- 1Programa de Doctorado en Ciencia, Tecnología y Medio Ambiente, Escuela de Posgrado, Universidad Nacional del Altiplano Puno, Puno, Perú
- 2Laboratorio de Contaminantes Orgánicos y Ambiente del IINDEP de la Universidad Nacional de Moquegua, Moquegua, Perú
- 3Escuela profesional de Ingeniería Ambiental de la Universidad Nacional de Moquegua, Moquegua, Perú
Antibiotic residues have been found in environmental samples, such as water, soil, and even food, and usually come from wastewater, presenting environmental and human health risks. This study aimed to improve the elimination of the antibiotics tetracycline (Tet) and chloramphenicol (Chlor) by modifying three factors: contact time (3–7 days), plant biomass (10–14 g), and antibiotic concentration (5–15 mg/L Tet and 10–20 mg/L Chlor). An approach that optimizes time and resources, response surface methodology (RSM), was applied with a Box–Behnken design (BBD) to two plant species (L. gibba and A. filiculoides), i.e., one experimental design was used for each species. Antibiotic residues in water and plant samples were analyzed by liquid chromatography. The optimal conditions for Tet removal were 6.04 d, 11.4 g, and 13.4 mg/L with Lemna and 6.3 d, 11.9 g, and 14.7 mg/L with Azolla; the optimal conditions for Chlor removal were 7.8 d, 13.6 g, and 10.2 mg/L with Lemna and 4.6 d, 12.3 g, and 8.7 mg/L with Azolla. The results showed that the removal efficiency of antibiotics increased depending on the species used, reaching a maximum of up to 100%. Tet was better removed than Chlor, reaching maximum removal values of 100% and 84% with Azolla and Lemna, respectively. Chlor removal reached 70% and 64% with Azolla and Lemna, respectively. The mean bioconcentration factors (BCFs) of Tet were 2.9% in Lemna and 4.9% in Azolla, and the BCFs for Chlor were 38.1% in Lemna and 37.8% in Azolla. Thus, in general, better results were obtained with Azolla. In summary, the results demonstrate that this design and the selected plants contribute to the removal of antibiotics, presenting a sustainable and recommended alternative for the treatment of wastewater contaminated with antibiotic residues.
Highlights
• Azolla absorbs tetracycline and chloramphenicol in its structure better than Lemna.
• Tetracycline is also degraded by other abiotic factors.
• Chloramphenicol is better absorbed than tetracycline by plants.
• The longer the contact time is, the better the removal of antibiotics is.
• Intermediate amounts of biomass give better antibiotic removal results.
1 Introduction
In recent years, the residues of antibiotics such as chloramphenicol (Chlor) and tetracycline (Tet) have been found in aquatic ecosystems (Carvalho and Santos, 2016; Sorinolu et al., 2021). Such aquatic resources are contaminated with antibiotic residues from wastewater; given that after uptake, these compounds are not fully assimilated by the body, and a large percentage of ingested antibiotics is eliminated through excreta (Topal et al., 2016). Then, these residues reach wastewater, which is inefficiently treated in treatment plants, finally reaching the aquatic ecosystem, and are found in rivers, lakes, and marshes, among others (Pan et al., 2014; Topal et al., 2014; Baciak et al., 2016; Vilca et al., 2021). Unfortunately, insufficiently treated wastewater is not the only source of pollution; other sources include direct discharges of wastewater, landfill leachate, sewer leaks, manure storage ponds, runoff, and leaching of agricultural land contaminated with manure and direct discharges to crop fields (Carvalho and Santos, 2016). Therefore, natural degradation is not sufficient to degrade these quantities constantly.
Tet is one of the most commonly used antibiotics in human and veterinary medicine (Baciak et al., 2016) and is found in the environment, where it affects non-target species such as plants (Topal et al., 2014). Tet inhibits the growth of plants and alters the bacterial community (Brain et al., 2004; Maldonado et al., 2022). The danger of the presence of Chlor in the environment lies in its ability to generate resistance to antibiotics (Boonsaner and Hawker, 2013; Nguyen et al., 2022). Other secondary effects of Chlor on human health are associated with its carcinogenicity and the development of aplastic anemia (Bhattacharjee, 2016; Holanda et al., 2019; Yao et al., 2019; Reis et al., 2020), leukemia, and bone marrow suppression (Yao et al., 2019), which can sometimes be deadly (Bhattacharjee, 2016). This danger is due to the high lipophobicity of Chlor (pKa = 11) (Carvalho and Santos, 2016), which leads to easy bioaccumulation and biomagnification in living beings (Reis et al., 2020). For this reason, Chlor has been banned in the US and other developed countries; however, it is still being used in developing countries (Bhattacharjee, 2016; Yao et al., 2019).
Various removal methods exist, such as reverse osmosis, ozonation, photolysis/photocatalysis, adsorption, biodegradation by bacteria, ionizing radiation, and electrochemical oxidation (Zhao et al., 2019), which have high operating/maintenance costs (Amare et al., 2018). Phytoremediation is a technology based on plants that are capable of accumulating, translocating, and concentrating high amounts of toxic compounds in the aerial parts of their structure (Rahman and Hasegawa, 2011). Among them, the species L. gibba and A. filiculoides have been widely used in studies of the removal of toxic compounds (Baciak et al., 2016; Daud et al., 2018; Balarak et al., 2021), wastewater (Amare et al., 2018), and antibiotic residues with efficient results (Topal et al., 2014, Topal et al., 2016; Balarak et al., 2018; Bianchi et al., 2020; Topal et al., 2020; Balarak et al., 2021; Besharati et al., 2021).
Additionally, there are several environmental factors that influence the removal of these wastes, such as photodegradation, species biomass (Chattoraj et al., 2014; Naghipour et al., 2016; Pithawala and Jain, 2020), contact time with the compound (Chattoraj et al., 2014; Holanda et al., 2019; Pithawala and Jain, 2020), concentration in the medium (Chattoraj et al., 2014; Holanda et al., 2019), temperature, biodegradation, and adsorption on suspended solids and sediments (Carvalho and Santos, 2016; Topal et al., 2016; Holanda et al., 2019; Bianchi et al., 2020), as well as the phytoremediation process used (phytoextraction, phytostabilization, phytotransformation, volatilization, or rhizofiltration) (Rahman and Hasegawa, 2011). However, considering each factor individually is not enough to degrade these wastes. Given that, for example, antibiotics have toxic effects on various species (Kołodziejska et al., 2013; Carvalho and Santos, 2016), it is worth investigating whether the removal efficiency would improve if several environmental factors were controlled simultaneously.
Considering the complexity of the environmental factors that influence the degradation of antibiotics, these processes can be modeled by various statistical models, such as response surface methodology (RSM) (Jadhav et al., 2021). These methods are used to optimize treatment time and cost and involve the use of mathematical models and statistical techniques to find the optimum of each variable considered in the study (Samimi and Shahriari Moghadam, 2018; Foroughi et al., 2020). Such methodologies are rarely used in antibiotic removal processes with biological species. However, studies using these methodologies show better results than those without the application of this approach, as shown by one study of the biodegradation of Chlor that achieved 30% removal in a short period (24 h) (Holanda et al., 2019). Similarly, when Lemna was used to remove ammonium, up to 88% removal was achieved (Samimi and Shahriari Moghadam, 2018), as well as up to 89.2% phosphorus removal (Naghipour et al., 2016) and 86% lead removal were achieved (Pithawala and Jain, 2020). Relevant studies show that the application of Lemna and Azolla species with this experimental design can provide efficient results in antibiotic removal and offer another treatment alternative for target compounds, optimizing time and resources.
This study aimed to find an optimal combination of the parameters for the antibiotic’s removal (Tet and Chlor) considering three parameters (biomass, contact time, and antibiotic concentration) and two plant species through RSM, in order to study the effects of factor interaction, with two experimental group designs, one for Lemna and another for Azolla.
