- College of Agronomy, Inner Mongolia Agricultural University, Hohhot, China
Legume–brassica intercropping is widely used to increase productivity in modern, sustainable agricultural systems. However, few studies have assessed the linkages between soil properties and soil microorganisms. Soil microorganisms play a key role in soil nutrient turnover and plant community composition. To elucidate the responses of soil microbial community diversity and structure to intercropping, we conducted a 2-year experiment based on common vetch (CV) monoculture, rape (R) monoculture, and common vetch–rape intercropping (IRCV) with phosphorus (P) addition in alkaline soil. The microbial communities of bacteria and fungi in the rhizosphere soil were examined based on high-throughput sequencing targeting the 16S rRNA and ITS genes, respectively. In addition, we analyzed changes in soil properties and enzyme activities. Intercropping significantly increased dry matter (up to 98.86% and 81.48%, respectively dry matter is the aboveground biomass.) compared with common vetch monoculture. Intercropping decreased soil bulk density and pH and enhanced soil available phosphorus (AP) by 14.54–34.38%, 7.25–22.67%, soil organic matter (SOM) by 15.57–22.85, 6.82–15.57%, soil sucrase (Suc.) by 13.69–16.10%, 35.57–40.24% compared to monoculture common vetch and rape, respectively. However, bacterial alpha diversity was higher under rape monoculture than IRCV. In addition, the dominant soil bacterial phyla Proteobacteria (1.25–3.60%), Gemmatimonadetes (7.88–15.16%), Bacteroidetes (9.39–11.76%), and Rokubacteria (0.49–5.69%) were present at greater abundance with IRCV relative to those with CV and R, but phyla Chloroflexi was significantly decreased by 11.56–12.94% with IRCV compared with the other two treatments. The redundant analysis showed that SOM and AP were positively correlated with the dominant bacterial and fungal flora. Common vetch–rape intercropping resulted in increased biomass and altered soil microbial community composition as well as soil properties. Our results showed that intercropping systems positively improve soil microbial activity; this strategy could help in the cultivation of multiple crops and improve soil properties through sustainable production.
Introduction
In terms of area, China is the third-largest country in the world; with a population of 1.41 billion, it accounted for 18% of the global population in 2020 (Wang et al., 2021). This poses a challenging task in providing food security, including the demand for dairy products, beef, and mutton, which is gradually increasing due to the increasing population. High-quality pastureland plays an indispensable role in producing high-quality and safe dairy products, beef, and mutton. Since 2008, China’s imports of both alfalfa hay and oat grass have shown an exponentially increasing trend (Wang et al., 2015). Inner Mongolia’s farming–pastoral ecotone comprises most of the farming–pastoral ecotone in north China, which is suitable for pasture cultivation (Wei et al., 2020). Common vetch (Vicia sativa L.) and rape (Brassica napus L.) are high-quality forage crops known for their drought and cold resistance (Yuan et al., 2021; Ping et al., 2022). The growing of common vetch and rape in Inner Mongolia is of great importance for the development of animal husbandry.
Intercropping is the practice of growing two or more crops on the same land at the same time, which is a typical pattern of aboveground crop diversity (Gong et al., 2019). Intercropping has been widely used all over the world because of its advantages in increasing yield, preventing diseases, and utilizing resources (He et al., 2010; Yin et al., 2017). Following intercropping, the quantity and composition of organic matter in the input soil increases, and the physical and chemical properties of the soil also change (Nyawade et al., 2020). One study showed that, compared with monoculture, the soil water content following millet and mung bean intercropping increased by 12.1% (Wang et al., 2021). After continuous intercropping of wheat and corn for many years, the soil organic carbon (SOC) content increased by an average of 3% compared with the SOC following monoculture (Cong et al., 2014). Additionally, intercropping has a positive effect on the number of soil microorganisms and enzyme activity. Compared with monoculture, intercropping of maize and peanuts improved the functional diversity of soil microbial communities and soil enzyme activity (Zhang et al., 2012). Changes following intercropping can directly or indirectly affect the microbial activity and functional diversity of microorganisms (Zhao et al., 2017; Nyawade et al., 2019). A comparison of microbial diversity could be useful in understanding how rhizosphere communities mediate the impacts of various agricultural practices on crop yields (Zhang et al., 2010). However, few studies have investigated common vetch and rape intercropping, and the mechanism underlying the changes observed following their intercropping remains unclear.
Microbial characteristics are one of the most critical indicators of soil health because soil microorganisms play a critical role in soil nutrient transformation and cycling (Franchini et al., 2007; Yang et al., 2021). Soil management and planting systems can increase soil microbial biomass and the activity and quantity of beneficial microorganisms (Balota et al., 2003). The change in soil available phosphorous (AP) concentration is a key parameter that previous studies have shown can shift the diversity and composition of the soil microbial community (He et al., 2016; Ling et al., 2017). Soil enzymes are a type of bioactive substance and mainly come from the decomposition of plant roots, soil microorganisms, and animal and plant residues (Meng and Wu, 2004). They participate in soil biochemical reactions, promote the process of soil physiological and biochemical reactions, and are an indispensable part of soil ecology (Xue et al., 2003); therefore, the study of soil microorganisms and enzymes is of great importance for the evaluation of soil quality and fertility. Some chemicals secreted by microorganisms living in soil can have an important effect on the formation and activity of enzymes. Therefore, it is worth investigating how the changes in soil physical and chemical properties caused by intercropping affect the metabolic activity and functional diversity of underground soil microorganisms.
To explore the responses in soil properties and soil microbial community diversity and structure to common vetch–rape intercropping compared with monocropping, we conducted a 2-year experiment, with additional application of P. Our objectives were to 1) investigate the responses in the soil microbial community composition to the intercropping system and 2) identify the main properties of soil that drive changes in soil microbial community composition in three crop intercropping systems.
Materials and methods
Experimental site
The field experiment was conducted at an agricultural experimental station (40°56′ N, 110°48′ E), Inner Mongolia Autonomous Region, North China, in 2019 and 2020. The altitude is 1,052 m, and the climate is a typical semi-arid and temperate continental monsoon climate, with mean annual precipitation and temperature 400 mm and 7.2°C, respectively, and with average annual evaporation of 1851.7 mm and accumulation temperature of 2,800°C. The initial soil chemical properties (depth 0–20 cm) in 2019 were as follows: pH 8.45 (soil: water = 1:2.5), soil organic matter content (SOM) 16.56 g kg−1, total nitrogen (TN) 0.53 g kg−1, total phosphorus (TP) 0.54 g kg−1, total potassium (TK) 16.64 g kg−1, available nitrogen (AN) 90.4 mg kg−1, available phosphorus (AP) 15.51 mg kg−1, and available potassium (AK) 140.20 mg kg−1.
Experimental design
The random block design was adopted in this experiment, and the plots were 5 × 6 m in size. As shown in Figure 1, three treatments were set up, with five replicates of each: 1) monoculture of common vetch (CV), 2) monoculture of rape (R), and 3) intercropping of rape/common vetch (IRCV). In the intercropping system, four rows of common vetch and two rows of rape were planted. The inter-row distance between rows of rape and adjacent rows of common vetch was 25 cm, the same as on the monoculture plots (Figure 1). In all treatments, rape and common vetch were sown at a seeding rate of 15 kg hm−2 and 75 kg hm−2, respectively. Basal nutrients supplied were 90 kg P hm−2 (as calcium superphosphate) and 120 kg N hm−2 (as urea), with no further fertilizers applied during the following growth period. All fertilizers were uniformly broadcast and incorporated into the upper 30 cm of the soil prior to sowing. Two applications of irrigation of 180 mm (1800 m3 hm−2) each were made on 1 to 10 June and 1 to 5 July in both years. No herbicides were used during the growth period, but insect control followed standard farming practices (Li et al., 2018) and was carried out as needed. Common vetch, as well as rape, was sown on 30 April 2019 and 4 May 2020 and harvested on 25 July 2019 and 3 August 2020.
