- 1Department of Basic and Applied Sciences, School of Engineering and Sciences, GD Goenka University, Gurugram, Haryana, India
- 2Department of Botany and Microbiology, College of Science, King Saud University, Riyadh, Saudi Arabia
- 3Laboratory for Quantum Semiconductors and Photon-based BioNanotechnology, Department of Electrical and Computer Engineering, Faculty of Engineering, Université de Sherbrooke, Sherbrooke, QC, Canada
A huge amount of food waste is being generated every day globally. Usually, India generates ∼350 million tons of food waste every year. Therefore, there is an urgent need to initiate research focusing on the management and hygienic methods of reuse of food waste together with advanced user-friendly methods of converting it into some useful products thereby generating wealth from food waste. A promising approach seems to biosynthesize silver nanoparticles (AgNPs) from such unutilized food. An alternative clean technology does not rely on the use of toxic chemicals and solvents. It is commonly allied with traditional nanoparticle synthesis processes. In the present work, the peels of two vegetables, pea (Pisum sativum) and bottle gourd (Lagenaria siceraria), were used to generate AgNPs. AgNPs were obtained by dissolving 1.5 ml of the peel extract of each vegetable in 50 ml of silver nitrate (AgNO3) and incubating for 24 h at room temperature. For the confirmation of AgNP production UV–visible spectroscopy was used. Field Emission Scanning Electron Microscopy (FESEM), X-ray diffraction (XRD), and attenuated total reflection-infrared spectroscopy (ATR-IR Analysis) were used to characterize them. Furthermore, AgNPs in different concentrations were used to test antibacterial activity against bacteria Escherichia coli through the disc diffusion method. Thus, our research indicates that AgNPs can be a safe and environmentally beneficial production technology from unutilized vegetable wastes that may play an important role in the management of food waste in the future and has antibacterial potential to preserve vegetables from bacterial contamination.
1 Introduction
Since the most recent period, the discovery of a sustainable and eco-friendly biochemical approach and synthesizing methodology for generating silver nanoparticles (AgNPs) have received considerable attention (Shankar et al., 2004; Bhattarai et al., 2018). Nanostructures are a relatively new feature of science and technology concerned with the fabrication and modification of molecular arrangement on the nanoscale dimension. Pharmacology—therapeutic administration, electromechanical, biological sensors, cosmetology, optic energy, agricultural regions, scanning, and many more are all progressively being associated with nanoparticles (Ahamed et al., 2010; Xing et al., 2021). Kitchen-generated biodegradable matter was not considered a substantial material a few years back (Baiano, 2014; Annu et al., 2018). Recent concerns about the nutrition, conservation, ecological constraints, and socioeconomic consequences of kitchen organic waste have boosted research into creating alternatives to overcome food waste and encourage appropriate waste disposal strategies (Kantor et al., 1997; Patra and Baek, 2016). As a result, effective and economic measures in order to reduce food waste and establish improved food waste utilization strategies that can help with global supply chain planning are acceptable (Gustavsson et al., 2011; Ghosh et al., 2015; Carmona-Cabello et al., 2018). The goal of these strategies is to quantify the efficiency and benefits produced from kitchen household waste, reducing the quantity of the garbage that ends up in landfills (Chaudhry et al., 2008).
The present article highlights the biosynthesis and different characterization methods of AgNPs. The manufacturing of AgNPs from vegetable wastes and silver nanoparticle synthesis is a one-of-a-kind blend (Madhumitha et al., 2011; Kim et al., 2012; Yang et al., 2014). There is an emergent interest in green-synthesized AgNPs by utilizing vegetable-based composites since they are economically efficient, do not require the use of harmful chemicals, and are deemed environment friendly (Vigneshwaran et al., 2007; Tavakoli et al., 2015). The plant extract reacts as a stabilizing agent attributed to the presence of antioxidant and antibacterial activities. A considerably large variety of compounds can be identified in kitchen wastes. Therefore, silver nanostructures have captivated the interest of researchers because of their exceptional properties (Ram et al., 2014). A number of beneficial substances are found in the outer peels of fruits and vegetables which are commonly discarded as garbage. These materials could be used as raw resources for the synthesis of AgNPs, which can be an effective strategy of food waste management (Patra and Baek, 2016). Fruit and vegetable peels have 15% more phenolic compounds than their pulp (Gorinstein et al., 2001; Soong and Barlow, 2004). Improved efficiency, productivity, and sustainability have been reported in biologically synthesized AgNPs (Chung et al., 2016). AgNPs are the potential candidates for inhibiting the growth of the bacteria indicating a promising approach for preserving vegetables and fruits (Carmona-Cabello et al., 2018; Dhanker et al., 2021). The objective of the present research article was to synthesize and characterize AgNPs from peels of two vegetables: pea (Pisum sativum) and bottle gourd (Lagenaria siceraria), and to compare their antibacterial activities. A graphical representation about the synthesis of AgNPs, characterization and its antibacterial activity from the peels of pea (Pisum sativum) and bottle gourd (Lagenaria siceraria) has been shown in Figure 1.
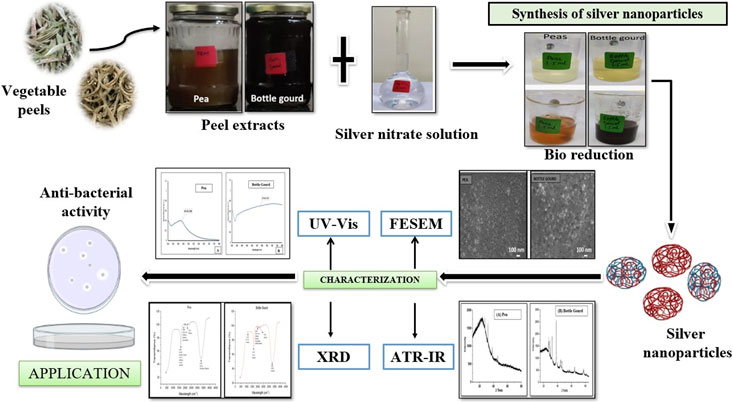
FIGURE 1. Graphical representation about the synthesis of silver nanoparticles, characterization and its antibacterial activity from the peels of pea (Pisum sativum) and bottle gourd (Lagenaria siceraria).