2 Materials and methods
2.1 Reagents and solvents
The antibiotics Tet and Chlor (98%) were obtained from Sigma-Aldrich. The salts for a quick, easy, cheap, effective, rugged, and safe (QuEChERS) method were obtained from Thermo Scientific, USA. Methanol (HPLC grade), acetonitrile (HPLC grade), and formic acid (HPLC grade) were obtained from BAKER ANALYZED.
2.2 Estimation of antibiotics in water and plant samples
A 1-ml water sample was filtered through a syringe filter (PTFE, 0.22 μm, 13 mm) and subjected to ultrahigh-performance liquid chromatography (UHPLC, Agilent 1290 Infinity II, United States) with diode ray detection (DAD) based on a previous study for the determination of the concentration of antibiotics (Santos et al., 2019). A 2-µl sample was injected into an InfinityLab Poroshell EC-C8 column (2.1 mm × 150 mm, 1.9-Microm, Agilent, United States) with a column temperature of 50°C. The mobile phase consisted of 0.1% formic acid in water (A) and 0.1% formic acid in acetonitrile (B). A gradient elution was performed: 0 min−90% B, 7 min −60% B. The flow of the mobile phase was 0.350 ml/min. The effluent of the column was monitored at 280.4 nm to detect the antibiotics. The limit of detection (LOD) and limit of quantification (LOQ) were 0.08 and 0.24 μg/ml for Tet, and 0.016 and 0.048 μg/ml for Chlor, respectively (Table 1). The five-point calibration curves (0.5, 1, 3, 6, and 10 μg/ml) showed good linearity for Tet (r2 = 0.9978) and Chlor (r2 = 1) in the range of the expected sample concentrations (Gomes et al., 2017).
For the determination of antibiotics in plants, the modified QuEChERS method was applied based on a previous study (He et al., 2018; Zhang et al., 2019) The plant samples were crushed in a mortar. A total of 10 g of the crushed sample was placed in a 50 ml centrifuge tube, 10 ml of acetonitrile was added, the mixture was homogenized, and then, 4 g of magnesium sulfate (MgSO4), 1 g of sodium chloride (NaCl), and 0.049 g of monosodium phosphate (NaH2PO4) were added. Then, the tube was closed and placed in a vortexer for 3 min. After ultrasonication for 20 min, the tube was centrifuged for 5 min at 3,500 rpm. One milliliter of the supernatant was transferred to a 2 ml centrifuge tube, and dispersive solid-phase extraction sorbents (25 mg PSA, 25 mg C18, 2.5 mg GCB, and 150 mg MgSO4) were added. The sample was placed in a vortexer for 1 min and then centrifuged for 5 min at 3,500 rpm. The supernatant was filtered through a 0.22-μm 13 mm PTFE syringe filter into a vial for the subsequent UHPLC analysis. The recovery percentages were 116.47% and 87.57% for Tet and Chlor, respectively.
2.3 Calculation methods
2.3.1 Water
The formula used to determine the removal percentage is the one reported by Amare et al. (2018):
where ci represents the initial concentration (mg/L) and cf denotes the concentration at the selected time (Bianchi et al., 2020).
2.3.2 Plant
The bioconcentration factor (BCF) was calculated as the ratio of the antibiotic concentration in plant tissues (µg/g) to the concentration in water (µg/L) at the end of the exposure phase (Boonsaner and Hawker, 2013; Boonsaner and Hawker, 2015).
2.4 Design of experiments and data analysis
A Box–Behnken design (BBD) with three levels and three variables was used to obtain the optimal conditions to maximize the percentage of antibiotic elimination (% eliminated). The factors were contact time (3–7 days), biomass (10–14 g), and antibiotic concentration (5–15 mg/L Tet and 10–20 mg/L Chlor). A total of 17 trials were conducted with RSM to investigate the effects of these processes. The experiments were performed in two groups, one for Lemna and one for Azolla, for a total of 34 tests. Two different responses were recorded: the percentage of Tet elimination (Y1) and the percentage of Chlor elimination (Y2) (Table 2).
where Y is the response variable; β0, βi, βi, and βij are the regression coefficients for the intercept, the linear term, the quadratic term, and the interaction, respectively; Xi and Xj are the independent factors; and n is the number of factors, which in this case is 3.
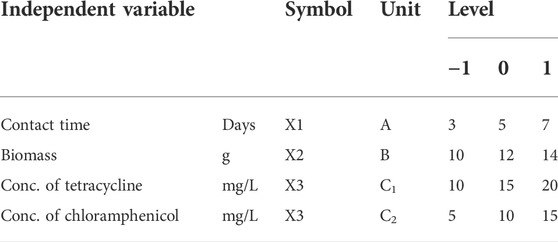
TABLE 2. Predictor’s variables and their values selected for the Box–Behnken design (BBD) for antibiotic removal.
2.5 Plant culture medium and experimental setup
The macrophytes were collected from the ecotourism boardwalk of Puno. They were previously cultured in a modified Swedish standard medium (SIS) for L. gibba and the same medium without nitrogen for Azolla (OECD, 2006; Garcia-Rodríguez et al., 2015).
The experiments were performed in plastic pots that were externally covered with aluminum to prevent the passage of light. Five hundred milliliters of the test solution with various concentrations of Tet (10, 15, and 20 mg/L) and Chlor (5, 10, and 15) was added to each plastic pot, with different amounts of biomass (10, 12, and 14 g) of L. gibba or A. filiculoides, corresponding to 34 pots in total, 17 for Lemna and 17 for Azolla. The daily lighting regime was 12 h of light: 12 h of darkness following the recommendations of the OECD (OECD, 2006). At days 3, 5, and 7 of exposure, some experimental units were selected, from which 1 ml of the water sample and 10 g of the plant biomass were sampled for analysis. The final biomass was also considered to determine the effect of antibiotics on plant growth.
2.6 Statistical analysis
The validity of each experimental series was assessed using analysis of variance (ANOVA) and the regression model corresponding to the response surface analysis. The optimal conditions were obtained by applying the predictive equation from the RSM analysis (Holanda et al., 2019). The analysis was performed using the rsm package in free software R Studio and version R 4.1.
3 Results
3.1 Evaluation of antibiotic degradation through an experimental design with L. gibba
3.1.1 Tetracycline
Duckweed is a plant with a high capacity to remove contaminants from the environment; in this case, it was applied in conjunction with other parameters for the removal of antibiotic compounds. A design with the response surface modeling was used to determine the optimal value of each parameter (contact time, biomass, and concentration) to improve the response variable, which was the percentage of antibiotic elimination. Table 3 shows the variables and their combinations, the response variables, and the predicted variables. In general, all combinations showed certain removal percentages, with Tet removals of 5%–84% and Chlor removals of 50%–64% with L. gibba. This indicates that a longer contact time has better removal results (Figure 3). The obtained and predicted data were compared, and R2 was 0.99 for Tet and 0.95 for Chlor, which confirms the suitability of the model obtained.
