of non-labile P forms after inorganic P (Pi) addition (Khan and Joergensen 2012). P precipitated and adsorbed with native soil P and calcium, iron, and aluminum ions which become unavailable to plants. According to Huang et al. (2017), the Olsen P contents in soil ranging from 5 to 10 mg kg−1 would be optimum for crop production, while lower contents (<5 mg kg−1) affect plant growth.
Many agricultural soils require a substantial amount of costly fertilizer to achieve adequate crop production (Muhammad et al., 2007). This causes serious environmental challenges such as P leaching, soil acidity, and soil hardness, which reduce soil fertility (Ahmed et al., 2019). To address these issues, Khan et al. (2019) found the impact of the combined application of organic addition with various inorganic inputs on soil fertility and rice production. Their findings show that the cumulative use of organic and inorganic fertilizer increased P availability and crop yield. The inorganic fertilizer’s use efficiency could be decreased by improving soil fertility with organic manure (Ayaga et al., 2006). The combination of inorganic and organic amendments such as farmyard manure and crop residues is recommended to overcome soil fertility challenges (Biswas et al., 2017).
Organic amendments, including farmyard, poultry, and goat manure, are commonly used to mitigate soil alkalinity by improving the nutrient supply and water retention capacity of soils (Ojo et al., 2016). The P availability for plants was increased by adding organic amendments to calcareous soils (Huang et al., 2017). This was attributed to the release of organic acids contending with phosphates for adsorption sites on the soil colloid’s surface or P mineralization during decomposition. Many cumulative mechanisms might be involved, such as soil pH improvement and inorganic P release from decomposing residues (Chen et al., 2016). There is considerable information that applying organic manure to soil can increase P solubility, decrease P fixation, and improve P availability to plants (Bader et al., 2020).
Soil microbial biomass is a living component of soil organic matter and an active pool for plant-available N and P. Several scientists have previously documented increases in microbial biomass carbon after adding organic amendments to the soil, such as compost, poultry manure, and compost (Marschner, 2008). Phosphorus (P) incorporation into the microbial biomass and its associated pool are reported mechanisms that substantially increase P availability in soil-plant systems (Muhammad et al., 2007). The use of organic manure and inorganic P together promotes soil microbial biomass growth and labile microbial P enriched metabolites, which serve as a labile P pool in soil (Brookes, 2001). During the microbial biomass turnover process, this phosphorus could be released slowly and more efficiently taken up by the plants (Kouno et al., 2002). Several studies indicated that when organic manure and P fertilizer were applied together, the microbial biomass phosphorus (MBP) pool was larger than when P fertilizer or organic manure were applied separately (Reddy et al., 2005; Ayaga et al., 2006; Khan and Joergensen 2009; Li and Marschner 2019; Peng et al., 2021). According to most studies, calcareous soils have a negative impact on plant development by restricting root carbon release into the soil (Zhang et al., 2016). As a result, soil alkalinity causes a decrease in soil microbial biomass (Halvorson et al., 1990).
Soil organic carbon (SOC) is the largest C pool in the terrestrial ecosystem, which directly or indirectly affects the soil’s physicochemical and biological properties (Kan et al., 2020; Zhao et al., 2020). Particulate organic matter (POC) is a labile fraction of SOC that could reflect initial changes in the soil immediately as compared to other fractions. Furthermore, mineral-associated organic carbon (MAOC) is a relatively non-labile fraction associated with silt and clay-sized particles, and it is primarily composed of low molecular weight compounds derived from microbial activities (Kleber et al., 2015). Soil fertility is influenced due to variations in SOC under organic and inorganic amendments (Lal 2013).
Soil phosphorus forms and their distributions in the soil vary under different sources of manure application, including compost and animal manure (Schachtman et al., 1998). The soil’s chemical characteristics are potentially affected by the application of different types of manure sources, which change the quantity and distribution of distinct soil P forms. Calcium-bounded phosphorus is the dominant fraction and is frequently present in calcareous soils (Khan and Joergensen, 2009). Calcium phosphate minerals were divided into three groups: 1) Ca2-P (brushite and monetite) is water-soluble P, which is readily available to plants; and 2) Ca8-P (b-tricalcium and P octacalcium P) is a moderately labile P fraction that can be used by selective plants (Jiang and Gu 1989); and 3) Ca10-P (hydroxyapatite), is a stable form that is unavailable to plants. Al-P and Fe-P are less available to plants, and occluded P (goethite) is not available to plants (Adhikari and Pandey 2019). In maize soils, organic and Pi addition can influence the total P storage and create changes in soil P contents, which could provide useful information on the effects of P inputs and P transformations (Linu et al., 2019). In P-limited soil conditions, the bioavailability of both the soil organic and inorganic P chemical fractions can be different (Bol et al., 2016). Consequently, exploring the behaviors of soil P at the expense of organic and inorganic inputs is critical to clarifying the P fraction distribution and maize P uptake mechanism in calcareous soil.
Recent studies have resulted that P-enriched organic treatments could improve P availability and plant growth more than the sole addition of Pi fertilizers (Aziz et al., 2010). This might be mainly because of microorganisms that act as a sink for inorganic P during the decomposition of soil organic matter or organic amendments (Rawat et al., 2020). According to Muhammad et al. (2007), the addition of P-enriched compost significantly increased P availability and maize yield, possibly due to higher microbial growth and turnover rate. Some studies have suggested that soil microbial activities are quite linked with SOC, P fractions, and utilization in different soil systems.
However, the relationship of soil microbial pools with SOC and P factions has not been fully understood. Therefore, this study was conducted to evaluate the response of SOC, P fraction, and microbial pool as well as plant growth. We hypothesized that organic amendments could induce microbial activity by providing soil C as an energy source, and in return, microbes could acquire more P from the soil Pi pool, thereby increasing plant growth. The basic objectives of this study are 1) to evaluate variation in MBP and P fractions in maize soil under inorganic and organic applications to better understand soil P behavior; and 2) to assess the relationship between SOC and P fractions and the plant’s P uptake.
Materials and methods
Collection and pre-analysis sample
Organic manures used in this study were collected from a livestock farm located at the University of Agriculture in Faisalabad, Pakistan, and were sieved (2 mm) before the start of the experiment in order to ensure a homogeneous product. A subsample of manure is sieved and dried at 65°C for elemental analysis by following standard procedures (Peters et al., 2003). Soil samples were collected from the university ISES research farm (Table 1).
