- 1School of Natural Resources and the Environment, University of Arizona, Tucson, AZ, United States
- 2Southwest Watershed Research Center, USDA-ARS, Tucson, AZ, United States
- 3Sustainable Agricultural Water Systems Research Unit, USDA-ARS, Davis, CA, United States
Changing rainfall patterns will alter soil water availability to plants and microbes and likely impact soil CO2 efflux (Fs) in semiarid ecosystems. However, our understanding of the response of Fs to compound changes in rainfall event size and frequency remains relatively limited. To address this knowledge gap, we examined how compound changes in rainfall size and frequency impact Fs in a semiarid grassland by deploying automated soil chambers at a rainfall manipulation experiment. All plots within the experiment received equal total summer growing season precipitation that was temporally repackaged into regular events of inversely varied size and frequency, with event sizes ranging from 5 to 50 mm and dry intervals ranging from 3.5 to 21 days. We found that repackaging rainfall into few/large events with long dry intervals decreased seasonal cumulative Fs. Repackaging influenced key aspects of pulses including mean, maximum, and antecedent (day before irrigation) values of soil moisture and Fs and their rate of decline during drying intervals. Soil moisture explained substantial variation in Fs (R2 > 0.84) for all treatments; however, the sensitivity of Fs to soil moisture decreased in the few/large regime compared to the reference and many/small regimes. Dynamics in plant phenology (quantified by plot greenness) and soil temperature interacted with soil moisture to influence the seasonal evolution of Fs pulses and cumulative efflux. Our findings demonstrate that soil moisture and vegetation responses to changes in rainfall size and frequency impact soil CO2 efflux pulses and seasonal emissions in semiarid grasslands. These results, coupled with the knowledge that CO2 efflux pulses play an outsized role in dryland carbon exchange, indicate the possibility of future climate-mediated shifts in the carbon cycling of semiarid ecosystems.
Introduction
Intensification of precipitation driven by climate warming is changing the intensity, frequency, and length of time between storms (McCabe et al., 2010; Polade et al., 2014; Guerreiro et al., 2018; Fowler et al., 2021). In the southwest United States, widespread warming has been accompanied by increases in precipitation variability and the frequency of prolonged drought (Demaria et al., 2019; Zhang et al., 2021), and general circulation models predict further intensification of precipitation, with a shift toward larger, fewer events with longer dry intervals (Cook et al., 2020; Moustakis et al., 2021). Due to tight carbon-water coupling in globally-expansive arid and semiarid ecosystems (Noy-Meir, 1973; Huxman et al., 2004; Schwinning and Sala, 2004), precipitation changes that alter the amount and timing of soil moisture availability have implications for the terrestrial carbon sink (Poulter et al., 2014; Ahlström et al., 2015; Biederman et al., 2016).
Soil moisture regulates the metabolic activity of plants and soil organisms in arid and semiarid ecosystems (Jenerette et al., 2008) and therefore exerts strong control over soil CO2 efflux (
Although prior work has examined
Rainfall manipulation experiments are a useful tool to examine how interactive aspects of precipitation impact ecosystem processes (Knapp et al., 2015). Whereas many studies have investigated plant responses to rainfall manipulation (e.g., Heisler-White et al., 2008; Gherardi and Sala, 2015), comparatively few have examined the response of soil processes to simultaneous changes in event size and frequency (Griffin-Nolan et al., 2021; Rousk and Brangarí 2022). Although prior studies have provided useful information on ecosystem-scale respiration responses to repackaging (Liu Z et al., 2017), automated chamber systems can better capture transient
Materials and methods
Site description
We conducted this experiment at the Rainfall Manipulation in the Santa Rita Experimental Range (RainManSR) site in southeast Arizona, United States (31.79° N, 110.90° W; elevation: 1,075 m). While the experiment was conducted in plots under rainout-exclusion shelters, the surrounding ecosystem is a semiarid grassland that has experienced significant increases in mesquite (Prosopis velutina) shrub cover in the 20th century. The ecosystem is composed mainly of perennial bunchgrasses, short trees/shrubs, and bare soil which can support annual grasses and forbs given adequate rainfall. Mean annual temperature is 18.6°C and mean annual precipitation (1922–2021) is 377 mm, roughly 50% of which occurs during the summer (July–September) monsoon season (https://cals.arizona.edu/SRER/data.html). Soils are well-drained sandy loams (78% sand, 8% clay, 14% silt). Plots within the experiment represent a mixture of C4 perennial bunchgrasses, C4 annual grasses, and C3 forbs. Because the ambient ecosystem has inherently heterogeneous cover, native perennial bunchgrass (Digitaria californica) seedlings were transplanted in November 2019 into all plots at a density of 20 plants m−2. This was done to ensure a relatively consistent plant community at the start of the experiment and to better capture vegetation responses to rainfall manipulation and potential impacts on the plant-mediated component of Fs.
