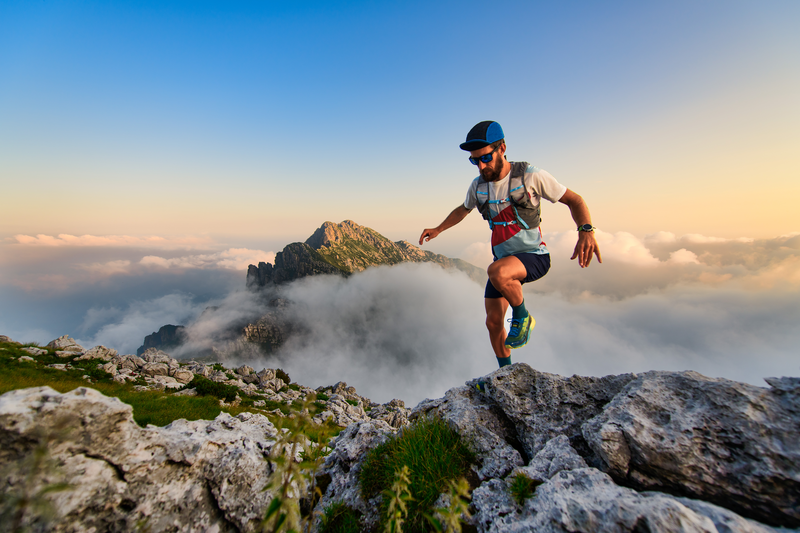
94% of researchers rate our articles as excellent or good
Learn more about the work of our research integrity team to safeguard the quality of each article we publish.
Find out more
ORIGINAL RESEARCH article
Front. Environ. Sci. , 08 September 2022
Sec. Water and Wastewater Management
Volume 10 - 2022 | https://doi.org/10.3389/fenvs.2022.941841
This article is part of the Research Topic Emerging Approaches for Sustainable Management for Wastewater View all 8 articles
The increasing amount of sewage has become a serious concern globally, demanding sustainable solutions. The constructed wetland system (CWS) can be installed at the wastewater discharge site and properly screened bio-purifiers can be used for efficient wastewater treatment. Filter-feeding zooplankton have the potential to graze on bacteria and reduce biological oxygen demand. However, higher suspended particles choke the zooplankton filtering appendages. An integrated application of zooplankton and macrophytes can solve the problems by reducing contaminants and providing the surface area for zooplankton to take refuge. Using three different approaches: (a) screening of natural bio-purifiers through time series study, (b) assessment of sewage treatment potential of screened bio-purifiers in the CWS and, (c) evaluation of the growth potential of screened bio-purifiers in untreated wastewater, this study explored the combined use of macrophyte and zooplankton in the CWS. The 3-year time series study in the vicinity of the wastewater discharge area recorded the zooplankton, Brachionus angularis and Moina macrocopa as highly indicative zooplankton. The top two highly indicative macrophytes are Typha and Phragmites. Under laboratory conditions, the population growth rates of B. angularis and M. macrocopa were significantly higher in wastewater without any external nutrient source than those in control with algae as a food source. The integrated application of zooplankton (B. angularis and M. macrocopa) macrophytes (Typha and Phragmites) in CWS yielded upto 56% reduction in the total bacterial count and upto 92% reduction in BOD along with substantial increase in the DO level. The present results strongly suggested the use of CWS planted with macrophytes and inoculated by zooplankton. The usefulness of this system is further supported by the natural occurrence of selected species, as it is easy to maintain, has low installation cost, and excellent efficiency in treating wastewater. As both the zooplankton species are preferred live feed for rearing of fish larvae, the present results suggested the use of zooplankton and macrophytes for treatment of wastewater, reduction of sludge, and harvest of live feeds for the aquaculture industry to augment circularity and promote decentralized wastewater treatment.
In recent years, rapid urbanization has led to the large-scale generation of untreated or partially treated wastewater. Increasing unplanned urbanization, coupled with modern lifestyles is estimated to escalate the water demand to 55%–60% (IUCN, 2013) by 2050 for the 10 billion projected population. Wastewater from various sources has been reported to be contaminated by multiple pollutants such as nutrients (Van Puijenbroek et al., 2019), pathogens (Vermeulen et al., 2015; Hofstra and Vermeulen, 2016) from human excretion, plastics (Avio et al., 2017; Boucher and Friot, 2017; Lebreton et al., 2017; Schmidt et al., 2017; Siegfried et al., 2017), and chemicals from personal care products (Diamond and Cohen, 2018; Van Wijnen et al., 2019). Clean drinking water and sanitation are not available to billions of people, particularly in rural areas. According to the UN report, only one-third of the world’s population has access to clean drinking water, and more than 673 million people defecate in the open (United Nations Sustainable Development Goal, 2020). According to the UN Sustainable Development Goal Report (United Nation, 2019) more than one-third of the nations in Northern Africa, Western, Central, and Southern Asia are suffering from medium to high water stress. In India, only about a third of the sewage produced each day can be treated (CPCB, 2021). The sustained inflow of untreated or partially treated sewage to river systems has led to the contamination of Ganga water with pathogens and nutrients. The large volume of untreated sewage is a major threat to the availability of clean water in different parts of the globe and is also expected to pollute the coastal and river water (Damania et al., 2019), and impede the progress toward the accomplishment of Sustainable Development Goal 6 (SDG, clean water for all), 11 (sustainable cities), and 14 (life below water). So, the increasing amount of sewage has become a serious concern throughout the world, demanding sustainable solutions.
The conventional treatment methods are not often appropriate because of the high startup, operational, and maintenance expenses, and requirements for human personnel (Capodaglio, 2017; Oladoja, 2017). The conventional sewage treatment method is also known for higher energy consumption and sludge production, which significantly increases on-site and off-site greenhouse gas emissions. This method uses mixed bacterial cultures with suspended or attached growth in activated sludge systems, trickling filters, membranes, or moving bed biofilm reactors (Koutsou et al., 2018). Recent public health and economic problems have been exacerbated by the COVID-19 epidemic. So, larger scale scientific efforts have been directed toward the development of alternative cost-effective processes that utilize various macrophytes, zooplankton, phytoplankton, bacterial biofilm, or fungi in domestic or industrial wastewater treatment. These processes aimed to achieve desirable performance, fixation of CO2, and biomass production that could have multiple utility. By considering these and other relevant aspects, natural remedial approaches that are low-cost, easy to use, and based on natural ecological roles can help to lessen these problems (Langergraber and Muellegger, 2005). The biota is the best indicator of ecological changes since it gives insights into the real biological impact of the change (Zhou et al., 2008). Biological treatment can be a sustainable solution for the treatment of wastewater using natural bio-purifiers that are adapted to the local environmental conditions and that are self-replicative in the treatment system. So, for biological treatment it is important to search for proper bio-purifiers that are evolutionarily adapted to wastewater conditions, proven to be bio indicators of pollution, and originate from the same locality.
Zooplankton have been shown to thrive in wastewater. A study reported a total of 22 zooplankton species in the contaminated municipal wastewater ponds (Adhikari et al., 2017). Filter-feeding zooplankton can remove pollutants from wastewater (Ebert, 2005), utilize pathogen bacteria as a food source (Kumar and Rao, 1999; Kumar, 2003), and kill disease vectors (Kumar and Rao, 2003; Kumar and Hwang, 2008). However, many environmental factors influence and govern the spatial and seasonal growth and succession of zooplankton, including the environmental variables such as DO, nutrients, pH, and TDS (Jafari et al., 2011). Freshwater zooplankton are often considered a bioindicator of water pollution and trophic health, being an essential biological component that serves as a bio-purifier of wastewater (Parmar et al., 2016). It is relevant to explore the possibility of using these pollution-tolerant species in wastewater treatment systems. Such nature-based methods of wastewater treatment require the use of wetlands, lagoons, ponds, and other aerobic treatment systems (Stottmeister et al., 2003; Matamoros et al., 2012; Hunter et al., 2019; Zhang et al., 2019; Zraunig et al., 2019).
Zooplankton, particularly Cladocera and Rotifera, can operate as natural biofilters, as changes in aquatic ecosystems are highly susceptible to changes in zooplankton populations. Environmental disruptions can be seen in changes in species composition, abundance, and distribution of body size (US EPA, 2021). Organic matter ammonia, ammonium and nitrite, and metals are among the pollutants that affect zooplankton (Schlüter and Groeneweg, 1985; Lyu et al., 2013; Serra et al., 2014; Okamoto et al., 2015). Nevertheless, zooplankton are not able to survive and proliferate in case of severely polluted raw sewage. It is possible to overcome this problem by combining zooplankton with microbe biofilms and/or macrophytes, as zooplankton are filter feeders and have limited access to microorganisms adhered to the reactor’s or plant’s surface (Langis et al., 1988). Contributed by high tolerance to contaminants, capability of purification, easy management, and high productivity, macrophytes are considered an appropriate candidate for phytoremediation in natural treatment systems. Although macrophytes have efficiency to improve treatment potential of a constructed wetland, selection of plant species for natural treatment to reclaim sewage water is a major challenge that needs proper attention.