Growth conditions and plant material
A pot trial was set up in the greenhouse of the Institute of Soil and Environmental Sciences, University of Agriculture Faisalabad, Pakistan. The study treatments were organized in a completely randomized design (CRD) with four replicates using organic C sources (goat, cow, poultry, and mixed manures) and inorganic P (P2O5 form) applied at three different application rates, that is, P0 = 0, P50 = 50, and P100 = 100 mg kg−1 by using diammonium phosphate (DAP) fertilizer source. The treatments consisted of: no manure (control treatment with P0, P50, and P100 mg P2O5 kg−1; first, goat manure (GM) with P0, P50, and P100 mg kg−1 P2O5; second cow manure (CM) with P0, P50, and P100 mg kg−1 P2O5; third, poultry manure (PM) with P0, P50, and P100 mg kg−1 P2O5 and finally, mixed manure (MM) fertilization with P0, P50, and P100 mg kg−1 P2O5. The mixed manure (MM) was developed by the combination of all of the three aforementioned manures in an equal ratio (1:1:1). Considering a manure input rate of 5% (w/w) (oven-dried weight) was taken. The soil was thoroughly mixed and three maize seeds (cv Nilam) were initially sown in each plastic pot (32 cm in diameter, 24 cm in depth). Water holding capacity was maintained at 50%. In addition, the recommended dose of nitrogen and potassium were applied to the pots at the rate of 120:40 kg ha−1 in split doses for normal plant growth, and development and to avoid nutrient deficiency (Reddy 2012). After 7 days of emergence, the plants were thinned to one plant per pot. Eight weeks of plants were harvested to measure the plant growth indexes. Plant height was measured by using a measuring tape. Leaf chlorophyll contents (SPAD) were recorded with a SPAD-502 m, while leaf area (LA) was recorded by using a measurement tape. Plant roots were removed gently from each pot and washed to remove soil particles and allowed to be stored in paper bags. Furthermore, an electric balance was used to measure the fresh root and shoot weights.
Determination of plant nutrient uptake
Shoots and roots were carefully washed using water (distilled) and were dried in an oven (105°C) for 1 h and then at 70°C to ensure final weight. Dry shoot and root samples of 200 mg were processed with sulfuric acid 8 ml in a sealed chamber (Li and Marschner 2019). Total P concentration in dried samples was measured with the vanadate molybdate method by visible spectrophotometer/UV as recommended by El-Gizawy et al. (1992). Total P uptake was calculated from the formula given below;
After the final harvest (56 days), the soil sample (50 g moist bases) was taken and analyzed for different P fractions, microbial biomass C, and microbial biomass P contents. Additionally, samples collected (air-dried) were analyzed for soil available P.
Determination of microbial biomass C, P, and olsen P
Microbial biomass P was estimated by the chloroform fumigation extraction method (Brookes et al., 1982) in the 50 g sample taken from each treated pot. Three parts of soil weighing 5 g (oven-dried for 24 h at 105°C), were separated into a 50 ml flask. One part was untreated, the second part was treated with P KH2PO4, and the third part was fumigated (24h, 25°C) with ethanol-free chloroform. These three soil parts were extracted with 0.5 M NaHCO3 by shaking at 150 rev min−1 for 30 min and filtered by using a 0.45 μm filter paper. MBP was calculated by using a 0.4 conversion digit. The MBC was analyzed by the chloroform fumigation-extraction method and calculated as follows: C = EC/KEC, where EC is the difference between organic carbon extracted from fumigated and non-fumigated samples, and KEC is 0.4 (Wu et al., 1990). The Olsen-P concentration was analyzed by extracting 0.5 M NaHCO3 (8.5 pH) following Olsen (1954).
Determination of soil C fractions
For soil organic C analysis, the soil samples (oven-dried) were ground, sieved (0.25-mm), and analyzed as suggested by Walkley and Black, (1934). Particulate organic matter (POM) and mineral-associated organic carbon (MAOC) were analyzed by the method of Cambardella and Elliott, (1992). POM was determined by shaking 10 g of oven-dried soil for 18 h in 30 ml of sodium hexametaphosphate (5 g/L). The supernatant was passed through a sieve (53-μm). The retained material passed on the screen was oven-dried at 50°C for 24 h and used to measure C according to the aforementioned method. POC was determined as the carbon (C) content in the screen retained POM. Meanwhile, the screen-passed material was also measured for C and identified as mineral-associated organic carbon (MAOC).
Determination of soil pi fractions
According to Jiang and Gu (1989), soil inorganic P (Pi) was divided into distinct inorganic P fractions; this extraction method has been used for P fractionation of alkaline soils (Wang et al., 2010b). Different inorganic P fractions such as Ca2-P, Ca8-P, Ca10-P, Al-P, Fe-P, and O-P were extracted by following the sequential extraction method (Figure 1).
Briefly, the soil sample of about 0.5 g (oven-dried bases) was added into centrifuge tubes of 50 ml. By following the sequence shown in Figure 1, the selected extractants of 25 ml (with a defined pH) were taken into tubes and shaken by using an automated reciprocating shaker machine for the required time. After shaking, the tubes were allowed to centrifuge (10 min, 4500 rpm). After, completing the centrifugation process, the supernatants were transferred carefully into a new tube to avoid any contamination. Finally, the extracted supernatants were evaluated with a UV/visible spectrophotometer at 880 nm following the molybdate blue method of Murphy and Riley (1962) to analyze the inorganic phosphorus (Pi) contents in the soil fractions.
Statistical analysis
SPSS version 25.0 was used for the statistical analysis. Two-way ANOVA (analysis of variance) was used to analyze the influence of organic manure and inorganic P (Pi) on recorded data. To compare the cumulative effects of organic C and Pi amendments, the significance of the difference between treatments was evaluated by the HSD test (honestly significant difference test).
Results
Plant growth and P uptake
Inorganic and organic fertilization alone or in combination affect the growth indexes of maize. In this study, the combined application of PM and P100 significantly increased the maize growth attributes (Table 2). The average increase in plant height (117 cm), shoot fresh weight (101 g), shoot dry weight (13 g), root fresh weight (33.34 g), root dry weight (4.8 g), and leaf area (422 cm2) was observed under the combined application of PM and P100 to the maize plant. The chlorophyll content of maize leaf increased under the cumulative effect of manures and Pi than that of control (Table 2). The addition of PM and P100 together increased the 180 mg P uptake by the plant compared to the control. Compared with the control, the P contents were significantly increased in the root (0.21%) and shoot (0.4%) of maize (Table 2). The P concentration in different plant parts of maize (shoot and root) were increased in the order of PM > CM > MM > GM > control, respectively (Table 2).