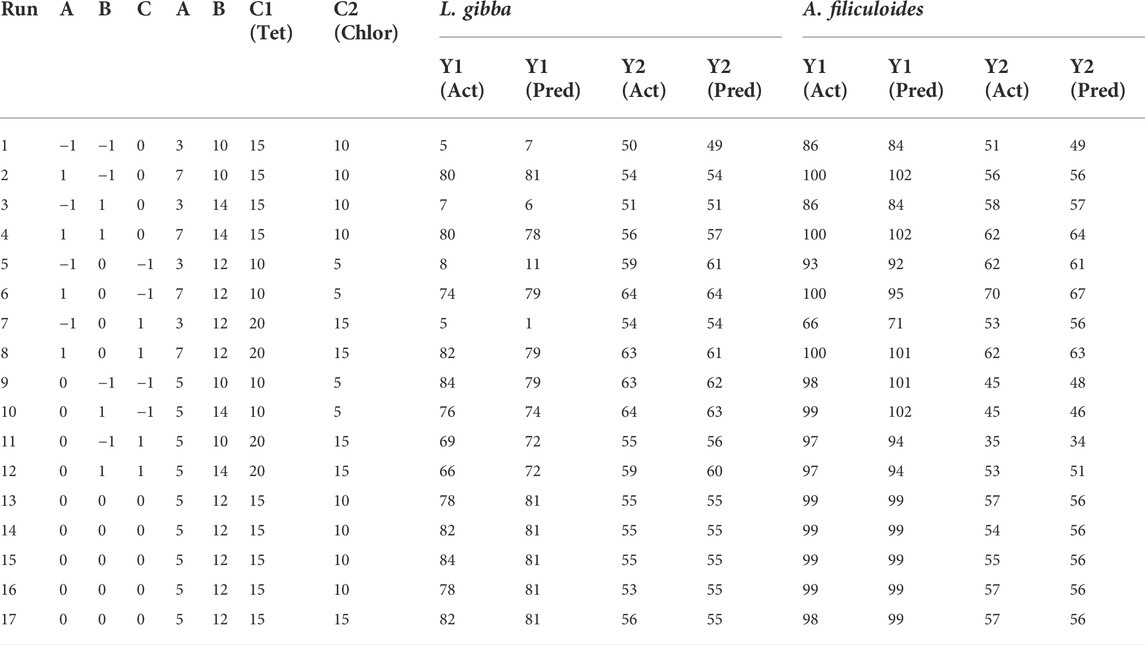
TABLE 3. Experimental design and responses based on the actual and predicted values with L. gibba and A. filiculoides, for tetracycline percentage removal (Y1) and chloramphenicol percentage removal (Y2).
According to Table 3, the variable with the greatest influence on the percentage of Tet removal in L. gibba was the contact time (p < 0.05), where the quadratic term for this variable was also significant (p < 0.05), while the other variables did not show statistical significance. Likewise, the intercept was significant [Pr (>|t|) = 0.02 and is < 0.05] with an R2 adj of 0.98, which suggests that the applied model is reliable. This result is corroborated by the ANOVA, in which first-order (FO) and pure quadratic (PQ) variables are significant for this model, while the interactions of the variables have no influence on the removal results.
3.1.2 Chloramphenicol
According to Tables 3, 4, the variable with the greatest influence on the percentage of Chlor removal in L. gibba is the concentration (p < 0.05), where the same is observed for the quadratic of concentration (p < 0.05), while the other variables do not show statistical significance. The R2 adj. is 0.886, which suggests that the applied model is reliable, and the comparison of the predicted data in Table 3 with the experimental data shows an R2 of 0.95. This result is corroborated by the ANOVA, in which the first order and quadratic variables are significant. Therefore, in the case of Chlor, the most influential variable is the concentration.
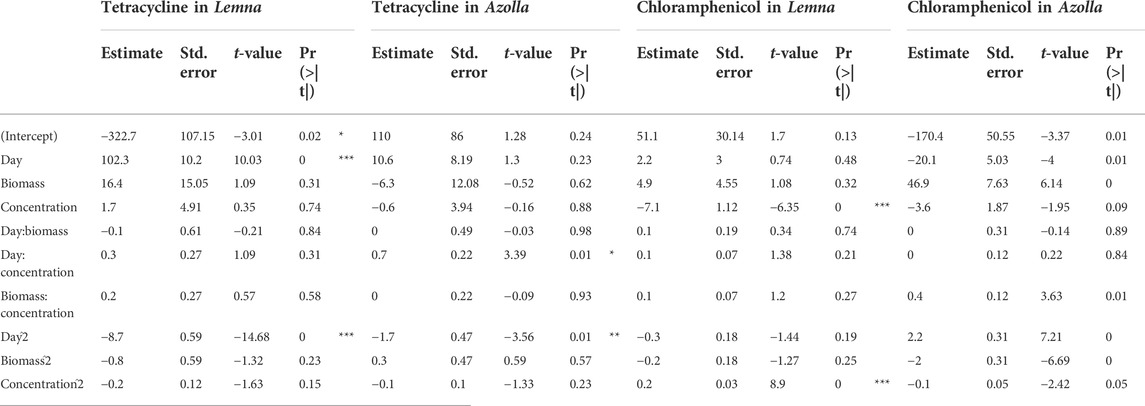
TABLE 4. Regression coefficients obtained for the RSM-generated model. Signif. codes: 0 ***; 0.001 **; 0.01 *.
3.2 Evaluation of antibiotic degradation with A. filiculoides through an experimental design
3.2.1 Tetracycline
In relation to the removal of Tet, the variable that most influences the removal percentage in A. filiculoides is the interaction of the variables’ contact time and concentration (p < 0.05), where the same is observed for the quadratic term of the contact time (p < 0.05) (Tables 4, 5), while the other variables do not show statistical significance. The model R2 adj. is 0.80, which suggests that the applied model is reliable, and the comparison of the predicted data in Table 3 with the experimental data shows an R2 of 0.91. The removal value reaches a maximum of 100%, although the BCF results indicate that the amount absorbed by this plant is minimal, with a maximum of 12% (Table 6). In this case, absorption in the tissues of the plant was not a relevant factor in Tet removal.
3.2.2 Chloramphenicol
The model of Chlor removal by Azolla was adequate with R2 = 0.90, and the variables that most influenced the removal percentage were contact time, biomass, and concentration, as well as the quadratic terms of all independent variables. This is corroborated by ANOVA, which shows that the contributions of the first-order variables, the pure quadratic (PQ), and the two-way interaction (TWI) were significant.
3.3 Effects of antibiotics on plant growth
Figure 1 shows the net increase in biomass influenced by the concentrations of the antibiotics Tet and Chlor. Lemna was more affected by increases in concentration over time, while for Azolla, although a decrease occurred on the fifth day, the biomass increased by up to 31.02% by the seventh day, which suggests that Azolla better tolerates the investigated antibiotic concentrations.
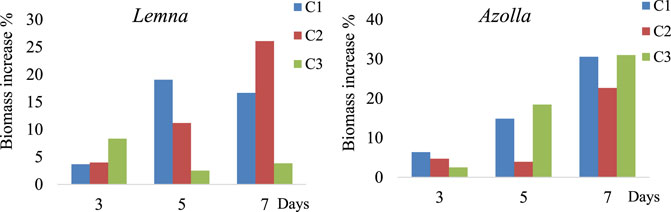
FIGURE 1. Biomass increment percentage in Lemna and Azolla during the experimental period of 7 days. With three concentrations, C1 = 5 and 10 mg/L, C2 = 10 and 15 mg/L, and C3 = 15 and 20 mg/L for tetracycline and chloramphenicol, respectively.
The BCF results showed that Tet was generally less absorbed by plants, with maximum values of 7.9% and 12.2% in Lemna and Azolla, respectively, while Chlor had maximum values of 57.3% and 58.3%, showing that Azolla absorbed a greater amount of both antibiotics.
3.4 Determination of the optimal points for the removal of antibiotics
3.4.1 Optimal point for the removal of antibiotics with L. gibba
The optimal conditions for Tet removal with L. gibba were contact time: 6 days, biomass: 11.4 g, and concentration: 13.4 mg/L, and the optimal conditions for Chlor removal were contact time: 7.8 days, biomass: 13.6 g, and concentration: 10.2 mg/L. Figure 2 presents the qualitative validation results. In the case of Tet, contact time was a very influential factor in the percentage of removal, even more than biomass, followed by the concentration of the antibiotic (Figures 2A–C). For Chlor, intermediate values of the contact time and biomass were observed (a), and in relation to biomass:concentration, better results were obtained at lower concentrations and intermediate biomass values between approximately 11 and 13 g. Regarding the interaction of contact time:concentration, better results were obtained with a greater number of days and lower concentration.
