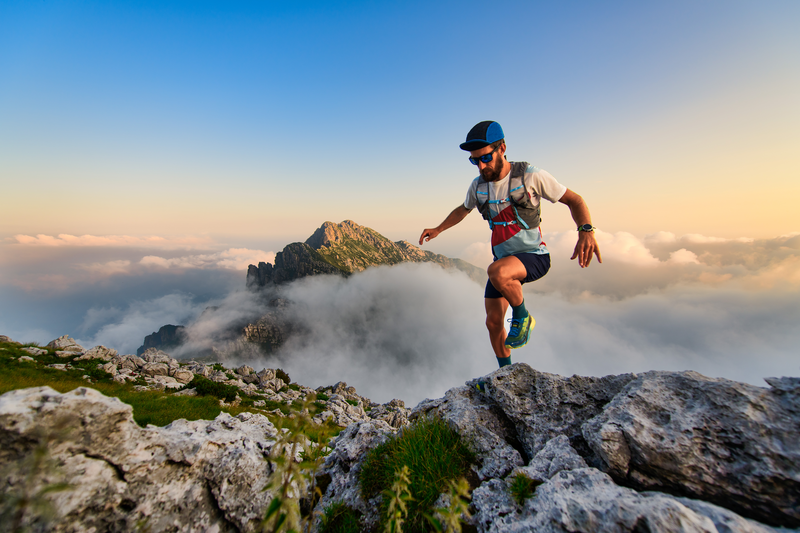
94% of researchers rate our articles as excellent or good
Learn more about the work of our research integrity team to safeguard the quality of each article we publish.
Find out more
SYSTEMATIC REVIEW article
Front. Environ. Sci. , 15 August 2022
Sec. Conservation and Restoration Ecology
Volume 10 - 2022 | https://doi.org/10.3389/fenvs.2022.947783
This article is part of the Research Topic Disturbance, Resilience and Restoration of Wetlands View all 20 articles
Steel slag substrate constructed wetlands (SSCWs) can effectively remove phosphorus (P) from sewage through Ca-P precipitation and adsorption. Nonetheless, the disadvantages of a high pH value of the effluent and low nitrogen (N) removal efficiency limit the practical application of SSCWs. To improve these shortcomings, plant cultivation and combining steel slag with other substrate materials have been applied in SSCWs. However, related studies have not obtained a unanimous consensus elucidating such improvements. To accurately evaluate improvements, we statistically analyzed the experimental data reported in 27 related papers and found that combining steel slag with other substrate materials in SSCWs significantly increased the removal amount of total nitrogen (TN) (51.58 mg TN/L) and ammonium nitrogen (NH4+-N) (74.15 mg NH4+-N/L) but reduced the removal amount of total phosphorus (TP) (7.76 mg TP/L). In these combined substrate SSCWs, plant cultivation could compensate for the decline in TP removal amount and improve upon the simultaneous removal of N and P (6.02 mg TP/L, 62.18 mg TN/L, and 69.16 mg NH4+-N/L). Moreover, compared with vertical flow SSCWs, horizontal flow enables plant-cultivated and combined substrate SSCWs to achieve a higher TP removal capacity (6.38 mg TP/L). In addition, operational parameters, including temperature, hydraulic retention time (HRT), pH value, and influent concentration, significantly affected the N and P removal capacity of SSCWs. Our research results provide a theoretical reference for the design and operation of SSCWs for efficient N and P removal.
As early as the 1980s, research on the application of steel slag to remove phosphorus (P) began (Yamada et al., 1986). The use of steel slag, a by-product of the iron and steel industry, as a substrate in constructed wetlands (CWs) to treat nitrogen (N) and P in sewage (Park et al., 2016) provides broad prospects for the recycling of steel slag waste. Steel slag substrate CWs (SSCWs) remove pollutants through physical, chemical, and biological processes mediated by substrate, plants, and microorganisms. Among these components, the steel slag substrate plays a particularly prominent role in P removal, and the main mechanism is Ca-P precipitation and adsorption (Pratt et al., 2007). On the one hand, CaO in steel slag is hydrolyzed to form Ca-P precipitates with PO43− in sewage (Baker et al., 1998; Kim et al., 2006). On the other hand, active sites such as metal oxide and hydroxyl oxide (Mo (OH)) sites adsorb P onto the surface of steel slag and gradually form hydroxyapatite (HAP) crystals (Drizo et al., 2006; Chazarenc et al., 2007). In column experiments of basic oxygen furnace steel slag, the PO43− removal efficiency was higher than 99%, and the removal capacity reached 3.1 mg P/g (Blanco et al., 2016). The TP removal rate from urban sewage by vertical flow SSCWs can reach 76% (Ge et al., 2016). Vertical and horizontal flow SSCWs can be connected in series, and the total phosphorus (TP) removal rate from construction sewage can reach 62% (Barca et al., 2013).