The success and sustainability of the biological treatment system would depend upon the survival probability and adaptability of bio-purifiers in a wastewater environment. In general, zooplankton are the first heterotrophic compartment that could respond to the change in the contamination level, bacterial community, and other anthropogenic pressures. The evaluation of their growth and prediction of the trend in the wastewater systems are necessary, and this can make sure of a reasonable decision in the CWS phytoremediation engineering projects.
Bacterivorous and particulate-feeding zooplankton are recognized to play a vital role in enhancing the efficacy of wastewater treatment processes (Kampf et al., 2007). For water ecotoxicology tests, Brachionidae rotifers and Moinidae cladocerans are commonly utilized. (Harmon and Wiley, 2010; Hader and Erzinger, 2017). It is also possible to use these species in wastewater treatment methods because they are filter feeder organisms that consume algae and other organic waste particles, including protists and bacteria (Kumar, 2003; Gliwicz and Wrzosek, 2008; Kumar and Hwang, 2008). Previous studies have shown that daphnid cladocerans can remove small suspended particles of particle size smaller than 30 mm (Pau et al., 2013), as well as lower pathogenic bacterial burdens such as E. coli and other coliforms (Shiny et al., 2005; Serra et al., 2014; Burnet et al., 2017). These findings suggest prospecting of wastewater adapted zooplankton for biofilter (removal of suspended solid material) and disinfectant (removal of bacterial load) in wastewater.
Previous studies on another cladoceran zooplankton, Daphnia spp. have suggested that after optimization of the process, it is possible to use Daphnia spp. in the treatment of tertiary wastewater (Matamoros et al., 2012; Serra et al., 2014; Maceda-Veiga et al., 2015; Serra and Colomer, 2016; Müller et al., 2018). Despite this, these zooplankton enable to remove nitrogen or phosphorus compounds and are vulnerable to a variety of other pollutants such as high organic matter, heavy metal load, ammonia, ammonium, and nitrite (Lyu et al., 2013; Okamoto et al., 2015; Serra et al., 2019). So, the presence of suitable macrophytes in the CWS is likely to complement the removal process. A combination of suitable macrophytes that can reduce the nutrient content and selected zooplankton populations may overcome this challenge. To determine whether macrophytes and zooplankton can be integrated in a constructed wetland for wastewater treatment, this study uses (i) time series data from the affected portion of the Ganges River, (ii) laboratory-scale experiments to study population dynamics of screened zooplankton species in wastewater, and (iii) an integrated application of macrophytes and zooplankton in the CWS.
In order to optimize the efficiency of natural bio-purifiers, the present study adopted three different approaches, namely, (i) screening of Gangetic bio-purifiers through a time series study of zooplankton, macrophytes, and water quality parameters in the wastewater discharge region, (ii) survival probability and population dynamics of screened bio-purifiers (which are also considered as a live feed in the aquaculture industry) in wastewater, and (iii) evaluation of the treatment potential of the screened Gangetic bio-purifiers in the constructed wetland at the wastewater discharge site.
For the selection of potent, ecologically viable, locally adapted, and naturally occurring bio-purifiers, we conducted 3 years’ time series study of prokaryotic and eukaryotic microbes (zooplankton) at the sewage discharge site in the River Ganges, from June 2016 to March 2019 and 7 months’ time series study of macrophyte community from November 2018 to May 2019 in the vicinity of the mouth of the sewage drain.
The study was conducted at the southern bank of the River Ganga, referred as Kalighat (25.61983°N and 85.15867°E) in Patna, Bihar, India. The sampling site was selected based on the wastewater discharge site (Figure 1). A ∼10 km long sewage canal originating from Digha, Patna, passing beside the southern bank of River Ganga empties its content at the western (downstream) side of the Kalighat (25.61983°N and 85.15867°E). This is mix wastewater channel of both domestic and biomedical wastes. Four major drains (Table 1) from different parts of the Patna city merge with this sewage canal before emptying the sewage into the River Ganges at Kalighat (CPCB, 2018). The exact sampling point was located at ∼10 m downstream of the mouth of the discharge channel.
FIGURE 1. Map showing sites of time series study, constructed wetland system (CWS) and floodplain between Anatghat and Kalighat for monitoring of macrophytes.
TABLE 1. Major drains that merge with the wastewater canal which empties the sewage into the River Ganga at Kalighat.
Selected water parameters (Supplementary Table S1), Chl a, bacterial concentration (CFU and coliform), and zooplankton community were analyzed at each sampling during 36 sampling events, whereas the macrophyte community was analyzed during seven sampling cruises from November 2018 to May 2019 from the sewage affected areas in the vicinity between Antaghat and Kalighat (Figure 1).
Surface water samples were collected in cleaned acid-washed polyethylene bottles. During each sampling, we measured water temperature, pH, and electrical conductivity (EC) on-site, and the samples were transported to the laboratory for the estimation of dissolved oxygen (DO), biological oxygen demand (BOD5), phosphate, nitrate, and Chl a. Winkler’s method was followed for measuring DO. The dissolved macro-nutrients (nitrate and phosphate) were estimated using a spectrophotometer (PerkinElmer UV/VIS Spectrometer Lambda 25).
All glassware and culture media used for analyses were autoclaved at 121°C, 15 lbs pressure for 15 min. The standard plate count method was used for the enumeration of bacterial community (Aneja, 2007). Isolation of bacterial strains from water samples was performed on nutrient agar media using the serial dilution plate technique. Serial dilutions in the range of 10–1, 10–2, 10–3, 10–4, 10–5, and 10–6 were carried out. Total bacterial density in terms of colony forming unit (CFU ml−1) was counted after 24 ± 1.5 h incubation at 37°C. After incubation, the colonies representing different morphologies were picked up and further sub-cultured in the same medium to get pure isolates and were stored at 4°C for further studies. For sequencing and characterization (phylogenetic analysis), the bacterial strains with 50%–70% occurrence were isolated and cultured (Böttger, 1989; Aneja, 2007).
The TC concentration in wastewater was determined by technique described by Aneja (2007), using multiple fermentation tubes. This technique involved three steps as follows:
MacConkey broth Purple, a widely used differential medium for the determination of coliforms in water samples, was used. Tests were conducted in sets—the Ist set consisted of three double-strength test tubes while the remaining two sets consisted of six single-strength test tubes containing sterilized MacConkey broth and inverted Durham tubes. Inoculating the test tubes with 10, 1, and 0.1 ml of wastewater, respectively, we incubated them at 37°C and observed them after 24 and 48 h of the incubation period. Production of acid and gas in Durham tubes was indicative of positive for coliform in the presumptive test.
Furthermore, an eosin methylene blue (EMB) agar plate was used to eliminate false positives from other heterotrophic organisms. We incubated EMB agar plates for 24 ± 1.5 h at 37°C after streaking with a loopful from each test tube that was found to be positive in the presumptive test.
MacConkey broth tubes and nutrient agar slants were prepared and inoculated with distinct colonies obtained on EMB agar plates. The production of acid and gas production was observed after incubation for 24 h at 37°C similar to the presumptive test. Last, gram-staining of isolates on agar slants was performed following the standard technique (Aneja, 2007).
Zooplankton samples were collected in five replicates using a nitex plankton net (mesh size of 53 μm) and preserved in 4% (w/v) buffered formalin in a glass bottle (vol 100 ml) immediately after collection. In the laboratory, zooplankton samples were segregated and identified under a compound microscope (Olympus CX21) using standard keys (Michael and Sharma, 1988; Edmondson, 1992; Sharma, 1992; Dumont, 1994; Fernando, 2002 and other revisional references). The numbers per liter of each genus were quantified and calculated using the following formula:
where N denotes the number of plankton per liter, “A” is the average number of plankton in all counts in a counting cell of 1 mm3 capacity, “C” is the volume of original concentrate in ml, and “L” is the volume of total water filtered, expressed in liter.
The screening of macrophytes was based on dominant species and high phytoremediation capacity among dominant species. The stretch of approximately 1.5 km from Antaghat to Kalighat, Patna, Bihar, was sampled monthly from November 2018 to May 2019 by randomly placing a quadrate (5 m × 5 m) along the drain. This area is subjected to anthropogenic activities such as discharge of untreated sewage, agriculture practice, construction work, and grazing for cattle. Standard identification keys Cook (1996), Biswas et al. (2013), Fassett (2006), and Subramanyam (1962) were used for identification.
