- 1Key Laboratory of Crop and Livestock Integrated Farming, Ministry of Agriculture and Rural Affairs, Institute of Agricultural Resources and Environment, Jiangsu Academy of Agricultural Sciences, Nanjing, China
- 2Discipline Microbiology, School of Life Sciences, University of KwaZulu-Natal, Pietermaritzburg, South Africa
Using farmland to digest biogas slurry is an effective measure to overcome the bottleneck of sewage treatment in livestock and poultry farms. However, there is limited research on the soil adsorption characteristics of biogas slurry ammonium nitrogen (NH4+-N). In addition, the maximum adsorption capacity (Qm) of farm soil is unclear. In this study, three typical farmland tillage layer soils (silty loam, loam, and sandy loam) were used to analyze adsorption characteristics through adsorption kinetics experiments (adsorption for 0.25, 0.5, 1, 2, 4, 6, 12, 18, or 24 h with NH4+-N concentrations of 42.90 mg/L) and thermodynamic experiments (adsorption for 3 days with NH4+-N concentrations of 54.25, 88.66, 105.85, 133.71, 178.80, 273.54, and 542.87 mg/L). The Qm value was fitted by models, and its relationship with soil properties was discussed. The results showed the following: 1) the adsorption of biogas slurry NH4+-N by the three types of soils was a composite kinetic process that comprised two stages of rapid and slow reactions. Rapid adsorption predominantly occurred within 0–1 h, and the adsorption capacity accounted for 35.24%–43.55% of the total adsorption. The ExpAssoc equation produced a good fit for the adsorption kinetic behavior in the three soil types. 2) The equilibrium adsorption could be described by the Langmuir equation, the Freundlich equation, the PlPlatt model, and the Langevin model isotherm, among which the Langevin model had the best fit, with a coefficient of determination R2 close to 1. The theoretical saturated Qm fitting results of NH4+-N were 1038.41–1372.44 mg/kg in silty loam, 840.85–1157.60 mg/kg in loam, and 412.33–481.85 mg/kg in sandy loam. The optimal values were 1108.55, 874.86, and 448.35 mg/kg for silty loam, loam, and sandy loam, respectively. 3) The Qm value was significantly positively correlated with soil organic matter, total nitrogen, available phosphorus, available potassium, cation exchange capacity, and particle content of 0.02–0.002 mm (p < 0.01), but significantly negatively correlated with soil pH (p < 0.05). This study can provide a reference for the safe application of biogas slurry on farmland.
Introduction
Biogas slurry is the residual liquid substance produced by anaerobic fermentation in biogas engineering using biodegradable organic wastes, such as human and livestock manure or various agricultural and forestry wastes. It is produced together with methane, carbon dioxide, and other gases under certain conditions of water content, temperature, and the action of methane bacteria in closed containers (Han et al., 2014). Biogas production has become an important energy-saving and emission-reduction technology for the harmless treatment and energy utilization of livestock and poultry manure in China. According to recent estimates, the biogas slurry produced by the anaerobic fermentation of livestock and poultry manure is more than 1.3 billion tons each year (Lu et al., 2010; Zhu and Huang, 2010). Returning biogas slurry to farmland soil as fertilizer is an effective method of economical use (Bradford et al., 2008; Ning et al., 2019; Liu et al., 2020). However, unreasonable application will not only reduce the use efficiency of biogas slurry, but it will also cause the environmental pollution of farmland water and the reduction of crop yields (Gao et al., 2011; Chen et al., 2013; Wang et al., 2016). The safe utilization of biogas slurry resources is expected to become an increasingly important topic in agriculture, energy use, and environmental protection and thus urgently needs to be investigated.
Biogas slurry is rich in a variety of nutrients required for plant growth and development, such as nitrogen, phosphorus, potassium, copper, zinc, organic acids, hydrolases, and amino acids. The total nitrogen (TN) content of biogas slurry is 0.53–3.24 g/kg (Jin et al., 2011; Ni and Zhang, 2017). Many researchers have studied the use of biogas slurry nitrogen as a measurement parameter for farmland reuse and discussed the safe dosage for fertility enhancement, yield, quality improvement, and environmental emission reduction. For example, Wang et al. (2010) found that applying biogas slurry equivalent to 2–3 times the amount of chemical fertilizer could obtain a pepper yield similar to that of conventional chemical fertilizer treatment, as well as improve pepper quality, increase the contents of soil organic matter (SOM), TN, available nitrogen, phosphorus, and potassium, and save on fertilizer costs. Yang et al. (2017) showed that compared with chemical fertilizer, the application of an equal amount of biogas slurry nitrogen led to no significant differences in the rice yield, nitrogen utilization rate, or soil residual inorganic nitrogen, while the ammonia volatilization per unit rice yield was significantly reduced by 22.6%. Most of these studies were based on the nitrogen fertilizer required for crop growth and replaced chemical nitrogen fertilizer with different proportions of biogas slurry nitrogen, but they did not consider whether the applied biogas slurry nitrogen exceeded the maximum nitrogen adsorption capacity of the topsoil.
Ammonium nitrogen (NH4+-N) is the main component of biogas slurry nitrogen, accounting for 46.42%–92.86% of TN (Ham and DeSutter, 1999; Jin et al., 2012; Ni and Zhang, 2017). In addition to being consumed and retained by ammonia volatilization, biological absorption (Li X. et al., 2021), and soil adsorption, a considerable portion of NH4+-N over-applied to soil can leach into deep soil through nitrification or into the water table along with surface runoff, thus causing nitrogen pollution in groundwater and surface water (Kithome et al., 1998; Wang et al., 2016; He et al., 2021). A large number of studies reported that the nitrate content in the areas adjacent to concentrated animal feeding operations exceeded the safety standards, thereby creating a bottleneck for livestock and poultry feeding development (Ciravolo et al., 1979; Feinerman et al., 2004). Considering the environmental behavior of NH4+-N, adsorption is an important process that can block and delay the further migration and transformation of nitrogen in order to inhibit nitrogen loss (Li W. et al., 2021). Studies of the soil adsorption of NH4+-N have focused on the use of a single chemical ammonium salt solution to explore the adsorption mode, deduce the adsorption mechanism, and estimate the adsorption capacity, generally using NH4Cl solution (Xue et al., 1996; Li et al., 2009; Tian, 2011; Cong et al., 2017). Solutions of NH4NO3, (NH4)2SO4, and NH4H2PO4 have also been used (Dalal, 1975). Furthermore, the soils used in previous research were mostly vermiculitic-type clay loam, kaolinitic sandy soils, and some tropical soils (Dalal, 1975; Lumbanraja and Evangelou, 1994; Wang and Alva, 2000; Kumar and Kothiyal, 2011). There are few studies on the adsorption characteristics of ammonium nitrogen biogas slurry solutions co-existing with complex components (Kumar and Kothiyal, 2012; Zhao et al., 2013), and there is a lack of research on the adsorption characteristics of ammonium nitrogen from biogas slurry in common agricultural production land topsoil.
