- 1School of International Economics and Management, Beijing Technology and Business University, Beijing, China
- 2School of Economics, Beijing Technology and Business University, Beijing, China
- 3Asia-Australia Business College, Liaoning University, Liaoning, China
As a global issue, climate warming has received extensive attention in recent years. Scientific identification of the network evolution, transfer path, and influencing factors of embodied carbon in trade is of great significance for the global joint response to climate change challenges. In this paper, based on the latest World Input-Output Database and carbon emissions data of 42 countries (the total carbon emissions of 42 countries account for about 80% of the world’s total carbon emissions), we use social network analysis to describe the global trade embodied carbon emission from 2000 to 2014, and explore the relevant factors that influence global embodied carbon emissions. From the overall network, a small number of countries produce more carbon emissions, of which China is gradually in the center of the global carbon emissions network, while the United States and the European Union are always in the center. The point entry degree of developed economies is larger, while the point out degree of developing countries is higher, indicating that part of carbon emissions from developed countries are transferred to developing countries through economic links. Through QAP analysis, it is found that factors such as population, energy structure, geographic distance, final consumption and trade agreements are the main factors of the evolution of the embodied carbon network, and these factors can explain 42.3% of the pattern of the implied carbon network in trade. Moreover, the decrease of the degree of proximity indicates that the path of carbon emission correlation between countries is shortening and it is necessary for the world to join hands to respond to climate change.
1 Introduction
Economic globalization has brought closer economic and trade ties among countries, yet the development of industry and manufacturing has also led to increasingly severe environmental problems. The production and trade of high-carbon products have contributed to a large number of carbon emissions, which have had a serious impact on the world’s climate. The global climate has been heating up in recent years, and problems such as melting icebergs and rising sea levels have emerged, which will eventually affect the survival and development of mankind. Governments are also gradually realizing the seriousness of the problem and the urgency of managing environmental issues, and countries around the world have adopted global agreements to control greenhouse gas emissions, for example, the Paris Agreement signed in 2016 that aims to keep the global average temperature rise within 2°C and strives to keep the temperature rise within 1.5°C.
Carbon emissions from a country not only have an impact on its environment and economy but the carbon-containing products produced in that country are also exported to other countries through global trade networks, causing environmental problems in other countries and thus affecting climate change in the world as a whole. Therefore, the problem of carbon emissions cannot be solved by a single country or a few countries alone but requires the joint efforts of all governments. Furthermore, it is necessary to look at the issue of carbon emissions from a global perspective, explore the footprint of carbon emissions in terms of economic activities among countries, and study the structural characteristics of carbon emission linkages among countries.
The current research on the issue of international carbon emissions mainly includes international carbon emissions measurement, international carbon leak, and embodied carbon in international trade. Since the different measurement methods lead to different results, it is important to study the measurement of global trade carbon emissions for all countries. Firstly, the IPCC method (Villarino et al., 2014) is one of the internationally recognized and common methods for measuring carbon emissions (Winjum et al., 1998), and the United Nations Framework Convention on Climate Change requires parties to regularly prepare national greenhouse gas emission inventories, which provides a reliable method for countries to measure carbon emissions (Antweiler et al., 2001). Due to the different industrial conditions and production differences in each country, the carbon emission inventories formulated by each country are basically different, so there are also relatively large differences in the carbon emissions finally measured by countries. At present, domestic and foreign scholars still use the IPCC method a lot. Winjum et al. calculated through the IPCC method that the carbon storage in the world’s wood forest products is gradually increasing (Winjum et al., 1998). Jin et al. used the IPCC method to calculate the scale of carbon emissions in the city and its nine municipal districts, providing support for the formulation of urban low-carbon development strategies (Jin et al., 2013). Furthermore, another option is the life cycle analysis (Rosenbaum et al., 2011; Pan et al., 2018), which is based on the life cycle of a particular product from the micro-level (Luo, et al., 2014). It is a quantification of the energy consumed by a product throughout its life cycle and is often used to calculate the embodied carbon emissions of industrial materials or buildings. Pan, W et al. developed a systematic methodological framework for examining the carbon emissions of high-rise buildings, based on the life cycle approach and proposed a multidimensional life cycle carbon approach including temporal, spatial, functional and methodological variables, the results reveal carbon-intensive processes in a building’s life cycle (Pan et al., 2018). Finally, the input-output method is also a basic method for measuring carbon emissions, which is often used to calculate the embodied carbon in international trade (Machado et al., 2001). Input-output method can be divided into single-region input-output method and multi-region input-output method, the latter is developed on the basis of the former. When many scholars use the single-region input-output model to calculate the carbon emissions of a country’s export trade, they find that the carbon emissions of exports are more than those of imports, and there are net exports of implied carbon to varying degrees (Sanchez-Choliz and Duarte, 2004; Dawkins et al., 2019, Gao et al., 2020). Jing-Li Fan et al. used a multi-regional input-output model to establish a link between implied carbon and global value chains to measure the carbon efficiency of international trade. It is found that the export carbon intensity of developing and emerging economies is significantly higher than that of import carbon intensity, while the opposite is true for developed countries (Fan et al., 2019).
The problem of carbon leakage arises because countries adopt different levels of policies on carbon emissions (Yu et al., 2021, King and van den Bergh, 2021), with countries with stringent policies transferring carbon emissions to countries with less stringent policies through global trade (Kuik and Hofkes. 2010), while the traditional “Kyoto Model” only considers direct national carbon emissions, and ignores inter-country transfers (Green, 2015), which further exacerbates carbon leakage (Munksgaard and Pedersen, 2001). Later as some scholars suggested, “producer responsibility” could be not the only principle, and the responsibility of consumers should also be considered, to better define the responsibility of carbon emissions and promote better global climate governance (Ferng. 2003; Turner. 2014; Baloch. 2022).