2 Materials and methods
2.1 Materials
Vegetable peels of pea (Pisum sativum) and bottle gourd (Lagenaria siceraria) were collected from the kitchen. It was washed 2–3 times with tap water and rinsed in deionized water to remove impurities and dust. The washed peels were shade-dried out until the moisture was removed.
2.2 Preparation of peel extract
The dried vegetable peels of pea (Pisum sativum) and bottle gourd (Lagenaria siceraria) from kitchen waste were grinded to get a coarse powder and stored in containers for the synthesis of silver nanoparticles (AgNPs). For the preparation of the plant extract, 30 g of the powdered peels was boiled at 60°C in 300 ml deionized water for 40 min. Then, it was left undisturbed for 3 h. After 3 h, the color of the aqueous solution shifted from colorless to yellow after boiling. The boiled content was filtered by utilizing the Buckner funnel with an aid of a vacuum pump and Whatman filter paper no. 1 to separate the aqueous extracts. The collected aqueous extracts thus formed were stored in Borosil glass flasks at 4°C until the next use has been shown in Figure 2.
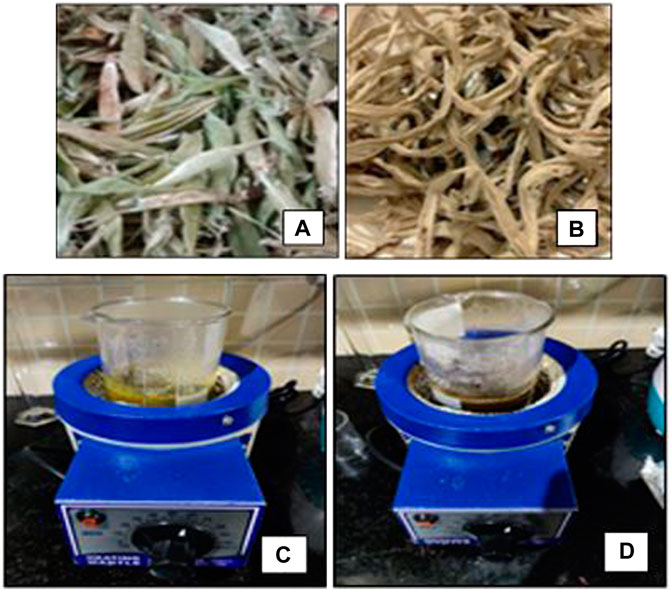
FIGURE 2. Dried vegetable peels of pea (Pisum sativum) (A) and bottle gourd (Lagenaria siceraria) (B) and the extraction of both vegetable peels (C,D).
2.3 Synthesis of silver nanoparticles
For the fabrication of AgNPs, 1 mM silver nitrate (AgNO3) in an aqueous solution was formed. 1.5 ml of the peel extract was added to 50 ml of the aqueous solution of AgNO3 for the reduction of silver-to-silver ions. The solution was boiled for 30 min at 650C. It was held at room temperature for a 24 h incubation period. The formation of AgNPs was confirmed by a color change from light yellow to dark brown in the vegetable peels which has been shown in Figures 3, 4. The obtained solutions were centrifuged at 3,000 rpm for 10 min and rinsed with deionized water multiple times before being stored in water.
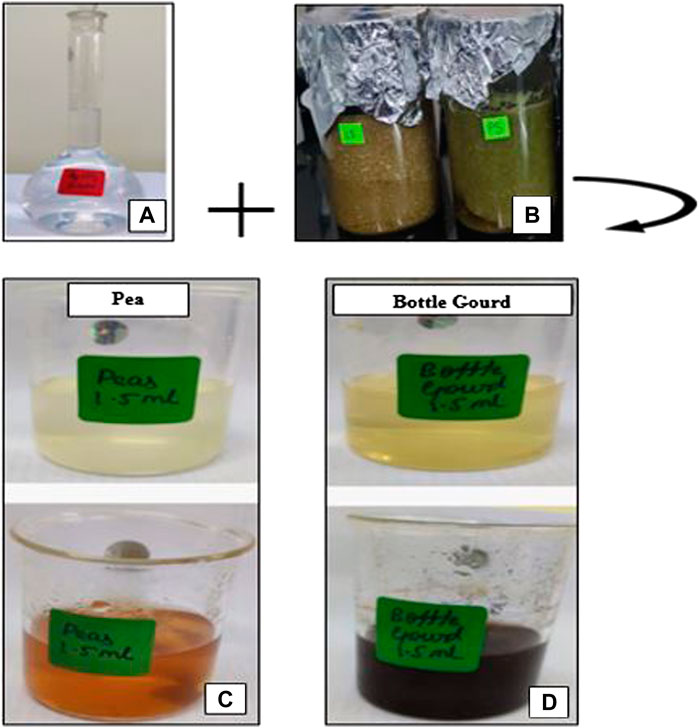
FIGURE 3. Formulation of silver nanoparticles with pea (Pisum sativum) (A) and bottle gourd peels (Lagenaria siceraria) (B) extracted with 1 mM silver nitrate solution. Color change observed before and after the incubation period during the synthesis of silver nanoparticles (C,D).
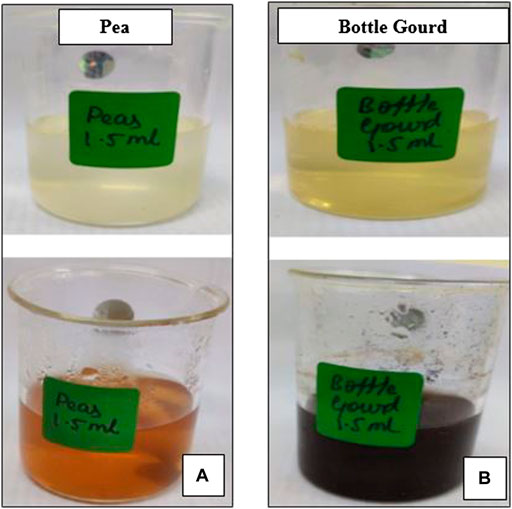
FIGURE 4. Color changed observed during the synthesis of silver nanoparticles at a constant volume of pea (Pisum sativum) (A) and bottle gourd peels (Lagenaria siceraria) (B) extract with the silver nitrate solution.