The most dominant bacterial strain occurring in maximum samples was isolated and cultured for further characterization. DNA isolation of bacterial strain KG1 was performed and the DNA quality was examined on 1.2% agarose gel. A total of 16S rRNA-specific primers (8F and 1492R) were used for the amplification of isolated DNA using a Verity® 96-Well Thermal Cycler (model number 9902) (Böttger, 1989). The PCR process yields a single discrete amplicon band of 1,500 bp (Figure 2). The PCR product was further purified enzymatically before being subjected to Sanger sequencing. On the ABI 3730xl Genetic Analyzer, a bi-directional DNA sequencing reaction of PCR product was performed by M13F and M13R primers using the BDT v3.1 Cycle sequencing kit. Using aligner software, a consensus sequence of 1,484 bp of 16S rRNA was generated from forward and reverse sequence data (Harmsen, 2004). The generated raw sequence data were submitted to NCBI (National Center for Biotechnology Information), a public repository under the accession number–ON844181 (https://www.ncbi.nlm.nih.gov/nuccore/ON844181.1/).
FIGURE 2. 1.2% Agarose gel showing single 1500 bp of 16S rDNA amplicon. Lane 1: 100bp DNA ladder; Lane 2: 16S rDNA amplicon..
The relationship between the isolated strain and the other 10 species based on similarity was analyzed using phylogenetic trees based on evolutionary distance data of 16S rRNA gene sequences. The neighbor-joining method was used to analyze the evolutionary history of the species (Saitou and Nei, 1987). Furthermore, evolutionary distances between the species were calculated using the maximum likelihood method following Tamura et al. (2004) and were represented in units of the number of base substitutions per site. The coding positions involved were first + second + third + non-coding. Pairwise deletion was performed for each sequence pair to remove all the ambiguous positions in the analysis. A total of 1,575 positions were present in the final dataset. Mega X software was used for the phylogenetic analysis (Kumar et al., 2018).
The two species of zooplankton: Cladocera–M. macrocopa and Rotifera–B. angularis, were frequently recorded in the time series study. These two species were isolated from the zooplankton samples collected at Kalighat. Cultures were developed following the methods by Kumar and Hwang (2008). The organisms were isolated in a glass cavity block with a 10 ml medium including Chlorella vulgaris as food. The culture medium was changed daily. As the number of M. macrocopa. and B. angularis increased, they were transferred to a larger volume of medium containing natural water that was progressively changed to tap water. To elucidate the survival and population growth potential of M. macrocopa and B. angularis in wastewater, the population growth rate was followed. The required number of neonates was obtained from the ovigerous females, which were acclimatized to the wastewater for 2 days prior to the experiment. The experimental design consisted of triplicate sets (n = 3) of control and treatments. Each experimental beaker contained 100 ml medium with C. vulgaris, concentrated from the mass culture and resuspended to obtain 2.5 × 106 cells/mL in a 150 ml beaker containing 100 ml of solution in control and each treatment with wastewater. In each control and treatment beaker, 20 neonate individuals (age<8 h) of both B. angularis and M. macrocopa were introduced. At 24 ± 1.5 h intervals, the total number of live individuals was enumerated and transferred to a fresh medium containing 100 ml of wastewater and placed at 25 ± 2°C temperature. Daily observations were continued till asymptote was observed in the population growth. The population growth rate (r) was calculated from the exponential phase of population growth using the equation.
where N0 is the initial population density and Nt is population density after time t.Where the population growth curve showed crests and troughs, we used an average of r values calculated using different intervals of time for 8–12 days during which the stable age distribution was assumed to have been attained.
To estimate the bactericidal potential of M. macrocopa and B. angularis in the wastewater, the heterotrophic plate count (HPC) and total coliform count (TCC) using the most probable number (MPN) in control and treatments were performed at 24 ± 1.5 h intervals following the protocol prescribed in APHA (2012).
The CW was built near the Antaghat sewage drain (latitude 37°30N and longitude 127°30E). In the present study, CW (Figure 3) was developed as a free water surface system, in which the surface of the water, flowing through the system is exposed to the atmosphere (Tousignant et al., 1999). Table 2 explains the morphometric details, structural components, their dimension, and purpose of various structures of CWS. The Antaghat sewage drain discharged approximately 30 MLD of sewage water per day and was used as an influent for the CW, composed of domestic wastewater and urban runoff. This CWS consisted of an oxidation tank of 236ft3, a treatment tank of 75 ft3, and finally the constructed wetland with six ridge–furrows in an area of 7 ft × 15 ft (Figure 3).
FIGURE 3. Photograph of CWS, (A) Photograph of CWS, showing inlet, outlet and stages during plantation; (B) schematic presentation of media in treatment bed and Morphometric details with ridges and riverbed pebbles used at Antaghat wastewater discharge site at the southern bank of the River Ganges at Patna, Bihar.
Each pair of ridges contains river pebbles of different sizes (diameters 1″, 2″, and 3″). The furrows were planted with Typha, Phragmites, and Cyperus spp. To simulate riverbeds, riverbed pebbles were placed into the treatment tank. To create turbulence for the mixing of atmospheric oxygen with the sewage water, the loose stone barrier was constructed between the oxidation and treatment tanks. Structural details and operational parameters are described in Table 2.
To maintain a constant flow of sewage, a steel-made cylindrical regulator (mouth diameter–4 ft) was installed and connected with a 9” PVC pipe to adjust the inlet valve. The flow rate was measured using a flow meter (Model FP111; Global water a xylem brand).
From March 2020, sewage effluent at Antaghat sewage drainage was percolated through CWS maintaining 0.28 to 0.80 MLD flow rates. Retention times varied from 24 to 50 h. Influent and effluent water samples were collected from CWS every day and analyzed.
The selected macrophytes—Typha, Phragmites, and Cyperus were collected from the surrounding area and were planted in the furrow beds. The macrophytes were allowed to establish themselves in the CW after the ridge, groove, and beds were filled with sewage water up to 10 cm above the bottom. After 15 days of plantation, an inoculum of B. angularis and M. macrocopa from laboratory culture was applied in the treatment tank. Finally, after 2 months of plantation, the CWS was made operational and raw wastewater was passed through the CWS by regulating the sewage flow.
To elicit the strength of association between pollution parameters (BOD, bacterial count, and nutrient level) and species occurrence, a correlation matrix was computed using Pearson’s product–moment correlation. All the time series data for 36 sampling events were subjected to Bray–Curtis similarity analysis. The species abundance data were square-root transformed to obtain the symmetric normal distribution for constructing the Bray–Curtis similarity matrix with average linkage group classification (Field et al., 1982). Using indicator species analysis (Dufrêne and Legendre, 1997; Nesemann et al., 2017), highly indicative species were identified for the cluster recording heavy pollution load.
For analysis of macrophyte occurrence, the ecological indices, namely, (i) relative frequency (RF), (ii) relative dominance (RD), (iii) relative abundance (RA), and (iv) important value index (IVI) were calculated (Dash et al., 2020).
A genus can have a maximum importance value of 300 (100 + 100 + 100). This method provides an idea of how common a species is in a certain area, as well as how widespread it is within that area (Ghosh and Biswas, 2015).
To identify significant differences in population growth rates of M. macrocopa and B. angularis between wastewater treatment and control, the rates of population increase (r) were subjected to one-way ANOVA. Prior to the ANOVA test, the population growth rate data were arc sin transformed to reduce heteroscedasticity. All the data analyses were carried out using Microsoft excel and PRIMER-VI.
Table 3 provides average values of water quality parameters estimated at the time series (TS) station and the values estimated for the wastewater at the mouth of the sewage drain, discharged into the River Ganga at Antaghat, Patna. The total dissolved solids varied from 120 to 598 mgL−1 at the TS station, whereas it showed from 344 to 507 mgL−1 values in the wastewater. The dissolved oxygen (DO) concentration was never detected from the wastewater, which ranged from 4.1 to 7.7 mg L−1 at the TS station. BOD values varied from 79 to 90 mgL−1 in the wastewater before discharge which was found to be in the range of 0.9–3.8 mg L−1 at the TS station. The cluster analyses for hydrographic parameters (Figure 4A) segregated samples on the basis of bacterial and total coliform concentrations. The samples recording higher bacterial and coliform concentrations were grouped together (mainly from August to December post-monsoon samples) and segregated at the first hierarchical level. The samples with higher BOD values also join this cluster at a 40% similarity level. The top two highly indicative zooplankton species for this cluster are Brachionidae rotifers and Moinidae cladocerans.
TABLE 3. Average values of selected parameters analyzed at the time series site and wastewater discharge point (Antaghat).
FIGURE 4. Results of time series study for screening of biopurifiers (A) Hierarchical dendrogram of 36 samples identified by the Bray Curtis cluster analysis for water quality parameters; (B) Relative abundance and occurrence frequency of 14 highly abundant zooplankton species, collected at time series study site (Kalighat) in the River Ganges during June 2016 to March 2019 in the vicinity of the sewage drain mouth.