The concept of embodied carbon was developed from the concept of “embedded energy” proposed at the meeting of the International Federation of Advanced Research Institutions (IFIAS) Energy Analysis Working Group in 1974. Some scholars add the name of resources or pollutant emissions after “embodied” to conduct specific research, and finally derive the concept of embodied carbon. The United Nations Framework Convention on Climate Change defines embodied carbon as “the carbon dioxide emitted during the process of obtaining, manufacturing, processing and transporting goods from raw materials to the products purchased by consumers” (Fan et al., 2019). At present, most of the research on embodied carbon is focused on the measurement of embodied carbon and the division of responsibility for the emission of embodied carbon. The method used for the measurement of embodied carbon is mainly the input-output model. The single-regional input-output model was initially used, and later, with globalization and international division of labor, the multi-regional input-output model was widely adopted. The division of responsibility for embodied carbon emissions is mainly divided into two perspectives: producers and consumers. Han, MY used a multi-regional input-output model to analyze and found that consumption in countries such as the United States and the European Union caused about 30% of carbon emissions. They argue that it is more fair to measure embodied carbon emissions responsibility from a consumer perspective (Han et al., 2018). Zhao, GM used the global multi-regional input-output table to calculate the carbon emission intensity under different trade modes, and analyzed the relevant factors affecting the carbon emission intensity. The results show that the carbon emission intensity of developing countries is higher than that of developed countries. Industrialization can promote the reduction of carbon emission intensity in developing countries. The increase of PGDP can also reduce the carbon emission intensity in the process of global trade (Zhao and Liu, 2020). Regarding the study of global trade embodied carbon, most scholars have found that developed countries are the net importers of embodied carbon while developing countries are mostly the net exporters of embodied carbon, Developed countries should consider giving certain technical assistance to developing countries or help developing countries share the pressure of carbon emission reduction. (Tian et al., 2014; Dong et al., 2017; Jakob 2021; Mangr and Ahin, 2022; Wu et al., 2022).
The above literature provides the basis and reference for the research of this paper, but there are still places to be improved and supplemented: In the study of the trade embodied carbon, some literatures use the unweighted network, so the corresponding conclusions can be deeper. In addition, some people who use weighted networks for research can also try different weight settings to more comprehensively grasp the evolution path of the network. On the other hand, most studies pay more attention to the network itself, ignoring the research on the influencing factors of the network, and some related studies try to increase the variables in the econometric model, but there is a problem of multicollinearity.
Therefore, based on the existing literature, this paper attempts to observe the evolution of trade-implied carbon networks by combining unweighted and weighted networks, and applies world input-output tables and carbon emission data to construct a global carbon emission matrix (Sanchez-Choliz and Duarte, 2004; Han et al., 2018), forming a multi-node, multi-path Global Trade Embodied Carbon Emission Network, and analyzing the inter-region relationship of global carbon emissions from multiple perspectives. The network analysis can link countries instead of looking at a country’s carbon emission in isolation, therefore can more objectively observe the trajectory of carbon dioxide flow in the network and analyze the position and connection of each node in the network, which is in line with the realistic carbon linkage characteristics and provides a new research perspective and research method for global trade embodied carbon research at the same time. Finally, by exploring the specific influencing factors of the global trade embodied carbon network, it can provide solutions for reducing the embodied carbon emissions of trade, help countries to form a more consistent multilateral climate agreement, and balance the relationship between economic development and carbon emissions. While achieving high-quality economic development, we reduce carbon emissions and carbon leakage, and actively build a community with a shared future for mankind.
This paper makes changes on the basis of the multi-regional input-output model, and integrates the characteristics of each country’s own carbon emissions in the basic form of the input-output table to construct an international carbon emissions matrix. Then, the network analysis method is used to reveal the global characteristics of the global trade implied carbon network, and the network analysis is consistent with the characteristics of carbon emissions in reality. At the same time, the multi-regional input-output model and network analysis are consistent. In addition to clarifying the internal structure and framework of the entire network, the combination of the two can also reveal the status of each economy in the network and the connections between them. Finally, there is currently no literature that deeply explores the specific factors that affect the embodied carbon emissions network. This paper uses the QAP analysis method to explain the influencing factors of the embodied carbon emission network, so as to provide solutions for reducing the embodied carbon emissions of trade and formulating carbon emission reduction policies for the country.
We organize the remainder of the article as follows: In Section 2, we introduce the data source used and build a trade embodied carbon network. In Section 3, we analyze its evolution trend and characteristics of global trade embodied carbon network. In Section 4, we use the QAP (Quadratic Assignment Procedure) analysis method to deconstruct the influencing factors of the global trade embodied carbon network. In Section 5, we discuss the similarities and differences between the conclusions of this paper and those of previous literature, summarize the innovations and shortcomings of this paper, and discuss future research directions in this field. In Section 6, we present the conclusions and policy suggestions.
2 Methodology and Data
2.1 Data Source
\This study uses the data of a multiregional input-output (MRIO) system, the World Input-Output Database (WIOD). It is established based on the officially available data published by statistical agencies, which covers countries and regions accounting for about 85% of the global GDP with high reliability and wide representation. Therefore, this paper selects the WIOD database to analyze the structural evolution of the Global Trade Embodied Carbon Network. Based on the availability and reliability of the data, this paper uses the 2016 released version of the world input-output table, which contains the input-output data of 28 EU countries and 14 other major countries in the world from 2000 to 2014, and the rest of the countries and regions are grouped into ROW region. In addition, this paper has combined 28 EU countries as the whole EU region data for analysis, which can avoid the impact on the subsequent analysis due to missing data of some EU countries. The basic form of the input-output table is shown in Table 1. It is assumed that there is a total of N countries in the world (i, j = 1, 2, …, N), each with M industries. Zij denotes the made-in-country i intermediate inputs used by country j, Fij denotes the made-in-country i final goods used by country j, Wi′ denotes the value-added in country i, and Xi denotes the total output of country i.
The multiregional input-output table can be expressed in matrix form as:
In Eq. 1, X denotes the total output matrix of country i, A denotes the matrix of direct consumption coefficients from country j to country i, and F denotes the final demand column vector.
To measure the carbon emission of a country, we use the indicator of CO2 emissions (kt) from the WDI(World Development Indicators) of the World Bank. The combination of world input-output tables and inter-country carbon emissions data enables the measurement of inter-country carbon emissions and carbon transfers. With countries defined as nodes, the global carbon emission model is constructed as follows:
Among them,
2.2 Indices
Based on establishing a multiregional carbon emission matrix, this paper analyses the position and role of 15 major economies in the Global Trade Embodied Carbon Network by using the Social Network Analysis (SNA). This paper firstly analyzes the structural characteristics of the international carbon emission network from the perspective of the centerline that characterizes the individual location, the network density, centrality, and related indicators of the network will be calculated.