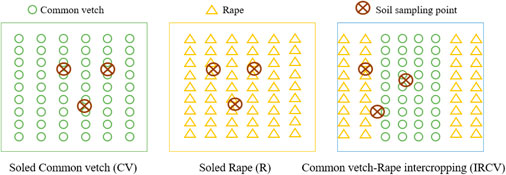
FIGURE 1. Schematic diagram of the planting pattern and soil sampling. CV, R, and IRCV represent common vetch monoculture, rape monoculture, and two rows of rape alternated with four rows of common vetch, respectively.
Soil sampling
After harvesting the common vetch and rape in July 2019 and August 2020, soil augers were use to collect soil samples (0–30 cm deep) from three points on each plot (Figure 1). The soil from each plot was mixed thoroughly and sieved (2 mm). Each soil sample was divided into three portions. One part was stored in a –80°C freezer and subsequently used for the analysis of soil microbial community structure and diversity detection; the second portion was stored in a 4°C refrigerator prior to being used detect the soil microbial biomass; and the third portion of each soil sample was dried at room temperature and ground to a powder for the detection of soil nutrients, pH, and soil enzymes. The ring knife method was used to determine soil bulk density (SBD), with the ring soil samples scraped out and any roots manually removed. The soils were dried at 105°C to constant weight.
Analysis of soil nutrients, pH, and SBD
Soil organic matter (SOM) content was determined by the potassium dichromate volumetric method (Finzi et al., 2015). TN content was measured using the method described by Finzi et al. (2015). TP content and AP were determined using the NaOH molybdenum stibium anti-color method (Ren et al., 2016), and soil pH was determined by the potentiometric method, where the soil to water ratio was 1:2.5 (Bao, 2005). Soil bulk density (SBD) was calculated by the gravimetric weight (Vos et al., 2005).
Analysis of soil microbial biomass and soil enzymatic activity
Soil microbial biomass carbon (MBC), microbial biomass nitrogen (MBN), and microbial biomass phosphorus (MBP) were measured using the chloroform fumigation-extraction technique, modified from the methods of Brookes et al. (1982), Brookes et al. (1984), and Brookes et al. (1985). Soil urease activity was determined by the indophenol blue colorimetric method (Wang et al., 2009), soil sucrase activity was determined by the 3,5-dinitrosalicylic acid method (Mi et al., 2018), and soil acid phosphatase activity was determined by the disodium phenyl phosphate method (Guan et al., 1986).
Microbial DNA extraction, PCR amplification, and Illumina NovaSeq sequencing
A Power Soil ® DNA Isolation Kit (MO BIO Laboratories) was used to extract soil DNA. The 16S rRNA gene of bacteria (in the V3–V4 region) was amplified using the primers 338 F (5′- ACTCCTACGGGAGGCAGCA-3′) and 806 R (5′- GGACTACHVGGGTWTCTAAT-3′) (Mori et al., 2014). We amplified the rRNA gene of fungi (ITS1 region) using ITS1-F (5′-CTTGGTCATTTAGAGGAAGTAA-3′) and ITS2-R (5′-GCTGCGTTCTTCATC GATGC-3′) primers (White et al., 1990; Gardes and Bruns, 1993).
The paired-end reads of the fungal ITS genes and bacterial 16S rRNA genes were processed, and the ITS regions and 16S sequences were screened for quality control according to the methods of Guo et al., 2019. We clustered all tags with >97% identity into operational taxonomic units (OTUs). The tags were then classified into different taxonomies according to the Silva and UNITE databases for soil bacterial and fungal communities, respectively. There were 44,529 OTUs for soil bacteria and 3,237 OTUs for soil fungi after removing those OTUs that did not belong to either the soil bacterial or fungal communities.
Statistical analysis
Microsoft Excel 2019 software and SPSS 23.0 software were used for the statistical analysis of data. Duncan’s multiple range test was used for the analysis of variance (p < 0.05). The relationships among soil properties and forage aboveground biomass were examined by performing Pearson’s correlation analysis. The Canoco 5 (Microcomputer Power, Ithaca, NY, United States) was used for principal coordinates analysis (PCoA) and redundancy discriminatory analysis (RDA). Origin software (Version 8.5; Northampton, MA, United States) was used to draw figures.
Results
Aboveground biomass
The yield from rape/common vetch intercropping was 31.44 t hm−2 in 2019 and 24.99 t hm−2 in 2020. In 2019, the yield of common vetch monoculture was 15.81 t hm−2, and the yield of rape monoculture was 31.08 t hm−2. In 2020, the yield of common vetch monoculture was 13.77 t hm−2, while the yield of rape monoculture was 27.60 t hm−2. Intercropping significantly increased dry matter (refers to aboveground biomass, up to 98.86% and 81.48%, respectively ) compared with that obtained by common vetch monoculture.
Soil properties
As shown in Table 1, the intercropping patterns affected soil nutrients and pH. Specifically, regarding the soil physical properties, pH was lower with intercropping compared with the cropping systems of common vetch or rape monoculture, which were decreased by 0.42 and 0.43, respectively. Compared with the common vetch or rape monoculture cropping systems, SBD under intercropping significantly decreased by 1.85% and 2.75% in 2019 and 4.92% and 1.69%, in 2020, respectively. For soil chemical properties, however, intercropping significantly increased SOM, TN, and AP levels (p < 0.05), ranging from 19.26 to 23.66 g kg−1 for OM, 0.52 to 0.62 g kg−1 for TN, 0.88 to 0.94 for g kg−1 TP, and 22.19 to 29.82 mg kg−1 for AP (in 2020). Similarly, compared with monoculture, the SOM, TN, and AP content was higher under intercropping treatment (in 2019, p > 0.05).
Both monoculture and intercropping systems had significant effects on the soil MBC, MBN, and MBP (Figure 2). Compared with common vetch monoculture, the content of MBC, MBN, and MBP in the rhizosphere soil of the intercropping system was significantly higher (p < 0.05), by 29.13%, 39.44%, and 35.46% in 2019 and by 33.70%, 31.92%, and 32.70% in 2020, respectively.
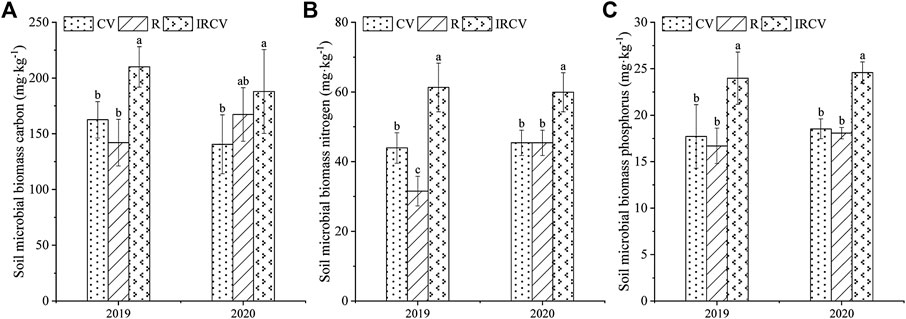
FIGURE 2. Changes in soil MBC (A), MBN (B), and MBP (C) under three cropping systems in 2019–2020. Different lowercase letters represent significant differences among treatments (p < 0.05). CV, R, and IRCV represent the common vetch monoculture, rape monoculture, and R and CV intercropping, respectively.