However, the strong alkalinity of steel slag is not conducive to plant growth and microbial survival, and it leads to a high pH of the effluent, which is attributed to the low efficiency of N removal by plants and microbes. Although ion exchange adsorption between NH4+ and metal ions (such as Al3+, Fe2+, and Mn4+) in steel slag can remove some ammonium nitrogen (NH4+-N) (Xu et al., 2019), when compared with microbial nitrogen removal, the nitrogen removed by adsorption is limited. This makes simultaneously removing N and P efficiently in SSCWs difficult. Combing steel slag with other materials, mixed or layered filling, might address these difficulties by relieving the strong alkalinity in SSCWs, whereas the reduced proportion of steel slag in the substrate could decrease P removal (Shen et al., 2020).
Due to the ability of plant roots to absorb N and P (Cui et al., 2015; Yun et al., 2015; Yuan et al., 2017; Saeed et al., 2020), as well as the active microbial metabolism of roots, plant cultivation in combined substrate SSCWs might be an effective way to compensate for the reduced P removal capacity of a combined substrate. The plants cultivated in these SSCWs are mainly common wetland plants, such as Vetiveria zizanioides, Canna indica, Acorus calamus, and Phragmites australis, which demonstrate high nutrient levels absorption rates and tolerance to saline and alkaline conditions. However, the role of plants in SSCWs has been controversial. Some studies showed that the death and decay of plants under excessively alkaline conditions can cause the release of P and reduce the removal amount of P (Lu et al., 2021; Zheng et al., 2021). In addition, the influence of operation parameters on the capacity of SSCWs to remove N and P also lacks a summative general mechanism. Based on these aspects, we systematically analyzed existing research results on SSCWs to clarify the following questions: 1) Can vegetated SSCWs improve the capacity to simultaneously remove N and P?, and 2) What is the general rule whereby operation parameters affect the capacity to remove N and P?
In this study, a comprehensive literature search was conducted to collect research data on SSCWs. The databases considered mainly included the Web of Science, Scopus, and Springer Link. The main keywords used included CWs, steel slag, nitrogen removal and phosphorus removal. This search contained almost all studies on SSCWs. These studies are described in Section 3, and the results are summarized and discussed. Next, not all articles could be employed for further analysis because they were review articles, and certain articles did not contain data on N and P removal amounts. By reading the title, abstract, and text of the articles during screening, 27 articles (Huang and He, 2011; Wu et al., 2011; Xiong et al., 2011; Barca et al., 2013; Shilton et al., 2013; Barca et al., 2014; Ren et al., 2014; Shi et al., 2014; Cui et al., 2015; Ge et al., 2015; Hussain et al., 2015; Yun et al., 2015; Zhang et al., 2015; Blanco et al., 2016; Ge et al., 2016; Lu et al., 2016; Mohamed et al., 2016; Ahmad et al., 2017; Shi P. et al., 2017; Park et al., 2017; Yuan et al., 2017; Adera et al., 2018; Xu et al., 2019; Chen et al., 2020; Hamdan et al., 2020; Saeed et al., 2020; Wan et al., 2020) met our requirement and were finally selected for data analysis, which provided a highly uniform dataset and reliable results. Datasets of the substrate filling mode, plant cultivation, flow direction of CW, steel slag particle size (SSPS), temperature (T), hydraulic retention time (HRT), hydraulic loading rate (HLR), pH value (pH), influent concentration (Cin), total nitrogen (TN), NH4+-N, and TP removal amounts (∆C = Cin − Cout) were extracted from these articles, and some original data were modified.
To reveal the influence of substrate filling mode, plant cultivation and flow direction on TP, TN, and NH4+-N removal, a single-factor analysis of variance was carried out of the TP, TN, and NH4+-N removal amounts achieved by CWs with steel slag substrate filling, layered steel slag and other substrate filling, mixed steel slag and other substrate filling, non-planted and planted CWs, and horizontal flow and vertical flow. Before analysis, the sample data must conform to a normal distribution, and the variance must be homogeneous. Otherwise, the data were converted until the above conditions were met, and variance analysis was subsequently performed. For the sample data that still did not meet the above conditions after conversion, a Kruskal–Wallis nonparametric analysis was performed. For example, during the grouping of the substrate filling modes, the NH4+-N removal data still did not conform to a normal distribution after conversion, so Kruskal–Wallis nonparametric analysis was performed. Then, samples with insufficient data were finally removed.
Next, the Pearson correlation coefficient is widely adopted to measure the degree of correlation between two variables. This value varies between –1 and 1. A negative value suggests a negative correlation, and a positive value indicates a positive correlation. To quantify the relationship between the TP, TN, and NH4+-N removal amounts and SSPS, T, HRT, HLR, pH, and Cin, a Pearson correlation coefficient matrix was established. In addition, to describe the interaction between the TP, TN, and NH4+-N removal amounts and the abovementioned operation parameters, redundancy analysis (RDA) was conducted.
To quantitatively describe the relationship between the TP, TN, and NH4+-N removal amounts and the operation parameters, a regression analysis method was applied. In a multiple linear regression model, the dependent variable is a linear function of the independent variables Xi, as follows:
where Y is the predicted value of the dependent variable, and X1, X2, ... Xn are the independent variables. The dependent variables included the TP, TN, and NH4+-N removal amounts ∆C = Cin − Cout (mg/L), and the independent variables were the SSPS (mm), T (°C), HRT (d), HLR (m/d), pH, and Cin (mg/L). All variables inserted in the model were considered significant at p < 0.05.