Network density refers to the ratio of the number of relationships in the actual network to the maximum possible number of relationships and reflects the closeness of the connections between the nodes in a network. The specific formula is shown in Eq. 3. wherein D represents the network density, M represents the number of relationships present in the actual network, and N represents the number of nodes in the network.
Centrality is applied to analyze the importance of a node in the network with higher centrality meaning that a country is more central in the network. Centrality is generally classified into three types, namely Degree Centrality, Betweenness Centrality, and Closeness Centrality.
The formula for calculating Degree Centrality is shown in Eq. 4. Wherein DCi denotes the degree centrality of country i, and
2.3 Models
In the method of SNA, QAP is commonly used to analyze the influencing factors of a network, and estimate the relation among matrices. The process of QAP analysis includes correlation analysis and regression analysis. Correlation analysis is to study the correlation between two “relationship matrices”, to explain the influence of one relationship on another, while regression analysis is to study the regression relationship between one matrix and multiple relationship matrices. For relational data, it is difficult to use the general least square method and other conventional statistical test methods, because these test methods have relatively strict assumptions, and the nodes in the network are connected, indicating that there is a direct or indirect dependence between nodes, which does not conform to the independent and identically distributed hypothesis. At the same time, there is also a correlation between various independent variables. If the conventional test method is used, the multicollinearity problem will occur, leading to the increase of variance of parameter estimates, and the significance suggestion of variables will lose significance. Therefore, the QAP analysis method is adopted in this paper. QAP analysis method has no requirement on whether independent variables are independent of each other, which makes it more robust in dealing with relational data. QAP analysis is mainly divided into two steps. First, the correlation between dependent variables and independent variables is determined by QAP analysis, and independent variables with no significant correlation are eliminated. Then, the standardized regression coefficients of each variable matrix are determined by QAP regression analysis.
The existing research on the influencing factors of the carbon emission relationship between countries is mainly based on the following aspects. 1) Population and consumption. Population is a factor that affects carbon dioxide emissions. With the increase of world population, carbon dioxide emissions will also increase. Li et al. employed the pooled mean group estimator under the framework of the panel autoregressive distributed lag model, it was found that population size and population density could increase CO2 emissions (Li et al., 2022). At the same time, a series of activities due to people’s consumption will also produce a large amount of carbon dioxide emissions (Munksgaard and Pedersen, 2001). Lenzen analyzed the causes of greenhouse gas emissions in Australia from the perspective of final consumption, and the results showed that the consumption of goods and services contributed to 49% of carbon dioxide emissions (Lenzen, 1998); 2) The impact of bilateral trade and trade agreements on carbon emissions. A part of carbon emissions will be transferred to other countries along with international trade or international investment, so trade between countries and trade agreements signed will have an important impact on the generation and transfer of carbon emissions. Dou et al. analyzed the impact of the signing of the China-Japan-Korea trade agreement on regional carbon emissions. By comparing the carbon emissions before and after the signing of the agreement, it was found that trade openness has a positive impact on the greenhouse effect. Expanding trade openness not only directly affects carbon emissions directly, but also has indirect impacts by affecting three main effects (i. e., scale effect, technical effect, and structure effect) (Dou et al., 2021); 3) The impact of geographic distance on carbon emissions. The distance between countries will affect the scale of trade between two countries. The closer the two countries are, the lower the transportation cost for their trade, and the greater the possibility of trade (Chaney. 2011). while trade has a promoting effect on carbon emissions; 4) The impact of fuel consumption on carbon emissions, the combustion of solid fuels can produce a large amount of carbon dioxide emissions, Hanif studied the impact of solid fuel, fossil fuel and renewable energy consumption on environmental degradation in developing economies in sub-Saharan Africa, The results show that the consumption of fossil and solid fuels greatly increases carbon dioxide emissions on the one hand and air pollution on the other (Hanif, 2018).
Based on the above analysis, in this paper, population (POP), trade volume between countries (BT), final consumption (FC), and carbon dioxide emissions from solid fuel consumption (SFC) were selected as explanatory variables. The data of these variables were obtained from the WDI database and United Nations Merchandise Trade Statistics Database. In addition, geographical distance (GI) between countries and whether they belong to the same regional trade agreement (RTA) are also important factors affecting the evolution of the carbon network embodied in global trade. Therefore, the model is as follows.
In Eq. 8, the explained variable (P) is the international carbon emission matrix calculated by Eq. 2. Pairwise difference values of POP, BT, FC, and SFC data are carried out, and then absolute values are taken to form the variable matrix. GI is constructed as follows: if two countries belong to the same continent, the corresponding element is 1, otherwise, 0, and a binary symmetric matrix is finally formed. The construction method of RTA is similar to that of GI, that is, if there is a trade agreement between two countries, the corresponding element is taken as 1, otherwise, 0, and a binary symmetric matrix is finally formed. Through QAP correlation analysis on the matrix of explained variables and each matrix of explaining variables, the explaining variables with no significant correlation were eliminated. Then QAP regression analysis was carried out on the explained variable matrix and significant explaining variable matrix, and the corresponding regression coefficients were obtained.
3 Evolution of Global Trade Embodied Carbon Network
3.1 Nodes Position of the Network
Each country works as a node of carbon emissions in and out via international trade with other countries/regions. To calculate how many other nodes transfer carbon emissions in to or out of a node, we use the in-degree and out-degree to measure the flow. The flow refers to the corresponding value in the carbon emission matrix C. In this paper, 15 major economies in the world are selected and classified according to developed, developing, and emerging economy countries. In order to analyze the structural characteristics of the Network, the Global Trade Embodied Carbon Network needs to be binarized, and then the network density of 15 economies, the point-out degree, point-in degree, and three centrality indicators of each country are calculated according to Eqs 3–7.