Studying the adsorption characteristics of biogas slurry ammonium nitrogen in typical farmland soils and predicting the maximum adsorption capacity of the cultivated layer soil are significant for guiding the safe digestion of biogas slurry in farmland. In this study, three types of common farmland soils were collected: silty loam, loam, and sandy loam. Through analyses of adsorption kinetics and adsorption thermodynamics, the adsorption characteristics of these soils for biogas slurry ammonium nitrogen were analyzed, kinetic models were fitted, and the relationships between soil physical and chemical properties and adsorption capacity were explored to provide a scientific basis for the safe application of biogas slurry in farmland and the prevention and control of water pollution.
Materials and Methods
Materials
Biogas slurry was collected from a large pig farm (Jiangsu Yangyu Ecological Agriculture Co., Ltd.) in Xinjie Town of Taixing City, China (Figure 1). The pig farm has 138 standardized brick-and-concrete pens with a structure area of more than 100,000 m2. The farm produces around 120,000 commercial pigs annually. It has sewage collection tanks of 1,200 m3, fermentation tanks of 4,000 m3, oxidation ponds of 35,000 m3, one sewage treatment system, and a high-efficiency organic fertilizer farm with an annual output of more than 10,000 tons. The biogas slurry sample was generated from pig feces through primary anaerobic fermentation in a continuous stirred tank reactor (CSTR) and a secondary anaerobic fermentation using a black-film-sealed storage process. Once collected, the samples were stored in sealed plastic barrels and were mixed well and filtered through a 0.25-mm mesh screen before the analysis. Samples were then diluted corresponding to the NH4+-N concentration with deionized water for backup use. The basic quality indices of the biogas slurry are shown in Table 1.
The experimental soils were collected from the 0–20 cm plough layer of basic farmland in the plain water network area of the Yangtze River Basin in China. The soil textures were silty loam, loam, and sandy loam. The soils were dried naturally, while stones, plant roots, and other DEBRIS found in the soils were removed. The soils were then crushed with a round wooden stick, sieved through a 2-mm mesh screen, and finally fully mixed and placed into a clean plastic storage box for future use. The particle composition and basic physical and chemical properties of the soils are listed in Tables 2, 3.
Adsorption Kinetics Experiments
Soil samples (10 g) were weighed and placed in 250-ml conical bottles. Two hundred milliliters of biogas slurry (NH4+-N concentration 42.90 ± 0.66 mg/L) was added to each conical bottle at a soil:water ratio of 1:20, and three drops of toluene were added to inhibit microbial activity. Biogas slurry without soil was used as a blank control. The conical bottles were sealed with a silica gel plug, placed in a thermostatic oscillator (set temperature 25 ± 1°C), and oscillated at 140 r/min for 0.25, 0.5, 1, 2, 4, 6, 12, 18, or 24 h. The conical bottles were taken out for biogas slurry sample collection, and a 20-ml biogas slurry sample was taken each time. The biogas slurry sample was centrifuged at a speed of 3800 r/min for 10 min. The concentration of NH4+-N in the supernatant was determined by an automatic flow analyzer (SKALAR SAN++). The above experiments were carried out through destructive sampling. Three batches of experiments were set up. Each batch was set up with nine replicates for each treatment, and one bottle was taken out from the thermostatic oscillator for each round of sampling, which was then discarded after sampling. The average value was taken, and the amount of NH4+-N adsorbed onto the biogas slurry was calculated after the blank was deducted according to the difference in NH4+-N concentration before and after adsorption.
Adsorption Thermodynamic Experiments
A 2.5 g soil sample was mixed with 50 ml biogas slurry with initial diluted concentrations of NH4+-N of 54.25, 88.66, 105.85, 133.71, 178.80, 273.54, and 542.87 mg/L in a polyethylene centrifuge tube. Two drops of toluene were added to inhibit microbial activity, and different concentrations of biogas slurry without soil samples were used as blank controls. The sealing cover of the centrifuge tube was tightened, and samples were mixed with a vortex oscillator and oscillated at 180 r/min on a thermostatic oscillator (set temperature 25 ± 1°C) for 1 h. Samples were then incubated in a thermostat at the same temperature for 3 days, oscillating twice a day at an interval of 12 h for 1 h each time. After cultivation, the samples were centrifuged at 3800 r/min for 10 min. The concentration of supernatant NH4+-N was measured by an automatic flow analyzer (SKALAR SAN++). Each experiment was repeated three times. The amount of NH4+-N absorbed by the tested soil was calculated based on the differences between the initial and final NH4+-N concentration in the supernatant.
Calculation Methods
Adsorption Capacity Calculation Formula
In the formula, Q is the adsorption capacity of NH4+-N (mg/kg); C0 is the initial concentration (mg/L); Ct is the concentration of the solution at the time of measurement (mg/L); V is the volume of the solution (ml); and M is the soil sample weight (g).
Adsorption Fitting Equations
The fitting equations of adsorption kinetics are shown in Table 4. Fitting equations for adsorption thermodynamics are shown in Table 5.
Data Processing
Microsoft Office Excel (2010) software was used for test data processing and table drawing, Origin 2017 software was used for drawing and curve fitting, and the significance of Pearson correlations between variables was tested by IBM SPSS Statistics 13.0 version (IBM Corp., NY, United States).