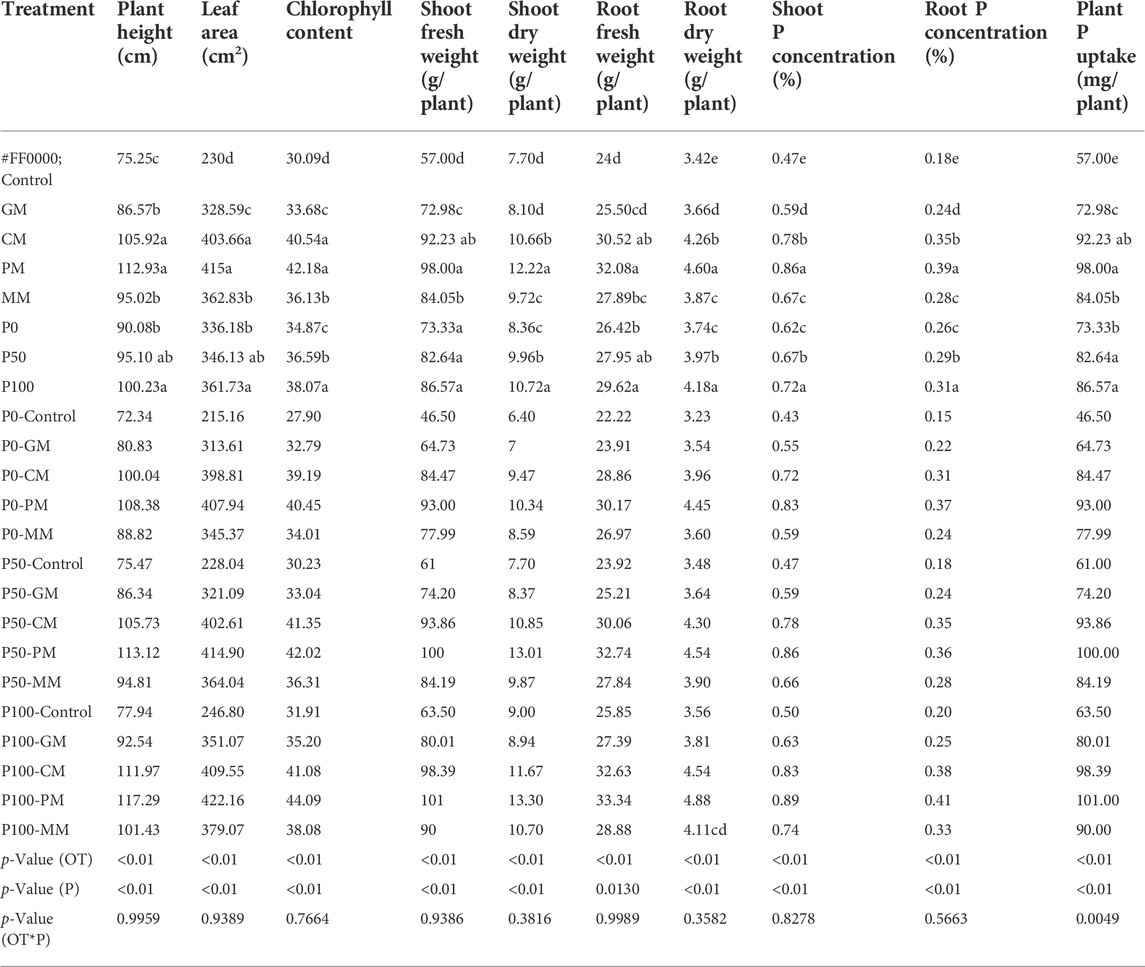
TABLE 2. Maize plant growth characteristics and P uptake under different manure types and inorganic P rates.
Variation in soil pi fractions
In the current study, the size of the individual P pool was significantly (p < 0.05) affected by organic and inorganic amendments (Table 3). The ANOVA demonstrated a significant effect of the treatments with inorganic P (Pi) addition rates of P0, P50, and P100 on the relative proportion of extracted P in bulk soil fractions. The overall organic treatment effect indicated the increasing trends, that is, PM > CM > GM > MM > control. The PM showed a significant increase in the Pi fractions NaHCO3-P, NH4Ac-P, NH4F-P, and NaOH-P occluded P (O-P), and Ca10-P in calcareous soils.
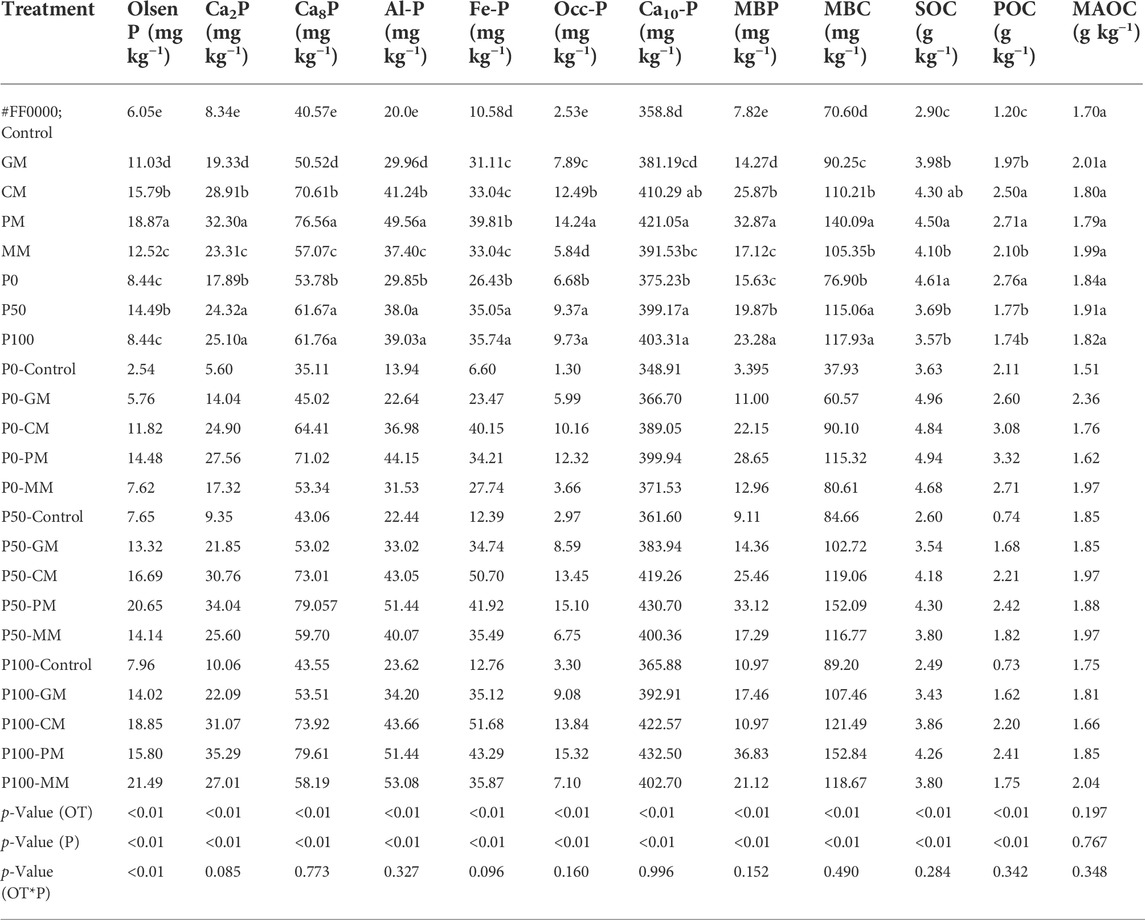
TABLE 3. Different phosphorus and carbon fractions distribution under different manure types and inorganic P rates.