Soil sucrase and urease activities in the intercropping soil were significantly higher by 40.24% and 16.47% in 2019 and 35.57% and 16.84% in 2020, respectively, than with rape monoculture (Figures 3A,B, p < 0.05). Intercropping increased sucrase activity by 16.10% and 13.69% compared with common vetch monoculture in both years (Figure 3A). However, the soil alkaline phosphatase activity in the monoculture soil (except for common vetch monoculture in 2019) was higher than that in the intercropping soil, but this difference was not significant (Figure 3C).
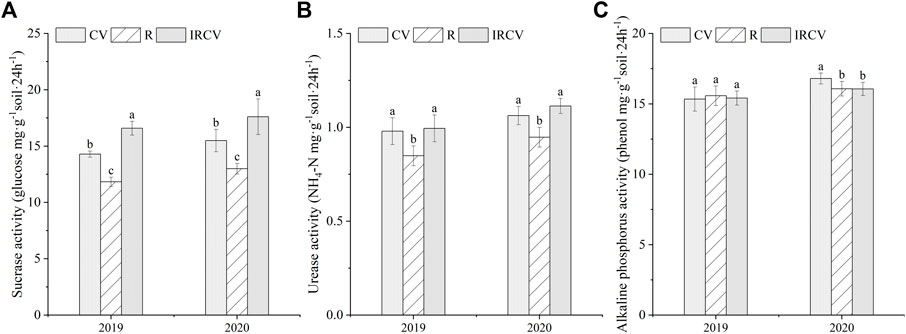
FIGURE 3. Changes in soil enzyme activities under the three cropping systems in 2019–2020. Different lowercase letters represent significant differences among treatments (p < 0.05). Soil sucrase (A), urease (B), and soil alkaline phosphatase (C). CV, R, and IRCV represent common vetch monoculture, rape monoculture, and R and CV intercropping, respectively.
Correlation between aboveground biomass and soil properties
Pearson correlation coefficients were calculated between the aboveground biomass and soil properties (Table 2). The aboveground biomass of common vetch and rape was significantly positively correlated with SOM and AP. In addition, aboveground biomass was significantly negatively correlated with SBD and ALP.
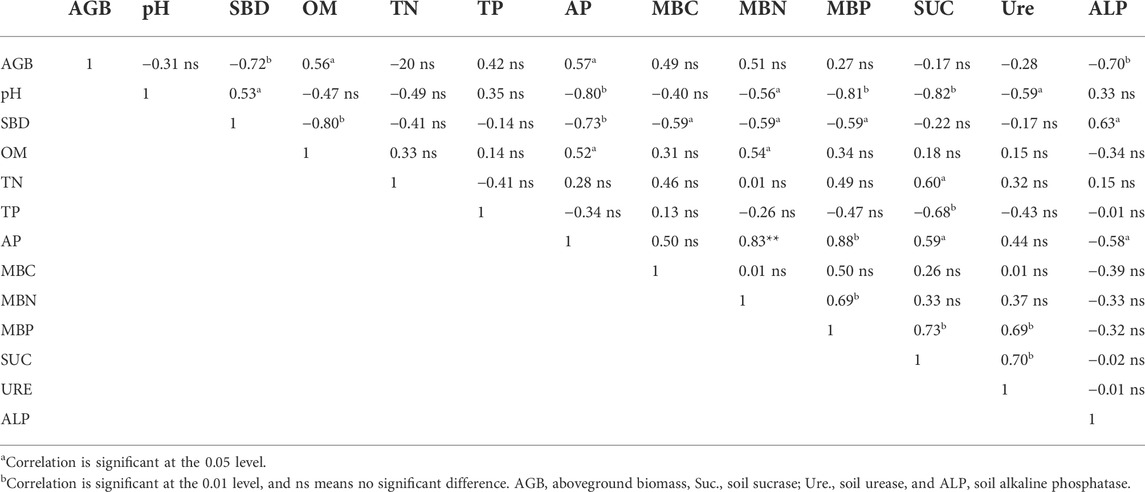
TABLE 2. Correlation coefficients between aboveground biomass and soil properties for all treatments.
Soil bacterial and fungal community diversity
The soil bacterial abundance index values (observed OTUs and Chao1 index) were significantly lower in the intercropped soil than in the monoculture soil. Bacterial diversity index values based on the Shannon and Simpson indices showed no significant difference between monoculture and intercropping. The Shannon index was the highest with the R treatment, followed by the IRCV and CV treatments (Table 3). The soil fungi abundance index values (observed OTUs and Chao1 index) were highest with the CV treatment, followed by the IRCV and R treatments (Table 4). However, the Shannon and Simpson indices were lowest with the CV treatment (Table 4).
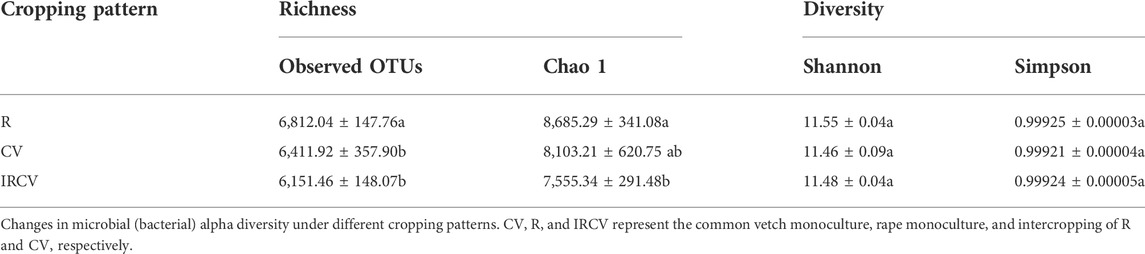
TABLE 3. Richness, diversity of the bacterial communities of the rhizosphere soil under different treatments.
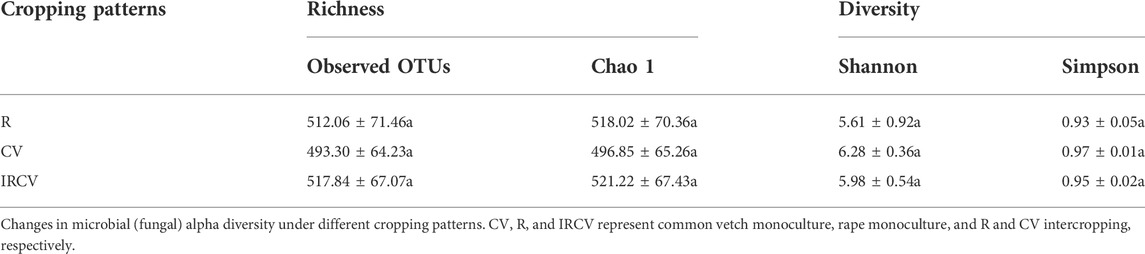
TABLE 4. Richness, diversity of the fungal communities of the rhizosphere soil under different treatments.
PCoA showed that the fungal communities seen between rape and common vetch were clearly separated (Figure 4B), which indicates that the crop species influenced the soil microbial community. However, the difference in bacterial communities between treatments was not significant (Figure 4A).