Results and Analysis
Adsorption Kinetic Characteristics
The adsorption rates of three types of soils on biogas slurry ammonium nitrogen (NH4+-N) are shown in Table 6. The adsorption process of NH4+-N from biogas slurry was a composite kinetic process comprising two stages of initial rapid adsorption followed by slow adsorption. The initial rapid adsorption generally occurred within 0–1 h. The ratio of the rapid adsorption amounts to the total amount of adsorption was 35.24%–43.55%. After 1 h, the process entered the slow adsorption stage. The total adsorption amounts in order from highest to least were adsorbed onto silty loam, loam, and sandy loam.
Adsorption Kinetic Model Fitting
Figures 2A–D shows the fitting results for the adsorption kinetics of NH4+-N in the three kinds of soils obtained by the Elovich equation, a parabolic diffusion equation, a first-order reaction equation, and the ExpAssoc equation. The four equations could effectively simulate the dynamic adsorption process. The fit of the ExpAssoc equation with relevant parameters produced a confidence interval for R2 (0.9923 < R2 < 0.9966) that was better than for the other models (Table 7). Therefore, the ExpAssoc equation was used to fit the adsorption kinetics of NH4+-N in the three kinds of soils.
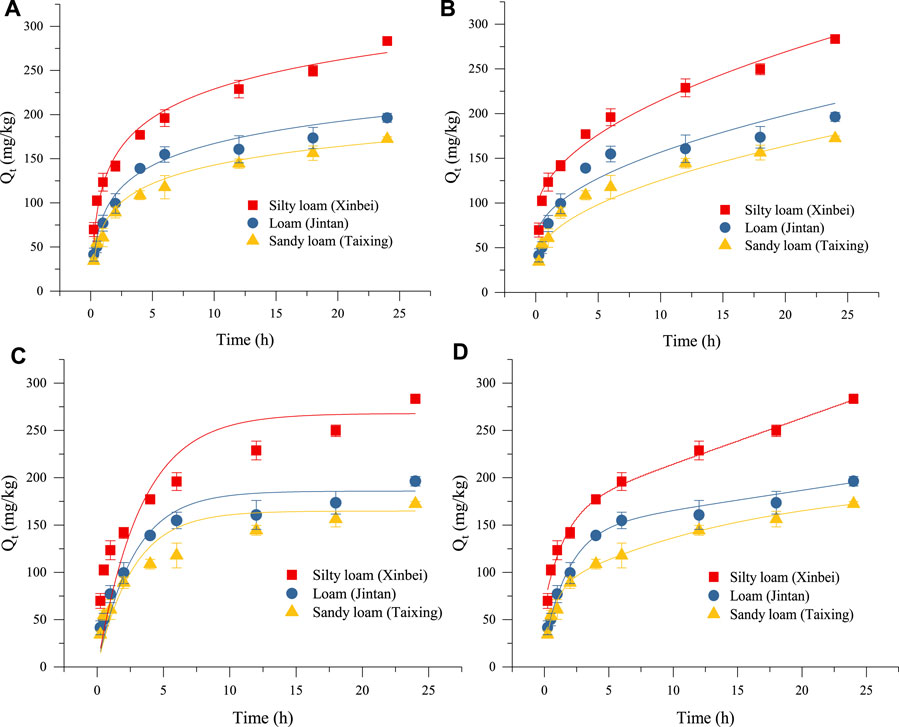
FIGURE 2. Fitting of adsorption kinetics models: (A–D) show the fitting results for the adsorption kinetics of NH4+-N in the three kinds of soils obtained by the Elovich equation, a parabolic diffusion equation, a first-order reaction equation, and the ExpAssoc equation, respectively.
Adsorption Thermodynamic Characteristics
The isothermal adsorption curves of the three soils for biogas slurry NH4+-N at a temperature of 25 ± 1°C are shown in Figure 3. With the increase of the initial biogas slurry NH4+-N concentration, the adsorption isotherms of three soils on NH4+-N increased, primarily due to the increase of initial biogas slurry NH4+-N concentration and the increase of the driving force of the soil itself as an adsorbent, an effect that was conducive to the generation of adsorption. When the initial biogas slurry NH4+-N concentration was less than 200 mg/L, the NH4+-N adsorption capacity of the three soils increased significantly with the increase of initial concentration; when the initial concentration of NH4+-N in biogas slurry was greater than 200 mg/L, the NH4+-N adsorption capacity increased slowly and tended to balance with the increase of initial concentration. When the initial biogas slurry NH4+-N concentration was low, the adsorption sites of the soil itself were not completely occupied. As the initial biogas slurry NH4+-N concentration increased, the unused adsorption sites were gradually occupied and thus were unable to be used, ultimately reaching a saturated state so that the soil and the NH4+-N solution tended to balance, and the soil adsorption capacity of NH4+-N reached its maximum value.
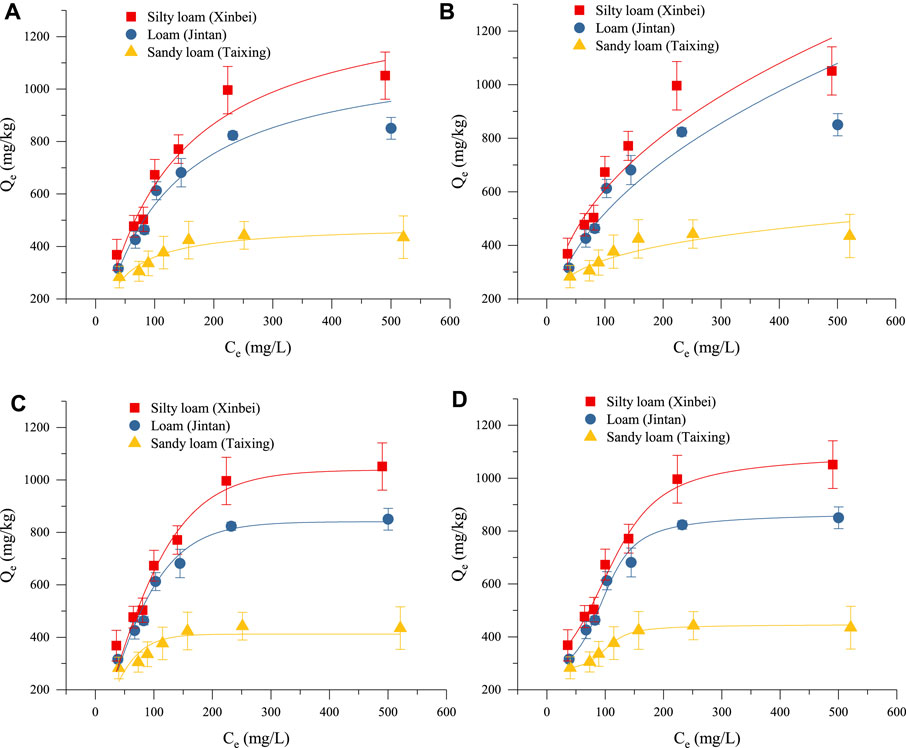
FIGURE 3. Fitting effects of adsorption thermodynamic models: (A–D) show the fitting results for the adsorption thermodynamics of NH4+-N in the three kinds of soils obtained by the Langmuir equation, the Freundlich equation, the Plplatt equation, and the Langevin model, respectively, at a temperature of 25 ± 1°C.