2.4 Characterization of silver nanoparticles
2.4.1 Morphological examination
The incubated extracts of both studied vegetable peels were recorded for the synthesis of AgNPs based on the color change.
2.4.2 UV-VIS spectroscopy
The synthesized AgNPs from both vegetable peels were observed after 24 h for their optical measurements regularly through a UV–Vis spectrophotometer (Ocean Insight HR 2000+ high-resolution spectrometer) as a confirmation of the nanoparticle synthesis. The scanning was conducted within the 200–800 nm frequency range at the resolution of 1 nm. Double distilled water was used as a blank reference and UV–visible spectra were recorded using a 1 cm Quartz cuvette.
2.4.3 Field emission scanning electron microscopy
The technique was utilized for examining the morphological appearance of the synthesized AgNPs. It aids in defining the dimension, shape, and surface morphology. With the help of field emission scanning electron microscopy, the micrographs were obtained from the JSM-7001F model. As the prepared biogenic colloidal AgNPs were stored in water, to obtain the images, the samples were drop-casted on a cover slip, dried, and followed by gold sputtering.
2.4.4 X-ray diffraction
Crystalline metallic AgNPs was examined by X-ray diffraction (XRD). The XRD pattern was examined by using the Rigaku smart lab X-ray diffractometer. The diffracted intensities were investigated between the ranges of 20–80° 2θ angles.
2.4.5 Attenuated total reflection-infrared spectroscopy (ATR-IR analysis)
The impact of the functional groups as in stability (reducing) and fabrication of metal nanoparticles contained in the different vegetable extracts was investigated using ATR-IR spectroscopic techniques by using Shimadzu IRSpirit.
2.4.6 Antibacterial properties
Escherichia coli (E. coli) was isolated and maintained as a pure culture in G. D. Goenka University, India. The standard disc diffusion method was used to investigate the antibacterial activity of AgNPs made from the peel extracts of both vegetables against the human pathogenic bacteria E. coli. On nutrient agar plates, 100 μl of fresh overnight cultures were dispersed. Different concentrations of AgNPs were taken for examining the zone of inhibition. Each plate was loaded with five sterile paper discs measuring 6 mm wide, each containing sterile water and varying volumes of AgNPs (2 μl, 4 μl, 8 μl, and 10 μl). The control was then correlated with the inhibition zones (deprived of AgNPs). The Minimal Bactericidal Concentration (MBC) analysis using the control was accomplished.
3 Results
3.1 Synthesis of AgNPs
By combining 1.5 ml of the aqueous extract of the vegetable peels with 50 ml of the 1 mM silver nitrate solution, nanocomposites were synthesized. A change in the color of the mixture into dark brown indicated the formation of AgNPs. The shift in coloration revealed the reduction in silver (Ag) ions resulting in the synthesis of AgNPs.
3.2 UV–VIS spectral analysis
Using UV–visible spectroscopy, the absorption spectra of the different reaction mixtures were examined. The two extracts of pea and bottle gourd peels exhibited distinct peaks in the visible range extending from 200 to 800 nm in the UV-Vis spectra of the biologically synthesized nanoparticles at 1 mM, demonstrating the formation of silver nanoparticles. The maximum absorption peak for pea was observed at 431 nm and for bottle gourd it was observed at 573 nm (Figure 5). The formation of dark brown coloration in the reaction medium indicated the synthesis of AgNPs. Additionally, this happened due to the excitement of plasmon resonance in the nanoparticles having a distinctive color (pale yellow to dark brown).
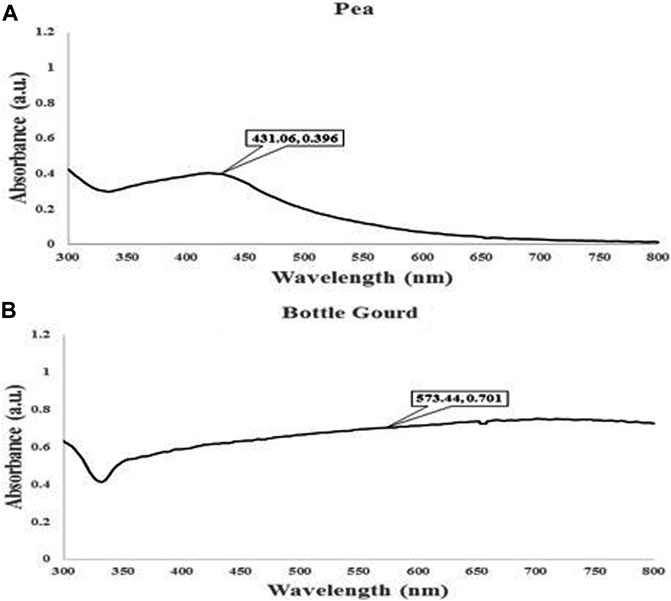
FIGURE 5. UV–vis. spectrum analysis for the maximum absorbance at 431 nm for pea (Pisum sativum) (A) and 573 for bottle gourd (Lagenaria siceraria) (B).
3.3 Field emission scanning electron microscopy
Field emission scanning electron microscopy (FESEM) was used to analyze the surface properties and topology of the synthesized NPs. The obtained FESEM micrographs are provided in Figure 6 showing without any aggregation, spherical AgNPs were observed. The large number of AgNPs generated by the peel extracts has been shown in the FESEM images. The distribution of AgNPs is excellent in case of bottle gourd (Lagenaria siceraria) and peas (Pisum sativum). Along with the distribution of the NPs, the size of the nanoparticles also varied for each vegetable peel. For both the nanoparticles, the size varied from 30 to 50 nm. The size, shape, and dispensability of NPs rely on the experimental conditions and on the studied reducing/capping agent (Sharma et al., 2020). In the present study, the experimental conditions were kept constant for synthesis of both nanoparticles whereas the reducing agent (considered plant extracts) was a variable component.