The total bacterial density CFU ml−1 varied from 7.8 to 4,000 × 103 CFU ml−1 and the total coliform concentration varied from 2,600 to 86,000 MPN ml−100 from June 2016 to March 2019. The estimated fecal coliform counts at the TS station were above the acceptable level of 500 and the maximum permissible limit of 2,500 MPN (most probable number) per 100 ml (CPCB, 2017). In general, post-monsoon (August to December samples) recorded higher bacterial and coliform counts.
The most abundant strain frequently recorded from the wastewater discharge site was characterized as Fictibacillus phosphorivorans on the basis of 16S rRNA gene sequencing.
The KG1 strain analysis involved a total of 11 nucleotides including query sequences with codon positions including first+second+third + non-coding. The phylogenetic tree with the sum of branch length = 0.7658 is illustrated in Figure 5. The majority of the branches contained 10 reference/type strains corresponding to named species (Bacillus cucumis, Bacillus niacin, Bacillus macauensis, Bacillus megaterium, Bacillus tianshenii, Bacillus cohnii, Bacillus thuringiensis, Bacillus cereus, Anoxybacillus caldiproteolyticus, and Salirhabdus euzebyi strains) that are located in different branches but are genotypically related. On the basis of the sequence similarity score, the present strain KG1 (Fictibacillus phosphorivorans), is separated on 93% of similarity from Fictibacillus macauensis, Bacillus niacin, and Bacillus cucumis strains (Figure 5).
FIGURE 5. Molecular Phylogenetic tree of KG1 strain (A) Fictibacillus phosphorivorans with ten other bacterial strain and (B) most dominant bacterial strain Fictibacillus phosphorivorans isolated from time series study site in the River Ganges at Patna, Bihar.
Integrating all the 36 samples, 31 taxa of zooplankton were identified from the TS station. Rotifera dominated the zooplankton community followed by Cladocera and Copepoda. The bloom period of rotifers coincided with a lower coliform count (Figure 6) followed by higher copepod concentrations. The most abundant taxa occurring in maximum samples were B. angularis among Rotifera and M. macrocopa among Cladocera (Figure 4B).
FIGURE 6. Percent composition of Rotifera, Cladocera and Copepoda with total coliform and bacterial density recorded during June 2016 to March 2019 in the vicinity of the sewage drain mouth at Kalighat Patna.
Table 4 records pairwise multiple correlations among abiotic and biotic parameters analyzed for the TS station. Dissolved oxygen also correlated negatively with bacteria CFU (R = −0.86; P = 0.0001) and total coliform (Table 4: R = −0.93; P = 0.0001). Rotifer zooplankton correlated negatively with the nitrate level (R = −0.56; P= 0.02) and bacterial (CFU) concentrations (R = −0.48; P= 0.03). Cladocera (mainly M. macrocopa) recorded a significant negative correlation with bacteria CFU (R = -0.40; Table 4).
TABLE 4. Pairwise multiple correlations using Pearson correlations (R) among zooplankton major groups (Rotifera, Copepoda, and Cladocera), with biotic (bacterial density CFU, total coliform, and Chl a) and abiotic parameters (pH, EC, temperature, BOD, DO, TDS, chloride, alkalinity, nitrate, and phosphate). *p < 0.1, **p < 0.05, and ***p < 0.01.
We recorded 24 genera of macrophytes between the Antaghat and Kalighat floodplain, affected by the sewage canal near the discharge site (Figure 7). The top 10 macrophyte species (Table 5) showing higher IVI values are known for their phytoremediation properties. Based on important value index values, we identified dominant macrophyte taxa. The top three dominant genera, namely, Typha, Phragmites, and Cyperus were selected for the present study in the constructed wetland.
FIGURE 7. Macrophytes with mean Importance Value Index (IVI) recorded at wastewater affected floodplain between Antaghat and Kalighat during Nov 2018 to May 2019.
TABLE 5. Dominant macrophytes species found in the study area and their mean Important Value Index (IVI) with their potential for phytoremediation properties.
Both the zooplankton species realized their higher population growth potential in sewage water without any external nutrient source (Figures 8A,B). In the case of smaller rotifer, B. angularis, the population growth followed an increasing trend with an average growth rate (r-value) of 0.26 as compared to control (autoclave tap water with C. vulgaris as food) with a r-value of 0.23. After day 11, an exponential increase in the population of B. angularis was observed in wastewater treatment (Figure 8A). The population growth of cladoceran, M. macrocopa showed a peak at sixth day in wastewater medium with a maximum population size of 36 with the average r-value of 0.154 in the observational period. B. angularis showed a higher population growth rate and faster development than M. macrocopa in the wastewater medium. The present results suggest that both the species can be harvested from the constructed wetlands for larval culture in the aquaculture industry.
FIGURE 8. Population growth trajectories of (A) B. angularis and (B) M. macrocopa in wastewater and in control with C. vulgaris as food.
In the treatment with B. angularis, 8% reduction in the HPC and 22% reduction in the MPN count was recorded from the control (Figures 9A,B), whereas in M. macrocopa treatment, HPC and MPN count decreased, respectively, by 28 and 21% (Figures 9C,D).
FIGURE 9. Bactericidal potential of Gangetic biofilters in wastewater. B. angularis driven bacterial population decline in (A) HPC & (B) TCC. M. macrocopa driven bacterial population decline in (C) HPC and (D) TCC. (HPC, Heterotrophic Plate count; TCC, Total Coliform Count).
The constructed wetland with the integrated application of zooplankton, namely B. angularis and M. macrocopa, and macrophytes, namely Typha sp., Phragmites sp., and Cyperus sp. yielded up to 92% reduction in BOD (Figure 10A) and up to 56% reduction in the total bacterial counts (Figure 10B) with a substantial increase in DO (Figure 10C) from the undetectable level.
FIGURE 10. Purification potential of CWS (A) Biological Oxygen Demand (BOD) concentration in the inlet and outlet site, and percentage reduction in BOD at outlet of CWS, (B) Total bacterial count in the inlet and outlet site of the CWS and percentage reduction in bacterial density at outlet of CWS; and (C) Dissolve Oxygen concentration in the inlet and outlet.
Further population growth rates of B. angularis and M. macrocopa were significantly higher in the wastewater system and higher biomass was obtained from the treatment pond. Both the zooplankton species are preferred live food for the rearing of fish larvae.
Water, being the elixir of life and a vehicle of sustainable development, is the foundation of various ecosystem services (UNEP, 2009). The use of water resources and subsequent discharge by humans degrade the service provided by the lotic and lentic ecosystems. Unplanned and unregulated discharges of untreated or partially treated sewage and industrial effluents combined with agricultural runoff have been degrading the quality of all sources of water around the world (Noguera-Oviedo and Aga, 2016). The data presented in this study convincingly point to the ecological way of treating the wastewater and recycling of nutrients that are converted to biomass, useful for the aquaculture industry. Recycled resources from waste are likely to reduce the cost of treatment, and can also be a smart option for disposal. The natural ecological approaches for treatment before the discharge of wastewater and harvest of biota for industrial applications are the viable solutions for low-cost treatment of sewage, reclamation of nutrients from it, and protection of river ecosystems from sewage-mediated degradation. The use of wastewater for irrigation, aquaculture, and other agricultural activities is an age-old practice in many parts of India and Africa. In these regions, contaminated water is often the only source of water for agriculture. In small-scale farming systems, wastewater is often preferred because of the high content of nutrients, which often eliminates the need for other expensive fertilizers (UNICEF-WHO, 2017). However, the recycling of wastewater in aquaculture and agriculture might be a key risk to the community health, ecology, soil, and groundwater contamination because of chemical and microbial contaminants. The present study has used naturally occurring plant genera: Typha, Phragmites, and Cyperus spp. These plants have been used in phytoremediation to treat different types of wastewaters. They have a high potential to bioaccumulate various nutrients, heavy metals, and micropollutants (Table 5). These macrophytes have limited ability to reduce the bacterial load from the wastewater and might attract insect vectors and spread vector-borne disease (Kumar and Hwang, 2006). Several constraints of constructed wetlands such as unpredictability of biomass production, higher land requirement, creation of habitat for insect (mosquito) pests, and sensitivity to pathogenic bacteria can be solved by using proper species such as macrophytes, adapted to local conditions and bacterivorous zooplankton such as Brachionus and Moina used in the present study and other larvicidal zooplankton as copepods (Kumar et al., 2008). As a result, the use of integrated wastewater systems with biological filters appears to be a viable future wastewater treatment option. Bacteria, fungi, algae, protozoa, gastropods, and zooplankton all use macrophytes as a shelter (US EPA, 2021). These naturally occurring organisms, which live within and outside plant parts or in water, contribute to the elimination of pollutants (Ijaz et al., 2016). Some zooplankton species are able to utilize bacteria as food source and regulate bacterial density in the natural system (Kumar and Hwang, 2008) whereas many species of copepod zooplankton are larvivorous, and being used in operational vector control programs in many counties (Kocher et al., 2018; Ranathunge et al., 2019; Nunes-Silva et al., 2020). They are able to control mosquito immatures even in the presence of alternative food sources (Kumar and Rao, 2003; Kumar and Hwang, 2006; Kumar et al., 2008). According to a recent study, a single biological treatment does not reduce effluent loads sufficiently (Wang et al., 2018), but integrated wastewater treatment systems with biological filters perform better. The use of particular microbes in association with specific macrophytes in constructed wetlands is a relatively new method of improving the system’s pollutant removal effectiveness (Keizer-Vlek et al., 2014; Ladislas et al., 2015). The population growth patterns recorded in this study for B. angularis and M. macrocopa under the controlled laboratory condition exclusively on resources from the wastewater strongly suggest the use of these zooplankton species along with macrophytes in the constructed wetlands to improve the treatment potential.