Adsorption Thermodynamic Model Fitting
The Langmuir equation and Freundlich equation are classical models used to study the thermodynamic characteristics of soil NH4+-N adsorption. The Plplatt equation and the Langevin model were the equations selected in this experiment to achieve better fitting. Figures 3A–D shows the fitting results of four equations on the adsorption thermodynamics of biogas slurry NH4+-N at a temperature of 25 ± 1°C. When fitting the theoretical saturated maximum adsorption capacity (Qm) values of biogas slurry NH4+-N for the three kinds of soils, the Qm value fitted by the Langmuir equation was highest, and the Qm value fitted by the Plplatt equation was closer to the measured average value. From the R2 values (Table 8), the adsorption thermodynamic behavior of biogas slurry NH4+-N in this experiment was best fitted by the Langevin Model. The optimal theoretical saturated Qm values calculated by the sum of the parameters a and b were 1108.55, 874.86, and 448.35 mg/kg for silty loam, loam, and sandy loam, respectively.
Correlation Between Adsorption Capacity and Soil Physical and Chemical Properties
Correlations between adsorption characteristic parameters and soil properties are shown in Table 9. The Qm of the soil for biogas slurry NH4+-N was positively correlated with SOM, TN, available phosphorus (AP), available potassium (AK), cation exchange capacity (CEC), and the proportion of 0.02–0.002 mm particles (p < 0.01), while it was negatively correlated with soil pH (p < 0.05). The adsorption constant Kl was negatively correlated with SOM, TN, AP, AK, and the content of particles with the particle size of 0.02–0.002 mm (p < 0.01), negatively correlated with soil NH4+-N and CEC (p < 0.05), and positively correlated with soil pH (p < 0.01). The maximum buffer capacity (MBC) of the soil was negatively correlated with SOM, NH4+-N, AP, AK, and the content of particles with the particle size of 0.02–0.002 mm (p < 0.01), negatively correlated with soil TN (p < 0.05), and positively correlated with soil pH (p < 0.01).
Discussion
Adsorption Mechanism
Adsorption can be defined as the accumulation of solutes at the solid–liquid interface. This process includes the transfer of solute molecules from the solution, the removal of solvent molecules from the solid surface, and the process of solute molecules attaching to the solid surface (Stumm, 1992). Farmland soil media are heterogeneous aggregates with complex structures. A large number of organic and inorganic colloids and oxides are interlaced and mixed (Li W. et al., 2021). There are electric fields and residual force fields on the surface of the media that have extremely high surface energy. They can interact with ions, protons, and molecules in the soil liquid and gas phases, and they have strong adsorption on ammonium nitrogen. There are many kinds of active groups in the media, and all kinds of organic and inorganic groups can interact with ammonium. Therefore, the adsorption behavior of ammonium nitrogen in farmland soil media is complex, and the adsorption behavior in different types of media is significantly different (Krishnamoorthy and Overstreet, 1950). At present, the adsorption mechanism of ammonium nitrogen in soil media is mainly discussed at the macro and micro levels. Most macroscopic research has focused on distinguishing the different active surfaces of soil media using the differences in the extraction capacity of different extractants (Lumbanraja and Evangelou, 1994; Wang and Alva, 2000). Microscopically, the occurrence of ammonium in the medium can be identified at the molecular level using X-ray diffraction (XRD), Fourier transform infrared spectroscopy (FTIR), and other spectral means (Sherman and Smulovitch, 1970; Saruchi and Kumar, 2020). Generally, the adsorption mechanism cannot be accurately described through only a single means. The present study characterized the adsorption characteristics of the three soils, and conducted a preliminary discussion based on the factors affecting the adsorption (Shen et al., 1997), but did not conduct an in-depth study of the adsorption mechanism. This will require further study.
Characteristics of Adsorption Kinetics
Soil is an important site for nitrogen circulation and transformation, and the only way for nitrogen to enter groundwater (He et al., 2021; Li X. et al., 2021). The adsorption of NH4+-N in the soil shows corresponding regularity with time change, which is one of the important characteristics of soil chemical reaction kinetics. The study of kinetics can reveal the limiting factors and control conditions that affect the adsorption rate. Commonly used adsorption kinetics equations include first-order reaction equations, second-order reaction equations, the Elovich equation, the parabolic diffusion equation, and the ExpAssoc equation. The Elovich equation reflects not only a simple adsorption process but also a complex process involving soil expansion, the activation and deactivation of adsorption sites, and surface diffusion (Sparks and Jardine, 1984). In the present experiment, the coefficient R2 for the three types of soil adsorption data was the highest for the Elovich equation, indicating that adsorption was a heterogeneous diffusion process. Further analyzing the restrictive factors of the process revealed that the goodness of fit of the parabolic diffusion equation (0.81 < R2 < 0.97) was higher than that of the first-order reaction equation (0.78 < R2 < 0.92) (Table 7), indicating that the chemical adsorption process was not the rate-limiting step of the process, while the intra-particle diffusion was the main rate-limiting step. Due to the differences in soil media and environmental conditions, the relationship between adsorption capacity and time often differed during the adsorption process. Previous studies have shown that different kinetics models or the same model have different fits with different soils. In the present study, the best fitting models for the soil adsorption of NH4+-N were the first-order reaction equation and the Elovich equation (Xue et al., 1996). The adsorption of NH4+-N in silty sand, sandy silt, silt, and silty clay of four typical soils primarily occurred during 0–2 h, and the adsorption kinetics conformed to the second-order reaction equation (Tian, 2011). However, the fitting effect of the adsorption kinetics curve with the ExpAssoc equation was the best (Zhao et al., 2013). The results of this study were consistent with those of Zhao et al. (2013).