By analyzing the point-out and point-in degrees of each economy in Table 2, it can be found that the point-in degree was generally higher in developed countries, such as the EU, the United States, Japan, Canada, etc., while the point-out degree was higher in countries such as China, Russia, India, etc. This is mainly due to the high demand for raw materials, primary products, and energy resources in the United States, EU, Japan, etc., which requires the import of labor-intensive products from developing countries, thus creating a carbon emission linkage with foreign countries. In contrast to developed countries, developing countries such as China, Russia, and India are rich in labor and more abundant in resources, so they can produce a large amount of basic or intermediate products, of which some are used to meet domestic demand and some are exported to other countries, receiving carbon input from foreign countries while exporting their products, therefore their point-out degree was higher. By analyzing the changes of the point-out and point-in degrees from 2000 to 2014, it can be found that the point-out degree of most developed countries was increasing, so was the point-in degree of developing countries, because developing countries and emerging economies are gradually increasing the economic linkages with other countries as their economies develop, and the final products produced by developed countries will flow through trade to various countries. In addition, Table 2 shows that China, the EU, and the United States had larger point-out and point-in degrees, mainly because of their large market size and rapid economic growth, which generates a large number of economic linkages with other countries through trade in intermediate goods and processing trade, thus establishing carbon emission linkages.
Comparing the in-degree in 2000 and 2014 in Figure 1A, we can find the carbon emission transferred into BRIC countries has increased in the past decades, as emerging markets are the main exporters of international trade. And comparing the out-degree in 2000 and 2014 in Figure 1B, we can find that the carbon emission outflow from Asian countries has increased, as their economic growth and import grew faster in the past decade. In addition, most of the countries in Figure 1 are above the 45° line, indicating the increase in the centrality of countries in the embodied carbon emissions network. Generally, China is the main actor in both inflow and outflow as it is the transfer station between developing countries and developed countries in international trade, while trade embodied carbon emission is transferring out more from developed countries and flowing into developing countries.
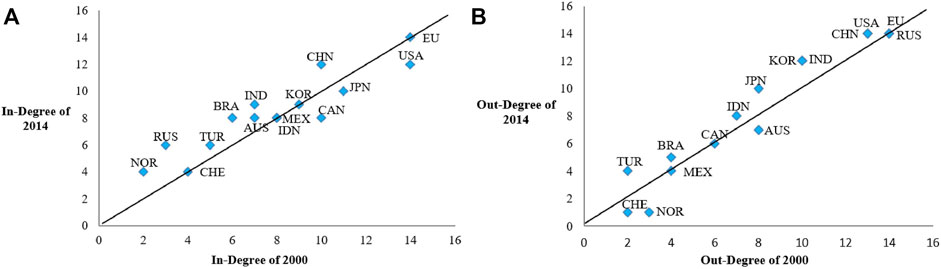
FIGURE 1. Change of In-Degree and Out-Degree of main economies. (A) In-Degree of main economies in 2000 and 2014. (B) Out-Degree of main economies in 2000 and 2014.
3.2 Centrality of the Network
In addition to the changes in point-out and point-in degrees for each country, this paper also calculates the changes in the three centrality indicators for the period 2000–2014, and its results are shown in Table 3.
Degree centrality measures the number of countries where a country has direct carbon linkages with other countries. As seen from Table 3, the degree centrality of most countries increased significantly, such as Brazil, China, India, Russian Federation, Turkey, and other countries. But some countries, with their degree centrality not too high due to the limitation of economic volume or geographical location, were located at the edge of the Network. In developed countries or regions like the EU, Australia, Japan, etc., the degree centrality did not increase significantly, with only a slight increase or without changes. While some developed countries saw a decrease in degree centrality, such as the United States, Canada, Switzerland, etc. This may be related to the high economic growth of China, India, and other countries. With the rapid development of emerging economies and its advantage of low labor costs, some industries have been transferred from developed countries to developing countries and emerging economies, thus generating carbon emission linkages with other countries and further increasing network density. In addition, the EU, China, and the United States were the top three countries in terms of degree centrality, largely contributed by their own huge trade volume. These three countries have established carbon emission linkages with most countries in the world through international trade, contributing closer carbon emission linkages by the increase of trading volume, therefore, they were at the center of the Global Trade Embodied Carbon Network.
The betweenness centrality of developed countries and regions such as Canada, Japan, the United States, and the European Union decreased to varying degrees, among which the United States experienced the biggest decrease, reaching 15.026. This is contributed by the fact that as globalization further deepens and an increasing number of countries are participating in the division of labor and trade, the position of developed countries in the Global Trade Embodied Carbon Network has been somewhat weakened. In contrast to such decline in developed countries, the betweenness centrality of most developing countries or emerging economies experienced an increasing trend, among which China, Russian Federation, and India were the ones with a faster increase. These countries, with their rapid economic development, have broken the trade barriers set by developed countries and weakened the central position of developed countries in the Global Trade Embodied Carbon Network. In addition, other economies such as the EU, China, United States, Russia had higher betweenness centrality, which reflects their ability to regulate and control the production and carbon emission linkage of other countries, indicating that the global trade and carbon emission linkage had more paths through these countries. And the total carbon emission generated through these countries had a higher proportion in the total global carbon emission, as a consequence, they were in a higher position in the Global Trade Embodied Carbon Network.
Unlike betweenness centrality, most developing countries or emerging economies showed a decreasing trend in closeness centrality, such as China, India, Russian Federation, Brazil, etc. Such decrease indicates that these developing countries have a shorter path to obtain carbon emission linkages with other countries, to be specific, it is easier to obtain carbon emission linkages between countries, and the carbon emissions are more likely to be transferred from the center of the Network to more peripherally located countries in response to international trade. It can also be seen from Table 3 that the economies with lower closeness centrality were mainly European Union, China, United States, Russian Federation, etc, indicating that the above countries had shorter paths to carbon emission linkages with other countries and passed through fewer countries, so they were at the center of the Global Trade Embodied Carbon Network.
3.3 Overall Evolution of the Network
Figure 2 shows the Global Trade Embodied Carbon Unweighted Networks in 2000 and 2014, and Figure 3 shows the Weighted Networks of Embodied Carbon in Global Trade in 2000 and 2014. As the Global Trade Embodied Carbon Unweighted Networks indicate, the size of the whole Network was expanding and the carbon emission linkages between countries were gradually becoming stronger. In the year 2000, some countries did not have direct carbon linkages therefore needed a third country to act as a “bridge” between them. Afterwards with the developed economy and the increased global carbon emissions, two countries that needed a third country to make carbon linkages before 2014 could now trade carbon emissions directly, such as South Korea and Turkey, Indonesia and Brazil, etc. It can also be observed that countries with larger economies were more central in the overall network, with a relatively stable position and the overall structure of the network.