We employed SPSS 26 software (IBM, United States) for variance analysis, the Kruskal–Wallis test for nonparametric analysis, and set the condition for statistical significance at p < 0.05. An RDA was conducted by Canoco 5. Also, R 4.0.3 was used for illustrations.
In the initial application stage, SSCWs stuffed with single steel slag as substrate were used to remove P from sewage with different P concentrations. Following that, SSCWs with a combined and modified substrate were used to comprehensively improve the quality of septic tank sewage (Huang and He, 2011; Hussain et al., 2015), domestic sewage (Wu et al., 2011; Yun et al., 2015; Mohamed et al., 2016; Hamdan et al., 2020), urban sewage (Barca et al., 2013), pond sewage (Shilton et al., 2013), secondary effluent of sewage treatment plants (Xiong et al., 2011; Zhang et al., 2015; Chen et al., 2020), polluted river waters (Shi et al., 2014; Ge et al., 2015; Ge et al., 2016), hydroponic sewage (Park et al., 2017), agricultural sewage (Lu et al., 2016; Yuan et al., 2017), dairy farm sewage (Adera et al., 2018), leachate (Saeed et al., 2020), and other sewage. Besides, SSCWs demonstrate high P recovery potential. Table 1 summarizes the latest crucial scientific research on SSCWs, most of which were carried out on the scale of batch experiments (Baker et al., 1998; Kim et al., 2006; Blanco et al., 2016) in laboratories (Huang and He, 2011; Wu et al., 2011; Barca et al., 2014; Ren et al., 2014; Zhang et al., 2015; Ge et al., 2016; Ahmad et al., 2017). Some of the studies were conducted on a pilot scale (Hussain et al., 2015; Yun et al., 2015; Mohamed et al., 2016; Yuan et al., 2017; Chen et al., 2020; Hamdan et al., 2020), nothing in which full-scale field applications are not yet available.
TABLE 1. Summary of steel slag substrate constructed wetlands studies carried out at different areas with various experiment scale treatment of scale, sewage type, plant, substrate, and removal rates.
Steel slag substrates stuffed in SSCWs mainly include BOF steel slag (Baker et al., 1998; Hussain et al., 2015; Blanco et al., 2016; Ge et al., 2016), electric arc furnace (EAF) steel slag (Drizo et al., 2006; Barca et al., 2013; Barca et al., 2014; Adera et al., 2018), and modified steel slag (Ahmad et al., 2017; Park et al., 2017). Due to the addition of limestone in the steelmaking process, BOF steel slag contains high concentrations of CaO and exhibits high P removal capacity (Barca et al., 2013). Therefore, in some studies, when BOF slag is rapidly cooled, the content of unstable crystalline-free CaO and the alkalinity of SSCWs will be significantly reduced (Park et al., 2016). Regarding P removal capacity, BOF steel slag performs better than EAF steel slag. However, with a low pH value and many adsorption sites, the latter can remove P not only by precipitation but also by adsorption (Ge et al., 2016).
Subsurface SSCWs are most widely used according to the flow direction and are divided into unsaturated vertical flow (Huang and He, 2011; Wu et al., 2011; Xiong et al., 2011; Shilton et al., 2013; Ren et al., 2014; Shi et al., 2014; Cui et al., 2015; Hussain et al., 2015; Zhang et al., 2015; Blanco et al., 2016; Ge et al., 2016; Lu et al., 2016; Mohamed et al., 2016; Ahmad et al., 2017; Yuan et al., 2017; Adera et al., 2018; Hamdan et al., 2020; Saeed et al., 2020) and saturated horizontal flow (Barca et al., 2013; Barca et al., 2014; Ren et al., 2014; Cui et al., 2015; Ge et al., 2015; Park et al., 2017; Yuan et al., 2017; Adera et al., 2018; Xu et al., 2019; Chen et al., 2020) SSCWs. Next, vertical flow SSCWs require only a small amount of ground to operate but provide appropriate contact time and anaerobic time between water and substrate to ensure a better nitrogen and phosphorus removal performance (Yun et al., 2015). The intermittent water inflow and aeration condition (Shi X. et al., 2017) can promote the nitrification process and P transformation process. Like vertical flow SSCWs, horizontal flow SSCWs also demonstrate enough contact time to remove P in the sewage, but they require a larger amount of ground. Besides, CO2 interference is avoided for saturated operation, and little CaCO3 blockage is generated in the horizontal flow SSCWs. In addition, the alkalinity of effluent can be neutralized by spraying CO2 on the effluent. With low cost and minimal technical requirements, horizontal flow SSCWs exhibit enough contact time to remove P in the sewage. No air entering CO2 avoids interference, and almost no CaCO3 will be generated, thereby reducing the blockage (Ren et al., 2014). However, in the light of the alkalinity of the effluent, the pH value of the sewage can be neutralized by spraying CO2 in later stages (Park et al., 2017).