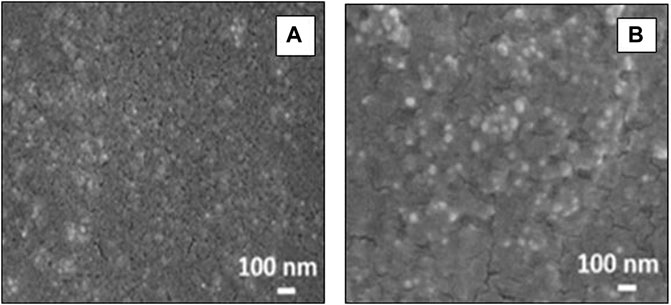
FIGURE 6. Field emission scanning electron microscopy (FESEM) micrograph of biosynthesized silver nanoparticles for pea (Pisum sativum) (A) and bottle gourd (Lagenaria siceraria) (B).
3.4 X-ray diffraction
The X-ray diffraction (XRD) studies of the manufactured AgNPs have been described in Figure 7. The XRD technique is an exceptional methodology for supporting the fabrication of nanoparticles because it delivers the information about structural configurations and the gap between the particles. The XRD study of silver nanoparticles showed the peak at 38.20° (2θ) which was responsible for (111) for pea peels (Pisum sativum). In the case of bottle gourd (Lagenaria siceraria), the peaks were observed at 38.12°, 44.32°, 64.56°, and 77.40° which are responsible for (111), (200), (220), and (311) Bragg’s reflection, correspondingly. These standards were coordinated with JCPDS file NO. 04-0783. Based on these outcomes, green-manufactured silver nanoparticles are possibly face-centered (FCC) and cubic-structured. The crystallite size of the silver nanoparticles was measured by Scherrer’s equation, which is, D = kλ/β Cosθ. Where D is crystallite size, k is a constant equal to 0.94, λ is the X-ray wavelength (1.54 Ǻ), and β is full with a half maximum of the high-intensity peak (FWHM). The average crystallite size of the AgNPs was found to be 20–70 nm for both. The XRD results were reliable with the abundant deliberated data associated with the cubic symmetry of silver nanoparticles showing that the silver nanostructures were formed.
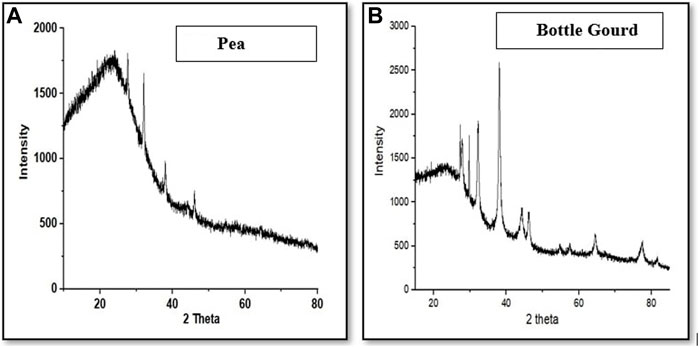
FIGURE 7. X-ray diffraction (XRD) characterization of silver nanoparticles for pea (Pisum sativum) (A) and bottle gourd (Lagenaria siceraria) (B).
3.5 Attenuated total reflection-infrared spectroscopy (ATR-IR analysis)
The functional groups of the biosynthesized silver nanoparticles that are mainly responsible for the reducing Ag+ to Ag0 were identified utilizing the ATR-IR analysis (Figure 8). The ATR-IR spectra of pea (Pisum sativum) displays maxima at approximately 1,644 cm−1, 1,964 cm−1, 2,141 cm−1, and 3,305 cm−1 that can be attributed to various groups. (N = H) Amine, (C = C) alkenes, and (C = O) amide have shown a bump at 1,644 cm−1, while allenes (X = C = Y), ketenes, and isocyanates have shown a vibration at 1,964 cm−1, the band at 2141 cm−1 assigned to the Alkyne group, whereas, at 3,305 cm−1 (OH) corresponds to the vibrations of alcohol and phenols. The ATR-IR spectra for bottle gourd (Lagenaria siceraria) have shown that the Amine group’s (C–N) asymmetric stretching showed a bump at 1,141 cm−1. The C = C oscillations or aromatic ring (C = C) correlated to the peak at 1,627 cm−1. (X = C = Y) Allenes, ketenes, isocyanates, and isothiocyanates have shown a vibration at 1,992 cm−1, the band at 2,192 cm−1 assigned to the Alkyne group, whereas, at 3,283 cm−1(OH) for the corresponded vibrations of alcohol and phenols.
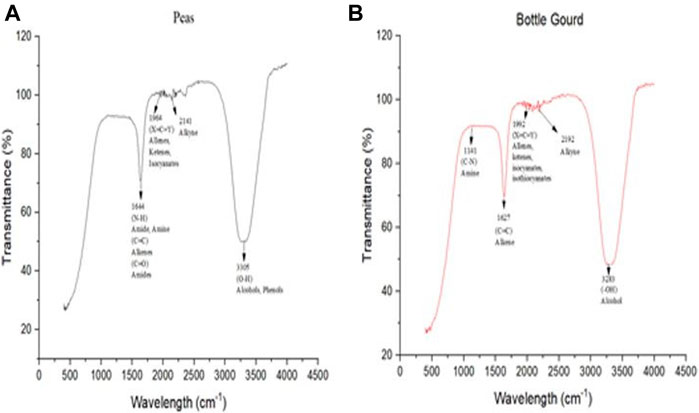
FIGURE 8. Attenuated total reflection-infrared spectroscopy (ATR-IR) spectra from 400 cm−1 to 4000 cm−1 of silver nanoparticles for pea (Pisum sativum) (A) and bottle gourd (Lagenaria siceraria) (B).