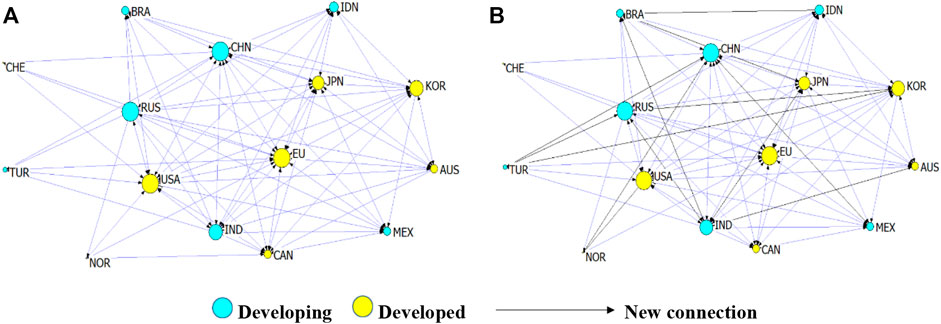
FIGURE 2. Comparison of unweighted networks of global trade embodied carbon in 2000 and 2014. (A) Network in 2000. (B) Network in 2014.
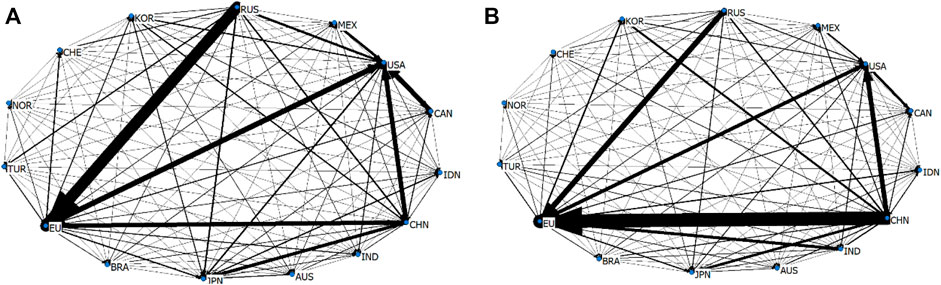
FIGURE 3. Comparison of weighted networks of global trade embodied carbon in 2000 and 2014. (A) Network in 2000. (B) Network in 2014.
Figure 3 about the Weighted Networks of Embodied Carbon in Global Trade visualizes the magnitude of carbon flows on the basis of Figure 2. To be specific, the most significant export carbon flows in 2000 were from Russia to the EU, followed by the export carbon flows from the United States to the EU. The year of 2014 witnessed the changes in the export carbon flows, as the most significant export carbon flows were from China to the EU, followed by the export carbon flows from Russia to the EU. It can be seen that the carbon flow-out and carbon flow-in centering China was more closely related in 2014 compared to 2000, and the carbon flows with major economies such as European countries, the United States and Japan were more significant.
4 Influencing Factors of the Network
4.1 QAP Correlation Analysis
QAP correlation analysis can test the correlation between global trade implied carbon network and selected explanatory variables. UCINET software is used for correlation analysis, and the correlation analysis results obtained through 5,000 random substitutions are shown in Table 4. The Obs Value column in Table 4 is calculated according to the international carbon emission matrix and the matrix of other explanatory variables. The Minimum column and Maximum column in Table 4 are the Minimum and Maximum of the correlation coefficient of 5,000 times of random displacement. p ≥ 0 and p ≤ 0 represent the probability that the correlation coefficient calculated by random permutation is greater than or less than the actual correlation coefficient Table 5.
The results of correlation analysis in 2000 show that population, trade volume between countries, final consumption, carbon dioxide emissions from solid fuel consumption, and geographical distance all significantly affect the formation of the global carbon emission relationship. Among them, trade volume between countries, final consumption, and carbon dioxide emissions from solid fuel consumption are both significant at the 1% level, population and geographical distance are significant at the 10% level. It shows that the increase of population, trade volume between countries, final consumption and carbon dioxide emissions from solid fuel consumption and the two countries in the same continent will play a role in promoting international carbon emissions.
From the results of correlation analysis in Table 5, it can be seen that population, trade volume between countries, final consumption, carbon dioxide emissions from solid fuel consumption, geographical distance, and trade agreements all significantly affect the formation of the global carbon emission relationship. The correlation coefficient between international carbon emission matrix P and POP is 0.323, indicating that population increase will promote the development of the global carbon emission relationship. The correlation coefficient between carbon emission matrix P and BT is 0.372, indicating that trade between countries plays a very important role in the carbon connection between countries, because most carbon emissions are transferred from one country to another through commodity trade. The correlation coefficients of international carbon emission matrix P, FC and SFC are 0.469 and 0.345 respectively, indicating that national final consumption and carbon dioxide emissions from solid fuel consumption will also have a positive impact on carbon emissions. The correlation coefficient between P and GI of international carbon emission matrix is 0.258, indicating that geographical distance also has an impact on international carbon emission to a certain extent. Countries in the same continent are bound to have more economic ties due to the convenience of transportation, so carbon emission correlation will increase accordingly. Finally, the correlation coefficient between carbon emission matrix P and RTA is 0.205, indicating that trade agreements are conducive to the development of bilateral trade, and increased trade between countries will make carbon emissions more closely correlated. Therefore, the above factors have promoted the development of a carbon implicit network in global trade to a certain extent.
The QAP correlation analysis results in 2000 and 2014 show that RTAs were not significantly indigenous to the international carbon emission matrix P in 2000, but became significantly indigenous in 2014. It may be because there were relatively few trade agreements signed between countries in 2000, and the impact on international carbon transfer was minimal. However, with the increase of trade agreements signed, trade between countries is growing, and the resulting carbon transfer is also increasing. So as of 2014, the signing of the trade agreement has had a significant positive impact on the international carbon emission matrix. In addition, the correlation coefficients of POP, BT, GI and RTA to the international carbon emission matrix P have increased to varying degrees, while the correlation coefficients of FC and SFC to the international carbon emission matrix P show a downward trend, which shows that POP, BT, GI, and RTA are becoming more and more important for the impact of global trade embodied carbon emissions. Although the correlation coefficient of FC and SFC to the international carbon emission matrix P is declining, its own coefficient is larger than other variables, so FC and SFC also play an important role in the embodied carbon emissions of global trade.