In addition, contentious issues exist regarding the pollutant removal efficiency of SSCWs. For example, one study proposed that EAF steel slag would release P when removing low P concentration sewage (Drizo et al., 2006) and believed that steel slag was not suitable for removing low P concentration sewage, which is in contrast to the results of other studies. Moreover, contradictions are found on the issue of whether the main P removal mechanism is adsorption or precipitation (Barca et al., 2014; Ge et al., 2016). In fact, the acid–base condition determines the main mechanism of P removal. Under alkaline conditions, precipitation, depending on the content of CaO in the substrate, is the main removal mechanism; thus, P can be quickly removed. In contrast, under acidic conditions, P is removed mainly by adsorption, and the removal process is slow and lasting (Ahmad et al., 2017; Xu et al., 2019). Moreover, HRT is a significant factor influencing removal efficiency. Too short HRT would result in incomplete removal of N and P, while a longer than the optimal time would lead to an unstable release of adsorbed P (Shilton et al., 2013), limited microbial growth, and increased pH value of effluent. In recent years, some studies focused on the issue of whether other pollutants in the sewage would influence P removal efficiency. On the one hand, researchers revealed that organic acids and organic colloids can block active surface sites of substrates and reduce P adsorption. On the other hand, some laboratory experiments showed that the inhibitory effect mentioned above can be mitigated over time, and eventually, P adsorption can be promoted, such as in iron oxide compounds, cations, and algae (Barca et al., 2013; Ge et al., 2016).
The substrate filling method imposed a significant influence on the removal of TP (p = 0.001), TN (p = 0.002), and NH4+-N (p = 0.009) (Figure 1). The degree of TP removal in SSCWs with different filling modes was ordered as follows: single steel slag substrate filling (14.95 mg TP/L) > layered combined substrate filling (7.19 mg TP/L) > mixed combined substrate filling (1.95 mg TP/L) (Figure 1A). The main reason why the TP removal amount of combined substrate filling is significantly lower than that of single steel slag filling is that in a limited-volume CW, the introduction of other substrates can reduce the steel slag amount, resulting in a decrease in the P removal amount during the adsorption and co-precipitation process. The main reason why the TP removal amount of mixed combined substrate filling is significantly lower than that of layered combined substrate filling is that mixed combined substrates can cause the steel slag surface to be partially covered, thus reducing the number of adsorption sites, or they can impede the release of calcium ions (Shi X. et al., 2017).
FIGURE 1. Influence of the substrate filling mode on pollutant removal by steel slag substrate constructed wetlands (SSCWs). (A) total phosphorus (TP) (B) total nitrogen (TN) (C) ammonium nitrogen (NH4+-N) (the pictures are all original data plots, and data conversion is performed during data analysis).
Next, the pH of SSCWs filled with single steel slag can reach as high as 12.4 (Gomes et al., 2018), which is not conducive to plant growth and microbial survival. These systems lack plant root adsorption and microbial metabolic activities and only achieve a small amount of physical adsorption. Compared to single steel slag, a combined substrate composed of steel slag and other substrate materials improved N removal due to the relatively suitable environment for microorganisms and plants (Shen et al., 2020). The TN removal of SSCWs with different combined substrate filling modes was as follows: layered combined substrate filling (14.75 mg TN/L) > mixed combined substrate filling (4.38 mg TN/L) (Figure 1B). The NH4+-N removal of SSCWs with different substrate filling modes was as follows: layered combined substrate filling (87.59 mg NH4+-N/L) > single steel slag substrate filling (40.46 mg NH4+-N/L) > mixed combined substrate filling (13.44 mg NH4+-N/L) (Figure 1C). These results indicate that the different substrate filling modes exert a profound impact on the removal amounts of N and P, and layered combined substrate SSCWs exhibit a better N removal capacity. Compared to layered combined substrate filling, mixed combined substrate filling causes other substrate materials to cover the adsorption sites of the steel slag substrate, thereby reducing the adsorption capacity of the steel slag substrate (Shi P. et al., 2017). Moreover, in the layered combined SSCWs, plants are usually cultivated in the non-steel slag materials layer with a milder acid–base condition (Cui et al., 2015; Yuan et al., 2017; Zheng et al., 2021), which better promotes the de-N effect of plants and microorganisms (Lan et al., 2018).
The strong alkalinity of single steel slag is not conducive to plant survival, so plants are usually cultivated in the combined substrate SSCWs. Plant cultivation in SSCWs significantly increased the removal amounts of TP by 6.02 mg TP/L (p = 0.026), TN by 62.18 mg TN/L (p = 0.000), and NH4+-N by 69.16 mg NH4+-N/L (p = 0.000) (Figure 2), indicating plant cultivation can improve the simultaneous N and P removal capacity of SSCWs. The adsorption of P by plant roots helps to remove P. However, the high pH can lead to plant death and the release of P through decaying plants (Lu et al., 2021; Zheng et al., 2021); thus, generating two opposing views on whether plant cultivation can improve the simultaneous removal of N and P. Our results suggest that the P adsorbed by plant roots offsets the P released by plant death. In this way, plant cultivation can enable the SSCWs to simultaneously remove N and P with high efficiency.