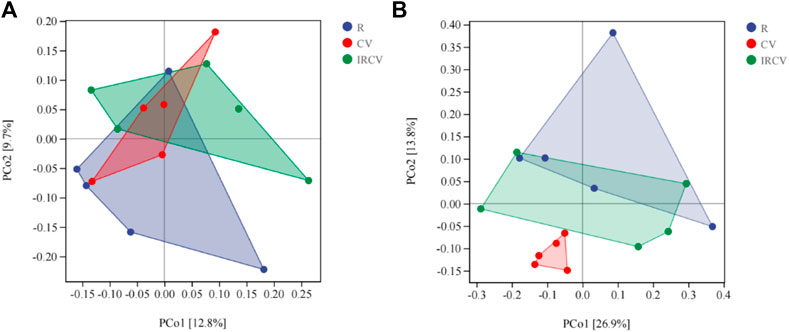
FIGURE 4. Principal coordinates analysis (PCoA) representation of soil bacterial (A) and fungal communities (B) in the three cropping sequences (OTUs with relative abundance of more than 0.01%). CV, R, and IRCV represent common vetch monoculture, rape monoculture, and R and CV intercropping, respectively.
Shifts of soil bacterial and fungal community composition and responses to soil properties and enzyme activities
None of the bacterial phyla showed a significant difference between treatments (one-way ANOVA p > 0.05). Proteobacteria (31.92–33.07%) were the most abundant, followed by Acidobacteria and Actinobacteria, and Gemmatimonadetes (Figure 5A). The relative abundance of Proteobacteria increased by 3.60% under IRCV treatment compared with CV treatment. The CV treatment had the highest relative abundance of Acidobacteria (21.35%), which was higher compared with their relative abundance under R treatment (7.94%). The IRCV treatment increased the relative abundance of Gemmatimonadetes, Bacteroidetes, and Rokubacteria by 15.12%, 9.55%, and 5.81%, respectively, compared with the R treatment. The relative abundance of Chloroflexi was significantly (p < 0.05) decreased under IRCV (7.08%) treatment compared with CV (8.14%) and R (8.01%) treatments. The relative abundances of the bacterial genera are shown in Figure 5C. The predominant phyla of the bacterial community were Subgroup_6, MND1, RB41, Sphingomonas, and KD4-96. The IRCV treatment increased the relative abundance of Subgroup_6, RB41, Rokubacteriales, 67_14, Subgroup_7, and Haliangium by 5.12%, 6.22%, 5.69%, 41.82%, 1.60%, and 4.10%, respectively, compared with the R treatment. Moreover, compared with R and CV, the relative abundance of Sphingomonas was decreased with the IRCV treatment by 12.90% and 20.37%, respectively.
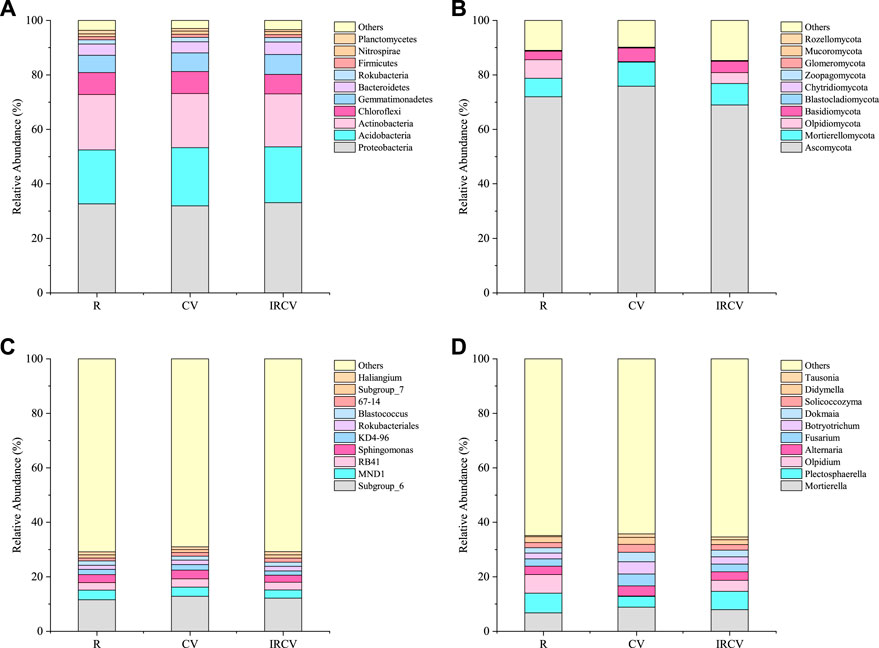
FIGURE 5. Relative abundances of the dominant bacteria [(A), at phylum level; (C), at genus level] and fungi [(B), at phylum level; (D), at genus level] in the three cropping patterns. The top ten dominant phyla and genera of bacteria and fungi, respectively, were used in the analysis. The relative abundance of each phylum or genus was calculated by the average relative abundance of this phylum or genus across all soils divided by the average total relative abundance of all phyla in each cropping sequence. CV, R, and IRCV represent common vetch monoculture, rape monoculture, and R and CV intercropping, respectively.
Soil fungal communities were dominated by Ascomycota (68.92%–75.84%, average 72.25%), Mortierellomycota (6.73%–8.86%, average 7.84%), Olpidiomycota (0.21%–6.83%, average 3.68%), and Basidiomycota (3.19%–4.94%, average 4.09%), which made up more than 87.86% of the total sequences. Blastocladiomycota, Chytridiomycota, Zoopagomycota, Glomeromycota, Mucoromycota, and Rozellomycota were detected at relatively low abundances (relative abundance <1%). Compared with the R treatment, IRCV increased the relative abundance of Mortierellomycota and Basidiomycota by 17.68% and 29.47%, respectively (Figure 5B). The fungal genera with a high relative abundance (>1%) are shown in Figure 5D. The community following CV treatment had a significantly lower relative abundance of Olpidium, which decreased by 96.93% and 94.75%, respectively, compared with R and IRCV. The abundance of Mortierella, Alternaria, Fusarium, Botryotrichum, Dokmaia, Solicoccozyma, Didymella, and Tausonia were elevated under CV treatment compared with R and IRCV treatments.
Redundancy analysis (RDA) was conducted to determine the influence of variations in soil properties on microbial community composition at the phylum level. For the bacterial community, axis one explained 71.85% and axis two explained 8.93% of the total variations. The variation in the bacterial community was mainly influenced by the soil ALP content (F = 7.1, p = 0.02) and soil TP content (F = 4.9, p = 0.048) (Figure 6A). Soil sucrase (F = 4.0, p = 0.04) was found to be the most important parameter influencing the community composition of soil fungi (Figure 6B).