In general, under no manure treatment (control) the average size of Pi fractions increased in the order of Ca8-P > Ca2-P (labile pool), Al-P > Fe-P (moderately labile pool), and Ca10-P > O-P (non-labile pool), respectively (Table 3). In the control treatment, the Ca2-P and C8-P were 1.89 and 9.16% of the total P in the soil (Figure 2). The P100 showed the highest increase in Ca2-P that was about 40.3%, whereas, the C8-P was increased by 14.8% (was more or less constant) as compared to P0. Moreover, Fe-P and Al-P constituted 2.31 and 4.51% of the total P in the soil in control, respectively (Figure 2). Compared with the control, the average increase of Fe-P was about 32.6% of the added P50 rate and 35.22% of the added P100 rate. The P50 and P100 exhibited the highest increase in Al-P about 27.3 and 30.75% with respect to control, respectively. In addition, P100 showed the maximum concentration of occluded P approximately, 46.96%. Similarly, P100 depicted the highest Ca10-P about 7.4% compared to P0. The occluded P and Ca10-P were 0.68 and 81.34% of the total P in the soil in control (Figure 2).
Data in Table 3 showed that the PM (p < 0.05) increased the concentration of Pi fractions in the following sequence: C8-P > Ca2-P, Al-P > Fe-P and Ca10-P > O-P. By applying PM, the Ca2-P and C8-P fractions were significantly increased, with a mean value of 32.30 and 76.56 mg kg−1, respectively. These fractions had a higher concentration of about 5.09% (Ca2-P) and 12.06% (C8-P) of the total soil P under PM (Figure 2). The increase in Ca2-P was observed in other organic manure that is 28.91 (CM), 19.33 (GM), 23.34 (MM), and 8.3 mg kg−1 (control). Whereas, the Ca8-P average increase by using other manure sources was about 70.61 (CM), 50.52 (GM), 57.08 (MM), and 40.57 mg kg−1 (control). The Ca2-P was increased by 17.89, 24.32, and 25.11 mg kg−1, while the Ca8-P was increased by 53.77, 61.67, and 61.76 mg kg−1 in P0, P50, and P100, respectively. The current study results showed that the Ca8-P was larger than the Ca2-P with a mean difference value of 44.29 mg kg−1 under the P100 and PM (Table 3). The significantly increased in Al-P with PM, the mean value was 49.27 mg kg−1, while other manures had mean values of 41.24 (CM), 29.95 (GM), 37.40 (MM), and 20 mg kg−1 (control) (Table 3). In addition, Al-P had almost 7.8% contribution to total P (Figure 2). Compared with P0, the maximum increase of Al-P was about 31% by P50, and similar results were observed with P100. When the PM and P100 were applied together, the average increase in Al-P contents was about 53.07 mg kg−1. The PM also significantly increase the Fe-P by about 36.92 mg kg−1 compared to the control. This fraction made up 6.28% of total P in soil (Figure 2). By using other manure, the mean value of Fe-P was approximately 47.51 (CM), 31.10 (GM), 33.03 (MM) and 10.57 mg kg−1 (control), respectively. The P0, P50, and P100 significantly increased the Fe-P concentration by approximately 26.42, 35.08, and 35.73 mg kg−1, respectively. In this study, the combined application of P100 and PM significantly increased the Fe-P fraction by almost 43.29 mg kg−1. In comparison to the labile and moderately labile pools, the occluded P (O-P) was slightly affected by PM addition (Table 3). The PM increased the O-P contents by 13.89 mg kg−1, which accounted for 2.24% of total P in soil (Figure 2). The O-P fraction was significantly affected with other manure types approximately of 12.49 (CM), 7.89 (GM), 5.84 (MM), and 2.53 mg kg−1 (control). The PM addition and P100 together increased the O-P concentration by about 14.02 mg kg−1, as compared to the control. The O-P increased about 45.75% under P100 (same for P50) compared to P0. Moreover, the Ca10-P was 66.46% of the total soil P (Figure 2). By PM, the highest Ca10-P contents (17.59%) were observed with respect to control. The PM and P100 together increased the Ca10-P concentration by about 432.50 mg kg−1. Whereas, the Ca10-P concentration increased about 410.26 (CM), 381.50 (GM), 391.53 (MM) and 358.8 mg kg−1 (control). In P0, P50, and P100, the Ca10-P fraction was increased by almost 375.23, 399.17, and 403.31 mg kg−1, respectively.
Variation in soil C fractions, olsen P and microbial biomass indices
The SOC, POC, and MAOC concentrations varied between the different organic amendments. The SOC fraction increased in the following order: PM > CM > MM > GT > control (Table 3). The maximum SOC was 4.2 g kg−1 under the combined addition of P100 and PM. Compared to the control, the average increase in SOC was about, 1.6 (PM), 1.4 (CM), 1.08 (GM), and 1.2 (MM) g kg−1. The Pi had increased the SOC, approximately 4.61, 3.69, and 3.57 g kg−1 under P0, P50, and P100, respectively. The different organic manure additions significantly increased POC that ranging between 1.2 and 2.7 g kg−1 (Table 3). The Pi significantly (p < 0.0 5) increased the POC that was 1.77 g kg−1 under P50 and 1.74 g kg−1 under P100. The higher POC was 2.4 g kg−1observed in the combined addition of P100 and PM. The effect of treatments on MAOC fraction increased in the following order: GT > MM > CM > PM > control (Table 3). Overall, the maximum C fractions size increased in PM by the following order: SOC > POC > MAOC.
The different organic manure amendments with Pi caused variations in Olsen P, MBC, and MBP (Table 3). The maximum Olsen P 21.5 mg kg−1 was observed by the combined addition of PM and P100. The Olsen P was increased by about 72 and 85% under P50 and P100. The average increase of Olsen P was 18.88 (PM), 15.79 (CM), 11.04 (GM), and 12.51 (MM) mg kg−1 than that of control (Table 3). Collectively, the MBC and MBP pools were higher at almost 69.4.5, 39.9, 20.25, 34.75 mg kg−1, and 25.07, 18.07, 6.47, 9.32 mg kg−1 in PM, CM, GM, MM in comparison to control, respectively (Table 3).
Discussion
Changes in soil properties (SOC, POC, and olsen P)
In our study, organic and inorganic fertilization significantly (p < 0.05) affected the soil chemical properties, microbial biomass indices, and inorganic P (Pi) fractions (Table 3), that could be due to the manure types and P application rates. Many previous studies have presented that the Pi forms are mainly influenced by microbial activities and soil chemical properties (Spohn and Widdig, 2017). Furthermore, the soil physicochemical properties significantly affected the microbial biomass and Pi fraction in calcareous soil. Among different organic amendments, PM had the greater effect on soil chemical and biological properties, resulting in native soil P lability. This was mainly due to organic amendments chemical composition, where PM had higher organic-P contents as compared to other amendments (CM, GM). Moreover, higher microbial activities (MBC, MBP) under PM might increase the soil available and total P by inducing SOC and POC concentration in the soil (Table 3). In the present research, total soil P (683.76 mg kg−1) and Olsen-P (21.5 mg kg−1) contents were highest in the PM with P100 (Figure 2; Table 3). According to Hassan et al. (2012), the critical Olsen-P level for wheat and maize growth is 14.6 and 13.2 mg kg-1, respectively. This study achieves quite a similar Olsen-P threshold hold levels with PM and P100 applications. The significant increase in the Olsen-P showed a positive correlation with Pi fractions pools, showing that the combined organic and inorganic fertilizer addition had positive effects on Olsen-P contents. This might be due to the organic manures being sufficient in P supply and organic manures with inorganic P are prevented from the adsorption and insoluble complexes in the soils (Chen et al., 2006). Moreover, organic inputs mobilized native soil P by accelerating microbial activities (Kouno et al., 2002).