Experiment design and precipitation manipulation
To block all ambient precipitation, large rainout-exclusion shelters were covered with transparent film with each shelter covering 12 plots (1.2 by 1.5 m). To hydrologically isolate the plots, the perimeter of each plot was trenched to a depth of 80 cm and wrapped in polyethylene film. Plots were then lined with steel flashing which extended from a depth of 50 cm to 10 cm above the soil surface. We used a completely randomized design with two replicates of each irrigation treatment in each shelter (n = 4 plots per treatment). All plots received 205 mm of total summer irrigation (the long-term mean seasonal precipitation amount) and were hand-irrigated using a digital flow meter. Irrigation was applied to the area within soil collars when the automated chambers were in the open position. The experiment began on July 14 when 38 mm was applied to all plots. Thereafter, irrigation treatments were imposed with the following combinations of mean event size and dry intervals: many/small (9 mm; 3.5 days); reference (34 mm; 7 days, which is the climatic normal precipitation frequency at this site), and few/large (51 mm; 21 days). For more details about the experimental design of RainManSR, Zhang et al. (2022).
Soil CO2 efflux measurements
We measured the net efflux of carbon dioxide (CO2) at the soil-atmosphere interface (
Environmental variables
We measured half-hourly volumetric water content (soil moisture,
Data analysis
To quantify the effects of rainfall repackaging on
where
Results
Dynamics in soil CO2 efflux and drivers under rainfall repackaging
The effects of rainfall repackaging on

TABLE 1. Linear mixed-effects model results for daily mean soil CO2 efflux (Fs), soil moisture (θ), soil temperature (Ts), and green chromatic coordinate (GCC) normalized anomalies.
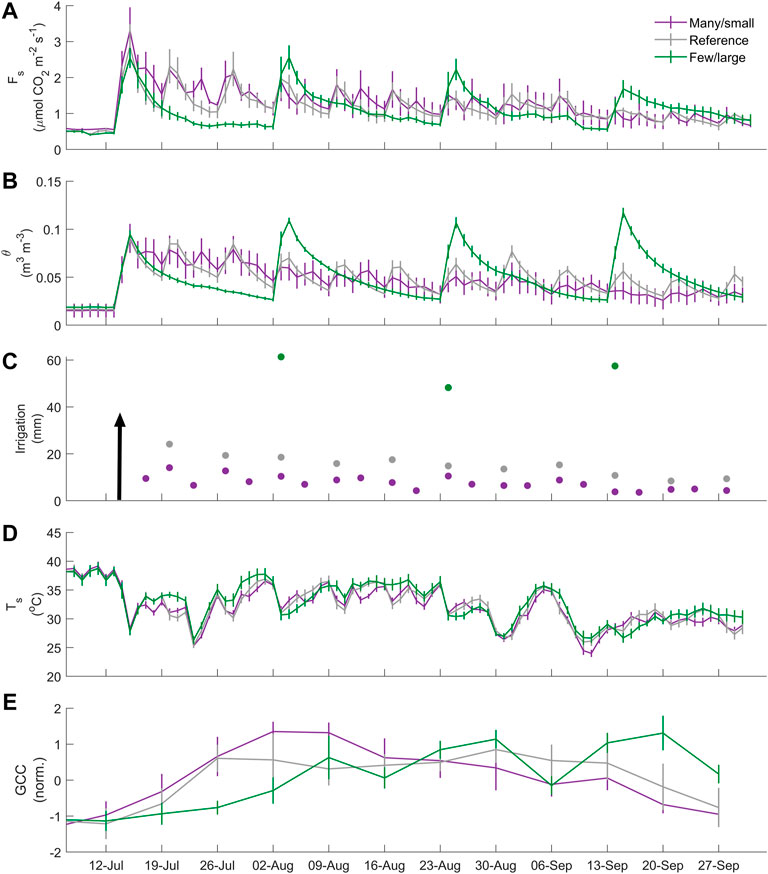
FIGURE 1. Growing season dynamics in (A) daily mean soil CO2 efflux (
Temporal rainfall repackaging alters cumulative soil CO2 efflux
Differences in pulse dynamics compounded to influence seasonal cumulative
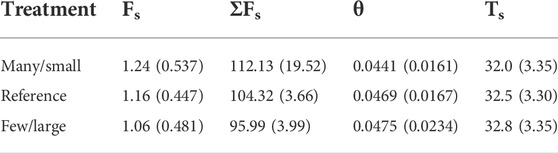
TABLE 2. Mean and standard deviation (parentheses) of seasonal average daily mean soil CO2 efflux (Fs), soil moisture (θ), soil temperature (Ts), and seasonal total Fs (ΣFs) for the three levels of rainfall repackaging.