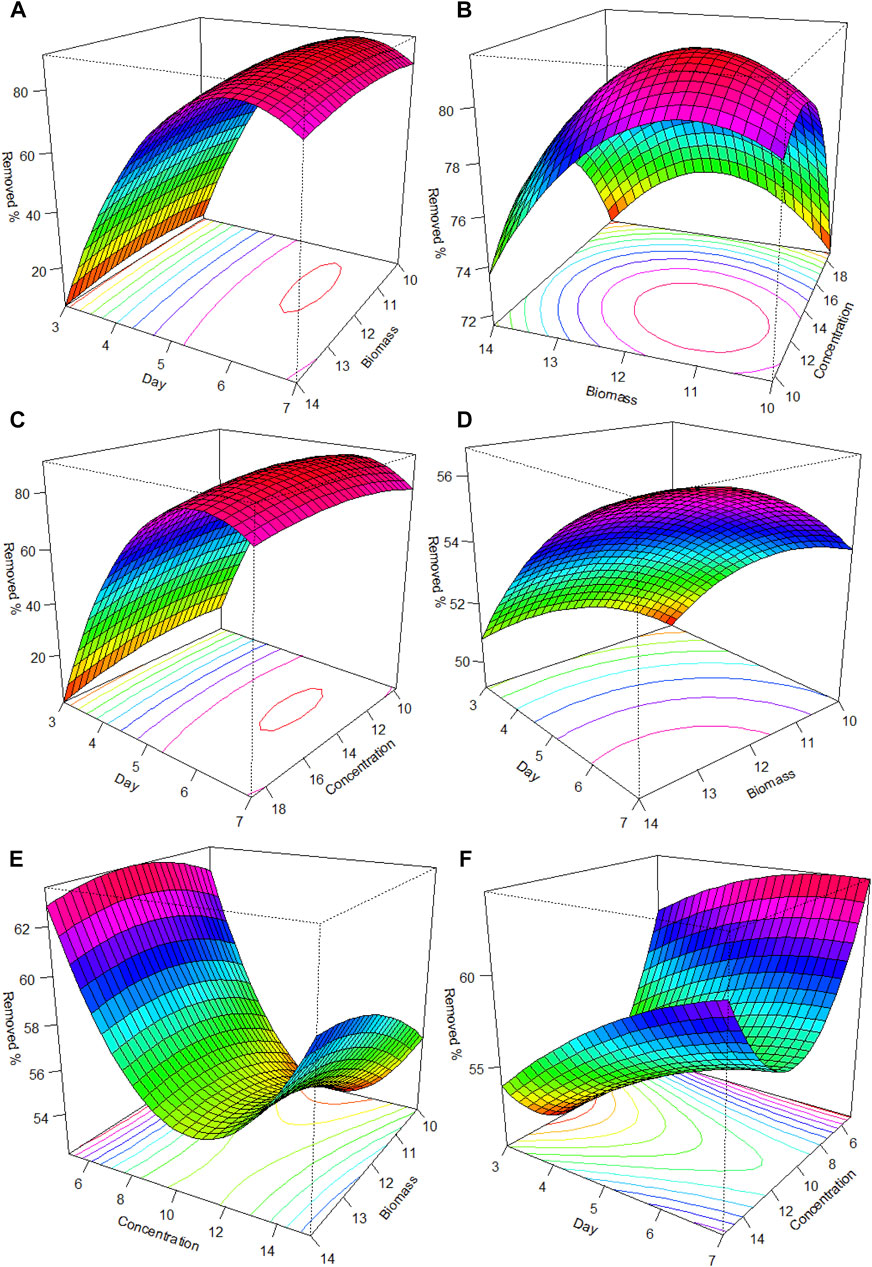
FIGURE 2. Response surface plot and contour plot of tetracycline percent removal, content as a function of (A) day and biomass; (B) biomass and concentration; and (C) day and concentration. For the percentage removal of chloramphenicol, content as a function of (D) day and biomass; (E) biomass and concentration; and (F) day and concentration.
3.4.2 Evaluation of antibiotic biodegradation through an experimental design with A. filiculoides
Regarding Tet and Chlor removal by Azolla, the optimal values were as follows: contact time: 6.3 days, biomass: 11.9 g, and concentration: 14.7 mg/L for Tet; and contact time: 4.6 days, biomass: 12.3 g, and concentration: 8.7 mg/L for Chlor. Figure 3 qualitatively shows the interactions and their effects on the removal percentage of the evaluated compounds. In general, for Tet, better percentages are observed at a greater number of days and the intermediate values for biomass (11–13 g) and concentration (12–16 mg/L). Similarly, for Chlor, the highest percentages of removal corresponded to a longer contact time, intermediate biomass, and lower antibiotic concentration.
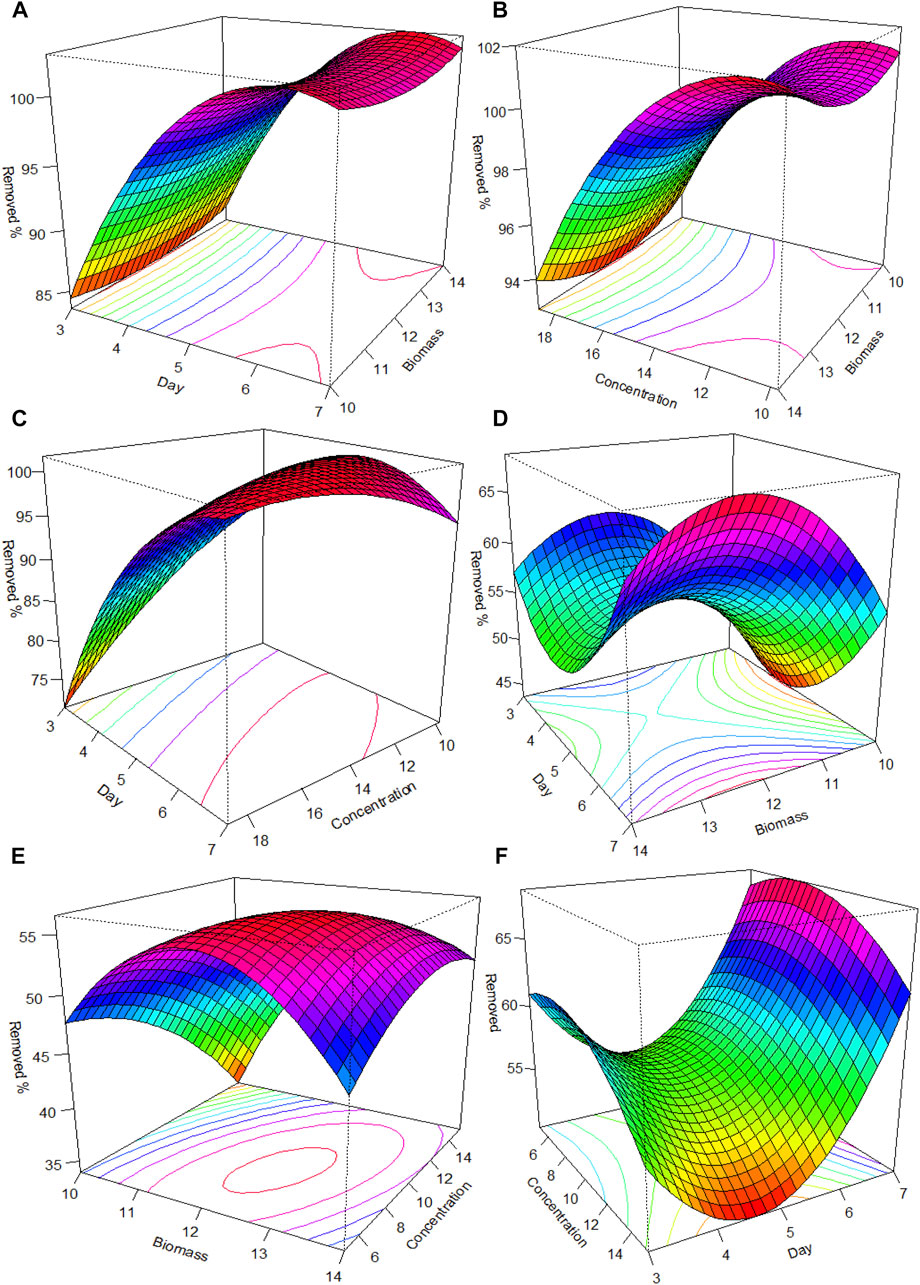
FIGURE 3. Response surface plot and contour plot of percent chloramphenicol removal, content as a function of (A) day and biomass; (B) biomass and concentration; and (C) day and concentration. For percent chloramphenicol removal, content as a function of (D) day and biomass; (E) biomass and concentration; and (F) day and concentration.