FIGURE 2. Influence of plant cultivation on pollutant removal in SSCWs. (A) TP (B) TN (C) NH4+-N (the pictures are all original data plots, and data conversion is performed during data analysis).
The average TP removal amount in horizontal flow SSCWs was 6.38 mg TP/L larger than that in vertical flow SSCWs (Figure 3). In SSCWs, Ca-P deposits cover the surface of steel slag, resulting in decreased P removal. The phenomenon is more serious in vertical SSCWs due to the frequent intermittent vertical flow transporting the deposits to the deeper substrates, then reducing the P adsorption. In contrast, the water flow remains horizontal and stable in horizontal flow SSCWs, enabling the sewage to be in full contact with steel slag, thus resulting in increased removal of P. In addition, strictly controlling the hydraulic residence time is necessary to reduce the pH while ensuring pollutant removal in horizontal flow SSCWs.
FIGURE 3. TP removal in horizontal and vertical flow SSCWs with plant cultivation (the pictures are all original data plots, and data conversion is performed during data analysis).
The TP removal amount of SSCWs was positively correlated with the HRT (r = 0.643; p = 0.000), pH (r = 0.430; p = 0.010), and Cin (r = 0.997; p = 0.000) (Figure 4A). With increasing HRT, the contact time between steel slag and sewage increased, consequently increasing the reaction time, thereby improving the TP removal amount. However, an excessively long HRT could increase the pH value of the effluent. In practical applications, the HRT should be reasonably controlled. Alkaline conditions are conducive to forming Ca-P, while acidic conditions are conducive to forming Fe-P and Al-P, each less stable than Ca-P. In addition, excessively high alkalinity could lead to the release of P (Shen et al., 2020). Considering the sewage discharge standards in most countries (pH 6–9), the pH of the effluent should be stabilized below 9. The positive correlation between TP removal and Cin suggests that in most experiments, steel slag can release enough Ca2+ to form precipitation with the high concentration of PO43– in sewage.
FIGURE 4. Pearson correlation analysis scatter plot and fitting line of the pollutant removal amount and experimental parameters. The error range of the experimental parameters with p < 0.05 is shown in figure. (A) TP (B) TN (C) NH4+-N.
The TN removal amount was positively correlated with T (r = 0.943; p = 0.005), HRT (r = 0.689; p = 0.004) and Cin (r = 0.887; p = 0.000) (Figure 4B). The NH4+-N removal amount was positively correlated with the HRT (r = 0.624; p = 0.004), pH (r = 0.573; p = 0.005) and Cin (r = 0.914; p = 0.000) (Figure 4C). With increasing temperature, the nitrification–denitrification rate was improved, and the TN removal amount increased.
Among these operation parameters, Cin was the most important factor influencing N and P removal amount (p = 0.002) (Figure 5), followed by HRT (p = 0.002), and then, T (p = 0.004) and pH (p = 0.008) decreased sequentially and positively correlated with N and P removal amount.
In addition, based on regression analysis, we quantitatively described the relationships between the TP, TN, NH4+-N removal amount and the operation parameters in SSCWs.
Eqs 2–4 described the above relationships in non-plant cultivation SSCWs.
where ∆C = Cin − Cout denotes the TP, TN, and NH4+-N removal amounts (mg/L); Cin and Cout are the influent and effluent concentrations (mg/L), respectively; SSPS is the steel slag particle size (mm); T is the temperature (°C); and pH denotes the acidity or alkalinity.
Eqs 5–7 described the above relationships in plant cultivation SSCWs:
where ∆C = Cin − Cout denotes the TP, TN, and NH4+-N removal amounts (mg/L); Cin and Cout are the influent and effluent concentrations (mg/L), respectively; T is the temperature (°C); and pH denotes the acidity or alkalinity.
Eqs 2 and 4, 5, and 7 indicate that plant cultivation weakens the dependence of SSCWs on SSPS for P and NH₄⁺-N removal. Compared to non-plant cultivation SSCWS, temperature is one of the determinants of P removal in plant cultivation SSCWs.
Our results indicate that layered combined substrates can significantly increase the TN and NH4+-N removal amounts in SSCWs but reduce the TP removal amount. Plant cultivation in combined substrate SSCWs can compensate for the decline in TP removal. Compared with vertical flow, horizontal flow SSCWs demonstrate a higher removal capacity of P. Next, T, HRT, pH, and Cin significantly affected the N and P removal capacity of SSCWs. To conclude, plant cultivation and combined substrates can improve the simultaneous removal of N and P in SSCWs. This study can serve as a reference to solve certain problems encountered in SSCWs.
The original contributions presented in the study are included in the article/Supplementary material, and further inquiries can be directed to the corresponding author.
JZ: Data curation and analysis, writing—original draft. YZ: Aided in interpreting the results and worked on the manuscript. XY: Worked on the technical details, supervised the findings of the work and helped in the development of manuscript. SD: Writing—review and editing. JY: Data curation. YM: Data curation.