3.6 Antimicrobial activity
The antimicrobial property of fabricated AgNPs was investigated using the disc diffusion technique against pathogen Escherichia coli (E. coli). It showed the greatest activity at selected concentrations of AgNPs. Table 1 depicted the maximum zone of inhibition of AgNPs. This meant that AgNPs generated from pea and bottle gourd peel extracts having antibacterial properties against E. coli. The significant antibacterial activity of peel-generated nanoparticles has been examined against E. coli in previous studies also (Sharma et al., 2016; Baran et al., 2021).
4 Discussion
In this study, vegetable peels of pea and bottle gourd were used to demonstrate green synthesis of silver nanoparticles (AgNPs). Thereafter, the effect of these NPs was tested against Escherichia coli (E. coli). Green nanotechnology is a potential and environmental friendly technology in which kitchen household waste can be utilized for the fabrication of AgNPs. In an aqueous medium, AgNPs have a yellowish brown color due to the resonance activity (Shankar et al., 2004). However, the green fabrication of AgNPs from kitchen waste peels is not a modern theory (Xing et al., 2021). Numerous authors have progressively suggested many ideal processes for the generation of nanoparticles (Kemp et al., 2009; Mata et al., 2009; Xing et al., 2021). Similar outcomes have been confirmed by our results also. According to several published reports, natural polymers tend to play a substantial role in the reduction of nanomaterials (Sharma et al., 2020). AgNPs have been synthesized from the extracts of vegetable waste peels containing phytochemicals (Sahu et al., 2012; Arunachalam et al., 2013). However, further large-scale research is needed for more realistic and positive outcomes for the betterment of humankind and a safe environment. AgNPs generated from discarded vegetables and fruits peels have shown antimicrobial properties against both Gram-positive and Gram-negative bacteria as stated in Table 2.
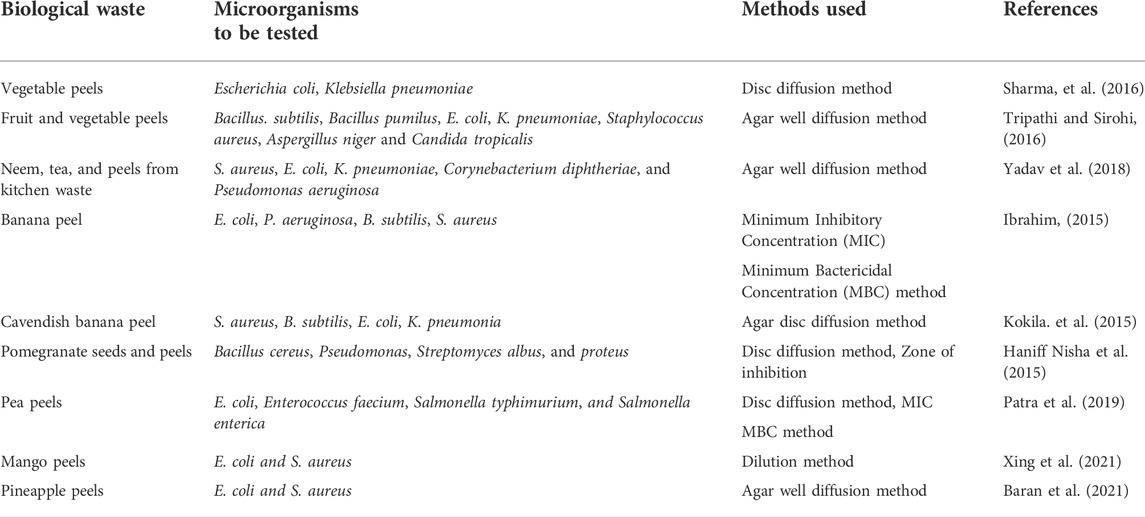
TABLE 2. Antimicrobial activities of silver nanoparticles synthesized using different fruit and vegetable waste peels.
The participation of the polypeptide group acts as a capping agent, which facilitates the stability of silver nanoparticles (Rodriguez et al., 2013). The functional groups of biosynthesized AgNPs that are mainly responsible for reducing Ag+ to Ag0 were identified utilizing the ATR-IR analysis. The ATR-IR spectra of pea (Pisum sativum) displayed maxima at approximately 1,644 cm−1, 1,964 cm−1, 2,141 cm−1, and 3,305 cm−1 that can be attributed to various groups. (N = H) Amine, (C = C) alkenes, and (C = O) amide have shown a bump at 1,644 cm−1. Allenes (X = C = Y), ketenes, isocyanates have shown a vibration at 1,964 cm−1, the band at 2,141 cm−1 assigned to the Alkyne group, whereas, at 3,305 cm−1 (OH) corresponds to the vibrations of alcohol and phenols. The ATR-IR spectra for bottle gourd (Lagenaria siceraria) have shown that the Amine group’s (C–N) asymmetric stretching shows a bump at 1,141 cm−1. The C = C oscillations or aromatic ring (C = C) correlated to the peak at 1,627 cm−1. Allenes (X = C = Y), ketenes, isocyanates, and isothiocyanates have shown vibration at 1,992 cm−1, the band at 2,192 cm−1 assigned to the Alkyne group, whereas, at 3,283 cm−1 (OH) corresponds to the vibrations of alcohol and phenols. As per the observations of the ATR-IR study, it is possible that phytochemicals and specific proteins found in the peel extracts of both vegetable wastes that are responsible for capping and stabilizing the nanostructures. Researchers have found that the peels of both pea and bottle gourd possess significant concentrations of active substances such as cellulose, micronutrients, and phenolic compounds (Naqvi et al., 2021). These natural compounds are well known as bioactive components with significant antioxidant and antibacterial characteristics (Kumar et al., 2015; Obembe et al., 2018; Castaldo et al., 2021) as details have been shown in Table 3.