4 Discussion
4.1 Evaluation of antibiotic degradation through an experimental design with L. gibba
4.1.1 Tetracycline
Treatment plants remove toxic compounds from the aquatic environment. However, when the supply of these pollutants is constant and abundant, the degradation capacity decreases greatly (Topal et al., 2016). An additional method, such as phytoremediation, is required to mitigate pollutant concentrations. The results of the current experimental design show efficient results in all combinations, which suggests that plants play a very important role. However, among the variables used, only the individual and quadratic terms for contact time were significant, not the other variables. This may be because, in general, the ranges of the variables analyzed were very narrow; for example, the intervals between the biomass values were 2 g, and the intervals for the concentration values were 5 mg.
Similarly, in a study in which Tet was removed with L. gibba, three initial concentrations (50, 100, and 300 μg/L) were analyzed with 5 g of Lemna in each experimental unit, and different hydraulic retention times (HRTs; up to 10 days) were considered; the removal results for the three concentrations were 99.8 ± 4.1, 99.9 ± 4.9, and 99 ± 4.9%, respectively, at 10 days (Topal et al., 2020). Thus, close values of the independent variables can provide similar removal results. In a study in which oxytetracycline (1 μg/L) was removed with Lemna minor, the plant showed the ability to tolerate stress and absorb oxytetracycline in its structure, resulting in a 79% removal in 14 days (Gomes et al., 2020). The reason for the lower removal percentage and longer time may be because, in the study mentioned, a less amount biomass of Lemna was used (20 individuals with two fronds each, corresponding to a few grams) (Gomes et al., 2020). Additionally, in another study with L. gibba, only 85% of Tet was removed after 13 days of exposure (Topal et al., 2014).
Additionally, the BCF values for Tet were low (Table 6), with an average of 2.9%, which indicates that there are other factors, such as hydrolysis and photodegradation (Topal et al., 2016; Bianchi et al., 2020). In contrast, in a study conducted by Topal et al. (2014), photodegradation and other environmental factors did not influence Tet removal in the control group, although this may be due to the HRT, which was 1.31 h in the experimental unit; in contrast, in this study, the minimum HRT was 3 days, and the maximum was 7 days. Additionally, these low BCF values may be due to the high concentrations used in the experiment, which could have phytotoxic effects on plants, since there is ample evidence of the phytotoxic effects of antibiotics on plants, mainly affecting their growth (Iatrou et al., 2017), causing chlorosis in leaves (Iatrou et al., 2015), and promoting the production of peroxide (H2O2), which in high quantities is toxic to plants (Gomes et al., 2017; Gomes et al., 2020). Despite this, this plant showed the ability to absorb this antibiotic and continue to increase its biomass (Figure 1). The other reason for the low concentrations of antibiotics in the tissues was phytovolatilization, which consists of plants absorbing pollutants, transferring them to the aerial parts and volatilizing the pollutants in the air (Rahman and Hasegawa, 2011). This mechanism increases the reliability of plant use for phytoremediation; even using dry biomass as a Tet adsorbent can produce efficient results in a few hours (Balarak et al., 2018). Therefore, not only wet biomass but also dry biomass can be useful in the removal of this compound.
4.1.2 Chloramphenicol
The results indicate that the first-order and quadratic variables are significant. Therefore, in the case of Chlor, the most influential variable is the concentration. Similarly, in a study in which this same RSM was applied to Chlor biodegradation, it was found that the variables that most influenced Chlor removal were concentration and time (Holanda et al., 2019). Even in adsorption tests with chemical compounds, concentration is a very important factor, so this variable is often considered in laboratory tests (Nguyen et al., 2022), as in the case of Chlor concentrations of 0.1–0.3 mmol/L (Li et al., 2018); 30, 50, 100 mg/L (Yao et al., 2019), and 5, 10, 20, 30, and 50 mg/L (Xiao et al., 2021), to evaluate the best concentrations for removal by the different methodologies applied. This is understandable given that high concentrations tend to exceed the remediation capacity of plants. Chlor has toxic effects on plants, as shown by toxicity studies demonstrating the phytotoxic effects of antibiotics at high concentrations. For example, in the case of Scenedesmus ubliquus, its growth decreased by 50% (Xiong et al., 2019); a similar phenomenon occurred in this study. In general, an inhibition of biomass was observed with an increasing concentration of antibiotics (Figure 1).
4.2 Evaluation of antibiotic degradation through an experimental design with A. filiculoides
4.2.1 Tetracycline
The results show that the plant was not an important factor in the removal of Tet. Therefore, other factors influenced Tet degradation. In the case of Azolla, one such factor is biodegradation by the symbiotic cyanobacteria associated with Azolla, i.e., Anabaena azollae and Arthrobacter (Forni et al., 1991; Forni et al., 2002; Bianchi et al., 2020), which, despite being affected by antibiotics, usually contribute to antibiotic degradation (Forni et al., 2002). In one study, the population of Arthrobacter increased with an increase in the amount of the antibiotic in treatment systems with plants, which contributed to the removal of the antibiotic from the medium (Tong et al., 2019).
Studies of Tet removal with Azolla wet biomass are scarce. However, studies in which Azolla is used to remove antibiotics show efficient results, such as in the study by Bianchi et al. (2020); not only was levofloxacin removed from the medium (1 μg/L), but an increase in Azolla biomass was stimulated. In another study, in which Azolla was exposed to sulfadimethoxine at high concentrations (50, 150, 300, and 450 mg/L), an increase in bioadsorption occurred as a function of increasing the dose. Despite sulfadimethoxine having toxic effects on the plant, Azolla was able to survive, increase its biomass after an initial adaptation process, and remove the tested antibiotic from the environment (Forni et al., 2002). Therefore, concentration and time influence the removal rate from an aquatic environment. Additionally, the dry biomass of Azolla was used in one study as an adsorbent to remove Tet from the aquatic environment with efficient results; the biomass of Azolla and other variables such as the initial concentration of Tet, contact time, and temperature positively influenced the removal of Tet from the aquatic environment (Mahvi et al., 2018). However, in another study, in which Azolla biomass was also used as an adsorbent, the removal results were lower than those obtained with other adsorbents (Besharati et al., 2021). This indicates that in general, living plant biomass offers better results, as was also evidenced in this study.
4.2.2 Chloramphenicol
The results indicate that the variables contact time:biomass and the quadratic terms of all the independent variables influenced the removal process. Similarly, a study in which Azolla was exposed to a drug (diclofenac) at different doses (0.1, 1, 10, and 100 mg/L) for different periods of time (24, 48, 72, and 240 h) found that time and dose influenced the toxicity of Azolla, resulting in lower removal for the medium (Vannini et al., 2018). Another study showed that a higher concentration decreased the removal rate (Xiao et al., 2021).