Adsorption Thermodynamic Characteristics
The adsorption of NH4+-N by soil is a dynamic equilibrium process. Under the same constant temperature, the curve of the adsorption amount (Q) with the equilibrium concentration (C) of the solution is normally referred to as the adsorption isotherm, and the corresponding mathematical expression is called the adsorption isothermal formula. This equation reflects the specific relationship between the adsorbent and the adsorption capacity, as well as the influence of different NH4+-N concentrations on the ability of the soil to adsorb NH4+-N. At present, the Langmuir equation, the Freundlich equation, the Henry equation, and the Temkin equation are commonly used to describe the adsorption of NH4+-N in soil. The Langmuir equation assumes that the medium surface is uniform and the adsorption is performed by single molecules; this model can be used to calculate the corresponding maximum saturated adsorption capacity, adsorption coefficient, and MBC, as well as to evaluate and predict the adsorption of NH4+-N in the soil. In this experiment, the Langmuir equation had a high degree of fit, indicating that the isothermal adsorption of NH4+-N in the three soils was mainly monolayer adsorption, while multi-molecular layer adsorption was of secondary importance. The Freundlich equation is often used to describe the adsorption of non-uniform surfaces, but the maximum saturated adsorption capacity cannot be calculated. The fitting parameter 1/n of the Freundlich equation in this study was between 0 and 1, indicating that the isothermal adsorption of NH4+-N by the three kinds of soils was relatively efficient. The Henry equation is suitable for low concentrations and weak adsorption, while the Temkin equation is only suitable for chemical adsorption. As the latter two models had poor fits to the experimental data, the fitting of these two equations was not presented in this paper. At the same time, in order to further improve the application and evaluation of the fitting results, for the maximum saturated adsorption capacity, the analysis screened out the Plplatt equation whose fitting Qm value was close to the measured average value, and the Langevin model whose fitting curve was more optimal. Although the adaptability of the assumptions that the adsorption model established to soil adsorption characteristics of NH4+-N was worthy of further validation, the law summarized by the analysis of the experimental data was basically consistent with the adsorption characteristics reflected by the empirical adsorption isotherm. Therefore, it was effective to use empirical adsorption isotherms to quantitatively describe the thermodynamic behavior of the soil adsorption of NH4+-N.
Soil Factors Affecting Adsorption
The NH4+-N Qm of soil is affected by soil texture, environmental temperature and humidity, artificial fertilization, and crop rotation. The Qm obtained by the Langmuir equation reflected the maximum saturated adsorption capacity of NH4+-N in the soil, as well as the number of soil adsorption sites. Li et al. (2009) found that Qm was significantly negatively correlated with soil pH and CEC, while it was significantly positively correlated with soil C:N. Research by Cong et al. (2017) showed that Qm was significantly positively correlated with soil pH and CEC, while it was significantly negatively correlated with SOM and TN content. Wang et al. (2015) reported that the greater the organic matter content, the greater the NH4+-N adsorption capacity of albic soil. Xue et al. (1996) found that the adsorption capacity of NH4+-N increased with the increase of soil CEC and soil clay content to varying degrees. The results of the present study showed that the Qm value was significantly negatively correlated with soil pH, consistent with the results of Li et al. (2009). A possible reason was that the biogas slurry was alkaline, and the pH values of weakly acidic soils in Xinbei and Jintan were neutralized, resulting in the release of adsorption sites originally occupied by H+ and thereby reducing the competition between H+ and NH4+ for adsorption sites. There was a significant positive correlation between the Qm value and CEC, consistent with the results of Cong et al. (2017), Xue et al. (1996), and Shen et al. (1997). Qm was positively correlated with SOM, consistent with the results of Li et al. (2009) and Wang et al. (2015). This might be because organic matter had a large number of different functional groups (Liu et al., 2010), a higher CEC, and a larger specific surface area (Khorram et al., 2015; Acosta et al., 2016; Kumar, et al., 2016); these were all factors that could increase the adsorption capacity of NH4+-N through surface complexation, ion exchange, and surface precipitation. These results have been verified in many studies aimed at the improving soil adsorption of NH4+ by biochar (Yao et al., 2012).
Jiang (2004) showed that the finer the soil particles, the lower the percentage of sand powder with a particle size of ≥0.01 mm, while the higher the percentage of clay particles with a particle size of <0.005 mm, the stronger the adsorption. Xue et al. (1996) reported that NH4+-N adsorption capacity was mainly affected by clay content, with clay > loam > sandy soil. Cong et al. (2017) showed that the NH4+-N adsorption capacity of soil was in the order of light clay > light loam. The results of this study showed that the Qm value of silty loam > loam > sandy loam was consistent with the above research results. Wan et al. (2004) found that there was a very significant positive correlation between fixed ammonium and clay content <0.01 mm, with clay content of 0.005–0.01 mm, and with clay content of 0.001–0.005 mm, but there was no significant correlation with clay content <0.001 mm. The results of this study showed that there was a significant positive correlation between the Qm value and the content of particles with particle size of 0.02–0.002 mm in the soil. The same trend was observed in the results of Wan et al. (2004). Therefore, it is speculated that the increase of soil fixed ammonium content is a characteristic that can be further verified based on the fact that the fixed ammonium soil content increased with the increase of ammonium ion concentration (Liang and MacKenzie, 1994; Juang et al., 2001). In addition, there was a very significant positive correlation between the Qm value and soil TN content, as well as a significant correlation with soil nitrate nitrogen. This may be the reason for the positive and negative electrical adsorption, and may be responsible for maintaining the balance between soil new ammonium nitrogen and nitrate nitrogen. The Qm value was positively correlated with the soil AK. This was because active K+ ions could provide adsorption sites and interlayer solid localization for NH4+ ions in external soil, which was in line with cation exchange theory (Rich and Black, 1964; Kittrick, 1966). The Qm value was positively correlated with soil AP in this experiment. There were two possible reasons: the positive and negative charge adsorption, and the combination of NH4+ ions and PO43− ions to form [(NH4) PO4]2−, subsequently forming complex precipitates Mg(NH4)[PO4]·6H2O with the Mg2+ and Ca2+ plasma in the soil, thereby increasing the adsorption of NH4+-N. The correlation between the Langmuir equation adsorption constant Kl value, the MBC value, and soil property-related indicators, and the correlation between the Qm value and soil property-related indicators were opposite (Li et al., 2009; Cong et al., 2017), indicating that the adsorption strength and the adsorption capacity were complementary. In other words, when the adsorption capacity is high, the adsorption strength is low. In addition, the fitted Qm values of the NH4+-N adsorption of the soils in this study were lower than the fitted value of a single chemical ammonium salt solution, thus indicating that the rich complex components of the biogas slurry interfered with the adsorption of ammonium nitrogen in the soil as described in Zhao et al. (2013). While a preliminary demonstration has been achieved in the present research, the specific mechanism of action requires further study.