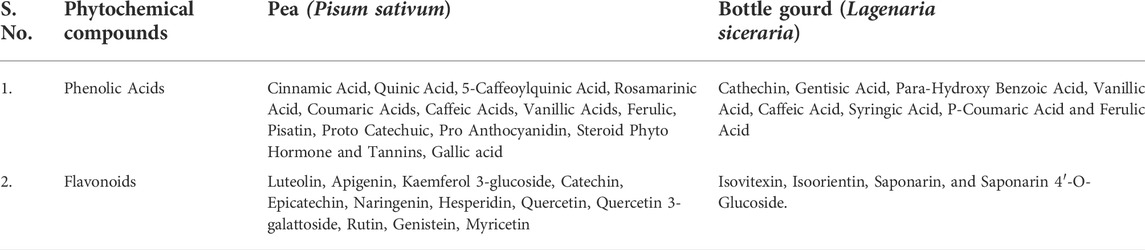
TABLE 3. Phenolic acid and flavonoid content in the pea (Pisum sativum) and bottle gourd (Lagenaria siceraria) (Kumar et al., 2015; Obembe et al., 2018; Castaldo et al., 2021; Naqvi et al., 2021).
Silver is believed to hold antibacterial qualities (Azam et al., 2012; Baran et al., 2021). AgNPs have in fact been used as an antibacterial agent in a wide range of medicinal applications (El-Rafie et al., 2012). Additionally, it has been found that AgNPs are toxic to Gram-negative bacteria (Azam et al., 2012; Xing et al., 2021). Our study has shown that generated AgNPs are potent antibacteria against Escherichia coli. Numerous researchers have observed similar findings in the kitchen food waste of different vegetable and fruit peels (Percival et al., 2007; Lara et al., 2009; Baran et al., 2021; Xing et al., 2021; Table 2).
5 Conclusion and future perspectives
The present study describes a sustainable and environmentally acceptable process for the production of AgNPs from kitchen-generated peel waste such as from the peels of bottle gourd (Lagenaria siceraria) and pea (Pisum sativum). Our analysis indicated that pea (Pisum sativum) and bottle gourd (Lagenaria siceraria) peels were used to produce AgNPs with a diameter of approximately 30–50 nm. Kitchen waste peels function in this situation as reducing agents. A number of techniques, including UV spectrophotometer, XRD, ATR-IR spectrometry, and FESEM were used to carry out the characterization of nanoparticles. The UV–Vis spectrum exhibited an AgNPs synthesis plasmon maximum absorption at 431 nm for pea (Pisum sativum) and 573 nm for bottle gourd (Lagenaria siceraria). According to the FESEM micrograph image analysis the fabricated nanoparticles were approximately spherical and had an average size of 30–50 nm. The ATR-IR analysis was conducted to identify potential functional groups that are in favor of providing nanoparticles and the stability of their synthesis. The phytochemicals and bioactive molecules included in the peel extract may have a role in the generation, capping, and stabilization of the nanoparticles. Additionally, antibacterial properties were tested against the highly toxic Gram-negative bacterium Escherichia coli. We have inferred from the outcomes that AgNPs generated from the peels of both vegetables have a potent antibacterial capability. Food waste is a major issue that is becoming worse every day. During and after harvesting, a huge amount of food is wasted. AgNPs are a sustainable and environmentally beneficial solution in such scenarios. AgNPs have various uses in multiple sectors. It has the potential to grant alternatives for the well-being of the population. The production of AgNPs from household waste will aid in the management of food wastage and consequently help in resolving challenges with environmental issues and prevent waste. However, determining the complete potential of AgNPs for managing wastage is still a concern for researchers as it creates opportunities for their research in the future.
Data availability statement
The original contributions presented in the study are included in the article/supplementary material; further inquiries can be directed to the corresponding author.
Author contributions
Deepa has written the draft of the manuscript. RD conceived the idea, supervised the content and critically edited the manuscript. FA and MAI have critically edited the manuscript and funded the research. All authors have approved the submitted version equally.
Acknowledgments
The authors express their gratitude to GD Goenka University for supporting the first author to pursue doctoral study. The authors express their special thanks to Soumita Talukdar, Assistant professor, GD Goenka University and Priyanka Tyagi, Assistant professor, GD Goenka University for their constructive comments and suggestions as research topic experts. The authors are thankful to the reviewers for their constructive comments and critical reviews. This research was funded by Researchers Supporting Project number (RSP-2021/364), King Saud University, Riyadh, Saudi Arabia.
Conflict of interest
The authors declare that the research was conducted in the absence of any commercial or financial relationships that could be construed as a potential conflict of interest.
Publisher’s note
All claims expressed in this article are solely those of the authors and do not necessarily represent those of their affiliated organizations, or those of the publisher, the editors, and the reviewers. Any product that may be evaluated in this article, or claim that may be made by its manufacturer, is not guaranteed or endorsed by the publisher.