The high removal percentages indicate that the combination of several factors under this design is efficient and sustainable for the removal of toxic compounds since it allows the optimization of time and resources (Holanda et al., 2019). Similar conclusions were obtained for the removal of ammonium (Samimi and Shahriari Moghadam, 2018), phosphorus (Naghipour et al., 2016), and lead (Pithawala and Jain, 2020). Therefore, this study highlights the benefits of using floating plants in phytoremediation (Rahman and Hasegawa, 2011). Phytoabsorption is an efficient mechanism for the removal of toxic compounds that is also relevant to Lemna and Azolla mainly because they are floating plants that are in direct contact with pollutants through the roots. The roots are a suitable place for bacterial development because plants release exudates (Kotyza et al., 2010), favoring bacterial activity in the process of degradation of toxic compounds (Hijosa-Valsero et al., 2011; Martin et al., 2014; Santos et al., 2019) through rhizoremediation (Martin et al., 2014).
The results show that this approach with floating species is not only ecologically viable but also sustainable over time once the optimal species are identified. This alternative treatment technology is self-sufficient and economical (Daud et al., 2018). In addition, the species used can easily adapt to different environmental conditions (Rahman and Hasegawa, 2011) and are even considered to be among the most prolific species in the world (Rahman and Hasegawa, 2011). These results indicate that the application of the current approach in the removal of antibiotics is viable mainly for developing and emerging countries in which such contamination problems are more critical (Bhattacharjee, 2016; Yao et al., 2019).
4.3 Effects of antibiotics on plant growth
The results suggest that Azolla tolerates the investigated concentrations of antibiotic contaminations better than Lemna. Similarly, one study observed that after 3 days of exposure to Tet, L. gibba showed chlorosis at 1 mg/L, which resulted in a decrease in biomass (Brain et al., 2004). In another study, the EC50 (average effective dose) was 3.26 mg/L oxytetracycline, which thus affected the increase in biomass (Kołodziejska et al., 2013). These species were also tested in other studies with antibiotics; for example, Bianchi et al. (2020) found that levofloxacin at 1 μg/L had stimulatory effects on plant growth (Lemna minuta and A. filiculoides) after 8 days of exposure. In another study in which Azolla was exposed to an antibiotic (sulfadimethoxine), the increase in biomass after 5 weeks of exposure had a logistic relationship with dose (Forni et al., 2002).
4.4 Bioconcentration factor
Bioconcentration studies of antibiotics show that Chlor is well absorbed by plants, as evidenced in a study that analyzed crop plants with wastewater irrigation and found that Chlor had a relatively high BCF for different crops, such as rice, corn, and Chinese radish (Pan et al., 2014; Sorinolu et al., 2021). It is also worth mentioning that plants contribute to the removal of antibiotic compounds, decreasing their toxicity and concentration (Kotyza et al., 2010) by the biotransformation of metabolites through enzymes (Kurade et al., 2021) and by sequestration through antibiotic storage in plant organelles such as the vacuole (Shitan and Yazaki, 2020), plasma membrane, extracellular wall, peripheral extracellular space, and cell wall (Maldonado et al., 2022). Simultaneously, some residual metabolites are eliminated in the form of CO2 (Kurade et al., 2021). Additionally, the results showed that Azolla stores more antibiotics than Lemna. This could be due to symbiotic bacteria such as Anabaena and Arthrobacter (Forni et al., 1991; Maldonado et al., 2022). These bacteria promote the ability of plants to tolerate and assimilate antibiotic compounds, as evidenced in other studies in which the absorption of antibiotics increased as a function of increasing antibiotic concentration (Forni et al., 2002). It may even be possible for plant growth to increase in media with antibiotics (Bianchi et al., 2020).
5 Conclusion
In this study, it was found that Lemna removes Tet (84%) better than Chlor (64%), and the most influential factor is the contact time. Similarly, Azolla removes Tet (100%) better than Chlor (70%), and the variables that have the greatest influence are contact time for Tet and contact time, biomass, and concentration for Chlor. Regarding the variables considered, the intermediate values of biomass and time and the lower values of antibiotic concentration generally produced better results. In relation to the increase in biomass, Azolla showed better results than Lemna. Similarly, regarding BCF, Tet bioaccumulates more than Chlor, and in the case of both antibiotics, Azolla accumulates more than Lemna. The fact that all the tested concentrations were partially removed indicates that higher concentrations of these antibiotics can be used for their removal. This model can also be used as a prediction guide for the use of floating species such as Lemna and Azolla as antibiotic absorbents under different environmental conditions, and can even be applied in real or pilot scale.
Data availability statement
The original contributions presented in the study are included in the article/Supplementary Material; further inquiries can be directed to the corresponding author.
Author contributions
IM: contributed to the conception and planning of the research, performed the experiments, analyzed the data, and wrote the manuscript. AV: contributed to developing an antibiotic analysis method in chromatography and data collection. DC: contributed to developing an antibiotic analysis method in chromatography and data collection. FV: contributed to the conception and planning of the research, critically reviewed the manuscript, and checked the format. All authors have read and agreed to the final contents of the manuscript.
Funding
The authors are grateful for the financial support by the Concytec—World Bank Project “Improvement and Expansion of the Services of the National System of Science, Technology and Technological Innovation” 8682-PE, through its executing unit ProCiencia (contract number 01-2018-FONDECYT/BM-PhD Programs in Strategic and General Areas).
Acknowledgments
The authors would like to thank the Universidad Nacional de Moquegua and the project “Presencia de residuos de antibióticos en el rio Osmore, en agua potable de las ciudades de Ilo y Moquegua y su relación con la salud en la población”, approved by resolution of the organizing committee N° 0310-2020-UNAM, which allowed the implementation of the laboratory where the chromatographic analysis was performed and at the same time financed the present publication.
Conflict of interest
The authors declare that the research was conducted in the absence of any commercial or financial relationships that could be construed as a potential conflict of interest.
Publisher’s note
All claims expressed in this article are solely those of the authors and do not necessarily represent those of their affiliated organizations, or those of the publisher, the editors, and the reviewers. Any product that may be evaluated in this article, or claim that may be made by its manufacturer, is not guaranteed or endorsed by the publisher.