Conclusion
The soil adsorption of biogas slurry NH4+-N predominantly occurred within 0–1 h, and the adsorption capacity within 0–1 h accounted for 35.24%–43.55% of the total adsorption. The ExpAssoc equation produced a good fit for the adsorption kinetic behavior. The optimal theoretical saturated adsorption capacity (Qm) values fitted by the Langevin model were 1108.55, 874.86g, and 448.35 mg/kg for silty loam, loam, and sandy loam, respectively. The Qm was significantly positively correlated with SOM, TN, AP, AK, CEC, and particle content of 0.02–0.002 mm, but significantly negatively correlated with soil pH.
Data Availability Statement
The raw data supporting the conclusion of this article will be made available by the authors, without undue reservation.
Author Contributions
ZW: conceptualization, methodology, investigation, data curation, writing—original draft, and funding acquisition. LZ: investigation and data curation. GS and WZ: data curation. JS: conceptualization, data curation, supervision, and funding acquisition. XY: methodology and funding acquisition. AO: conceptualization, supervision, and funding acquisition. EK: conceptualization, methodology, writing—review and editing.
Funding
This work was supported by the National Key Research and Development Program of China (2018YFD0800105); the Jiangsu Agricultural Science and Technology Innovation Fund of China (CX(20)2014); the Jiangsu Province Key Research and Development Project (Modern Agriculture) of China (BE2019395); and the Collaborative Extension Plan of Jiangsu Major Agricultural Technologies of China (2020-SJ-047-04-01).
Conflict of Interest
The authors declare that the research was conducted in the absence of any commercial or financial relationships that could be construed as a potential conflict of interest.
Publisher’s Note
All claims expressed in this article are solely those of the authors and do not necessarily represent those of their affiliated organizations, or those of the publisher, the editors, and the reviewers. Any product that may be evaluated in this article, or claim that may be made by its manufacturer, is not guaranteed or endorsed by the publisher.
Acknowledgments
We thank LetPub (www.letpub.com) for its linguistic assistance during the preparation of this manuscript.
References
Acosta, R., Fierro, V., Martinez de Yuso, A., Nabarlatz, D., and Celzard, A. (2016). Tetracycline Adsorption onto Activated Carbons Produced by KOH Activation of Tyre Pyrolysis Char. Chemosphere 149, 168–176. doi:10.1016/j.chemosphere.2016.01.093
Bradford, S. A., Segal, E., Zheng, W., Wang, Q., and Hutchins, S. R. (2008). Reuse of Concentrated Animal Feeding Operation Wastewater on Agricultural Lands. J. Environ. Qual. 37, S97–S115. doi:10.2134/jeq2007.0393
Chen, D., Jiang, L., Huang, H., Toyota, K., Dahlgren, R. A., and Lu, J. (2013). Nitrogen Dynamics of Anaerobically Digested Slurry Used to Fertilize Paddy Fields. Biol. Fertil. Soils. 49 (6), 647–659. doi:10.1007/s00374-012-0752-8
Ciravolo, T. G., Martens, D. C., Hallock, D. L., Collins, E. R., Kornegay, E. T., and Thomas, H. R. (1979). Pollutant Movement to Shallow Ground Water Tables from Anaerobic Swine Waste Lagoons. J. Environ. Qual. 8 (1), 126–130. doi:10.2134/jeq1979.00472425000800010027x
Cong, R., Zhang, L., Lu, Y., Huang, Q., Shi, X., Li, X., et al. (2017). Adsorption-Desorption Characteristics of Soil Ammonium under Long-Term Straw Returning Condition. J. Plant Nutr. Fert. 23 (2), 380–388. doi:10.11674/zwyf.16307
Dalal, R. C. (1975). Effect of Associated Anions on Ammonium Adsorption by and Desorption from Soils. J. Agric. Food Chem. 23 (4), 684–687. doi:10.1021/jf60200a026
Feinerman, E., Bosch, D. J., and Pease, J. W. (2004). Manure Applications and Nutrient Standards. Am. J. Agric. Econ. 86 (1), 14–25. doi:10.1111/j.0092-5853.2004.00559.x
Gao, T., Chen, N., Li, W., Li, B., and Yuan, H. (2011). Effect of Highly Efficient Nutrient Solution of Biogas Slurry on Yield and Quality of Vegetables. Agric. Sci. Technol. 12 (4), 567–570. doi:10.3969/j.issn.1009-4229-B.2011.04.026
Ham, J. M., and DeSutter, T. M. (1999). Seepage Losses and Nitrogen Export from Swine‐Waste Lagoons: A Water Balance Study. J. Environ. Qual. 28 (4), 1090–1099. doi:10.2134/jeq1999.00472425002800040005x
Han, M., Liu, K., Wang, S., Qin, L., and Gao, C. (2014). Definition, Ingredient, Approaches and Risks for Reuse in Biogas Slurry. J. Agric. 4 (10), 54–57.