Coherent pulse responses of soil CO2 efflux and soil moisture
We next focus on a complete wetting-drying cycle to examine how tradeoffs in event size and frequency modulated pulse responses of
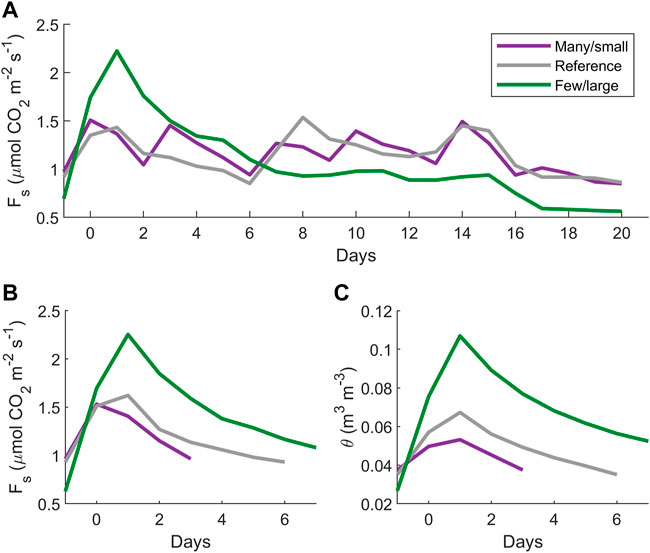
FIGURE 3. (A) Representative mid-growing season period showing soil CO2 efflux (
Environmental and vegetative controls on soil CO2 efflux
To explain the strong coherence among pulse patterns of θ and
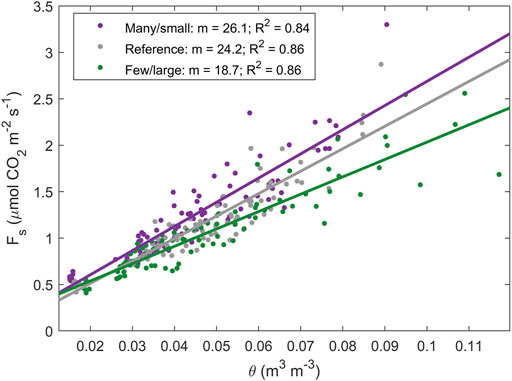
FIGURE 4. Response of daily mean soil CO2 efflux (
We next examined the relationship between
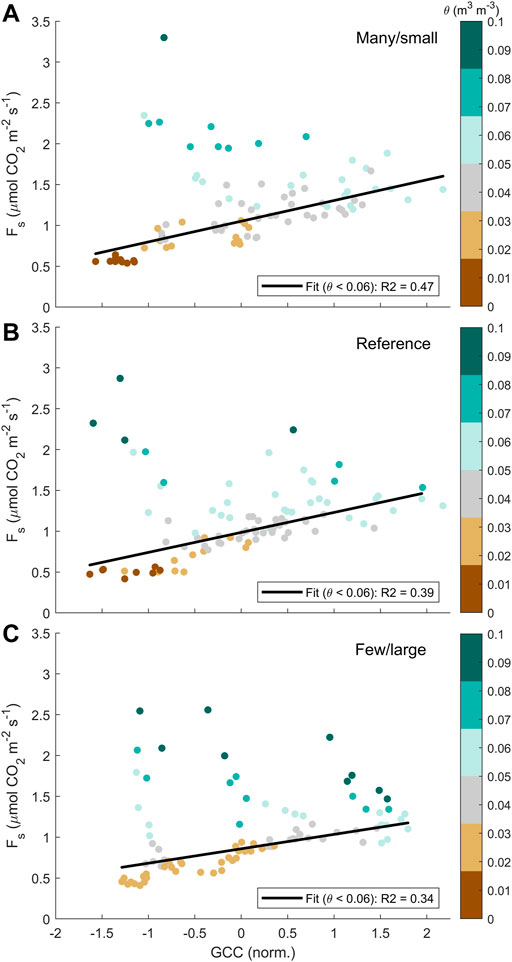
FIGURE 5. Response of daily mean soil CO2 efflux (
Seasonal evolution of soil CO2 efflux pulses
We next examined the seasonal evolution of
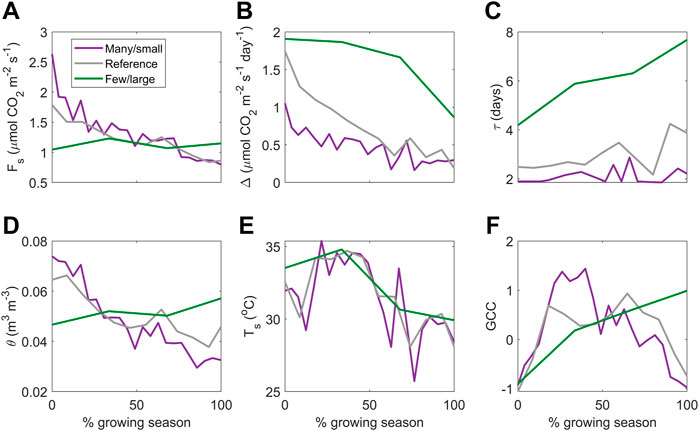
FIGURE 6. Seasonal evolution of the (A) average daily rate, (B) magnitude of pulse increase (
Discussion
Repackaging equal total rainfall into fewer, larger events decreased summer growing season cumulative
Plot greenness (GCC) and soil temperature (
Although
Together, these results indicate that changes in environmental and vegetative drivers influenced the seasonal pattern of cumulative soil CO2 emissions. The observed difference in cumulative
Dryland ecosystems have high spatial heterogeneity in soil properties and vegetation distribution, which presents challenges for understanding ecosystem responses to changes in climate, including rainfall size and frequency (Osborne et al., 2022). Because we observed large variability in seasonal total
Climate models project continued changes in rainfall toward extreme events separated by longer dry intervals (Ficklin et al., 2022). Our results show that such changes in rainfall patterns may result in reductions in
In conclusion, our findings suggest that projected changes in rainfall size and timing will likely have important effects on carbon losses from semiarid grasslands. Here we show that holding total amount constant, repackaging rainfall into fewer, larger events imposed more water stress that decreased cumulative seasonal soil CO2 efflux in a semiarid grassland. We also found evidence that temporal rainfall repackaging affects the seasonality of
Data availability statement
The raw data supporting the conclusion of this article will be made available by the authors, without undue reservation.
Author contributions
MR analyzed the data and wrote the manuscript with input from all coauthors. JB and WS designed the rainfall manipulation experiment and identified the repackaging treatments. MR, RS, JB, WS, and DM designed the soil chamber experiment. MR installed and maintained soil chamber systems with assistance from JB and WS.
Funding
Partial funding for this research came from USDA-ARS National Program 211.