Overall, organic amendments significantly stimulated the soil microbial pool by increasing the availability of C sources (POC). Among other amendments, PM had a higher moderately labile (Ca8-P) fraction, possibly due to higher nutrient contents, POC availability, and water holding capacity (Hoover et al., 2019). The MAOC had no relationship with microbial activities because that was known to be a recalcitrant C and a non-usable pool (Virk et al., 2021). This indicates that the only labile pool of C in the organic manures could increase P availability by inducing microbial activity. Natural manure fertilization is the source of essential nutrients for plants. Plants require P in the vegetative, and during reproductive development (Richardson et al., 2009). The data in Table 2 shows that plant biomass is considerably affected by both manures and Pi. The higher amount of approximately 236 mg of P uptake by the maize plant was estimated as compared to CM (197.74 mg) and GM (113.54 mg) under the dose rate of P100 (Table 2). This might be because PM contains about three to four times higher P concentration than manure from other livestock, except for pigs (Kleinman et al., 2005), which was also seen in the current study. The nutrient concentration for all types of manure varied when compared to other published studies, which was probably attributed to dietary habits, animal age, and the season (Penhallegon 2003; Brown 2013). In addition, this higher plant P uptake might be due to PM-treated soil having the potential to increase the labile SOC pool mainly POC, resulting in higher microbial activities and higher P availability (Ca8-P) inducing plant growth. Similarly, our results are also consistent with the previous research, which reported that applied Pi is mostly transformed into Ca2-P (more available for plant growth) instead of Ca8-P in calcareous soil (Marriott and Wander 2006; Liebisch et al., 2014). Furthermore, Hassan et al. (2012), found that organic input with Pi leads to a synergistic effect on plant growth compared to the application of the sole fertilizer in calcareous soils. The labile P and moderately labile P are always known as bioavailable P forms for plant uptake. Consequently, it improves the physicochemical and biological soil conditions for plant growth and development.
Changes in microbial biomass indices
Soil microbial dynamics are affected by various organic amendments. In C and P deficient soils, the microbial community acts more effectively when energy source C is added. A significantly higher MBC of 152.8 mg kg−1 was observed under the cumulative effect of organic and inorganic amendments (Table 3). In addition, a significant positive relationship between MBC with Pi fractions and a negative correlation with SOC and POC was observed, suggesting that an increase in MBC at the expense of SOC and POC resulted in P release from Pi fractions, in parallel to these results. Wu et al. (1990) reported that MBC pool mainly depends on the organic C availability. Moreover, the native microbial population is stimulated in response to the Pi addition (Ayaga et al., 2006). However, the relationship of MBC to organic C is inconsistent, showing either a positive or no correlation due to the microbial community structure and organic C quality (Brookes 2001).
The SOC and POC had a positive correlation with MBP, the increase in microbial biomass indicating the strong potential to accumulate radially available P into their bodies (Spohn and Widdig, 2017). The maximum increase in MBP of 36.8 mg kg−1 due to the cumulative effect of organic manure and Pi amendments was observed (Table 3). As suggested before, organic amendments induce MBC (in response to POC and SOC increment), but Pi with organic amendments could stimulate MBP and Olsen-P availability (Tang et al., 2014). An important finding was that the manure-treated soil (PM) had a larger size of microbial biomass C and microbial biomass P than that of other organic manures. This might be due to the difference in microbial P acquisition ability in different manure sources, or might be due to the difference in availability of C and P in manure sources (Kouno et al., 2002). In the current study, microbial biomass dynamics showed variations in different organic amendments, indicating that microbial activity depends on organic C availability (Nyawade et al., 2019).
Changes in soil P fractionation
The application of Pi and different manure together (p < 0.05) enhanced the P concentration in soil, this result is consistent with Zhang et al. (2021). P input increased the size of all Pi fractions such as the labile, moderately-labile, and non-labile (Table 3). Our study describes that the manure applied to soil has a direct influence on P availability. This was possibly due to different Pi amendment rates and manure having various impacts on P immobilization and its mobilization in calcareous soils (Khan and Joergensen 2009).
The Ca-P fraction was identified as the dominant P fraction in calcareous soil. Soil Pi forms Ca2-P considered as labile P or bio-available P form for plant uptake (Jiang and Gu 1989). In the current study, Ca2-P accounted for 5.09%, of total P as shown in Figure 2 by the combined addition of PM and P100. This indicated that the available P supply ability was well maintained by the Pi input combined with manure over inorganic input only (control with P100). According to Delgado et al. (2002), P fixation with Ca-P reduces the P accessibility for plant growth that may be available after the manure input into the soil, which has been proven in our PCA analysis (Figure 3). These study results are in accordance with Ahmed et al. (2019), which found that the Pi and pig manure addition increased the P labile forms by inducing microbial activities in response to C addition in soil. The average labile Pi content increases in the PM, possibly due to the higher SOC (4.5 g kg−1) and POC (2.7 g kg−1) concentration in the soil (Virk et al., 2021), that might move the P adsorption sites by exchanging the clay mineral surface charges, decreasing the sorption sites and improving the soil P availability. Noticeably, the association of Pi fractions and soil carbon (SOC, POC) also indicated that the input of organic manure reduced the Pi fixation and increase Ca2-P availability (Delgado et al., 2002).
The inorganic fractions (Ca8-P, Fe-P, and Al-P) were known as the moderately labile P pool. Ca8-P, Fe-P, and Al-P fractions accounted for 79.61, 43.29, and 50.03 mg kg−1, respectively, with the PM and Pi. During the manure decomposition process, organic acids are released, which lead to chemical bonding of P with minerals ions (Fe and Al), decreasing the soil P availability. As the labile P, the moderately labile P also improved by the PM, compared to the other manure source and control (Table 3). That is possibly due to the high nutrient content in PM mainly POC and SOC as compared to other manures (GM, CM), which have higher nutrients and water holding capacity. Several studies also resulted that the various source of organic amendments increased the moderately labile Pi pool as compared to inorganic fertilizer (Li et al., 2012). Malik et al. (2012) identified that manure sources containing P content can have synergetic effects on increasing organic P content and retention in soils that could be due to the P adsorption in organic matter that is transformed into inorganic P by plant roots. In calcareous soil, the Ca8-P fraction is mostly greater than Ca2-P this may explain that Ca8-P is more stable in P-limited soil and it needs higher Pi in the soil for the solution to transform into Ca2-P, which may not be possible under low Pi addition (Lindsay et al., 1962; Ayaga et al., 2006). However, the increase in the moderately labile P pool in the combined use of PM and Pi may explain the slow P release from an organic source which can contribute to the P cycling process in soil.