He, G., Liu, X., and Cui, Z. (2021). Achieving Global Food Security by Focusing on Nitrogen Efficiency Potentials and Local Production. Glob. Food Secur. 29, 100536. doi:10.1016/j.gfs.2021.100536
Jiang, G. (2004). Discussion about NH4+-N Adsorptive Ability in Soils. J. Chang'an Univ. Archit. Environ. Sci. Ed. 21 (2), 32–34,38. doi:10.3321/j.issn:1673-2049.2004.02.009
Jin, H., Chang, Z., Ye, X., Ma, Y., and Jin, Z. (2011). Physical and Chemical Characteristics of Anaerobically Digested Slurry from Large-Scale Biogas Project in Jiangsu Province. Trans. Chin. Soc. Agric. Eng. 27 (1), 291–296. doi:10.3969/j.issn.1002-6819.2011.01.047
Jin, H., Fu, G., Chang, Z., Ye, X., Chen, G., and Du, J. (2012). Distribution of Nitrogen in Liquid and Solid Fraction of Pig and Dairy Manure in Anaerobic Digestion Reactor. Trans. Chin. Soc. Agric. Eng. 28 (21), 208–214. doi:10.3969/j.issn.1002-6819.2012.21.029
Juang, T. C., Wang, M. K., Chen, H. J., and Tan, C. C. (2001). Ammonium Fixation by Surface Soils and Clays. Soil Sci. 166 (5), 345–352. doi:10.1097/00010694-200105000-00005
Khorram, M. S., Wang, Y., Jin, X., Fang, H., and Yu, Y. (2015). Reduced Mobility of Fomesafen through Enhanced Adsorption in Biochar-Amended Soil. Environ. Toxicol. Chem. 34 (6), 1258–1266. doi:10.1002/etc.2946
Kithome, M., Paul, J. W., Lavkulich, L. M., and Bomke, A. A. (1998). Kinetics of Ammonium Adsorption and Desorption by the Natural Zeolite Clinoptilolite. Soil Sci. Soc. Am. J. 62 (3), 622–629. doi:10.2136/sssaj1998.03615995006200030011x
Kittrick, J. A. (1966). Forces Involved in Ion Fixation by Vermiculite. Soil Sci. Soc. Am. J. 30 (6), 801–803. doi:10.2136/sssaj1966.03615995003000060040x
Krishnamoorthy, C., and Overstreet, R. (1950). An Experimental Evaluation of Ion-Exchange Relationships. Soil Sci. 69 (1), 41–54. doi:10.1097/00010694-195001000-00003
Kumar, V., and Kothiyal, N. C. (2012). Distribution Behavior and Carcinogenic Level of Some Polycyclic Aromatic Hydrocarbons in Roadside Soil at Major Traffic Intercepts Within a Developing City of India. Environ. Monit. Assess. 184, 6239–6252. doi:10.1007/s10661-011-2416-9
Kumar, V., and Kothiyal, N. C. (2011). Distribution Behavior of Polycyclic Aromatic Hydrocarbons in Roadside Soil at Traffic Intercepts within Developing Cities. Int. J. Environ. Sci. Technol. 8, 63–72. doi:10.1007/BF03326196
Kumar, V., Kothiyal, N. C., and Saruchi, (2016). Analysis of Polycyclic Aromatic Hydrocarbon, Toxic Equivalency Factor and Related Carcinogenic Potencies in Roadside Soil within a Developing City of Northern India. Polycycl. Aromat. Compd. 36 (4), 506–526. doi:10.1080/10406638.2015.1026999
Li, H., Han, Y., and Cai, Z. (2009). Equilibrium of Ammonium Adsorption in Paddy Soils of Taihu Region of China under Different Reaction Conditions. Chin. J. Soil Sci. 40 (1), 89–95. doi:10.3321/j.issn:0564-3945.2009.01.026
Li, W., Shi, Y., Zhu, D., Wang, W., Liu, H., Li, J., et al. (2021). Fine Root Biomass and Morphology in a Temperate Forest are Influenced More by the Nitrogen Treatment Approach Than the Rate. Ecol. Indic. 130, 108031. doi:10.1016/j.ecolind.2021.108031
Li, X., Zhang, C., Zhang, B., Wu, D., Zhu, D., Zhang, W., et al. (2021). Nitrogen Deposition and Increased Precipitation Interact to Affect Fine Root Production and Biomass in a Temperate Forest: Implications for Carbon Cycling. Sci. Total Environ. 765, 144497. doi:10.1016/j.scitotenv.2020.144497
Liang, B. C., and MacKenzie, A. F. (1994). Fertilization Rates and Clay Fixed Ammonium in Two Quebec Soils. Plant Soil 163, 103–109. doi:10.1007/bf00033946
Liu, C., Chen, Y., Li, X., Zhang, Y., Ye, J., Huang, H., et al. (2020). Temporal Effects of Repeated Application of Biogas Slurry on Soil Antibiotic Resistance Genes and Their Potential Bacterial Hosts. Environ. Pollut. 258, 113652. doi:10.1016/j.envpol.2019.113652
Liu, H., Dong, Y., Wang, H., and Liu, Y. (2010). Ammonium Adsorption from Aqueous Solutions by Strawberry Leaf Powder: Equilibrium, Kinetics and Effects of Coexisting Ions. Desalination 263 (1-3), 70–75. doi:10.1016/j.desal.2010.06.040
Lu, J., Zhu, L., Hu, G., and Wu, J. (2010). Integrating Animal Manure-Based Bioenergy Production with Invasive Species Control: A Case Study at Tongren Pig Farm in China. Biomass Bioenergy 34 (6), 821–827. doi:10.1016/j.biombioe.2010.01.026
Lumbanraja, J., and Evangelou, V. P. (1994). Adsorption-Desorption of Potassium and Ammonium at Low Cation Concentrations in Three kentucky Subsoils. Soil Sci. 157 (5), 269–278. doi:10.1097/00010694-199405000-00001
Ni, Z., and Zhang, M. (2017). Chemical Compositions of Nitrogen, Phosphorus, and Potassium in Biogas Slurry and Their Bio-Availability. Chin. J. Soil Sci. 48 (5), 1114–1118. doi:10.19336/j.cnki.trtb.2017.05.13