In constructed wetlands, BOD removal can be attributed to decomposing microorganisms, primarily by aerobic bacteria clinging to pebbles and plant roots and the physical and biological processes of sedimentation in the ridge-furrow region. With respect to the initial result and subsequent improvement in BOD removal (i.e., <92%), the performance of the present CWS seems promising and can be put for an operational treatment program after optimization. Unlike in the present case, several authors have setup gravel-based subsurface-flow systems (Ansola et al., 1995; Naylor et al., 2003; Brix and Arias, 2005) where the performance in terms of organic pollutant removal was better in planted beds as compared to similar unplanted beds. As in the present case, macrophytes provide a suitable habitat for various microorganisms in the rhizosphere that play an essential role in reducing organic matter in several types of wastewater.
The use of particular zooplankton species in association with specific macrophytes in the constructed wetland is a relatively new method of improving the system’s pollutant removal efficiency (Keizer-Vlek et al., 2014; Ladislas et al., 2015). Natural wetlands and lagoons necessitate a large area of land and might be challenging to maintain year-round discharge standards (Massoud et al., 2009; Young et al., 2017; Lutterbeck et al., 2018). However, a constructed wetland (CW) requires less area and can incorporate macrophytes and zooplankton efficiently, so it can be a preferred biological treatment system. CW can be installed at wastewater discharge sites and duly screened bio-purifiers can be used as a starter inoculum of bio-purifiers for the efficient functioning of the CW. Depending on the volume of wastewater, niche requirements of chosen bio-purifiers, and availability of land area, appropriate types of wetlands can be constructed.
The use of resources from wastewater adopting an ecological approach and treatment of wastewater prior to discharge or reuse would reduce the health and ecosystem risk. CWS technologies using indigenous plants to remove contaminants and using plankton to remove suspended particles and pathogens in the water column have potential to minimize the risk, treat the wastewater, control flood, and provide food and fiber through agriculture, aquaculture with an added opportunity to develop the landscape for compensation of the natural landscape transferred toward developmental activities (Hua, 2003). The CWS, when compared to other types of water treatment facilities, has lower maintenance costs, contains natural elements (bacteria and plants) and uses renewable energy sources (solar and kinetic) instead of high-tech methods, and has the ability to process high volumes of wastewater containing a variety of contaminants (Hua, 2003). The CWS was developed at the Neela Hauz and Kalindi Biodiversity Park, New Delhi, India, to treat sewage water 1 million liter per day before entering the lake which functions through oxidation ponds, gradient channels and physical treatment tanks, and finally, the constructed wetland consisting of consecutive ridges of pebbles of various sizes and furrows with many species of plants (Delhi Development Authority, 2021). However, optimization of CWS using selected species of filter feeders and time series study to screen locally adapted species have not been applied yet. For the first time, the present study uses species of water column dwellers zooplankton and wastewater adapted species of emergent macrophytes in the CWS.
The present results unequivocally suggest that the species of bacterivorous zooplankton—cladocerans and rotifers can utilize resources from wastewater conditions, and can realize the maximum growth potential in absence of competitors, predators, or any external nutrient source. Large populations of planktonic bacterivorous and particulate feeder organisms in the water column can rapidly eliminate bacterial biomass and reduce BOD (Saidin, 2016; Montemezzani et al., 2017). So, the present results convincingly suggest that both the zooplankton and macrophyte species used, herein, have the potential to regulate the density of the harmful microorganism and to remove the contaminants from the wastewater in a constructed wetland.
The present investigation demonstrates that the macrophyte–zooplankton CWS can be a sustainable, ecologically viable, and cost-effective method of removing BOD, bacterial load, other organic matter, ammoniacal nitrogen, and phosphorus from the wastewater. The results obtained, herein, point to the effectiveness of the system and specific inferences are made: (i) a higher removal of BOD and bacterial concentration was registered within 4 days of treatment. (ii) Bacterial and BOD removal is mainly performed by particulate feeder and bacterivorous zooplankton and emergent macrophyte assimilation. (iii) The aquaculturally relevant zooplankton biomass can be harvested after sixth day for Cladocera and after 11th day for Rotifera. (iv) The wastewater treated through the CWS can be recycled for irrigation, aquaculture, and washing. The CWS with integrated use of macrophytes and zooplankton has several benefits: (i) smaller area required, (ii) lower operational and installation costs, (iii) reclamation of abandoned and/or degraded floodplain, (iv) possibility of on-site treatment by farmers and owners of livestock farms for slurry treatment, (v) safe to reuse treated water for agriculture, aquaculture, and washing, and (vi) higher dissolved oxygen concentration in the water without the need of a mechanical aerator. The CWS is a nature-based system with zooplankton and macrophytes. Given its high aesthetic value and low maintenance requirement, it is a unique alternative for isolated communities. Therefore, the present results suggest an integrated use of rotifer, cladoceran zooplankton, and macrophytes for the treatment of wastewater, reduction of sludge, and harvest of live feeds for the aquaculture industry to augment circularity and promote decentralized wastewater treatment.
The original contributions presented in the study are included in the article/Supplementary Material; further inquiries can be directed to the corresponding author.
RK and FK conceived the concept. DP and RD designed the protocol, collected literature, and established cultures of species from sewage affected area. DY, AP, and KR performed the experiment in constructed wetland and performed time series study of macrophytes. DP performed the time series study in the vicinity of wastewater discharge area. RK, RD, and FK worked for interpretation of results, critically provided feedback, and edited the manuscript. All the authors approved submission of the manuscript.
Financial support was provided by the Department of Biotechnology (BT/PR20543/BCE/8/1398/2016).
We are thankful to the Department of Biotechnology for the project (BT/PR20543/BCE/8/1398/2016) to RK under river cleaning programme. DP thanks UGC for a National Fellowship. We are thankful to Yasir Arafat of Biodiversity Parks programme of CEMDE, University of Delhi for providing photography and useful discussions.
The authors declare that the research was conducted in the absence of any commercial or financial relationships that could be construed as a potential conflict of interest.
All claims expressed in this article are solely those of the authors and do not necessarily represent those of their affiliated organizations, or those of the publisher, the editors, and the reviewers. Any product that may be evaluated in this article, or claim that may be made by its manufacturer, is not guaranteed or endorsed by the publisher.
The Supplementary Material for this article can be found online at: https://www.frontiersin.org/articles/10.3389/fenvs.2022.941841/full#supplementary-material
Adhikari, S., Goswami, A. R., and Mukhopadhyay, S. K. (2017). Diversity of zooplankton in municipal wastewater-contaminated urban pond ecosystems of the lower Gangetic plains. Turk. J. Zool. 41 (3), 464–475. doi:10.3906/zoo-1601-12
Aneja, K. R. (2007). Experiments in microbiology, plant pathology and biotechnology. NewAge Int. 58.
Ansola, G., Fernandez, C., and De Luis, E. (1995). Removal of organic matter and nutrients from urban wastewater by using an experimental emergent aquatic macrophyte system. Ecol. Eng. 5 (1), 13–19. doi:10.1016/0925-8574(95)00017-D
Avio, C. G., Gorbi, S., and Regoli, F. (2017). Plastics and microplastics in the oceans: from emerging pollutants to emerged threat. Mar. Environ. Res. 128, 2–11. doi:10.1016/j.marenvres.2016.05.012
Biswas, R., Das, A. P., and Paul, T. K. (2013). Floristic diversity of Rasik Beel and its adjoining areas in Coochbehar district of West Bengal. India.
Böttger, E. C. (1989). Rapid determination of bacterial ribosomal RNA sequences by direct sequencing of enzymatically amplified DNA. FEMS Microbiol. Lett. 65 (1-2), 171–176. doi:10.1016/0378-1097(89)90386-8
Boucher, J., and Friot, D. (2017). Primary microplastics in the oceans: a global evaluation of sources, 10. Gland, Switzerland: IUCN.