This work was supported by the National Natural Science Foundation of China (41871100), the National Natural Science Foundation of China (41971136), and the National Natural Science Foundation of China (42171107).
The authors declare that the research was conducted in the absence of any commercial or financial relationships that could be construed as a potential conflict of interest.
All claims expressed in this article are solely those of the authors and do not necessarily represent those of their affiliated organizations, or those of the publisher, the editors, and the reviewers. Any product that may be evaluated in this article, or claim that may be made by its manufacturer, is not guaranteed or endorsed by the publisher.
Adera, S., Drizo, A., Twohig, E., Jagannathan, K., and Benoit, G. (2018). Improving Performance of Treatment Wetlands: Evaluation of Supplemental Aeration, Varying Flow Direction, and Phosphorus Removing Filters. Water Air Soil Pollut. 229, 100. doi:10.1007/s11270-018-3723-3
Ahmad, S. Z. N., Hamdan, R., Wan Mohamed, W. A., Othman, N., Mohd Zin, N. S., and Musa, S. (2017). Comparisons Study of Phosphate Removal in Unaerated and Aerated High Calcium Steel Slag Filter System of Different pH Feed. MATEC Web Conf. 103, 06018. doi:10.1051/matecconf/201710306018
Baker, M. J., Blowes, D. W., and Ptacek, C. J. (1998). Laboratory Development of Permeable Reactive Mixtures for the Removal of Phosphorus from Onsite Wastewater Disposal Systems. Environ. Sci. Technol. 32 (15), 2308–2316. doi:10.1021/es970934w
Barca, C., Meyer, D., Liira, M., Drissen, P., Comeau, Y., Andrès, Y., et al. (2014). Steel Slag Filters to Upgrade Phosphorus Removal in Small Wastewater Treatment Plants: Removal Mechanisms and Performance. Ecol. Eng. 68, 214–222. doi:10.1016/j.ecoleng.2014.03.065
Barca, C., Troesch, S., Meyer, D., Drissen, P., Andrès, Y., and Chazarenc, F. (2013). Steel Slag Filters to Upgrade Phosphorus Removal in Constructed Wetlands: Two Years of Field Experiments. Environ. Sci. Technol. 47 (1), 549–556. doi:10.1021/es303778t
Blanco, I., Molle, P., Sáenz de Miera, L. E., and Ansola, G. (2016). Basic Oxygen Furnace Steel Slag Aggregates for Phosphorus Treatment. Evaluation of its Potential Use as a Substrate in Constructed Wetlands. Water Res. 89, 355–365. doi:10.1016/j.watres.2015.11.064
Chazarenc, F., Brisson, J., and Comeau, Y. (2007). Slag Columns for Upgrading Phosphorus Removal from Constructed Wetland Effluents. Water Sci. Technol. 56 (3), 109–115. doi:10.2166/wst.2007.499
Chen, X., Sun, X., Xu, P., Wang, S., Zhou, T., Wang, X., et al. (2020). Optimal Regulation of N/P in Horizontal Sub-surface Flow Constructed Wetland through Quantitative Phosphorus Removal by Steel Slag Fed. Environ. Sci. Pollut. Res. 27 (6), 5779–5787. doi:10.1007/s11356-019-06696-5
Cui, L., Ouyang, Y., Yang, W., Huang, Z., Xu, Q., and Yu, G. (2015). Removal of Nutrients from Septic Tank Effluent with Baffle Subsurface-Flow Constructed Wetlands. J. Environ. Manag. 153, 33–39. doi:10.1016/j.jenvman.2015.01.035
Drizo, A., Forget, C., Chapuis, R. P., and Comeau, Y. (2006). Phosphorus Removal by Electric Arc Furnace Steel Slag and Serpentinite. Water Res. 40 (8), 1547–1554. doi:10.1016/j.watres.2006.02.001
Ge, Y., Wang, X. C., Dzakpasu, M., Zheng, Y., Zhao, Y., and Xiong, J. (2016). Characterizing Phosphorus Removal from Polluted Urban River Water by Steel Slags in a Vertical Flow Constructed Wetland. Water Sci. Technol. 73 (11), 2644–2653. doi:10.2166/wst.2016.118
Ge, Y., Wang, X., Zheng, Y., Dzakpasu, M., Zhao, Y., and Xiong, J. (2015). Functions of Slags and Gravels as Substrates in Large-Scale Demonstration Constructed Wetland Systems for Polluted River Water Treatment. Environ. Sci. Pollut. Res. 22, 12982–12991. doi:10.1007/s11356-015-4573-9
Gomes, H. I., Mayes, W. M., Baxter, H. A., Jarvis, A. P., Burke, I. T., Stewart, D. I., et al. (2018). Options for Managing Alkaline Steel Slag Leachate: A Life Cycle Assessment. J. Clean. Prod. 202, 401–412. doi:10.1016/j.jclepro.2018.08.163