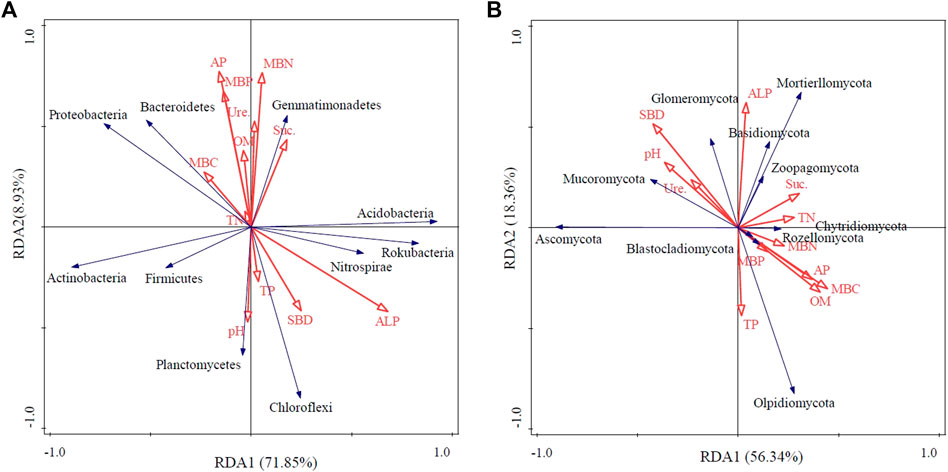
FIGURE 6. Redundancy analysis (RDA) of bacterial (A) and fungal (B) communities at the phylum level with soil variables. SBD, soil bulk density; SOM, soil organic matter; TN, total nitrogen; TP, total phosphorus, AP, available phosphorus, MBC, microbial biomass carbon; MBN, microbial biomass nitrogen; MBP, microbial biomass phosphorus, Suc., soil sucrase; Ure., soil urease, and ALP, soil alkaline phosphatase.
Discussion
Biomass and soil properties
Intercropping increased dry matter (Figure 7), but this was not our primary interest as these effects have already been shown, with higher uptake of nitrogen (Blessing et al., 2022) and phosphate (Li et al., 2007) through intercropping maize and beans. In 2019, there was no significant difference between the biomass obtained with intercropping treatment and that obtained with rape monoculture. In 2020, the yield with the intercropping treatment was significantly lower than that with the R treatment. This was due to the fact that intercropping treatment involved two rows of rape and four rows of common vetch. The biomass per plant of common vetch was lower than that of rape, leading to the above results.
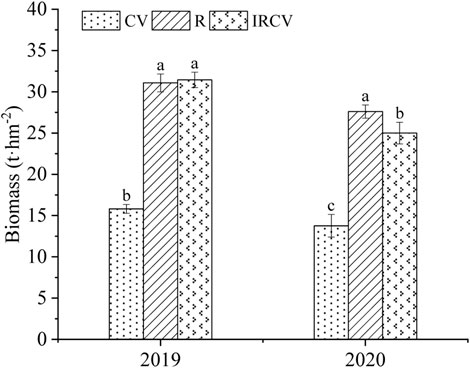
FIGURE 7. Biomass in the three cropping systems in 2019–2020. Different lowercase letters represent significant differences among treatments (p < 0.05).
The physical and chemical properties of soil are generally regarded to be indicators of soil quality (Schoenholtz et al., 2000). Gong et al. (2019) noted that proso millet/mung bean intercropping significantly increased soil nutrient retention, including N, P, K, and soil temperature, findings that were reflected in the current study. We found that SBD was significantly lower with the IRCV treatment than with the monocultures (Table 1) and corresponded with the opposite trends with higher SOC, TN, and available P content (Table 1) compared with the monoculture cropping systems. This indicated that intercropping can effectively maintain soil moisture and soil porosity. Soil pH showed a decreased trend with IRCV treatment relative to that seen with the R and CV treatments, although the differences among these three treatments were not significant in 2019, which contrasts with previous results reported by Betencourt et al. for chickpeas (2012). This result may be due to the fact that the distribution and infiltration of roots in the soil may help to improve the physical properties and structure of soil and further increase the nutrient supply to rhizosphere soil (Li et al., 2016). The root nodules of common vetch can increase soil N retention and reduce SOM loss, which helps to maintains a healthy ecosystem (Amusan et al., 2011; Jayaraman et al., 2020; Wang et al., 2022). Similar results have been reported by Soltangheisi et al. (2018) as the AP content increased in IRCV, as rape and common vetch can be both a valuable source of phosphorus for mobilizing more P. However, Zhang and Li (2003) found soil TN and AP content decreased, as intercropping used soil nutrients more efficiently than monoculture cropping. Our correlation analysis also showed that SOM and AP were positively correlated with aboveground biomass (Table 2), indicating that higher levels of soil nutrients increased the biomass of common vetch and rape.
As a result of management practices, microbial biomass and soil enzyme activity are also essential for soil metabolic capacity and nutrient cycling (Saha et al., 2008; Spohn and Kuzyakov, 2013). MBC, MBN, and MBP did indeed significantly increase with the intercropping treatment (Figure 2), which is direct evidence of the well-documented stimulation of microbial communities by intercropping. Besides microbial biomass, we also reported that soil sucrase, soil urease, and soil alkaline phosphatase (Figure 3) were enhanced in the rhizosphere, which are additional indications of the advantages of intercropping. These findings are in accordance with those of a previous study conducted by Ahmed et al. (2019). Liu et al. (2021) reported that F. multifora-A. paniculate intercropping system significantly increased microbial biomass and soil enzyme activity, which promoted the release of soil nutrients. These results could be due to variations in the origin, form, or persistence of different enzymatic groups under different crop patterns (Trasar-Cepeda et al., 2008). Pearson’s correlation analysis showed that MBP was significantly positively correlated with soil urease (Table 2), probably because the numbers of microorganisms present in soil are believed to be the drivers of enhanced microbial activities due to the presence of phosphatases (Turner and Haygarth, 2005). The findings of Dakora and Phillips (2002) are also in agreement with the factthat fungi is responsible for ALP. If this is the case, the observed changes in soil properties when common vetch was intercropped with rape could have contributed to mediating aboveground–belowground cycling in this intercropping system (Tang et al., 2016).
Microbial diversity and microbial composition
Differences in soil characteristics can explain differences between soil bacterial and fungal communities, because microbes provide nutrients for plant growth (Tian et al., 2019). Our results confirmed that bacterial alpha diversity was higher under monocropping, especially the R treatment (Table 3), but it was difficult to predict fungal alpha diversity for different intercropping systems (Table 4), indicating the higher sensitivity of the bacterial community than that of the fungal community to the common vetch–rape intercropping system. This result is supported by the work of Gong et al. (2019), which suggested that intercropping was beneficial for the control of pests and diseases (Jarvis et al., 2015). Additionally, the diversity of bacterial communities associated with intercropping of sugarcane with soybean was significantly increased compared with monoculture and played an indispensable role in shifting the root environment to help healthy plant growth (Malviya et al., 2021).
Soil nutrient content and soil structural characteristics are crucial factors affecting bacterial and fungal community composition (Mouhamadou et al., 2013). In this study, with respect to bacterial composition, we found that the phyla Proteobacteria, Gemmatimonadetes, Bacteroidetes, and Rokubacteria were significantly increased under intercropping treatments. This result is consistent with the findings of a recent study that investigated peanut–cassava intercropping, which reported that the percentage of Gemmatimonadetes in the rhizospheric soils of intercropping systems was higher than in monoculture soils (Tang et al., 2020). In contrast, Chloroflexi significantly decreased, which is similar to Li et al.’s (2021) results showing the effect on microbial community structure of intercropping Ophiopogon japonicus in a tea garden. Chloroflexi grows slowly and tends to congregate in low-nutrient environments (NüBel et al., 2001). Our correlation analysis between soil environmental variables and the dominant bacteria further confirmed this result, and there was a negative correlation between Chloroflexi and TN, TP, OM, and AP (Figure 6A). Regarding the fungal community, intercropping increased the relative abundance of Mortierellomycota and Basidiomycota, a finding that was in line with those described for arid farmlands on a global scale (Li et al., 2018).