The non-labile P fractions which are stable and insoluble P form, such as occluded P (O-P) and apatite P (Ca10-P), are known as the unavailable P pools for plant uptake (Cao et al., 2020). The Ca10-P fraction is dominated by all the P fractions in the soils. Noticeably, it had a significant correlation with microbial index, this might be due to higher organic source amendments (Yong-Fu et al., 2008). The Ca10-P is the recalcitrant pool due to geochemical properties on P cycling and higher CaCO3 contents in the soils, as suggested by Mehmood et al. (2015). Our results support the study of Zhang et al. (2021), who suggested that adding P fertilizer had a slight influence on the residual P forms but substantially enhanced the soluble Pi. While the stable P was supposed to be less available P pool, the variation in this P pool shows P cycling on a long-term basis in the soil system (Huang et al., 2017). It was concluded by Meason et al. (2009) that applying P adsorbed on the surface of the mineral can contribute to increasing the stable pool. Our study resulted that non-labile P concentrations were higher in Pi with manure addition, that resulted in the P sorption under excessive Pi input, that may be transformed to the moderately or labile P pools over time.
Conclusion
The Poultry manure addition increased the plant growth, P uptake, and Ca8-P pool (after Ca2-P is depleted), by improving the total SOC contents and labile organic C (POC) pool in P-limited soil. Present research demonstrated that SOC and microbial biomass (MBP, MBC) are the key drivers to improve the soil P availability and the cumulative effect of PM and Pi is not only a better approach for P acquisition for the maize plant but also has a positive effect on the chemical properties (SOC, POC and Olsen P) of the soil. Indeed, the increase in the soil labile and moderately labile P fractions are likely due to MBP turnover processes mediated by PM. Therefore, we concluded PM with Pi promotes soil MBP pool, which in turn improves soil P availability and utilization efficiency in maize crops. This study shed light on the Pi addition rate with PM should be further evaluated for P balance and crop yield in field conditions.
Data availability statement
The raw data supporting the conclusions of this article will be made available by the authors, without undue reservation.
Author contributions
Conceptualization, KK, and GF; methodology, KK, MN, and MR; software, KK; validation, MA and MN; data curation, MN; writing—original draft preparation, KK; writing—review and editing, KK, MA, MN, MS, NA, HA, and RG; supervision, MN and GF.
Funding
This research was funded by Researchers Supporting Project number (RSP-2021/123) King Saud University, Riyadh, Saudi Arabia.
Acknowledgments
The first author is thankful to the technical staff of the Soil and Environmental Microbiology Laboratory for providing technical support during the study analysis. The authors would like to thank Alexandria University, Alexandria, Egypt for supporting the research. Also the authors would like to extend their appreciation to Researchers Supporting Project number (RSP-2021/123) King Saud University, Riyadh, Saudi Arabia.
Conflict of interest
The authors declare that the research was conducted in the absence of any commercial or financial relationships that could be construed as a potential conflict of interest.
Publisher’s note
All claims expressed in this article are solely those of the authors and do not necessarily represent those of their affiliated organizations, or those of the publisher, the editors, and the reviewers. Any product that may be evaluated in this article, or claim that may be made by its manufacturer, is not guaranteed or endorsed by the publisher.
References
Adhikari, P., and Pandey, A. (2019). Phosphate solubilization potential of endophytic fungi isolated from Taxus wallichiana Zucc. roots. Rhizosphere 9, 2–9. doi:10.1016/j.rhisph.2018.11.002
Ahmed, W., Jing, H., Kaillou, L., Qaswar, M., Khan, M. N., Jin, C., et al. (2019). Changes in phosphorus fractions associated with soil chemical properties under long-term organic and inorganic fertilization in paddy soils of southern China. Plos One 14, 1–17. doi:10.1371/journal.pone.0216881
Ayaga, G., Todd, A., and Brookes, P. C. (2006). Enhanced biological cycling of phosphorus increases its availability to crops in low-input sub-Saharan farming systems. Soil Biol. Biochem. 38, 81–90. doi:10.1016/j.soilbio.2005.04.019
Aziz, T., Ullah, S., Sattar, A., Sciences, E., and Farooq, M. (2010). Nutrient availability and maize ( Zea mays ) growth in soil amended with organic manures. Int. J. Agric. Biol. 12.
Bader, A. N., Salerno, G. L., Covacevich, F., and Consolo, V. F. (2020). Native Trichoderma harzianum strains from Argentina produce indole-3 acetic acid and phosphorus solubilization, promote growth and control wilt disease on tomato (Solanum lycopersicum L.). J. King Saud Univ. - Sci. 32, 867–873. doi:10.1016/j.jksus.2019.04.002
Biswas, S., Hazra, G. C., Purakayastha, T. J., Saha, N., Mitran, T., Singha Roy, S., et al. (2017). Establishment of critical limits of indicators and indices of soil quality in rice-rice cropping systems under different soil orders. Geoderma 292, 34–48. doi:10.1016/j.geoderma.2017.01.003
Bol, R., Julich, D., Brödlin, D., Siemens, J., Kaiser, K., Dippold, M. A., et al. (2016). Dissolved and colloidal phosphorus fluxes in forest ecosystems—An almost blind spot in ecosystem research. J. Plant Nutr. Soil Sci. 179, 425–438. doi:10.1002/jpln.201600079
Brookes, P. (2001). The soil microbial biomass: Concept, measurement and applications in soil ecosystem research. Microbes Environ. 16, 131–140. doi:10.1264/jsme2.2001.131
Brookes, P. C., Powlson, D. S., and Jenkinson, D. S. (1982). Measurement of microbial biomass phosphorus in soil. Soil Biol. Biochem. 14, 319–329. doi:10.1016/0038-0717(82)90001-3
Brown, C. (2013). Available nutrients and value for manure from various livestock types. Toronto: Ontario Ministry of Agriculture and Food and the Ministry of Rural Affairs.
Cambardella, C. A., and Elliott, E. T. (1992). Particulate soil organic-matter changes across a grassland cultivation sequence. Soil Sci. Soc. Am. J. 56, 777–783. doi:10.2136/sssaj1992.03615995005600030017x
Cao, D., Lan, Y., Liu, Z., Yang, X., Liu, S., He, T., et al. (2020). Responses of organic and inorganic phosphorus fractions in Brown Earth to successive maize stover and biochar application: A 5-year field experiment in northeast China. J. Soils Sediments 20, 2367–2376. doi:10.1007/s11368-019-02508-y
Chen, Y. P., Rekha, P. D., Arun, A. B., Shen, F. T., Lai, W. A., and Young, C. C. (2006). Phosphate solubilizing bacteria from subtropical soil and their tricalcium phosphate solubilizing abilities. Appl. Soil Ecol. 34, 33–41. doi:10.1016/j.apsoil.2005.12.002
Chen, W., Yang, F., Zhang, L., and Wang, J. (2016). Organic acid secretion and phosphate solubilizing efficiency of Pseudomonas sp. PSB12: Effects of phosphorus forms and carbon sources. Geomicrobiol. J. 33, 870–877. doi:10.1080/01490451.2015.1123329
Delgado, A., Madrid, A., Kassem, S., Andreu, L., and Del Campillo, M. D. C. (2002). Phosphorus fertilizer recovery from calcareous soils amended with humic and fulvic acids. Plant Soil 245, 277–286. doi:10.1023/a:1020445710584
El-Gizawy, A. M., Gomaa, H. M., El-Habbasha, K. M., and Mohamed, S. S. (1992). “Effect of different shading levels on tomato plants 1. Growth, flowering and chemical composition,”. Symposium on soil and soilless media under protected cultivation in mild winter climates, 323, 341–348.