4.2 Correlation Analysis Among Explanatory Variables
Through QAP correlation analysis of the explanatory variable matrix, it is found that there is a high correlation between the two matrices of population (POP) and solid fuel consumption carbon dioxide emission (SFC) (as shown in Table 6). Therefore, in order to avoid the problem of multicollinearity, this paper conducts regression analysis on the relationship between final consumption, trade between countries, carbon dioxide emissions of solid fuel consumption, geographical distance, and carbon emissions implied by trade agreements and global trade. Similar to correlation analysis, 5,000 random substitutions are selected. Table 7
4.3 Result of QAP Regression
The results of QAP regression analysis in 2000 are shown in Table 7. According to QAP regression analysis, R2 in 2000 is 0.306, and adjusted R2 is 0.293, indicating that the five explanatory variables can explain 29.3% of the variation of the international carbon emission matrix relationship. Among the five explanatory variables, only GI has passed the 5% significance test, indicating that when other factors are taken into account, geographical distance has a significant indigenous impact on international carbon emission relations, that is, the carbon emission relations between countries on the same continent are relatively close.
The results of QAP regression analysis in 2014 are shown in Table 8. According to QAP regression analysis, R2 in 2014 is 0.434, and adjusted R2 is 0.423, indicating that the five explanatory variables can explain 42.3% of the variation of the international carbon emission matrix relationship. Table 8 shows the regression coefficient of the explanatory variable matrix. It can be seen that the standardized regression coefficient of FC is 0.330, which passes the significance test of 10%, indicating that FC still affects the international carbon emission relationship when other influencing factors are considered. The standardized regression coefficient of BT was 0.123, which passed the significance level test of 5%, indicating that when considering other factors, the closer the trade between countries, the closer the relationship between international carbon emissions will be. SFC standardized regression coefficient was 0.165, through the test of significance of 1%, carbon dioxide emissions of solid fuel consumption have a significantly positive influence. The standardized regression coefficients of GI and RTA were 0.203 and 0.160, respectively, which both passed the significance test of 5%, indicating that geographical distance and trade agreement also have a significant impact on international carbon emission relationship when other factors are considered. Closely connection among countries via RTA will enhance the relation of trade embodied carbon emission network.
The results of the QAP regression analysis in 2000 could only explain 29.3% of the variation of the international carbon emission matrix, but R2 in 2014 is 0.434, and adjusted R2 is 0.423, indicating that the five explanatory variables have an effect on the international carbon emission matrix. The explanatory nature of relationship variation is further enhanced. At the same time, considering the influence of other factors, each variable has a significant impact on the international carbon emission relationship.
5 Discussion
The results of this paper show that the scale of the embodied carbon network in trade is expanding, the links between countries have become closer, and the carbon emissions generated through international trade, international investment and other activities are also more active. And developed countries are net importers of embodied carbon, while developing countries are mainly net exporters of embodied carbon, which is consistent with previous research (Sun et al., 2019; Deng et al., 2021; Kim and Tromp, 2021; Mangr and Ahin, 2022; Wu et al., 2022; Wu et al., 2022). Compared with previous studies, this paper combines the unweighted network and the weighted network to explore the evolution of global trade embodied carbon emission network, and conducts a new deconstruction of the influencing factors. It was found that there are some differences in the impact of some factors on carbon emissions from previous studies, such as the impact of trade agreements on the trade implied carbon network. The conclusions drawn in this paper are different from some literature. Dou et al. found through research that China, Japan and South Korea can reduce the embodied carbon emissions in the process of trade by signing trade agreements. They believe that the signing of trade agreements will produce technology spillover effects (Dou et al., 2021). This paper argues that the signing of trade agreements has played a role in promoting trade embodied carbon, because the technological development levels of some countries that signed trade agreements in this paper are quite different, and the technological spillover effect of international investment is actually not obvious. Instead, the signing of trade agreements will expand the scale of trade between countries, resulting in more embodied carbon emissions.
In addition, this paper has some data limitations in the empirical research. For example, the construction of the world input-output table requires a lot of effort. We use the data from 2000 to 2014 and a limited number of regions and economies in the empirical research, empirical studies using up-to-date and detailed regions are needed in future research. In addition, the measurement of embodied carbon in processing export trade may be a trend in the future development of this field, because few scholars have been involved in this issue at present, but processing export trade accounts for a larger share of emerging economies' trade, analyzing the embodied carbon of processing export trade can more reasonably distinguish domestic and foreign carbon emissions, which is helpful to divide the carbon emissions responsibility of each country.
6 Conclusion and Implications
6.1 Main Conclusion
This paper analyzes the characteristics and evolutionary trend of the Global Trade Embodied Carbon Network by constructing a global carbon emission matrix and use QAP method to find the influencing factors of the network. The main conclusions drawn are as follows.
Firstly, seen from the overall Network, a small number of countries generate more carbon emissions, among which China is gradually at the center of the global carbon emissions network, and the United States and the European Union are always in a more stable position and at the center of the Global Trade Embodied Carbon Network. At the same time, with the increase of economic links between countries, the carbon emission linkages of individual countries become closer and more complex, and the size of the Global Trade Embodied Carbon Network is also increasing.
Secondly, in the Global Trade Embodied Carbon Network, developed countries transfer carbon through the import of basic raw materials and intermediate products, while developing countries and emerging economies receive carbon inputs from developed countries while exporting products, resulting in a higher point-in degree of developed countries and a higher point-out degree of developing countries. In addition, the point-in degree of most developing countries is significantly higher than their point-out degree, which indicates that they mainly participate in the Global Trade Embodied Carbon Network through the form of supply rather than consumption. The centrality analysis reveals that the position of developed countries in the network is relatively stable, and the degree centrality and betweenness centrality of developing countries have increased.
Thirdly, the QAP regression results showed that population, economic scale, solid fuel consumption and regional trade agreements, etc. are the main factors that boost the international trade embodied carbon emission network. International cooperation relationship in energy, consumption, trade and other fields can affect the carbon emission transfer network. So joint response to climate change is not isolated from international cooperation in other disciplines.