At the genus level, our study showed that the relative abundance of Sphingomonas decreased under IRCV treatment. Sphingomonas has been shown to exhibit N cycling and has been associated with the antagonistic activity of soil nutrients (Videira et al., 2009; Prakash et al., 2012; Xu et al., 2018). The fungal genus with a low relative abundance under IRCV treatment compared with CV treatment was Fusarium. Fusarium can cause legume stem disease (Cannon et al., 2012). The change in soil microbial community composition among different cropping patterns might be due to the soil temperature and moisture and indicated that common vetch–rape intercropping can maintain a healthy soil ecosystem.
Conclusion
In conclusion, IRCV significantly increased the biomass of common vetch. As a result of the intercropping of common vetch with rape, soil quality was improved by increasing the microbial biomass, the activity of some soil enzymes, bacterial diversity, and the relative abundances of potentially beneficial microorganisms. Our results also indicated that intercropping led to more bacterial diversity than that seen for fungi. The common vetch–rape intercropping system is a good example of a new strategy for ecological planting of pasture, which will be of great importance for sustainable development and animal husbandry. Moreover, intercropping significantly increased the soil AP content, which is a useful finding for improving the activation of insoluble P in soil.
Data availability statement
The raw data supporting the conclusion of this article will be made available by the authors, without undue reservation.
Author contributions
JQ and LL conceived and designed the experiments; YW and JY performed the experiments; XZ performed the statistical analysis; JQ and LL wrote the paper.
Funding
This study was funded by the Inner Mongolia Science and Technology special project on rational utilization technology and integration mode of water resources in dryland areas (2020ZD0005-0401).
Acknowledgments
We would like to thank the Farming ecological research Team for field and data collection.
Conflict of interest
The authors declare that the research was conducted in the absence of any commercial or financial relationships that could be construed as a potential conflict of interest.
Publisher’s note
All claims expressed in this article are solely those of the authors and do not necessarily represent those of their affiliated organizations, or those of the publisher, the editors and the reviewers. Any product that may be evaluated in this article, or claim that may be made by its manufacturer, is not guaranteed or endorsed by the publisher.
References
Ahmed, W., Jing, H., Kaillou, L., Qaswar, M., Khan, M. N., Jin, C., et al. (2019). Changes in phosphorus fractions associated with soil chemical properties under long-term organic and inorganic fertilization in paddy soils of southern China. PLoS One 14 (5), e0216881. doi:10.1371/journal.pone.0216881
Amusan, A. O., Adetunji, M. T., Azeez, J. O., and Bodunde, J. G. (2011). Effect of the integrated use of legume residue, poultry manure and inorganic fertilizers on maize yield, nutrient uptake and soil properties. Nutr. Cycl. Agroecosyst. 90, 321–330. doi:10.1007/s10705-011-9432-6
Balota, E. L., Colozzi-Filho, A., Andrade, D. S., and Dick, R. P. (2003). Microbial biomass in soils under different tillage and crop rotation systems. Biol. Fertil. Soils 38, 15–20. doi:10.1007/s00374-003-0590-9
Bao, S. D. (2005). Soil analysis in Agricultural chemistry (in Chinese). 3rd Edn. Beijing: China Agriculture Press.
Blessing, D. J., Gu, Y., Cao, M. J., Cui, Y., Wang, X. W., Asante-Badu, B., et al. (2022). Overview of the advantages and limitations of maize-soybean intercropping in sustainable agriculture and future prospects: a review. Chil. J. Agric. Res. 82, 177–188. doi:10.4067/s0718-58392022000100177
Brookes, P. C., Kragt, J. F., Powlson, D. S., and Jenkinson, D. S. (1985). Chloroform fumigation and the release of soil nitrogen: the effects of fumigation time and temperature. Soil Biol. Biochem. 17, 831–835. doi:10.1016/0038-0717(85)90143-9
Brookes, P. C., Powlson, D., and Jenkinson, D. (1984). Phosphorus in the soil microbial biomass. Soil Biol. Biochem. 16, 169–175. doi:10.1016/0038-0717(84)90108-1
Brookes, P. C., Powlson, D. S., and Jenkinson, D. S. (1982). Measurement of microbial biomass phosphorus in soil. Soil Biol. Biochem. 14, 319–329. doi:10.1016/0038-0717(82)90001-3
Cannon, P. F., Buddie, A. G., Bridge, P. D., de Neergaard, E., Lübeck, M., and Askar, M. M. (2012). Lectera, a new genus of the Plectosphaerellaceae for the legume pathogen Volutella colletotrichoides. MycoKeys 3, 23–36. doi:10.3897/mycokeys.3.3065
Cong, W., Hoffland, E., Li, L., Six, J., Sun, J., Bao, X., et al. (2014). Intercropping enhances soil carbon and nitrogen. Glob. Chang. Biol. 21, 1715–1726. doi:10.1111/gcb.12738
Dakora, F. D., and Phillips, D. A. (2002). Root exudates as mediators of mineral acquisition in low-nutrient environments. Plant Soil 245, 201–213. doi:10.1007/978-94-017-1570-6_23
Finzi, A. C., Abramoff, R. Z., Spiller, K. S., Brzostek, E. R., Darby, B. A., Kramer, M. A., et al. (2015). Rhizosphere processes are quantitatively important components of terrestrial carbon and nutrient cycles. Glob. Chang. Biol. 21, 2082–2094. doi:10.1111/gcb.12816
Franchini, J. C., Crispino, C. C., Souza, R. A., Torres, E., and Hungria, M. (2007). Microbiological parameters as indicators of soil quality under various soil management and crop rotation systems in southern Brazil. Soil Tillage Res. 92 (1-2), 18–29. doi:10.1016/j.still.2005.12.010
Gardes, M., and Bruns, T. D. (1993). ITS primers with enhanced specificity for basidiomycetes - application to the identification of mycorrhizae and rusts. Mol. Ecol. 2, 113–118. doi:10.1111/j.1365-294x.1993.tb00005.x
Gong, X., Liu, C., Li, J., Luo, Y., Yang, Q., Zhang, W., et al. (2019). Responses of rhizosphere soil properties, enzyme activities and microbial diversity to intercropping patterns on the Loess Plateau of China. Soil Tillage Res. 195, 104355. doi:10.1016/j.still.2019.104355
Guan, S. Y., Zhang, D., and Zhang, Z. (1986). Soil enzyme and its research methods. Beijing: Chinese Agricultural Press, 274–297.