Brix, H., and Arias, C. A. (2005). Danish guidelines for small-scale constructed wetland systems for onsite treatment of domestic sewage. Water Sci. Technol. 51 (9), 1–9. doi:10.2166/wst.2005.0275
Burnet, J. B., Faraj, T., Cauchie, H. M., Joaquim-Justo, C., Servais, P., Prévost, M., et al. (2017). How does the cladoceran Daphnia pulex affect the fate of Escherichia coli in water? PloS one 12 (2), e0171705. doi:10.1371/journal.pone.0171705
Capodaglio, A. G. (2017). Integrated, decentralized wastewater management for resource recovery in rural and peri-urban areas. Resources 6 (2), 22. doi:10.3390/resources6020022
CPCB, (2018). Observed Variation in Flow and Organic Load of Priority Drains monitored during Pre and Post Monsoon, 2018 (Discharged into Ganga) Bihar, Phase-III (UP Border to Jharkhand) Central Pollution Control Board, 6.
CPCB, (2021). Status of STPs. Available at: https://cpcb.nic.in/status-of-stps/.
Damania, R., Desbureaux, S., Rodella, A. S., Russ, J., and Zaveri, E. (2019). Quality unknown: the invisible water crisis. Washington, D.C: World Bank Publications.
Dash, A. K., Upadhyay, V. P., and Patra, H. K. (2020). Floristic assessment of semi evergreen forests of a peripheral site in Hadagarh Sanctuary, Odisha, India. International Journal of Biodiversity and Conservation, 12 (2), 104–112. doi:10.5897/ijbc2019.1386
Delhi Development Authority (2021). “Neela Hauz biodiversity Park (page no. 177),” in Biodiversity Parks: Nature reserves of Delhi. E-bbok published (New Delhi: Public Relations Department, Delhi Development Authority), 267.
Diamond, J. S., and Cohen, M. J. (2018). Complex patterns of catchment solute–discharge relationships for coastal plain rivers. Hydrol. Process. 2 (3), 388–401. doi:10.1002/hyp.11424
Dufrêne, M., and Legendre, P. (1997). Species assemblages and indicator species: the need for a flexible asymmetrical approach. Ecol. Monogr. 67 (3), 345–366. doi:10.2307/2963459
Dumont, H. J. (1994). On the diversity of the Cladocera in the tropics. Hydrobiologia 272, 27–38. doi:10.1007/bf00006510
Ebert, D. (2005). Ecology, epidemiology, and evolution of parasitism in Daphnia. Bethesda, MD: National Library of Medicine.
Edmondson, W. T. (1992). “Freshwater biology, wipple and ward reprint (Indian reprint),” in International books & periodicals supply service, 24B/5 (Karolbagh New Delhi.
Farahat, E. A., and Galal, T. M. (2018). Trace metal accumulation by Ranunculus sceleratus: implications for phytostabilization. Env. Sci. Poll. Resea. 25 (5), 4214–4222. doi:10.1007/s11356-017-0808-2
Fernando, C. H. (2002). Leiden, Netherlands: Backhuys Publishers.A guide to tropical Freshwater Zooplankton, Identification, ecology and impact on fisheries
Field, J. G., Clarke, K. R., and Warwick, R. M. (1982). A practical strategy for analysing multispecies distribution patterns. Mar. Ecol. Prog. Ser. 8, 37–52. doi:10.3354/meps008037
Gaballah, M. S., Ismail, K., Aboagye, D., Ismail, M. M., Sobhi, M., and Stefanakis, A. I. (2021). Effect of design and operational parameters on nutrients and heavy metal removal in pilot floating treatment wetlands with Eichhornia Crassipes treating polluted lake water. Environ. Sci. Pollut. Res. 28 (20), 25664–25678. doi:10.1007/s11356-021-12442-7
Ghosh, D., and Biswas, J. K. (2015). Macroinvertebrate diversity indices: A quantitative bioassessment of ecological health status of an oxbow lake in eastern India. J Adva. Envi. Heal. Rese. 3 (2), 78–90.
Gliwicz, Z. M., and Wrzosek, D. (2008). Predation-mediated coexistence of large and small-bodied Daphnia at different food levels. Am. Nat. 172 (3), 358–374. doi:10.1086/589890
Hamad, M. T. (2020). Comparative study on the performance of Typha latifolia and Cyperus Papyrus on the removal of heavy metals and enteric bacteria from wastewater by surface constructed wetlands. Chemosphere 260, 127551. doi:10.1016/j.chemosphere.2020.127551
Harmon, S. M., and Wiley, F. E. (2010). Effects of pollution on freshwater organisms. Water Environ. Res. 82 (10), 1945–2000. doi:10.2175/106143010x12756668802139
Hofstra, N., and Vermeulen, L. C. (2016). Impacts of population growth, urbanisation and sanitation changes on global human Cryptosporidium emissions to surface water. Int. J. Hyg. Environ. Health 219 (7), 599–605. doi:10.1016/j.ijheh.2016.06.005
Hua, S. C. (2003). The use of constructed wetlands for wastewater treatment. Selangor, Malaysia: Wetlands International—Asia Pacific.
Hunter, R. G., Day, J. W., Wiegman, A. R., and Lane, R. R. (2019). Municipal wastewater treatment costs with an emphasis on assimilation wetlands in the Louisiana coastal zone. Ecol. Eng. 137, 21–25. doi:10.1016/j.ecoleng.2018.09.020
Ijaz, A., Imran, A., Anwar ul Haq, M., Khan, Q. M., and Afzal, M. (2016). Phytoremediation: recent advances in plant-endophytic synergistic interactions. Plant Soil 405 (1), 179–195. doi:10.1007/s11104-015-2606-2
IUCN (2020). Freshwater and water security. Available at: https://www.iucn.org/theme/water/about/facts-and-figures.
IUCN (2013). IUCN annual report. Available at: https://portals.iucn.org/library/efiles/documents/IUCN-2014-017.pdf.
Jafari, N., Nabavi, M. S., and Akhavan, M. (2011). Ecological investigation of zooplankton abundance in the river Haraz, northeast Iran: Impact of environmental variables. Arch. Biol. Sci. 63 (3), 785–798. doi:10.2298/ABS1103785J
Jha, P., Jobby, R., and Desai, N. S. (2016). Remediation of textile azo dye acid red 114 by hairy roots of Ipomoea carnea Jacq. and assessment of degraded dye toxicity with human keratinocyte cell line. J. Haz. Mat. 311, 158–167. doi:10.1016/j.jhazmat.2016.02.058
Kampf, R., van der Geest, H., Sala, L., Romani, A., Comas, J., Claassen, T., et al. (2007). “Biological filtration of treated waste water by Daphnia: an alternative for technical filtration, or an addition?,” in Proceedings of the IWA 6th conference on wastewater reclamation and reuse for sustainability.
Kataki, S., Chatterjee, S., Vairale, M. G., Dwivedi, S. K., and Gupta, D. K. (2021). Constructed wetland, an eco-technology for wastewater treatment: A review on types of wastewater treated and components of the technology (macrophyte, biolfilm and substrate). J. Environ. Manage. 283, 111986. doi:10.1016/j.jenvman.2021.111986
Keizer-Vlek, H. E., Verdonschot, P. F., Verdonschot, R. C., and Dekkers, D. (2014). The contribution of plant uptake to nutrient removal by floating treatment wetlands. Ecol. Eng. 73, 684–690. doi:10.1016/j.ecoleng.2014.09.081
Khalid, N., Noman, A., Aqeel, M., Masood, A., and Tufail, A. (2019). Phytoremediation potential of Xanthium strumarium for heavy metals contaminated soils at roadsides. Int. J. Environ. Sci. Technol. (Tehran). 16 (4), 2091–2100. doi:10.1007/s13762-018-1825-5
Khamparia, S., and Jaspal, D. K. (2017). Evaluation of decoloration potential of Xanthium Strumarium seed hull for adsorption of Direct red 81 in aqueous solution. Environ. Dev. Sustain. 19 (5), 1933–1951. doi:10.1007/s10668-016-9836-1
Kocher, D. K., Jamwal, S., and Kaur, D. (2018). Mosquito larvae specific predation by native cyclopoid copepod species. Daday: Mesocylops aspericornis.
Koutsou, O. P., Gatidou, G., and Stasinakis, A. S. (2018). Domestic wastewater management in Greece: Greenhouse gas emissions estimation at country scale. J. Clean. Prod. 188, 851–859. doi:10.1016/j.jclepro.2018.04.039
Kumar, R. (2003). Effects of Mesocyclops thermocyclopoides (Copepoda: Cyclopoida) predation on the population growth patterns of different prey species. J. Freshw. Ecol. 18 (3), 383–393. doi:10.1080/02705060.2003.9663974
Kumar, R., and Hwang, J. S. (2006). Larvicidal efficiency of aquatic predators: a perspective for mosquito biocontrol. Zool. Stud. 45 (4), 447.