6.2 Implications
Based on the above analysis, we believe that when make global carbon emission reduction plans, it is important to fully consider the characteristics and relationships of countries in the global trade network, and formulate appropriate emission reduction strategies according to the different conditions of each country. In terms of consumption of goods and services, the government can encourage increased investment in research and development of cleaner production methods, and encourage relevant enterprises to improve cleaner production technologies through subsidies, so as to reduce the carbon content per unit product. Besides, trade opening has a positive impact on a country’s carbon emissions, which means that some countries transfer the production of products with relatively high carbon content to their own countries, so carbon taxes can be imposed on the export of highly polluting products. In addition, subsidies can be given to companies that produce highly polluting products to encourage them to transform. Third, change the energy structure. At present, most countries in the world are still dominated by fossil fuels and solid fuels, which will increase the generation of carbon emissions. Therefore, for countries with relatively advanced technologies, the consumption of clean energy such as solar energy and wind energy should be gradually increased, and the consumption of solid fuels and fossil fuels should be reduced. At the same time, appropriate assistance will be given to countries with immature technologies to help them upgrade their energy consumption structure.
Finally, all countries are closely connected in the world, therefore, we should strengthen multilateral cooperation, jointly build relevant international laws and low-carbon agreements, promote fair and mutually beneficial international trade rules, reduce the constraints of carbon emission reduction on the economic development of developing countries, and ensure the fair distribution of carbon emission reduction responsibilities among countries. At the same time, according the network analysis, carbon emission is transforming from developed countries to developing countries via international trade, appropriate assistance should be given to developing countries with low technologies to help them upgrade their production pattern.
Data Availability Statement
The original contributions presented in the study are included in the article/Supplementary Material, further inquiries can be directed to the corresponding author.
Author Contributions
All authors listed have made a substantial, direct, and intellectual contribution to the work and approved it for publication.
Funding
Major Projects of the National Social Science Foundation of China (21&ZD151); Key Project of Beijing Municipal Education Commission of Education, China (No. SZ202110011006).
Conflict of Interest
The authors declare that the research was conducted in the absence of any commercial or financial relationships that could be construed as a potential conflict of interest.
Publisher’s Note
All claims expressed in this article are solely those of the authors and do not necessarily represent those of their affiliated organizations, or those of the publisher, the editors and the reviewers. Any product that may be evaluated in this article, or claim that may be made by its manufacturer, is not guaranteed or endorsed by the publisher.
References
Antweiler, W., Copeland, B. R., and Taylor, M. S. (2001). Is Free Trade Good for the Environment? Am. Econ. Rev. 91 (4), 877–908. doi:10.1257/aer.91.4.877
Baloch, M. A., and Danish, fnm (2022). CO2 Emissions in BRICS Countries: what Role Can Environmental Regulation and Financial Development Play? Clim. Change 172, 9. doi:10.1007/s10584-022-03362-7
Chaney, T. (2011). The Network Structure of International Trade. Am. Econ. Rev. 104 (1135), 3600–3634. doi:10.3386/w16753
Dawkins, E., Moran, D., Palm, V., Wood, R., and Björk, I. (2019). The Swedish Footprint: a Multi-Model Comparison. J. Clean. Prod. 209 (FEB.1), 1578–1592. doi:10.1016/j.jclepro.2018.11.023
Deng, G., Lu, F., and Yue, X. (2021). Research on china's Embodied Carbon Import and Export Trade from the Perspective of Value-Added Trade. PLoS ONE 16 (11), e0258902. doi:10.1371/journal.pone.0258902
Dong, D., An, H., and Huang, S. (2017). The Transfer of Embodied Carbon in Copper International Trade: An Industry Chain Perspective. Resour. Policy 52, 173–180. doi:10.1016/j.resourpol.2017.02.009
Dou, Y., Zhao, J., Malik, M. N., and Dong, K. (2021). Assessing the Impact of Trade Openness on CO2 Emissions: Evidence from china-japan-rok Fta Countries. J. Environ. Manag. 296, 113241. doi:10.1016/j.jenvman.2021.113241
Fan, J. L., Zhang, X., Wang, J. D., and Wang, Q. (2019). Measuring the Impacts of International Trade on Carbon Emissions Intensity: A Global Value Chain Perspective. Emerg. Mark. Finance Trade 57, 1–17. doi:10.1080/1540496x.2019.1662783
Ferng, J. J. (2003). Allocating the Responsibility of CO2 Over-emissions from the Perspectives of Benefit Principle and Ecological Deficit. Ecol. Econ. 46, 121. doi:10.1016/s0921-8009(03)00104-6
Gao, Z., Geng, Y., Wu, R., Zhang, X., Pan, H., and Jiang, H. (2020). China's CO2 Emissions Embodied in Fixed Capital Formation and its Spatial Distribution. Environ. Sci. Pollut. Res. 27 (16), 19970–19990. doi:10.1007/s11356-020-08491-z
Green, F. (2015). Nationally Self-Interested Climate Change Mitigation: A Unified Conceptual Framework. UK: Grantham Research Institute on Climate Change and the Environment Leeds.
Han, M., Yao, Q., Liu, W., and Dunford, M. (2018). Tracking Embodied Carbon Flows in the Belt and Road Regions. J. Geogr. Sci. 28 (9), 1263–1274. doi:10.1007/s11442-018-1524-7
Hanif, I. (2018). Impact of Economic Growth, Nonrenewable and Renewable Energy Consumption, and Urbanization on Carbon Emissions in Sub-saharan Africa. Environ. Sci. Pollut. Res. 25 (15), 15057–15067. doi:10.1007/s11356-018-1753-4
Jakob, M. (2021). Climate Policy and International Trade - A Critical Appraisal of the Literature. Energy Policy 156 (1), 112399. doi:10.1016/j.enpol.2021.112399
Jin, X., Luo, L., and Chen, X. (2013). City Carbon Dioxide Emission Calculation for Low Carbon Development Strategies. Proceeding of the International Conference on Frontiers of Environment Energy and Bioscience (ICFEEB 2013), December 2013.