References
Ahamed, M., AlSalhi, M., and Siddiqui, M. (2010). Silver nanoparticle applications and human health. Clin. Chim. Acta 411 (23–24), 1841–1848. doi:10.1016/j.cca.2010.08.016
Annu, A., Ahmed, S., Kaur, G., Sharma, P., Singh, S., and Ikram, S. (2018). Fruit waste (peel) as bio-reductant to synthesize silver nanoparticles with antimicrobial, antioxidant and cytotoxic activities. J. Appl. Biomed. 16 (3), 221–231. doi:10.1016/j.jab.2018.02.002
Arunachalam, K., Annamalai, S., and Hari., S. (2013). One-step green synthesis and characterization of leaf extract-mediated biocompatible silver and gold nanoparticles from Memecylon umbellatum. Int. J. Nanomedicine 1307, 1315. doi:10.2147/ijn.s36670
Azam, A., Ahmed, O., Khan, M. S., Habib, S. S., and Memic, A. (2012). Antimicrobial activity of metal oxide nanoparticles against gram-positive and gram-negative bacteria: A comparative study. Int. J. Nanomedicine 7, 6003–6009. doi:10.2147/IJN.S35347
Baiano, A. (2014). Recovery of biomolecules from food wastes — a review. Molecules 19 (9), 14821–14842. doi:10.3390/molecules190914821
Baran, A., Keskin, C., Baran, M., Huseynova, I., Khalilov, R., Eftekhari, A., et al. (2021). Ecofriendly synthesis of silver nanoparticles using ananas comosus fruit peels: Anticancer and antimicrobial activities. Bioinorganic Chem. And Appl. 2021, 1–8. doi:10.1155/2021/2058149
Bhattarai, B., Zaker, Y., and Bigioni, T. (2018). Green synthesis of gold and silver nanoparticles: Challenges and opportunities. Curr. Opin. Green And Sustain. Chem. 12, 91–100. doi:10.1016/j.cogsc.2018.06.007
Carmona-Cabello, M., Garcia, I., Leiva-Candia, D., and Dorado, M. (2018). Valorization of food waste based on its composition through the concept of biorefinery. Curr. Opin. Green And Sustain. Chem. 14, 67–79. doi:10.1016/j.cogsc.2018.06.011
Castaldo, L., Izzo, L., Narváez, A., Rodríguez-Carrasco, Y., Grosso, M., and Ritieni, A. (2021). Colon bioaccessibility under in vitro gastrointestinal digestion of different coffee brews chemically profiled through UHPLC-Q-orbitrap HRMS. Foods 10 (1), 179. doi:10.3390/foods10010179
Chaudhry, Q., Scotter, M., Blackburn, J., Ross, B., Boxall, A., Castle, L., et al. (2008). Applications and implications of nanotechnologies for the food sector. Food Addit. Contam. Part A 25 (3), 241–258. doi:10.1080/02652030701744538
Chung, I., Park, I., Seung-Hyun, K., Thiruvengadam, M., and Rajakumar, G. (2016). Plant-Mediated synthesis of silver nanoparticles: Their characteristic properties and therapeutic applications. Nanoscale Res. Lett. 11 (1), 40. doi:10.1186/s11671-016-1257-4
Dhanker, R., Hussain, T., Tyagi, P., Singh, K., and Kamble, S. (2021). The emerging trend of bio-engineering approaches for microbial nanomaterial synthesis and its applications. Front. Microbiol. 12. doi:10.3389/fmicb.2021.638003
El-Rafie, M., Shaheen, T., Mohamed, A., and Hebeish, A. (2012). Bio-synthesis and applications of silver nanoparticles onto cotton fabrics. Carbohydr. Polym. 90 (2), 915–920. doi:10.1016/j.carbpol.2012.06.020
Ghosh, P. R., Sharma, S. B., Haigh, Y. T., Barbara Evers, A. L., and Ho, G. (2015). An overview of food loss and waste: Why does it matter? COSMOS 11, 89–103. doi:10.1142/s0219607715500068
Gorinstein, S., Martı́n-Belloso, O., Park, Y., Haruenkit, R., Lojek, A., Ĉı́ž, M., et al. (2001). Comparison of some biochemical characteristics of different citrus fruits. Food Chem. 74 (3), 309–315. doi:10.1016/s0308-8146(01)00157-1
Gustavsson, J., Cederberg, C., Sonesson, U., van Otterdijk, R., and Meybeck, A. (2011). Global food losses and food waste: Extent, causes and prevention. Rome, Italy: Food and Agriculture Organisation of the United Nations.
Haniff Nisha, M., Tamileswari, R., and Jesurani, Sr. S.Jayaraj Annapackiam CollegeAutonomous (2015). Analysis of anti-bacterial activity of silver nanoparticles from pomegranate (punicagranatum) seed and peel extracts. Int. J. Of Eng. Res. And V4 (04), 1044–1048. doi:10.17577/ijertv4is041104
Ibrahim, H. (2015). Green synthesis and characterization of silver nanoparticles using banana peel extract and their antimicrobial activity against representative microorganisms. J. Of Radiat. Res. And Appl. Sci. 8 (3), 265–275. doi:10.1016/j.jrras.2015.01.007
Kantor, L. S., Lipton, K., Manchester, A., and Oliveira, V. (1997). Estimating and addressing America's food losses. Food Review/Natl. Food Rev. 20 (1), 1–11. United States Department of Agriculture, Economic Research Service. doi:10.22004/ag.econ.234453
Kemp, M., Kumar, A., Mousa, S., Dyskin, E., Yalcin, M., Ajayan, P., et al. (2009). Gold and silver nanoparticles conjugated with heparin derivative possess anti-angiogenesis properties. Nanotechnology 20 (45), 455104. doi:10.1088/0957-4484/20/45/455104
Kim, H., Kim, H., Mosaddik, A., Gyawali, R., Ahn, K., and Cho, S. (2012). Induction of apoptosis by ethanolic extract of mango peel and comparative analysis of the chemical constitutes of mango peel and flesh. Food Chem. 133 (2), 416–422. doi:10.1016/j.foodchem.2012.01.053
Kokila, T., Ramesh, P., and Geetha, D. (2015). Biosynthesis of silver nanoparticles from cavendish banana peel extract and its antibacterial and free radical scavenging assay: A novel biological approach. Appl. Nanosci. 5 (8), 911–920. doi:10.1007/s13204-015-0401-2
Kumar, V., Verma, S., Choudhury, S., Tyagi, M., Chatterjee, S., and Variyara, P. S. (2015). Biocompatible silver nanoparticles from vegetable waste: Its characterization and bio-efficacy. Int. J. Nano Material Sci. 4 (1), 70–86.