Kumar, R., and Hwang, J. S. (2008). Ontogenetic shifts in the ability of the cladoceran, Moina macrocopa Straus and Ceriodaphnia cornuta Sars to utilize ciliated protists as food source. Int. Rev. Hydrobiol. 93 (3), 284–296. doi:10.1002/iroh.200711013
Kumar, R., Muhid, P., Dahms, H. U., Tseng, L. C., and Hwang, J. S. (2008). Potential of three aquatic predators to control mosquitoes in the presence of alternative prey: a comparative experimental assessment. Mar. Freshw. Res. 59 (9), 817–835. doi:10.1071/MF07143
Kumar, R., and Rao, T. R. (1999). Demographic responses of adult Mesocyclops thermocyclopoides (Copepoda, Cyclopoida) to different plant and animal diets. Freshw. Biol. 42 (3), 487–501. doi:10.1046/j.1365-2427.1999.00485.x
Kumar, R., and Rao, T. R. (2003). Predation on mosquito larvae by Mesocyclops thermocyclopoides (Copepoda: Cyclopoida) in the presence of alternate prey. Int. Rev. Hydrobiol. 88 (6), 570–581. doi:10.1002/iroh.200310631
Kumar, S., Stecher, G., Li, M., Knyaz, C., and Tamura, K. (2018). MEGA X: Molecular evolutionary genetics analysis across computing platforms. Mol. Biol. Evol. 35, 1547–1549. doi:10.1093/molbev/msy096
Kumari, M., and Tripathi, B. D. (2015). Efficiency of Phragmites australis and Typha latifolia for heavy metal removal from wastewater. Ecotoxicol. Environ. Saf. 112, 80–86. doi:10.1016/j.ecoenv.2014.10.034
Lacatusu, R., Lacatusu, A. R., Stanciu-Burileanu, M. M., Lazar, D. R., Lungu, M., Rizea, N., et al. (2012). Phytoremediation of a sludge depozit proceeded from a city wastewater treatment plant. Carpathian J. Earth Env. Sci. 7 (1), 71–79.
Ladislas, S., Gerente, C., Chazarenc, F., Brisson, J., and Andres, Y. (2015). Floating treatment wetlands for heavy metal removal in highway stormwater ponds. Ecol. Eng. 80, 85–91. doi:10.1016/j.ecoleng.2014.09.115
Langergraber, G., and Muellegger, E. (2005). Ecological sanitation—A way to solve global sanitation problems? Environ. Int. 31 (3), 433–444. doi:10.1016/j.envint.2004.08.006
Langis, R., Proulx, D., De la Noüe, J., and Couture, P. (1988). Effects of a bacterial biofilm on intensive Daphnia culture. Aquac. Eng. 7 (1), 21–38. doi:10.1016/0144-8609(88)90036-2
Lebreton, L., Van Der Zwet, J., Damsteeg, J. W., Slat, B., Andrady, A., and Reisser, J. (2017). River plastic emissions to the world’s oceans. Nat. Commun. 8 (1), 1–10. doi:10.1038/ncomms15611
Lutterbeck, C. A., Zerwes, F. V., Radtke, J. F., Köhler, A., Kist, L. T., and Machado, Ê. L. (2018). Integrated system with constructed wetlands for the treatment of domestic wastewaters generated at a rural property–evaluation of general parameters ecotoxicity and cytogenetics. Ecol. Eng. 115, 1–8. doi:10.1016/j.ecoleng.2018.01.004
Lyu, K., Cao, H., Chen, R., Wang, Q., and Yang, Z. (2013). Combined effects of hypoxia and ammonia to Daphnia similis estimated with life-history traits. Environ. Sci. Pollut. Res. 20 (8), 5379–5387. doi:10.1007/s11356-013-1555-7
Maceda-Veiga, A., Webster, G., Canals, O., Salvadó, H., Weightman, A. J., and Cable, J. (2015). Chronic effects of temperature and nitrate pollution on Daphnia magna: Is this cladoceran suitable for widespread use as a tertiary treatment? Water Res. 83, 141–152. doi:10.1016/j.watres.2015.06.036
Massoud, M. A., Tarhini, A., and Nasr, J. A. (2009). Decentralized approaches to wastewater treatment and management: applicability in developing countries. J. Environ. Manage. 90 (1), 652–659. doi:10.1016/j.jenvman.2008.07.001
Matamoros, V., Nguyen, L. X., Arias, C. A., Salvadó, V., and Brix, H. (2012). Evaluation of aquatic plants for removing polar microcontaminants: a microcosm experiment. Chemosphere 88 (10), 1257–1264. doi:10.1016/j.chemosphere.2012.04.004
Michael, R. G., and Sharma, B. K. (1988). Indian cladocera (Crustacea. Branchiopoda. Cladocera). Zool. Sur. Ind. 262.
Montemezzani, V., Duggan, I. C., Hogg, I. D., and Craggs, R. J. (2017). Screening of potential zooplankton control technologies for wastewater treatment High Rate Algal Ponds. Algal Res. 22, 1–13. doi:10.1016/j.algal.2016.11.022
Moteraghi, M., and Payandeh, K. (2021). Study of the efficiency of removal of lead and nickel metals from effluent using two species of latifolica and polygonum aviculare (case study: West ahwaz wastewater treatment plant). J. Water Wastewater 32 (4), 67–78. doi:10.22093/WWJ.2021.236815.3040
Müller, M. F., Colomer, J., and Serra, T. (2018). Temperature-driven response reversibility and short-term quasi-acclimation of Daphnia magna. PloS one 13 (12), e0209705. doi:10.1371/journal.pone.0209705
Mulugeta, M., and Lelisa, B. (2014). Removal of methylene blue (Mb) dye from aqueous solution by bioadsorption onto untreated Parthenium hystrophorous weed. Mod. Chem. Appl. 2 (4), 146. doi:10.4172/2329-6798.1000146
Naylor, S., Brisson, J., Labelle, M. A., Drizo, A., and Comeau, Y. (2003). Treatment of freshwater fish farm effluent using constructed wetlands: the role of plants and substrate. Water Sci. Technol. 48 (5), 215–222. doi:10.2166/wst.2003.0324
Nesemann, H. F., Sharma, G., Kumar, R., Sheetal, A., and Roy, S. (2017). Do the rivers of Chota Nagpur Dry Forests (Jharkhand), Damodar and Subarnarekha differ in biodiversity of aquatic macrozoobenthos, functional feeding groups and biological water quality? Aquat. Ecosyst. Health Manag. 20 (1-2), 116–129. doi:10.1080/14634988.2017.1300041
Noguera-Oviedo, K., and Aga, D. S. (2016). Lessons learned from more than two decades of research on emerging contaminants in the environment. J. Hazard. Mat. 316, 242–251. doi:10.1016/j.jhazmat.2016.04.058
Nunes-Silva, A., Lima-Keppe, I. D., Souza, R. V. D., and Perbiche-Neves, G. (2020). Survival of the copepod Mesocyclops longisetus during simulations of transport from hatchery to target areas for biological control of mosquito larvae. Zool. (Curitiba) 37, 1–4. doi:10.3897/zoologia.37.e37658
Okamoto, A., Yamamuro, M., and Tatarazako, N. (2015). Acute toxicity of 50 metals to Daphnia magna. J. Appl. Toxicol. 35 (7), 824–830. doi:10.1002/jat.3078
Oladoja, N. A. (2017). Appropriate technology for domestic wastewater management in under-resourced regions of the world. Appl. Water Sci. 7 (7), 3391–3406. doi:10.1007/s13201-016-0495-z
Parmar, T. K., Rawtani, D., and Agrawal, Y. K. (2016). Bioindicators: the natural indicator of environmental pollution. Front. Life Sci. 9 (2), 110–118. doi:10.1080/21553769.2016.1162753
Pau, C., Serra, T., Colomer, J., Casamitjana, X., Sala, L., and Kampf, R. (2013). Filtering capacity of Daphnia magna on sludge particles in treated wastewater. Water Res. 47 (1), 181–186. doi:10.1016/j.watres.2012.09.047
Ranathunge, T., Abeyewickreme, W., Iqbal, M. C. M., and Hapugoda, M. (2019). Use of cyclopoid copepods for control of Anopheles (Diptera: Culicidae) mosquito larvae to prevent re-emergence of malaria in Sri Lanka. J. Vector Borne Dis. 56 (3), 200. doi:10.4103/0972-9062.289393
Saidin, A. N. B. (2016). Water quality and zooplankton community structure of tembat river, hulu terengganu (doctoral dissertation). Pulau Pinang: Universiti Sains Malaysia.