Guo, Q. X., Yan, L. J., Korpelainen, H., Niinemets, U., and Li, C. Y. (2019). Plant-plant interactions and N fertilization shape soil bacterial and fungal communities. Soil Biol. Biochem. 128, 127–138. doi:10.1016/j.soilbio.2018.10.018
He, D., Xiang, X., He, J. S., Wang, C., Cao, G., Adams, J., et al. (2016). Composition of the soil fungal community is more sensitive to phosphorus than nitrogen addition in the alpine meadow on the Qinghai-Tibetan Plateau. Biol. Fertil. Soils 52 (8), 1059–1072. doi:10.1007/s00374-016-1142-4
He, X., Zhu, S., Wang, H., Xie, Y., Sun, Y., Gao, D., et al. (2010). Crop diversity for ecological disease control in potato and maize. J. Resour. Ecol. 1, 45–50. doi:10.3969/j.issn.1674-764x.2010.01.006
Jarvis, S. G., Woodward, S., and Taylor, A. F. S. (2015). Strong altitudinal partitioning in the distributions of ectomycorrhizal fungi along a short (300 m) elevation gradient. New Phytol. 206 (3), 1145–1155. doi:10.1111/nph.13315
Jayaraman, S., Sinha, N. K., Mohanty, M., Hati, K. M., Chaudhary, R. S., Shukla, A. K., et al. (2020). Conservation tillage, residue management, and crop rotation effects on soil major and micro-nutrients in semi-arid vertisols of India. J. Soil Sci. Plant Nutr. 21 (1), 523–535. doi:10.1007/s42729-020-00380-1
Li, L., Li, S. M., Sun, J. H., Zhou, L. L., Bao, X. G., Zhang, H. G., et al. (2007). Diversity enhances agricultural productivity via rhizosphere phosphorus facilitation on phosphorus-deficient soils. Proc. Natl. Acad. Sci. U. S. A. 104, 11192–11196. doi:10.1073/pnas.0704591104
Li, Q. S., Lei, W. X., Liu, J. X., Zhu, J. W., Shi, L. S., and Cai, Pumo. (2021). Effects of intercropping ophiopogon japonicus into tea plantation on its soil physicochemical properties and microbial community structure. J. South. Agric. 52 (12), 9. doi:10.3969/j.issn.2095-1191.2021.12.020
Li, Q. S., Wu, L. K., Chen, J., Khan, M. A., Luo, X. M., and Lin, W. X. (2016). Biochemical and microbial properties of rhizospheres under maize/peanut intercropping. J. Integr. Agric. 15 (1), 101–110. doi:10.1016/s2095-3119(15)61089-9
Li, X. F., Wang, C. B., Zhang, W. P., Wang, L. H., Tian, X. L., Yang, S. C., et al. (2018). The role of complementarity and selection effects in P acquisition of intercropping systems. Plant soil 422, 479–493. doi:10.1007/s11104-017-3487-3
Ling, N., Chen, D., Guo, H., Wei, J., Bai, Y., Shen, Q., et al. (2017). Differential responses of soil bacterial communities to long-term N and P inputs in a semi-arid steppe. Geoderma 292, 25–33. doi:10.1016/j.geoderma.2017.01.013
Liu, C., Cai, Q., Liao, P., Jiang, X., Tang, X., Yang, Q., et al. (2021). Effects of Fallopia multiflora–Andrographis paniculata intercropping model on yield, quality, soil nutrition and rhizosphere microorganisms of F. multiflora. Plant Soil 467 (1-2), 465–481. doi:10.1007/s11104-021-05106-5
Malviya, M. K., Solanki, M. K., Li, C.-N., Wang, Z., Zeng, Y., Verma, K. K., et al. (2021). Sugarcane-legume intercropping can enrich the soil microbiome and plant growth. Front. Sustain. Food Syst. 5, 606595. doi:10.3389/fsufs.2021.606595
Meng, L. J., and Wu, F. Z. (2004). Advances in soil enzymology. J. Northeast Agric. Univ. 33 (5), 622–626. doi:10.19720/j.cnki.issn.1005-9369.2004.05.024
Mi, J., Gregorich, E. C., Xu, S., McLaughlin, N. B., and Liu, J. (2018). Effects of a one-time application of bentonite on soil enzymes in a semi-arid region. Can. J. Soil Sci. 98 (3), 542–555. doi:10.1139/cjss-2018-0011
Mouhamadou, B., Puissant, J., Personeni, E., Desclos-Theveniau, M., Kastl, E. M., Schloter, M., et al. (2013). Effects of two grass species on the composition of soil fungal communities. Biol. Fertil. Soils 49 (8), 1131–1139. doi:10.1007/s00374-013-0810-x
Mori, H., Maruyama, F., Kato, H., Toyoda, A., Dozono, A., Ohtsubo, Y., et al. (2014). Design and experimental application of a novel nondegenerate universal primer set that amplifes prokaryotic 16S rRNA genes with a low possibility to amplify eukaryotic rRNA genes. DNA Res 21 (8), 217–227. doi:10.1093/dnares/dst052
NüBel, U., Bateson, M. M., Madigan, M. T., KüHl, M., and Ward, D. M. (2001). Diversity and distribution in hypersaline microbial mats of bacteria related to Chloroflexus spp. Appl. Environ. Microbiol. 67 (9), 4365–4371. doi:10.1128/AEM.67.9.4365-4371.2001
Nyawade, S. O., Karanja, N. N., Gachene, C. K. K., Gitari, H. I., Schulte-Geldermann, E., and Parker, M. L. (2019). Short-term dynamics of soil organic matter fractions and microbial activity in smallholder potato-legume intercropping systems. Appl. Soil Ecol. 142, 123–135. doi:10.1016/j.apsoil.2019.04.015
Ping, C., Wu, Y. J., Solonda, Ba G., De, J., Ren, Q. C., Qu, J., et al. (2022). Effects of fertilizer and mixed sowing ratio on vicia sativa production performance in Lhasa, Tibet. Chin. J. Herbivore Sci. 42, 39–42. doi:10.3969/j.issn.2095-3887.2022.02.007
Prakash, O., Green, S. J., Jasrotia, P., Overholt, W. A., Canion, A., Watson, D. B., et al. (2012). Rhodanobacter denitrificans sp. nov., isolated from nitrate-rich zones of a contaminated aquifer. Int. J. Syst. Evol. Microbiol. 62 (Pt 10), 2457–2462. doi:10.1099/ijs.0.035840-0
Ren, C. J., Zhao, F. Z., Kang, D., Yang, G. H., Han, X. H., Tong, X. G., et al. (2016). Linkages of C:N:P stoichiometry and bacterial community in soil following afforestation of former farmland. For. Ecol. Manag. 376, 59–66. doi:10.1016/j.foreco.2016.06.004
Saha, S., Prakash, V., Kundu, S., Kumar, N., and Mina, B. L. (2008). Soil enzymatic activity as affected by long term application of farm yard manure and mineral fertilizer under a rainfed soybean–wheat system in N-W Himalaya. Eur. J. Soil Biol. 44 (3), 309–315. doi:10.1016/j.ejsobi.2008.02.004
Schoenholtz, S. H., Van, Miegroet., and Burger, J. A. (2000). A review of chemical and physical properties as indicators of forest soil quality: challenges and opportunities. For. Ecol. Manage. 138, 335–356. doi:10.1016/S0378-1127(00)00423-0
Soltangheisi, A., Rodrigues, M., Coelho, M. J. A., Gasperini, A. M., Sartor, L. R., and Pavinato, P. S. (2018). Changes in soil phosphorus lability promoted by phosphate sources and cover crops. Soil Tillage Res. 179, 20–28. doi:10.1016/j.still.2018.01.006
Spohn, M., and Kuzyakov, Y. (2013). Phosphorus mineralization can be driven by microbial need for carbon. Soil Biol. Biochem. 61, 69–75. doi:10.1016/j.soilbio.2013.02.013
Tang, X., Placella, S. A., Daydé, F., Bernard, L., Robin, A., Journet, E. P., et al. (2016). Phosphorus availability and microbial community in the rhizosphere of intercropped cereal and legume along a P-fertilizer gradient. Plant Soil 407 (1-2), 119–134. doi:10.1007/s11104-016-2949-3