References
Amare, E., Kebede, F., and Mulat, W. (2018). Wastewater treatment by Lemna minor and Azolla filiculoides in tropical semi-arid regions of Ethiopia. Ecol. Eng. 120, 464–473. doi:10.1016/j.ecoleng.2018.07.005
Baciak, M., Sikorski, Ł., Piotrowicz-Cieślak, A. I., and Adomas, B. (2016). Content of biogenic amines in Lemna minor (common duckweed) growing in medium contaminated with tetracycline. Aquat. Toxicol. 180, 95–102. doi:10.1016/j.aquatox.2016.09.007
Balarak, D., Mostafapour, F., and Khatibi, A. (2018). Nonlinear isotherms and kinetics and application error functions for adsorption of tetracycline on Lemna minor. J. Pharm. Res. Int. 23, 1–11. doi:10.9734/jpri/2018/42583
Balarak, D., Mahvi, A. H., Shahbaksh, S., Wahab, M. A., and Abdala, A. (2021). Adsorptive removal of azithromycin antibiotic from aqueous solution by Azolla filiculoides-based activated porous carbon. Nanomaterials 11, 3281. doi:10.3390/nano11123281
Besharati, N., Alizadeh, N., and Shariati, S. (2021). Removal of tetracycline from aqueous solution by Azolla, fig leaves, eggshell and egg membrane modified with magnetite nanoparticles. Desalin. Water Treat. 225, 214–224. doi:10.5004/dwt.2021.27023
Bhattacharjee, M. K. (2016). Chemistry of antibiotics and related drugs. Cham: Springer International Publishing. doi:10.1007/978-3-319-40746-3
Bianchi, E., Biancalani, A., Berardi, C., Antal, A., Fibbi, D., Coppi, A., et al. (2020). Improving the efficiency of wastewater treatment plants: bio-removal of heavy-metals and pharmaceuticals by Azolla filiculoides and Lemna minuta. Sci. Total Environ. 746, 141219. doi:10.1016/j.scitotenv.2020.141219
Boonsaner, M., and Hawker, D. W. (2013). Evaluation of food chain transfer of the antibiotic oxytetracycline and human risk assessment. Chemosphere 93, 1009–1014. doi:10.1016/j.chemosphere.2013.05.070
Boonsaner, M., and Hawker, D. W. (2015). Transfer of oxytetracycline from swine manure to three different aquatic plants: implications for human exposure. Chemosphere 122, 176–182. doi:10.1016/j.chemosphere.2014.11.045
Brain, R. A., Johnson, D. J., Richards, S. M., Sanderson, H., Sibley, P. K., Solomon, K. R., et al. (2004). Effects of 25 pharmaceutical compounds to Lemna gibba using a seven-day static-renewal test. Environ. Toxicol. Chem. 23, 371. doi:10.1897/02-576
Carvalho, I. T., and Santos, L. (2016). Antibiotics in the aquatic environments: a review of the European scenario. Environ. Int. 94, 736–757. doi:10.1016/j.envint.2016.06.025
Chattoraj, S., Mondal, N. K., Das, B., Roy, P., and Sadhukhan, B. (2014). Carbaryl removal from aqueous solution by Lemna major biomass using response surface methodology and artificial neural network. J. Environ. Chem. Eng. 2, 1920–1928. doi:10.1016/j.jece.2014.08.011
Daud, M. K., Ali, S., Abbas, Z., Zaheer, I. E., Riaz, M. A., Malik, A., et al. (2018). Potential of duckweed (Lemna minor) for the phytoremediation of landfill leachate. J. Chem. 2018, 1–9. doi:10.1155/2018/3951540
Forni, C., Tel-Or, E., Grilli, M., and Grilli Caiola, M. (1991). Effects of antibiotic treatments on azolla-anabaena and arthrobacter. Plant Soil 137, 151–155. doi:10.1007/BF02187447
Forni, C., Cascone, A., Fiori, M., and Migliore, L. (2002). Sulphadimethoxine and Azolla filiculoides lam.: a model for drug remediation. Water Res. 36, 3398–3403. doi:10.1016/S0043-1354(02)00015-5
Foroughi, M., Rahmani, A. R., Asgari, G., Nematollahi, D., Yetilmezsoy, K., y Samarghandi, M. R., et al. (2020). Optimization and modeling of tetracycline removal from wastewater by three-dimensional electrochemical system: application of response surface methodology and least squares support vector machine. Environ. Model. Assess. 25, 327–341. doi:10.1007/s10666-019-09675-9
Garcia-Rodríguez, A., Matamoros, V., Fontàs, C., and Salvadó, V. (2015). The influence of Lemna sp. and Spirogyra sp. on the removal of pharmaceuticals and endocrine disruptors in treated wastewaters. Int. J. Environ. Sci. Technol. 12, 2327–2338. doi:10.1007/s13762-014-0632-x
Gomes, M. P., Gonçalves, C. A., de Brito, J. C. M., Souza, A. M., da Silva Cruz, F. V., Bicalho, E. M., et al. (2017). Ciprofloxacin induces oxidative stress in duckweed (Lemna minor L.): implications for energy metabolism and antibiotic-uptake ability. J. Hazard. Mat. 328, 140–149. doi:10.1016/j.jhazmat.2017.01.005
Gomes, M. P., Moreira Brito, J. C., Cristina Rocha, D., Navarro-Silva, M. A., and Juneau, P. (2020). Individual and combined effects of amoxicillin, enrofloxacin, and oxytetracycline on Lemna minor physiology. Ecotoxicol. Environ. Saf. 203, 111025. doi:10.1016/j.ecoenv.2020.111025
He, Z., Wang, Y., Xu, Y., and Lu, X. (2018). Determination of antibiotics in vegetables using QuEChERS-based method and liquid chromatography- quadrupole linear ion trap mass spectrometry. Food Anal. Methods 11, 2857–2864. doi:10.1007/s12161-018-1252-8
Hijosa-Valsero, M., Fink, G., Schlüsener, M. P., Sidrach-Cardona, R., Martín-Villacorta, J., Ternes, T., et al. (2011). Removal of antibiotics from urban wastewater by constructed wetland optimization. Chemosphere 83, 713–719. doi:10.1016/j.chemosphere.2011.02.004
Holanda, F. H. E., Birolli, W. G., Morais, E., dos, S., Sena, I. S., Ferreira, A. M., et al. (2019). Study of biodegradation of chloramphenicol by endophytic fungi isolated from Bertholletia excelsa (Brazil nuts). Biocatal. Agric. Biotechnol. 20, 101200. doi:10.1016/j.bcab.2019.101200
Iatrou, E., Gatidou, G., Damalas, D., Thomaidis, N., and Stasinakis, A. (2017). Fate of antimicrobials in duckweed Lemna minor wastewater treatment systems. J. Hazard. Mat. 330, 116–126. doi:10.1016/j.jhazmat.2017.02.005
Iatrou, E. I., Stasinakis, A. S., Aloupi, M., and Thomaidis, N. (2015). Cultivating duckweed Lemna minor in urine and treated domestic wastewater for simultaneous biomass production and removal of nutrients and antimicrobials. Ecological Engineering 84, 632–639. doi:10.1016/j.ecoleng.2015.09.071
Jadhav, D. A., Carmona-Martínez, A. A., Chendake, A. D., Pandit, S., and Pant, D. (2021). Modeling and optimization strategies towards performance enhancement of microbial fuel cells. Bioresour. Technol. 320, 124256. doi:10.1016/j.biortech.2020.124256
Kołodziejska, M., Maszkowska, J., Białk-Bielińska, A., Steudte, S., Kumirska, J., Stepnowski, P., et al. (2013). Aquatic toxicity of four veterinary drugs commonly applied in fish farming and animal husbandry. Chemosphere 92, 1253–1259. doi:10.1016/j.chemosphere.2013.04.057
Kotyza, J., Soudek, P., Kafka, Z., and Vaněk, T. (2010). Phytoremediation of pharmaceuticals-preliminary study. Int. J. Phytoremediation 12, 306–316. doi:10.1080/15226510903563900
Kurade, M. B., Ha, Y. H., Xiong, J. Q., Govindwar, S. P., Jang, M., y Jeon, B. H., et al. (2021). Phytoremediation as a green biotechnology tool for emerging environmental pollution: a step forward towards sustainable rehabilitation of the environment. Chem. Eng. J. 415, 129040. doi:10.1016/j.cej.2021.129040
Li, Y., Zhang, J., and Liu, H. (2018). Removal of chloramphenicol from aqueous solution using low-cost activated carbon prepared from Typha orientalis. Water (Switzerland) 10, 351. doi:10.3390/w10040351
Mahvi, A. H., Mostafapour, F. K., and Balarak, D. (2018). Biosorption of tetracycline from aqueous solution by Azolla Filiculoides: equilibrium kinetic and thermodynamics studies. Fresenius Environ. Bull. 27, 5759–5857. Available at: https://eprints.zaums.ac.ir/190/.