Kim, T. J., and Tromp, N. (2021). Analysis of Carbon Emissions Embodied in south korea's International Trade: Production-Based and Consumption-Based Perspectives. J. Clean. Prod. 320, 128839. doi:10.1016/j.jclepro.2021.128839
King, L. C., and van den Bergh, J. C. J. M. (2021). Potential Carbon Leakage under the Paris Agreement. Clim. Change 165, 1. doi:10.1007/s10584-021-03082-4
Kuik, O., and Hofkes, M. (2010). Border Adjustment for European Emissions Trading: Competitiveness and Carbon Leakage. Energy Policy 38 (4), 1741–1748. doi:10.1016/j.enpol.2009.11.048
Lenzen, M. (1998). Primary Energy and Greenhouse Gases Embodied in Australian Final Consumption: an Input-Output Analysis. Energy Policy 26 (6), 495–506. doi:10.1016/s0301-4215(98)00012-3
Li, Z., Zhou, Y., and Zhang, C. (2022). The Impact of Population Factors and Low-Carbon Innovation on Carbon Dioxide Emissions: a Chinese City Perspective. Environ. Sci. Pollut. Res. doi:10.1007/S11356-022-20671-7
Luo, F., Wang, H. C., and Zhong, Y. D. (2014). Tourists' Transportation Carbon Footprint Spatial Distribution. Popul. Resour. Environ. China 024 (002), 38–46. doi:10.3969/j.issn.1002-2104.2014.02.006
Machado, G., Schaeffer, R., and Worrell, E. (2001). Energy and Carbon Embodied in the International Trade of Brazil: an Input-Output Approach. Ecol. Econ. 39 (3), 409–424. doi:10.1016/s0921-8009(01)00230-0
Mangr, N., and Ahin, L. A. (2022). An Environmentally Extended Global Multi-Regional Input–Output Analysis of Consumption-Based and Embodied Import-Based Carbon Emissions of turkey. Environ. Sci. Pollut. Res. Int. 20, 1–14. doi:10.1007/s11356-022-19290-z
Munksgaard, J., and Pedersen, K. A. (2001). CO2 Accounts for Open Economies: Producer or Consumer Responsibility? Energy Policy 29, 327. doi:10.1016/S0301-4215(00)00120-8
Pan, W., Teng, Y., Li, K., and Yu, C. (2018). Implications of Prefabrication for the Life Cycle Carbon Emissions of High-Rise Buildings in High-Density Urban Environment. Constr. Res. Congr. 2018. doi:10.1061/9780784481301.049
Rosenbaum, R. K., Huijbregts, M. A. J., Henderson, A. D., Margni, M., Mckone, T. E., van de Meent, D., et al. (2011). USEtox Human Exposure and Toxicity Factors for Comparative Assessment of Toxic Emissions in Life Cycle Analysis: Sensitivity to Key Chemical Properties. Int. J. Life Cycle Assess. 16 (8), 710–727. doi:10.1007/s11367-011-0316-4
Sánchez-Chóliz, J., and Duarte, R. (2004). CO2 Emissions Embodied in International Trade: Evidence for spain. Energy Policy 32 (18), 1999–2005. doi:10.1016/s0301-4215(03)00199-x
Sun, C., Chen, L., and Huang, G. (2019). Decomposition Analysis of CO2 Emissions Embodied in the International Trade of russia. Sustainability 12 (1), 323. doi:10.3390/su12010323
Tian, J., Liao, H., and Wang, C. (2014). Spatial-Temporal Variations of Embodied Carbon Emission in Global Trade Flows: 41 Economies and 35 Sectors. CEEP-BIT Work. Pap. 78, 1125. doi:10.1007/s11069-015-1761-3
Turner, J. M. (2014). Counting Carbon: The Politics of Carbon Footprints and Climate Governance from the Individual to the Global. Glob. Environ. Polit. 14 (1), 59–78. doi:10.1162/glep_a_00214
Villarino, S. H., Studdert, G. A., Laterra, P., and Cendoya, M. G. (2014). Agricultural Impact on Soil Organic Carbon Content: Testing the IPCC Carbon Accounting Method for Evaluations at County Scale. Agric. Ecosyst. Environ. 185, 118–132. doi:10.1016/j.agee.2013.12.021
Winjum, J. K., Sandra, B., and Schlamadinger, B. (1998). Forest Harvests and Wood Products: Sources and Sinks of Atmospheric Carbon Dioxide. For. Sci. 44 (2), 272. doi:10.1093/forestscience/44.2.272
Wu, L., Zhou, Y., Qian, H., Ang, B., Bachmeier, L., Sadorsky, P., et al. (2022). Global Actions under the Paris Agreement: Tracing the Carbon Leakage Flow and Pursuing Countermeasures. Energy Econ. 106, 105804. doi:10.1016/j.eneco.2021.105804
Yu, B., Zhao, Q., and Wei, Y.-M. (2021). Review of Carbon Leakage under Regionally Differentiated Climate Policies. Sci. Total Environ. 782, 146765. doi:10.1016/j.scitotenv.2021.146765
Keywords: global trade embodied carbon emission, carbon footprint, social network analysis, environment policy, economic development, environmental emissions, sustainable development, input-output table
Citation: Di Y, Cao J, Guo Z and Khan MK (2022) Network Evolution and Influencing Factors of Global Trade Embodied Carbon Emission. Front. Environ. Sci. 10:943939. doi: 10.3389/fenvs.2022.943939
Received: 14 May 2022; Accepted: 17 June 2022;
Published: 07 July 2022.
Edited by:
Ehsan Elahi, Shandong University of Technology, ChinaReviewed by:
Umair Akram, RMIT University, VietnamShuo Zhang, China University of Political Science and Law, China
Xinru Han, Institute of Agricultural Economics and Development (CAAS), China
Copyright © 2022 Di, Cao, Guo and Khan. This is an open-access article distributed under the terms of the Creative Commons Attribution License (CC BY). The use, distribution or reproduction in other forums is permitted, provided the original author(s) and the copyright owner(s) are credited and that the original publication in this journal is cited, in accordance with accepted academic practice. No use, distribution or reproduction is permitted which does not comply with these terms.
*Correspondence: Zhichao Guo, Z3VvemhpY2hhb0B0aC5idGJ1LmVkdS5jbg==