Gichangi, E. M., Mnkeni, P. N. S., Brookes, P. C., et al. Gichangi, E. M., Mnkeni, P. N. S., and Brookes, P. C. (2011). Goat manure application improves phosphate fertilizer effectiveness through enhanced biological cycling of phosphorus. Soil Sci. Plant Nutr. 56, 853–860. doi:10.1111/j.1747-0765.2010.00515.x
Halvorson, H. O., Keynan, A., and Kornberg, H. L. (1990). Utilization of calcium phosphates for microbial growth at alkaline pH. Soil Biol. Biochem. 22, 887–890. doi:10.1016/0038-0717(90)90125-j
Hassan, H. M., Marschner, P., McNeill, A., and Tang, C. (2012). Growth, P uptake in grain legumes and changes in rhizosphere soil P pools. Biol. Fertil. Soils 48, 151–159. doi:10.1007/s00374-011-0612-y
Hoover, N. L., Yeow, J., Ann, L., Long, M., Kanwar, R. S., and Soupir, M. L. (2019). Long-term impact of poultry manure on crop yield , soil and water quality , and crop revenue. J. Environ. Manage. 252, 109582. doi:10.1016/j.jenvman.2019.109582
Huang, L. M., Jia, X. X., Zhang, G. L., and Shao, M. A. (2017). Soil organic phosphorus transformation during ecosystem development: A review. Plant Soil 417, 17–42. doi:10.1007/s11104-017-3240-y
Jiang, B., and Gu, Y. (1989). A suggested fractionation scheme of inorganic phosphorus in calcareous soils. Fertilizer Res. 20, 159–165. doi:10.1007/BF01054551
Kan, Z. R., Virk, A. L., He, C., Liu, Q. Y., Qi, J. Y., Dang, Y. P., et al. (2020). Characteristics of carbon mineralization and accumulation under long-term conservation tillage. Catena 193, 104636. doi:10.1016/j.catena.2020.104636
Khan, I., Fahad, S., Wu, L., Zhou, W., Xu, P., Sun, Z., et al. (2019). Labile organic matter intensifies phosphorous mobilization in paddy soils by microbial iron (III) reduction. Geoderma 352, 185–196. doi:10.1016/j.geoderma.2019.06.011
Khan, K. S., and Joergensen, R. G. (2009). Changes in microbial biomass and P fractions in biogenic household waste compost amended with inorganic P fertilizers. Bioresour. Technol. 100, 303–309. doi:10.1016/j.biortech.2008.06.002
Khan, K. S., and Joergensen, R. G. (2012). Compost and phosphorus amendments for stimulating microorganisms and growth of ryegrass in a Ferralsol and a Luvisol. J. Plant Nutr. Soil Sci. 175, 108–114. doi:10.1002/jpln.201100127
Kleber, M., Eusterhues, K., Keiluweit, M., Mikutta, C., Mikutta, R., and Nico, P. S. (2015). Mineral – organic associations : Formation , properties , and relevance in soil environments. Elsevier.
Kleinman, P. J. A., Wolf, A. M., Sharpley, A. N., Beegle, D. B., and Saporito, L. S. (2005). Survey of water-extractable phosphorus in livestock manures. Soil Sci. Soc. Am. J. 701, 701. doi:10.2136/sssaj2004.0099
Kouno, K., Wu, J., and Brookes, P. C. (2002). Turnover of biomass C and P in soil following incorporation of glucose or ryegrass. Soil Biol. Biochem. 34, 617–622. doi:10.1016/S0038-0717(01)00218-8
Lal, R. (2013). Soil carbon sequestration impacts on global. Science 304, 1623–1627. doi:10.1126/science.1097396
Li, J., and Marschner, P. (2019). Phosphorus pools and plant uptake in manure-amended soil. J. Soil Sci. Plant Nutr. 19, 175–186. doi:10.1007/s42729-019-00025-y
Li, Y., Wu, J., Liu, S., Shen, J., Huang, D., Su, Y., et al. (2012). Is the C:N:P stoichiometry in soil and soil microbial biomass related to the landscape and land use in southern subtropical China? Glob. Biogeochem. Cycles 26, 2012GB004399–14. doi:10.1029/2012GB004399
Liebisch, F., Keller, F., Huguenin-Elie, O., Frossard, E., Oberson, A., and Bünemann, E. K. (2014). Seasonal dynamics and turnover of microbial phosphorusin a permanent grassland. Biol. Fertil. Soils 50, 465–475. doi:10.1007/s00374-013-0868-5
Lindsay, W. L., Frazier, A. W., and Stephenson, H. F. (1962). Identification of reaction products from phosphate fertilizers in Soils1. Soil Sci. Soc. Am. J. 26, 446. doi:10.2136/sssaj1962.03615995002600050013x
Linu, M. S., Asok, A. K., Thampi, M., Sreekumar, J., and Jisha, M. S. (2019). Plant growth promoting traits of indigenous phosphate solubilizing Pseudomonas aeruginosa isolates from chilli (Capsicum annuum L.) rhizosphere. Commun. Soil Sci. Plant Anal. 50, 444–457. doi:10.1080/00103624.2019.1566469
Malik, M. A., Marschner, P., and Khan, K. S. (2012). Addition of organic and inorganic P sources to soil–Effects on P pools and microorganisms. Soil Biol. Biochem. 49, 106–113. doi:10.1016/j.soilbio.2012.02.013
Marriott, E. E., and Wander, M. (2006). Qualitative and quantitative differences in particulate organic matter fractions in organic and conventional farming systems. Soil Biol. Biochem. 38, 1527–1536. doi:10.1016/j.soilbio.2005.11.009
Marschner, P. (2008). The role of rhizosphere microorganisms in relation to P uptake by plants. Plant Ecophysiology,The Ecophysiol. Plant-Phosphorus Interact. (Dordrecht: Springer), 165–176. doi:10.1007/978-1-4020-8435-5_8
Meason, D. F., Idol, T. W., Friday, J. B., and Scowcroft, P. G. (2009). Effects of fertilisation on phosphorus pools in the volcanic soil of a managed tropical forest. For. Ecol. Manage. 258, 2199–2206. doi:10.1016/j.foreco.2009.04.001
Mehmood, A., Akhtar, M. S., Khan, K. S., Imran, M., and Rukh, S. (2015). Relationship of phosphorus uptake with its fractions in different soil parent materials. Int. J. Plant Soil Sci. 4, 45–53. doi:10.9734/IJPSS/2015/12684
Muhammad, S., Müller, T., and Joergensen, R. G. (2007). Compost and P amendments for stimulating microorganisms and maize growth in a saline soil from Pakistan in comparison with a nonsaline soil from Germany. J. Plant Nutr. Soil Sci. 170, 745–751. doi:10.1002/jpln.200625122
Murphy, J., and Riley, J. P. (1962). A modified single solution method for the determination of phosphate in natural waters. Anal. Chim. Acta X. 27, 31–36. doi:10.1016/S0003-2670(00)88444-5
Nyawade, S. O., Karanja, N. N., Gachene, C. K. K., Gitari, H. I., Schulte-Geldermann, E., and Parker, M. L. (2019). Short-term dynamics of soil organic matter fractions and microbial activity in smallholder potato-legume intercropping systems. Appl. Soil Ecol. 142, 123–135. doi:10.1016/j.apsoil.2019.04.015
Ojo, A. O., Adetunji, M. T., Okeleye, K. A., and Adejuyigbe, C. O. (2016). The effect of poultry manure and P fertilizer on some phosphorus fractions in some soils of southwestern Nigeria: An incubation study. Commun. Soil Sci. Plant Anal. 47, 2365–2377. doi:10.1080/00103624.2016.1216559
Olsen, S. R. (1954). Estimation of avaliable phosphorus in soils by extraction with sodium bicarbonate. Washington, DC: United states Dep Agric Washingt.