Maldonado, I., Moreno, E. G., and y Zirena, F. (2022). Application of duckweed (Lemna sp.) and water fern (Azolla sp.) in the removal of pharmaceutical residues in water: state of art focus on antibiotics. Sci. Total Environ. 838, 156565. doi:10.1016/j.scitotenv.2022.156565
Martin, B. C., George, S. J., Price, C. A., Ryan, M. H., and y Tibbett, M. (2014). The role of root exuded low molecular weight organic anions in facilitating petroleum hydrocarbon degradation: current knowledge and future directions. Sci. Total Environ. 472, 642–653. doi:10.1016/j.scitotenv.2013.11.050
Naghipour, D., Taghavi, K., Jaafari, J., Mahdavi, Y., Ghanbari Ghozikali, M., Ameri, R., et al. (2016). Statistical modeling and optimization of the phosphorus biosorption by modified Lemna minor from aqueous solution using response surface methodology (RSM). Desalination Water Treat. 57, 19431–19442. doi:10.1080/19443994.2015.1100555
Nguyen, L. M., Nguyen, N. T. T., Nguyen, T. T. T., Nguyen, T. T., Nguyen, D. T. C., and Tran, T. V. (2022). Occurrence, toxicity and adsorptive removal of the chloramphenicol antibiotic in water: a review. Environ. Chem. Lett. 25, 1929–1963. doi:10.1007/s10311-022-01416-x
OECD (2006). Test No. 221: Lemna sp. growth inhibition test. OECD Guidelines for the Testing of Chemicals, Section 2. Available at: http://www.oecd-ilibrary.org/environment/test-no-221-lemna-sp-growth-inhabition-test_9789264016194-en (Accessed April 25, 2022).
Pan, M., Wong, C. K. C., and Chu, L. M. (2014). Distribution of antibiotics in wastewater-irrigated soils and their accumulation in vegetable crops in the Pearl River Delta, Southern China. J. Agric. Food Chem. 62, 11062–11069. doi:10.1021/jf503850v
Pithawala, N. A., and Jain, B. (2020). Application of response surface methodology for optimization of lead (ii) removal from aqueous solution by Lemna major biomass. J. Adv. Sci. Res. 11, 232–242. Available at: http://www.sciensage.info/journal/1359303580JASR_3006121.pdf.
Rahman, M. A., and Hasegawa, H. (2011). Aquatic arsenic: Phytoremediation using floating macrophytes. Chemosphere 83, 633–646. doi:10.1016/j.chemosphere.2011.02.045
Reis, A. C., Kolvenbach, B. A., Nunes, O. C., and Corvini, P. F. X. (2020). Biodegradation of antibiotics: the new resistance determinants-part I. New Biotechnol. 54, 34–51. doi:10.1016/j.nbt.2019.08.002
Samimi, M., and Shahriari Moghadam, M. (2018). Optimal conditions for the biological removal of ammonia from wastewater of a petrochemical plant using the response surface methodology. Glob. J. Environ. Sci. Manag. 4, 315–324. doi:10.22034/GJESM.2018.03.005
Santos, F., Mucha, A. P., Alexandrino, D. A. M., Almeida, C. M. R., and Carvalho, M. F. (2019). Biodegradation of enrofloxacin by microbial consortia obtained from rhizosediments of two estuarine plants. J. Environ. Manage. 231, 1145–1153. doi:10.1016/j.jenvman.2018.11.022
Shitan, N., and Yazaki, K. (2020). Dynamism of vacuoles toward survival strategy in plants. Biochim. Biophys. Acta Biomembr. 1862, 183127. doi:10.1016/j.bbamem.2019.183127
Sorinolu, A. J., Tyagi, N., Kumar, A., and Munir, M. (2021). Antibiotic resistance development and human health risks during wastewater reuse and biosolids application in agriculture. Chemosphere 265, 129032. doi:10.1016/j.chemosphere.2020.129032
Tong, X., Wang, X., He, X., Xu, K., and Mao, F. (2019). Effects of ofloxacin on nitrogen removal and microbial community structure in constructed wetland. Sci. Total Environ. 656, 503–511. doi:10.1016/j.scitotenv.2018.11.358
Topal, M., Senel, G. U., Obek, E., and Arslan Topal, E. I. (2014). Removal of tetracycline and the degradation products by Lemna gibba L. Exposed to secondary effluent. Environ. Prog. Sustain. Energy 33, 676–680. doi:10.1002/ep
Topal, M., Uslu Şenel, G., Öbek, E., and Arslan Topal, E. I. (2016). Investigation of relationships between removals of tetracycline and degradation products and physicochemical parameters in municipal wastewater treatment plant. J. Environ. Manage. 173, 1–9. doi:10.1016/j.jenvman.2016.02.046
Topal, M., Öbek, E., Uslu Şenel, G., and Arslan Topal, E. I. (2020). Removal of tetracycline antibiotic by Lemna gibba L. from aqueous solutions. Water Environ. J. 34, 37–44. doi:10.1111/wej.12439
Vannini, A., Paoli, L., Vichi, M., Bačkor, M., Bačkorová, M., Loppi, S., et al. (2018). Toxicity of diclofenac in the Fern Azolla filiculoides and the lichen Xanthoria parietina. Bull. Environ. Contam. Toxicol. 100, 430–437. doi:10.1007/s00128-017-2266-4
Vilca, F. Z., Galarza, N. C., Tejedo, J. R., Cuba, W. A. Z., Quiróz, C. N. C., Tornisielo, V. L., et al. (2021). Occurrence of residues of veterinary antibiotics in water, sediment and trout tissue (Oncorhynchus mykiss) in the southern area of Lake Titicaca, Peru. J. Great Lakes. Res. 47 (4), 1219–1227. doi:10.1016/j.jglr.2021.04.012
Xiao, L., Li, J., Lichtfouse, E., Li, Z., Wang, Q., Liu, F., et al. (2021). Augmentation of chloramphenicol degradation by Geobacter-based biocatalysis and electric field. J. Hazard. Mat. 410, 124977. doi:10.1016/j.jhazmat.2020.124977
Xiong, Q., Hu, L. X., Liu, Y. S., Wang, T. T., and Ying, G. G. (2019). New insight into the toxic effects of chloramphenicol and roxithromycin to algae using FTIR spectroscopy. Aquat. Toxicol. 207, 197–207. doi:10.1016/j.aquatox.2018.12.017
Yao, B., Liu, Y., and Zou, D. (2019). Removal of chloramphenicol in aqueous solutions by modified humic acid loaded with nanoscale zero-valent iron particles. Chemosphere 226, 298–306. doi:10.1016/j.chemosphere.2019.03.098
Zhang, C., Deng, Y., Zheng, J., Zhang, Y., Yang, L., Liao, C., et al. (2019). The application of the QuEChERS methodology in the determination of antibiotics in food: a review. Trends Anal. Chem. 118, 517–537. doi:10.1016/j.trac.2019.06.012
Keywords: Biomass, Chloramphenicol, Concentration, Duckweed, Plant Uptake, Tetracycline, Water Fern
Citation: Maldonado I, Vega Quispe AP, Merma Chacca D and Zirena Vilca F (2022) Optimization of the elimination of antibiotics by Lemna gibba and Azolla filiculoides using response surface methodology (RSM). Front. Environ. Sci. 10:940971. doi: 10.3389/fenvs.2022.940971
Received: 10 May 2022; Accepted: 08 July 2022;
Published: 22 August 2022.
Edited by:
Juliana Heloisa Pinê Américo-Pinheiro, Brazil University, BrazilReviewed by:
Noureddine El Messaoudi, Université Ibn Zohr, MoroccoVineet Kumar, National Environmental Engineering Research Institute (CSIR), India
Edwin Hualpa Cutipa, National University of San Marcos, Peru
Copyright © 2022 Maldonado, Vega Quispe, Merma Chacca and Zirena Vilca. This is an open-access article distributed under the terms of the Creative Commons Attribution License (CC BY). The use, distribution or reproduction in other forums is permitted, provided the original author(s) and the copyright owner(s) are credited and that the original publication in this journal is cited, in accordance with accepted academic practice. No use, distribution or reproduction is permitted which does not comply with these terms.
*Correspondence: Franz Zirena Vilca, ZnppcmVuYXZAdW5hbS5lZHUucGU=