Hamdan, R., Ibrahim, I. I., Wan Mohamed, W. A., Al‐Gheethi, A., Othman, N., and Mohamed, R. (2020). Optimizing Vertical Flow Aerated Steel Slag Filter System with Nitrifiers Bacteria for Nutrients' Removal from Domestic Wastewater: a Pilot Study. J. Chem. Technol. Biotechnol. 96 (4), 1067–1079. doi:10.1002/jctb.6618
Huang, L., and He, X. (2011). Construction of Steel Slag-Limestone Wetland with Mixed Progressively-Sized and Anti-sized Bed for Living Sewage Treatment. Environ. Pollut. Control 33 (9), 25–28. doi:10.15985/j.cnki.1001-3865.2011.09.011
Hussain, S. I., Blowes, D. W., Ptacek, C. J., Jamieson-Hanes, J. H., Wootton, B., Balch, G., et al. (2015). Mechanisms of Phosphorus Removal in a Pilot-Scale Constructed Wetland/BOF Slag Wastewater Treatment System. Environ. Eng. Sci. 32 (4), 340–352. doi:10.1089/ees.2014.0376
Kim, E.-H., Lee, D.-W., Hwang, H.-K., and Yim, S. (2006). Recovery of Phosphates from Wastewater Using Converter Slag: Kinetics Analysis of a Completely Mixed Phosphorus Crystallization Process. Chemosphere 63 (2), 192–201. doi:10.1016/j.chemosphere.2005.08.029
Lan, W., Zhang, J., Hu, Z., Ji, M., Zhang, X., Zhang, J., et al. (2018). Phosphorus Removal Enhancement of Magnesium Modified Constructed Wetland Microcosm and its Mechanism Study. Chem. Eng. J. 335, 209–214. doi:10.1016/j.cej.2017.10.150
Lu, H., Xiao, L., Wang, T., Lu, S., Wang, H., Guo, X., et al. (2021). The Application of Steel Slag in a Multistage Pond Constructed Wetland to Purify Low-Phosphorus Polluted River Water. J. Environ. Manag. 292, 112578. doi:10.1016/j.jenvman.2021.112578
Lu, S., Zhang, X., Wang, J., and Pei, L. (2016). Impacts of Different Media on Constructed Wetlands for Rural Household Sewage Treatment. J. Clean. Prod. 127, 325–330. doi:10.1016/j.jclepro.2016.03.166
Mohamed, R. M. S. R., Al-Gheethi, A. A., Miau, J. A., and Kassim, A. H. M. (2016). Multi-component Filters for Domestic Graywater Treatment in Village Houses. jawwa 108 (7), E405–E415. doi:10.5942/jawwa.2016.108.0103
Park, J.-H., Kim, S.-H., Delaune, R. D., Kang, B.-H., Kang, S.-W., Cho, J.-S., et al. (2016). Enhancement of Phosphorus Removal with Near-Neutral pH Utilizing Steel and Ferronickel Slags for Application of Constructed Wetlands. Ecol. Eng. 95, 612–621. doi:10.1016/j.ecoleng.2016.06.052
Park, J.-H., Wang, J. J., Kim, S.-H., Cho, J.-S., Kang, S.-W., Delaune, R. D., et al. (2017). Phosphate Removal in Constructed Wetland with Rapid Cooled Basic Oxygen Furnace Slag. Chem. Eng. J. 327, 713–724. doi:10.1016/j.cej.2017.06.155
Pratt, C., Shilton, A., Pratt, S., Haverkamp, R. G., and Bolan, N. S. (2007). Phosphorus Removal Mechanisms in Active Slag Filters Treating Waste Stabilization Pond Effluent. Environ. Sci. Technol. 41 (9), 3296–3301. doi:10.1021/es062496b
Ren, L.-J., Xu, L.-L., Zhang, Y.-Y., Pan, W., Yin, S.-L., Zhou, Y., et al. (2014). Effects of Connection Mode and Hydraulic Retention Time on Wastewater Pollutants Removal in Constructed Wetland Microcosms. Clean. Soil Air Water 43 (12), 1574–1581. doi:10.1002/clen.201300842
Saeed, T., Miah, M. J., Majed, N., Hasan, M., and Khan, T. (2020). Pollutant Removal from Landfill Leachate Employing Two-Stage Constructed Wetland Mesocosms: Co-treatment with Municipal Sewage. Environ. Sci. Pollut. Res. 27, 28316–28332. doi:10.1007/s11356-020-09208-y
Shen, S., Li, X., Cheng, F., Zha, X., and Lu, X. (2020). Review: Recent Developments of Substrates for Nitrogen and Phosphorus Removal in CWs Treating Municipal Wastewater. Environ. Sci. Pollut. Res. 27, 29837–29855. doi:10.1007/s11356-020-08808-y
Shi, P., Jiang, Y., Zhu, H., and Sun, D. (2017a). Impact of Steel Slag on the Ammonium Adsorption by Zeolite and a New Configuration of Zeolite-Steel Slag Substrate for Constructed Wetlands. Water Sci. Technol. 76 (3), 584–593. doi:10.2166/wst.2017.232
Shi, P., Zhu, H., and Sun, D. (2014). Removal Efficiency of Typical Pollutants by Different Substrate Combinations for Constructed Wetlands. Huanjing Kexue Xuebao/Acta Sci. Circumstantiae 34 (3), 704–711. doi:10.13671/j.hjkxxb.2014.0122.html
Shi, X., Fan, J., Zhang, J., and Shen, Y. (2017b). Enhanced Phosphorus Removal in Intermittently Aerated Constructed Wetlands Filled with Various Construction Wastes. Environ. Sci. Pollut. Res. 24, 22524–22534. doi:10.1007/s11356-017-9870-z