Lara, H., Ayala-Núñez, N., Ixtepan Turrent, L., and Rodríguez Padilla, C. (2009). Bactericidal effect of silver nanoparticles against multidrug-resistant bacteria. World J. Microbiol. Biotechnol. 26 (4), 615–621. doi:10.1007/s11274-009-0211-3
Madhumitha, G., Rajakumar, G., Roopan, S., Rahuman, A., Priya, K., Saral, A. M., et al. (2011). Acaricidal, insecticidal, and larvicidal efficacy of fruit peel aqueous extract of Annona squamosa and its compounds against blood-feeding parasites. Parasitol. Res. 111 (5), 2189–2199. doi:10.1007/s00436-011-2671-2
Mata, Y., Torres, E., Blázquez, M., Ballester, A., González, F., and Muñoz, J. (2009). Gold(III) biosorption and bioreduction with the Brown alga Fucus vesiculosus. J. Of Hazard. Mater. 166 (2-3), 612–618. doi:10.1016/j.jhazmat.2008.11.064
Naqvi, S., Irfan, A., Zaheer, S., Sultan, A., Shajahan, S., Rubab, S., et al. (2021). Proximate composition of orange peel, pea peel and rice husk wastes and their potential use as antimicrobial agents and antioxidants. Vegetos 34 (2), 470–476. doi:10.1007/s42535-021-00213-1
Obembe, O., Bello, O., Ayanda, O., Aworunse, O., Olukanmi, B., Soladoye, M., et al. (2018). Solanecio biafrae: An underutilized nutraceutically-important african indigenous vegetable. Pharmacogn. Rev. 12 (23), 128. doi:10.4103/phrev.phrev_43_17
Patra, J., Das, G., and Shin, H. (2019). Facile green biosynthesis of silver nanoparticles using <em>Pisum sativum</em> L. outer peel aqueous extract and its antidiabetic, cytotoxicity, antioxidant, and antibacterial activity. Int. J. Nanomed. 14, 6679–6690. doi:10.2147/ijn.s212614
Patra, J. K., and Baek, K. H. (2016). Green synthesis of silver chloride nanoparticles using Prunus persica L. outer peel extract and investigation of antibacterial, anticandidal, antioxidant potential. Green Chem. Lett. Rev. 9 (2), 132–142. doi:10.1080/17518253.2016.1192692
Percival, S., Bowler, P., and Dolman, J. (2007). Antimicrobial activity of silver-containing dressings on wound microorganisms using an in vitro biofilm model. Int. Wound J. 4 (2), 186–191. doi:10.1111/j.1742-481x.2007.00296.x
Ram, P., Vivek, K., and Kumar, S. (2014). Nanotechnology in sustainable agriculture: Present concerns and future aspects. Afr. J. Biotechnol. 13 (6), 705–713. doi:10.5897/ajbx2013.13554
Rodriguez, P., Harada, T., Christian, D., Pantano, D., Tsai, R., and Discher, D. (2013). Minimal "self" peptides that inhibit phagocytic clearance and enhance delivery of nanoparticles. Science 339 (6122), 971–975. doi:10.1126/science.1229568
Sahu, N., Soni, D., Chandrashekhar, B., Sarangi, B., Satpute, D., and Pandey, R. (2012). Synthesis and characterization of silver nanoparticles using Cynodon dactylon leaves and assessment of their antibacterial activity. Bioprocess Biosyst. Eng. 36 (7), 999–1004. doi:10.1007/s00449-012-0841-y
Shankar, S., Rai, A., Ankamwar, B., Singh, A., Ahmad, A., and Sastry, M. (2004). Biological synthesis of triangular gold nanoprisms. Nat. Mat. 3 (7), 482–488. doi:10.1038/nmat1152
Sharma, B., Singh, I., Bajar, S., Gupta, S., Gautam, H., and Kumar, P. (2020). Biogenic silver nanoparticles: Evaluation of their biological and catalytic potential. Indian J. Microbiol. 60 (4), 468–474. doi:10.1007/s12088-020-00889-0
Sharma, K., Kaushik, S., and Jyoti, A. (2016). Green synthesis of silver nanoparticles by using waste vegetable peel and its antibacterial activities. J. Pharm. Sci. Res. 8 (5), 313–316.
Soong, Y., and Barlow, P. (2004). Antioxidant activity and phenolic content of selected fruit seeds. Food Chem. 88 (3), 411–417. doi:10.1016/j.foodchem.2004.02.003
Tavakoli, F., Salavati-Niasari, M., badiei, A., and Mohandes, F. (2015). Green synthesis and characterization of graphene nanosheets. Mater. Res. Bull. 63, 51–57. doi:10.1016/j.materresbull.2014.11.045
Tripathi, A., and Sirohi, R. (2016). Antimicrobial activities of silver nanoparticles synthesized from peel of fruits and vegetables. Biol. Insights 1, 29–34.
Vigneshwaran, N., Ashtaputre, N., Varadarajan, P., Nachane, R., Paralikar, K., and Balasubramanya, R. (2007). Biological synthesis of silver nanoparticles using the fungus Aspergillus flavus. Mater. Lett. 61 (6), 1413–1418. doi:10.1016/j.matlet.2006.07.042
Xing, Y., Liao, X., Liu, X., Li, W., Huang, R., Tang, J., et al. (2021). Characterization and antimicrobial activity of silver nanoparticles synthesized with the peel extract of mango. Materials 14 (19), 5878. doi:10.3390/ma14195878
Yadav, S. G., Patil, S. H., Patel, P., Nair, V., Khan, S., Kakkar, S., et al. (2018). Green synthesis of silver nanoparticles from plant sources and evaluation of their antimicrobial activity. Int. J. Sci. Res. Sci. Eng. Technol. 5 (4), 133–139.
Keywords: vegetable wastes, silver nanoparticles, green synthesis, characterization, antibacterial properties, clean technology
Citation: Deepa , Ameen F, Amirul Islam M and Dhanker R (2022) Green synthesis of silver nanoparticles from vegetable waste of pea Pisum sativum and bottle gourd Lagenaria siceraria: Characterization and antibacterial properties. Front. Environ. Sci. 10:941554. doi: 10.3389/fenvs.2022.941554
Received: 11 May 2022; Accepted: 19 July 2022;
Published: 26 August 2022.
Edited by:
Vineet Kumar, National Environmental Engineering Research Institute (CSIR), IndiaReviewed by:
Pankaj Kumar Arora, Babasaheb Bhimrao Ambedkar University, IndiaHanuman Singh Jatav, Sri Karan Narendra Agriculture University, India
Sirat Sandil, Eötvös Loránd University, Hungary
Copyright © 2022 Deepa, Ameen, Amirul Islam and Dhanker. This is an open-access article distributed under the terms of the Creative Commons Attribution License (CC BY). The use, distribution or reproduction in other forums is permitted, provided the original author(s) and the copyright owner(s) are credited and that the original publication in this journal is cited, in accordance with accepted academic practice. No use, distribution or reproduction is permitted which does not comply with these terms.
*Correspondence: Raunak Dhanker, raunakbiotech@gmail.com