Ning, J., Zhou, M., Pan, X., Li, C., Lv, N., Wang, T., et al. (2019). Simultaneous Biogas and Biogas Slurry Production from Co-Digestion of Pig Manure and Corn Straw: Performance Optimization and Microbial Community Shift. Bioresour. Technol. 282, 37–47. doi:10.1016/j.biortech.2019.02.122
Rich, C. I., and Black, W. R. (1964). Pottasium Exchange as Affected by Cation Size, pH, and Mineral Structure. Soil Sci. 97 (6), 384–390. doi:10.1097/00010694-196406000-00004
Saruchi, , and Kumar, V. (2020). Effective Degradation of Rhodamine B and Congo Red Dyes over Biosynthesized Silver Nanoparticles-Imbibed Carboxymethyl Cellulose Hydrogel. Polym. Bull. 77, 3349–3365. doi:10.1007/s00289-019-02920-x
Shen, S., Tu, S.-I., and Kemper, W. D. (1997). Equilibrium and Kinetic Study of Ammonium Adsorption and Fixation in Sodium-Treated Vermiculite. Soil Sci. Soc. Am. J. 61 (6), 1611–1618. doi:10.2136/sssaj1997.03615995006100060011x
Sherman, W. F., and Smulovitch, P. P. (1970). Pressure‐Scanned Fermi Resonance in the Spectrum of NH4+ Isolated in CsBr. J. Chem. Phys. 52, 5187–5193. doi:10.1063/1.1672759
Sparks, D. L., and Jardine, P. M. (1984). Comparison of Kinetic Equations to Describe Potassium-Calcium Exchange in Pure and in Mixed Systems. Soil Sci. 138 (2), 115–122. doi:10.1097/00010694-198408000-00004
Tian, H. (2011). Ammonia-Nitrogen Adsorption Characteristics onto Vadose Zone Soils in Luanhe Delta. Chin. J. Environ. Eng. 5 (3), 507–510.
Wan, D., Zhang, Y., Feng, Y., and Huang, Y. (2004). Fixed Ammonium Content of Soils on Cultivated Upland in Hunan Province and its Affecting Factors. Acta Pedol. Sin. 41 (3), 480–483. doi:10.3321/j.issn:0564-3929.2004.03.025
Wang, F. L., and Alva, A. K. (2000). Ammonium Adsorption and Desorption in Sandy Soils. Soil Sci. Soc. Am. J. 64 (5), 1669–1674. doi:10.2136/sssaj2000.6451669x
Wang, S., Wang, N., Zhang, J., and Fan, C. (2015). Influencing Factors of NH4+ Adsorption Characteristics on Albic Soil. Soil Fert. Sci. China. 2, 22–28. doi:10.11838/sfsc.20150205
Wang, Y., Fu, J., Wang, Q., Wang, J., Ma, J., and Jiang, L. (2010). Effect of Biogas Slurry Farmland Disposal and Utilization on Pepper´s Yield, Quality and Soil Fertility. Acta Agric. Zhejiangensis. 22 (6), 859–863. doi:10.3969/j.issn.1004-1524.2010.06.030
Wang, Z., Liang, Y., Sheng, J., Guan, Y., Wu, H., Chen, L., et al. (2016). Analysis of Water Environment Risk on Biogas Slurry Disposal in Paddy Field. Trans. Chin. Soc. Agric. Eng. 32 (5), 213–220. doi:10.11975/j.issn.1002-6819.2016.05.030
Xue, Q., Wei, Q., Gao, Y., Shi, H., and Qu, D. (1996). Kinetic Characteristics of NH4+ Adsorption-Desorption for Five Major Soils in Shanxi Province. Acta Pedol. Sin. 32 (2), 129–136.
Yang, R., Sun, Q., Zhao, H., Zou, G., Liu, B., and Li, L. (2017). Precision Application of Biogas Slurry and its Environmental Effects in Paddy Fields. J. Agro-Environ. Sci. 36 (8), 1566–1572. doi:10.11654/jaes.2016-1617
Yao, Y., Gao, B., Zhang, M., Inyang, M., and Zimmerman, A. R. (2012). Effect of Biochar Amendment on Sorption and Leaching of Nitrate, Ammonium, and Phosphate in a Sandy Soil. Chemosphere 89 (11), 1467–1471. doi:10.1016/j.chemosphere.2012.06.002
Zhao, W., Li, Y., Zhao, Q., Ning, Z., Zhou, C., Wang, H., et al. (2013). Adsorption and Desorption Characteristics of Ammonium in Eight Loams Irrigated with Reclaimed Wastewater from Intensive Hogpen. Environ. Earth Sci. 69 (1), 41–49. doi:10.1007/s12665-012-1932-4
Keywords: biogas slurry, wastewater, ammonium nitrogen, soil absorption, kinetics, thermodynamics
Citation: Wang Z, Zhang L, Sun G, Zhou W, Sheng J, Ye X, Olaniran AO and Kana EBG (2022) Adsorption Characteristics of Three Types of Soils on Biogas Slurry Ammonium Nitrogen. Front. Environ. Sci. 10:942263. doi: 10.3389/fenvs.2022.942263
Received: 12 May 2022; Accepted: 06 June 2022;
Published: 21 June 2022.
Edited by:
Tao Zhang, China Agricultural University, ChinaReviewed by:
Hamou Moussout, Ibn Tofail University, MoroccoJale Gülen, Yıldız Technical University, Turkey
Maulidan Firdaus, Sebelas Maret University, Indonesia
Vaneet Kumar, CT Group of Institutions, India
Copyright © 2022 Wang, Zhang, Sun, Zhou, Sheng, Ye, Olaniran and Kana. This is an open-access article distributed under the terms of the Creative Commons Attribution License (CC BY). The use, distribution or reproduction in other forums is permitted, provided the original author(s) and the copyright owner(s) are credited and that the original publication in this journal is cited, in accordance with accepted academic practice. No use, distribution or reproduction is permitted which does not comply with these terms.
*Correspondence: E. B. Gueguim Kana, a2FuYWdAdWt6bi5hYy56YQ==