Tang, X., Zhong, R., Jiang, J., He, L., Huang, Z., Shi, G., et al. (2020). Cassava/peanut intercropping improves soil quality via rhizospheric microbes increased available nitrogen contents. BMC Biotechnol. 20, 13. doi:10.1186/s12896-020-00606-1
Tian, X. L., Wang, C. B., Bao, X. G., Wang, P., Li, X. F., Yang, S. C., et al. (2019). Crop diversity facilitates soil aggregation in relation to soil microbial community composition driven by intercropping. Plant Soil 436 (1-2), 173–192. doi:10.1007/s11104-018-03924-8
Trasar-Cepeda, C., Leirós, M. C., and Gil-Sotres, F. (2008). Hydrolytic enzyme activities in agricultural and forest soils. some implications for their use as indicators of soil quality. Soil Biol. Biochem. 40 (9), 2146–2155. doi:10.1016/j.soilbio.2008.03.015
Turner, B. L., and Haygarth, P. M. (2005). Phosphatase activity in temperate pasture soils: potential regulation of labile organic phosphorus turnover by phosphodiesterase activity. Sci. Total Environ. 344 (1), 27–36. doi:10.1016/j.scitotenv.2005.02.003
Videira, S. S., de Araujo, J. L., Rodrigues Lda, S., Baldani, V. L., and Baldani, J. I. (2009). Occurrence and diversity of nitrogen-fixing Sphingomonas bacteria associated with rice plants grown in Brazil. FEMS Microbiol. Lett. 293 (1), 11–19. doi:10.1111/j.1574-6968.2008.01475.x
Vos, B. D., Meirvenne, M. V., Quataert, P., Deckers, J., and Muys, B. (2005). Predictive quality of pedotransfer functions for estimating bulk density of forest soils. Soil Sci. Soc. Am. 69, 500–510. doi:10.2136/sssaj2005.0500
Wang, J. X., Lu, X. N., Zhang, J. E., Wei, H., Li, M. J., Lan, N., et al. (2021). Intercropping perennial aquatic plants with rice improved paddy field soil microbial biomass, biomass carbon and biomass nitrogen to facilitate soil sustainability. Soil Tillage Res. 208, 104908. doi:10.1016/j.still.2020.104908
Wang, Q. Y., Zhou, D. M., and Long, C. (2009). Microbial and enzyme properties of apple orchard soil as affected by long-term application of copper fungicide. Soil Biol. Biochem. 41 (7), 1504–1509. doi:10.1016/j.soilbio.2009.04.010
Wang, W., Wang, M., Jin, B., and Liu, Y. (2015). Study on international trade structure of Chinese forage products and its enlightenment. Chin. Agric. Sci. Bull. 31 (26), 1–6. doi:10.3969/j.issn.1000-4149.2021.00.038
Wang, X., Duan, Y., Zhang, J., Ciampitti, I. A., Cui, J., Qiu, S., et al. (2022). Response of potato yield, soil chemical and microbial properties to different rotation sequences of green manure-potato cropping in North China. Soil Tillage Res. 217, 105273. doi:10.1016/j.still.2021.105273
Wei, Q., Sai, J., Wang, J., Cheng, Y., Lu, Z., Zhang, X., et al. (2020). Effects of different grazing patterns on soil nutrients in degraded farmland. Chin. Agric. Sci. Bull. 36 (24), 63–71.
White, T., Bruns, T., Lee, S., and Taylor, J. (1990). “Amplifcation and direct sequencing of fungal ribosomal RNA genes for phylogenetics,” in PCR protocols: a guide to methods and applications. Editors M. A. Innis, D. H. Gelfand, J. J. Sninsky, and T. J. White, PCR protocols: A guide to methods and applications. PCR protocols: a guide to methods and applications, 315–322.
Xu, L., Yi, M., Yi, H., Guo, E., and Zhang, A. (2018). Manure and mineral fertilization change enzyme activity and bacterial community in millet rhizosphere soils. World J. Microbiol. Biotechnol. 34, 8. doi:10.1007/s11274-017-2394-3
Xue, L., Chen, H. Y., and Kuang, L. G. (2003). Studies on soil nutrients, microorganisms and enzyme activities in mixed forest of Pinus elliottii. J. Appl. Ecol. 14 (01), 157–159.
Yang, W., Li, C., Wang, S., Zhou, B., Mao, Y., Rensing, C., et al. (2021). Influence of biochar and biochar-based fertilizer on yield, quality of tea and microbial community in an acid tea orchard soil. Appl. Soil Ecol. 166, 104005. doi:10.1016/j.apsoil.2021.104005
Yin, W., Chai, Q., Guo, Y., Feng, F., Zhao, C., Yu, A., et al. (2017). Reducing carbon emissions and enhancing crop productivity through strip intercropping with improved agricultural practices in an arid area. J. Clean. Prod. 166, 197–208. doi:10.1016/j.jclepro.2017.07.211
Yuan, Y., Tang, D., Sun, X., Lu, Y., Guo, Y., Zhang, Y., et al. (2021). Selection of rapeseed varieties for multiple cropping after wheat in Western Jilin. China Feed 19, 83–86. doi:10.15906/j.cnki.cn11-2975/s.20211917
Zhang, F., and Li, L. (2003). Using competitive and facilitative interactions in intercropping systems enhances crop productivity and nutrient-use efficiency. Plant Soil 248, 305–312. doi:10.1023/a:1022352229863
Zhang, N. N., Sun, Y. M., Li, L., Wang, E. T., Chen, W. X., Yuan, H. L., et al. (2010). Effects of intercropping and Rhizobium inoculation on yield and rhizosphere bacterial community of faba bean (Vicia faba L.). Biol. Fertil. Soils 46 (6), 625–639. doi:10.1007/s00374-010-0469-5
Zhang, Q., Huang, G., Bian, X., Jiang, X., and Zhao, Q. (2012). Effects of intercropping on maize quality, yield, soil microbial quantity and enzyme activity. Acta Eco. Sin. 22, 7082–7090. doi:10.5846/stxb201110151526
Zhao, M., Jones, C. M., Meijer, J., Lundquist, P. O., Fransson, P., Carlsson, G., et al. (2017). Intercropping affects genetic potential for inorganic nitrogen cycling by root-associated microorganisms in Medicago sativa and Dactylis glomerata. Appl. Soil Ecol. 119, 260–266. doi:10.1016/j.apsoil.2017.06.040
Keywords: common vetch, intercropping, rape, soil microbial community diversity, soil characteristics
Citation: Qu J, Li L, Wang Y, Yang J and Zhao X (2022) Effects of rape/common vetch intercropping on biomass, soil characteristics, and microbial community diversity. Front. Environ. Sci. 10:947014. doi: 10.3389/fenvs.2022.947014
Received: 18 May 2022; Accepted: 06 July 2022;
Published: 19 August 2022.
Edited by:
Tim George, The James Hutton Institute, United KingdomReviewed by:
Vineet Kumar, National Environmental Engineering Research Institute (CSIR), IndiaMetin Turan, Yeditepe University, Turkey
Copyright © 2022 Qu, Li, Wang, Yang and Zhao. This is an open-access article distributed under the terms of the Creative Commons Attribution License (CC BY). The use, distribution or reproduction in other forums is permitted, provided the original author(s) and the copyright owner(s) are credited and that the original publication in this journal is cited, in accordance with accepted academic practice. No use, distribution or reproduction is permitted which does not comply with these terms.
*Correspondence: Lijun Li, imaullj@163.com