Peng, Y., Duan, Y., Huo, W., Xu, M., Yang, X., Wang, X., et al. (2021). Soil microbial biomass phosphorus can serve as an index to reflect soil phosphorus fertility. Biol. Fertil. Soils 57, 657–669. doi:10.1007/s00374-021-01559-z
Penhallegon, R. (2003). Nitrogen-phosphorus-potassium values of organic fertilizers, 4. Eugene: Oregon State Univ. Extension Service, LC437.
Peters, J., Combs, S., Hoskins, B., Jarman, J., Kovar, J., Watson, M., et al. (2003). Recommended methods of manure analysis. WI: Univ Wisconsin Coop Ext Publ Madison.
Rawat, P., Das, S., Shankhdhar, D., and Shankhdhar, S. C. (2020). Phosphate-Solubilizing microorganisms: Mechanism and their role in phosphate solubilization and uptake. J. Soil Sci. Plant Nutr. 211, 49–68. doi:10.1007/S42729-020-00342-7
Reddy, D. D., Rao, S. A., and Singh, M. (2005). Changes in P fractions and sorption in an Alfisol following crop residues application. Z. Pflanzenernahr. Bodenk. 168, 241–247. doi:10.1002/jpln.200421444
Richardson, A. E., Barea, J., Mcneill, A. M., and Prigent-combaret, C. (2009). Acquisition of phosphorus and nitrogen in the rhizosphere and plant growth promotion by microorganisms. Plant Soil 32, 305–339. doi:10.1007/s11104-009-9895-2
Schachtman, D. P., Reid, R. J., and Ayling, S. M. (1998). Phosphorus uptake by plants: From soil to cell. Plant Physiol. 116, 447–453. doi:10.1104/PP.116.2.447
Spohn, M., and Widdig, M. (2017). Turnover of carbon and phosphorus in the microbial biomass depending on phosphorus availability. Soil Biol. Biochem. 113, 53–59. doi:10.1016/j.soilbio.2017.05.017
Tang, X., Bernard, L., Brauman, A., Daufresne, T., Deleporte, P., Desclaux, D., et al. (2014). Increase in microbial biomass and phosphorus availability in the rhizosphere of intercropped cereal and legumes under field conditions. Soil Biol. Biochem. 75, 86–93. doi:10.1016/j.soilbio.2014.04.001
Virk, A. L., Liu, W-S., Niu, J-R., Xu, C-T., Liu, Q-Y., Kan, Z-R., et al. (2021). Effects of diversified cropping sequences and tillage practices on soil organic carbon, nitrogen, and associated fractions in the north China plain. J. Soil Sci. Plant Nutr. 1, 1201–1212. –12. doi:10.1007/s42729-021-00433-z
Wang, J., Liu, W. Z., Mu, H. F., and Dang, T. H. (2010b). Inorganic phosphorus fractions and phosphorus availability in a calcareous soil receiving 21- year superphosphate application. Pedosphere 20, 304–310.
Walkley, A., and Black, I. A. (1934). An examination of the degtjareff method for determining soil organic matter, and a proposed modification of the chromic acid titration method. Soil Sci. 37, 29–38. doi:10.1097/00010694-193401000-00003
Wu, J., Joergensen, R. G., Pommerening, B., Chaussod, R., and Brookes, P. C. (1990). Measurement of soil microbial biomass C by fumigation-extraction-an automated procedure. Soil Biol. Biochem. 22, 1167–1169. doi:10.1016/0038-0717(90)90046-3
Yong-Fu, L. I., An-Cheng, L. U. O., Xing-Hua, W. E. I., and Xu-Guo, Y. A. O. (2008). Changes in phosphorus fractions, pH, and phosphatase activity in rhizosphere of two rice genotypes. Pedosphere 18, 785–794. doi:10.1016/s1002-0160(08)60074-0
Zhang, L., Xu, M., Liu, Y., Zhang, F., Hodge, A., and Feng, G. (2016). Carbon and phosphorus exchange may enable cooperation between an arbuscular mycorrhizal fungus and a phosphate-solubilizing bacterium. New Phytol. 210, 1022–1032. doi:10.1111/nph.13838
Zhang, Y., Li, Y., Wang, S., Umbreen, S., and Zhou, C. (2021). Soil phosphorus fractionation and its association with soil phosphate-solubilizing bacteria in a chronosequence of vegetation restoration. Ecol. Eng. 164, 106208. doi:10.1016/J.ECOLENG.2021.106208
Keywords: carbon addition, microbial biomass, physical fractions, P bioavailability, sequential fractionation
Citation: Khan KS, Ali MM, Naveed M, Rehmani MIA, Shafique MW, Ali HM, Abdelsalam NR, Ghareeb RY and Feng G (2022) Co-application of organic amendments and inorganic P increase maize growth and soil carbon, phosphorus availability in calcareous soil. Front. Environ. Sci. 10:949371. doi: 10.3389/fenvs.2022.949371
Received: 20 May 2022; Accepted: 01 August 2022;
Published: 01 September 2022.
Edited by:
Haigang Li, Inner Mongolia Agricultural University, ChinaCopyright © 2022 Khan, Ali, Naveed, Rehmani, Shafique, Ali, Abdelsalam, Ghareeb and Feng. This is an open-access article distributed under the terms of the Creative Commons Attribution License (CC BY). The use, distribution or reproduction in other forums is permitted, provided the original author(s) and the copyright owner(s) are credited and that the original publication in this journal is cited, in accordance with accepted academic practice. No use, distribution or reproduction is permitted which does not comply with these terms.
*Correspondence: Muhammad Naveed, mnaveeduaf@googlemail.com