Shilton, A., Chen, L., Elemetri, I., Pratt, C., and Pratt, S. (2013). Active Slag Filters: Rapid Assessment of Phosphorus Removal Efficiency from Effluent as a Function of Retention Time. Environ. Technol. 34 (2), 195–200. doi:10.1080/09593330.2012.689365
Wan, Z., Zhang, Y., Lu, S., Zhang, X., Liu, X., and Yang, Y. (2020). Effect of Packing Substrates on the Purification of Municipal Wastewater Treatment Plant Effluent. Environ. Sci. Pollut. Res. 27, 15259–15266. doi:10.1007/s11356-020-08068-w
Wu, J., He, F., Xu, D., Wang, R., Zhang, X., Xiao, E., et al. (2011). Phosphorus Removal by Laboratory-Scale Unvegetated Vertical-Flow Constructed Wetland Systems Using Anthracite, Steel Slag and Related Blends as Substrate. Water Sci. Technol. 63 (11), 2719–2724. doi:10.2166/wst.2011.573
Xiong, J., Qin, Y., Mahmood, Q., Liu, H., and Yang, D. (2011). Phosphorus Removal from Secondary Effluents through Integrated Constructed Treatment System. Mar. Pollut. Bull. 63 (5-12), 98–101. doi:10.1016/j.marpolbul.2011.04.020
Xu, R., Zhang, Y., Liu, R., Cao, Y., Wang, G., Ji, L., et al. (2019). Effects of Different Substrates on Nitrogen and Phosphorus Removal in Horizontal Subsurface Flow Constructed Wetlands. Environ. Sci. Pollut. Res. 26, 16229–16238. doi:10.1007/s11356-019-04945-1
Yamada, H., Kayama, M., Saito, K., and Hara, M. (1986). A Fundamental Research on Phosphate Removal by Using Slag. Water Res. 20 (5), 547–557. doi:10.1016/0043-1354(86)90018-7
Yuan, Z., Fu, S., Xu, X., and Wendling, L. A. (2017). Mineral Processing Residue Use as Substrate in a Modular Engineered Wetland for Wastewater Treatment. Environ. Process. 4, 523–547. doi:10.1007/s40710-017-0247-6
Yun, Y., Zhou, X., Li, Z., Uddin, S. M. N., and Bai, X. (2015). Comparative Research on Phosphorus Removal by Pilot-Scale Vertical Flow Constructed Wetlands Using Steel Slag and Modified Steel Slag as Substrates. Water Sci. Technol. 71 (7), 996–1003. doi:10.2166/wst.2015.059
Zhang, C., Tan, S., Li, J., and Peng, C. (2015). Polishing of Secondary Effluent by a Two-Stage Vertical-Flow Constructed Wetland. Pol. J. Environ. Stud. 24 (2), 923–928. doi:10.15244/pjoes/23868
Zheng, Y., Cao, T., Zhang, Y., Xiong, J., Dzakpasu, M., Yang, D., et al. (2021). Characterization of Dissolved Organic Matter and Carbon Release from Wetland Plants for Enhanced Nitrogen Removal in Constructed Wetlands for Low C-N Wastewater Treatment. Chemosphere 273, 129630. doi:10.1016/j.chemosphere.2021.129630
Keywords: steel slag, planting, constructed wetlands, nitrogen removal, phosphorus removal
Citation: Zhang J, Zou Y, Yu X, Ding S, Yan J and Min Y (2022) Vegetated Steel Slag Substrate Constructed Wetlands can Achieve High Efficiency Simultaneous Nitrogen and Phosphorus Removal. Front. Environ. Sci. 10:947783. doi: 10.3389/fenvs.2022.947783
Received: 19 May 2022; Accepted: 16 June 2022;
Published: 15 August 2022.
Edited by:
He Yixin, Chengdu Institute of Biology (CAS), ChinaCopyright © 2022 Zhang, Zou, Yu, Ding, Yan and Min. This is an open-access article distributed under the terms of the Creative Commons Attribution License (CC BY). The use, distribution or reproduction in other forums is permitted, provided the original author(s) and the copyright owner(s) are credited and that the original publication in this journal is cited, in accordance with accepted academic practice. No use, distribution or reproduction is permitted which does not comply with these terms.
*Correspondence: Xiaofei Yu, eXV4Zjg4OEBuZW51LmVkdS5jbg==
Disclaimer: All claims expressed in this article are solely those of the authors and do not necessarily represent those of their affiliated organizations, or those of the publisher, the editors and the reviewers. Any product that may be evaluated in this article or claim that may be made by its manufacturer is not guaranteed or endorsed by the publisher.
Research integrity at Frontiers
Learn more about the work of our research integrity team to safeguard the quality of each article we publish.