Saitou, N., and Nei, M. (1987). The neighbor-joining method: a new method for reconstructing phylogenetic trees. Mol. Biol. Evol. 4, 406–425. doi:10.1093/oxfordjournals.molbev.a040454
Schlüter, M., and Groeneweg, J. (1985). The inhibition by ammonia of population growth of the rotifer, Brachionus rubens, in continuous culture. Aquaculture 46 (3), 215–220. doi:10.1016/0044-8486(85)90207-8
Schmidt, C., Krauth, T., and Wagner, S. (2017). Export of plastic debris by rivers into the sea. Environ. Sci. Technol. 51 (21), 12246–12253. doi:10.1021/acs.est.7b02368
Serra, T., Colomer, J., Pau, C., Marín, M., and Sala, L. (2014). Tertiary treatment for wastewater reuse based on the Daphnia magna filtration–comparison with conventional tertiary treatments. Water Sci. Technol. 70 (4), 705–711. doi:10.2166/wst.2014.284
Serra, T., and Colomer, J. (2016). The hydraulic retention time on the particle removal efficiency by Daphnia magna filtration on treated wastewater. Int. J. Environ. Sci. Technol. (Tehran). 13 (6), 1433–1442. doi:10.1007/s13762-016-0985-4
Serra, T., Soler, M., Pous, N., and Colomer, J. (2019). Daphnia magna filtration, swimming and mortality under ammonium, nitrite, nitrate and phosphate. Sci. Total Environ. 656, 331–337. doi:10.1016/j.scitotenv.2018.11.382
Sharma, B. K. (1992)., 3. Calcutta, 1–121.Freshwater rotifers (rotifers: Eurotatoria) fauna of West Bengal state faunal seriesZoological Surv. India
Sharma, P., Tripathi, S., Sirohi, R., Kim, S. H., Ngo, H. H., and Pandey, A. (2021). Uptake and mobilization of heavy metals through phytoremediation process from native plants species growing on complex pollutants: Antioxidant enzymes and photosynthetic pigments response. Environ. Technol. Innov. 23, 101629. doi:10.1016/j.eti.2021.101629
Shiny, K. J., Remani, K. N., Nirmala, E., Jalaja, T. K., and Sasidharan, V. K. (2005). Biotreatment of wastewater using aquatic invertebrates, Daphnia magna and Paramecium caudatum. Bioresour. Technol. 96 (1), 55–58. doi:10.1016/j.biortech.2004.01.008
Siegfried, M., Koelmans, A. A., Besseling, E., and Kroeze, C. (2017). Export of microplastics from land to sea. A modelling approach. Water Res. 127, 249–257. doi:10.1016/j.watres.2017.10.011
Sinha, A. K., Barkakat, M., Nath, D., and Sharma, S. K. (2010). “Economic viability of bamboo dust based gasification plant for a paper mill international conference on renewable energies and power quality, las palmas de Gran canaria (Spain), 13th to 15th april,” in A comparative study of biomass materials using downdraft gasifier, 275.
Stottmeister, U., Wießner, A., Kuschk, P., Kappelmeyer, U., Kästner, M., Bederski, O., et al. (2003). Effects of plants and microorganisms in constructed wetlands for wastewater treatment. Biotechnol. Adv. 22 (1-2), 93–117. doi:10.1016/j.biotechadv.2003.08.010
Subramanyam, K. (1962).“Aquatic angiosperms: a systematic account of common Indian aquatic angiosperms,” in Botanical monograph (New Delhi: CSIR), 3, 1–190.
Tamura, K., Nei, M., and Kumar, S. (2004). Prospects for inferring very large phylogenies by using the neighbor-joining method. Proc. Natl. Acad. Sci. U. S. A. 101, 11030–11035. doi:10.1073/pnas.0404206101
Tousignant, E., Fankhauser, O., and Hurd, S. (1999). Guidance manual for the design, construction and operations of constructed wetlands for rural applications in Ontario.
UNEP (2009). UNEP-2009-annual-report. Available at: https://www.unep.org/resources/annual-report/unep-2009-annual-report.
UNICEF-WHO (2017). “Progress on drinking water, sanitation and hygiene—joint monitoring programme 2017 update and SDG baselines,” in The united nations childrens’ fund (UNICEF) (Geneva, Switzerland: World Health Organization WHO).
United Nation (2019). The sustainable development Goals. Report. Available at: https://unstats.un.org/sdgs/report/2019/.
United Nations Sustainable Development Goal (2020). Goal 6: Ensure access to water and sanitation for all. Available at: https://www.un.org/sustainabledevelopment/water-and-sanitation/.
US EPA (2021). National aquatic resource surveys. Available at: https://www.epa.gov/national-aquatic-resource-surveys/indicators-zooplankton.
Van Puijenbroek, P. J. T. M., Beusen, A. H. W., and Bouwman, A. F. (2019). Global nitrogen and phosphorus in urban waste water based on the Shared Socio-economic pathways. J. Environ. Manage. 231, 446–456. doi:10.1016/j.jenvman.2018.10.048
Van Wijnen, J., Ragas, A. M., and Kroeze, C. (2019). Modelling global river export of microplastics to the marine environment: Sources and future trends. Sci. Total Environ. 673, 392–401. doi:10.1016/j.scitotenv.2019.04.078
Vermeulen, L. C., Hofstra, N., Kroeze, C., and Medema, G. (2015). Advancing waterborne pathogen modelling: Lessons from global nutrient export models. Curr. Opin. Environ. Sustain. 14, 109–120. doi:10.1016/j.cosust.2015.05.003
Wang, K., Cai, J., Feng, J., and Xie, S. (2014). Phytoremediation of phenol using Polygonum orientale, including optimized conditions. Environ. Monit. Assess. 186 (12), 8667–8681. doi:10.1007/s10661-014-4034-9
Wang, M., Zhang, D., Dong, J., and Tan, S. K. (2018). Application of constructed wetlands for treating agricultural runoff and agro-industrial wastewater: a review. Hydrobiologia 805 (1), 1–31. doi:10.1007/s10750-017-3315-z
Young, P., Taylor, M., and Fallowfield, H. J. (2017). Mini-review: high rate algal ponds, flexible systems for sustainable wastewater treatment. World J. Microbiol. Biotechnol. 33 (6), 1–13. doi:10.1007/s11274-017-2282-x
Zhang, W., Gago-Ferrero, P., Gao, Q., Ahrens, L., Blum, K., Rostvall, A., et al. (2019). Evaluation of five filter media in column experiment on the removal of selected organic micropollutants and phosphorus from household wastewater. J. Environ. Manage. 246, 920–928. doi:10.1016/j.jenvman.2019.05.137
Zheng, Z. C., Li, T. X., Zeng, F. F., Zhang, X. Z., Yu, H. Y., Wang, Y. D., et al. (2013). Accumulation characteristics of and removal of nitrogen and phosphorus from livestock wastewater by Polygonum hydropiper. Agric. Water Manag. 117, 19–25. doi:10.1016/j.agwat.2012.10.017
Zhou, Q., Zhang, J., Fu, J., Shi, J., and Jiang, G. (2008). Biomonitoring: an appealing tool for assessment of metal pollution in the aquatic ecosystem. Anal. Chim. Acta X. 606 (2), 135–150. doi:10.1016/j.aca.2007.11.018
Zraunig, A., Estelrich, M., Gattringer, H., Kisser, J., Langergraber, G., Radtke, M., et al. (2019). Long term decentralized greywater treatment for water reuse purposes in a tourist facility by vertical ecosystem. Ecol. Eng. 138, 138–147. doi:10.1016/j.ecoleng.2019.07.003
Keywords: biological treatment system, zooplankton, locally adapted species, circularity, natural purifiers, constructed wetland
Citation: Prakash D, Kumar R, Rajan K, Patel A, Yadav DK, Dhanker R and Khudsar FA (2022) Integrated application of macrophytes and zooplankton for wastewater treatment. Front. Environ. Sci. 10:941841. doi: 10.3389/fenvs.2022.941841
Received: 11 May 2022; Accepted: 01 August 2022;
Published: 08 September 2022.
Edited by:
Vineet Kumar, National Environmental Engineering Research Institute (CSIR), IndiaReviewed by:
Vivek Rana, Central Pollution Control Board, IndiaCopyright © 2022 Prakash, Kumar, Rajan, Patel, Yadav, Dhanker and Khudsar. This is an open-access article distributed under the terms of the Creative Commons Attribution License (CC BY). The use, distribution or reproduction in other forums is permitted, provided the original author(s) and the copyright owner(s) are credited and that the original publication in this journal is cited, in accordance with accepted academic practice. No use, distribution or reproduction is permitted which does not comply with these terms.
*Correspondence: Ram Kumar, cmFta3VtYXJAY3ViLmFjLmlu
Disclaimer: All claims expressed in this article are solely those of the authors and do not necessarily represent those of their affiliated organizations, or those of the publisher, the editors and the reviewers. Any product that may be evaluated in this article or claim that may be made by its manufacturer is not guaranteed or endorsed by the publisher.
Research integrity at Frontiers
Learn more about the work of our research integrity team to safeguard the quality of each article we publish.