Acknowledgments
We thank N. Pierce for managing the field experiment and C. Devine for installing the camera system used to quantify plot greenness. Thank you R. Bryant (USDA-ARS) for helping deploy and repair the soil chamber systems. USDA-ARS is an equal opportunity employer. We thank the two reviewers for insightful comments that improved the clarity of this manuscript.
Conflict of interest
The authors declare that the research was conducted in the absence of any commercial or financial relationships that could be construed as a potential conflict of interest.
Publisher’s note
All claims expressed in this article are solely those of the authors and do not necessarily represent those of their affiliated organizations, or those of the publisher, the editors and the reviewers. Any product that may be evaluated in this article, or claim that may be made by its manufacturer, is not guaranteed or endorsed by the publisher.
Supplementary material
The Supplementary Material for this article can be found online at: https://www.frontiersin.org/articles/10.3389/fenvs.2022.940943/full#supplementary-material
References
Ahlström, A., Raupach, M. R., Schurgers, G., and Smith, B. (2015). The dominant role of semi-arid ecosystems in the trend and variability of the land CO2 sink. Science 348, 895–899. doi:10.1126/science.aaa166Available at: https://science.sciencemag.org/content/348/6237/895.abstract.
Austin, A. T., Yahdjian, L., Stark, J. M., Belnap, J., Porporato, A., Norton, U., et al. (2004). Water pulses and biogeochemical cycles in arid and semiarid ecosystems. Oecologia 141 (2), 221–235. doi:10.1007/s00442-004-1519-1
Barron-Gafford, G. A., Scott, R. L., Jenerette, G. D., and Huxman, T. E. (2011). The relative controls of temperature, soil moisture, and plant functional group on soil CO2 efflux at diel, seasonal, and annual scales. J. Geophys. Res. 116 (G1), G01023. doi:10.1029/2010jg001442
Bates, D., Mächler, M., Bolker, B., and Walker, S., 2014. Fitting linear mixed-effects models using lme4. arXiv preprint arXiv:1406.5823. Available at:.
Biederman, J. A., Scott, R. L., Goulden, M. L., Vargas, R., Litvak, M. E., Kolb, T. E., et al. (2016). Terrestrial carbon balance in a drier world: The effects of water availability in southwestern north America. Glob. Chang. Biol. 22 (5), 1867–1879. doi:10.1111/gcb.13222
Birch, H. F. (1958). The effect of soil drying on humus decomposition and nitrogen availability. Plant Soil 10 (1), 9–31. doi:10.1007/bf01343734
Cable, J. M., Ogle, K., Barron-Gafford, G. A., Bentley, L. P., Cable, W. L., Scott, R. L., et al. (2013). Antecedent conditions influence soil respiration differences in shrub and grass patches. Ecosystems 16 (7), 1230–1247. doi:10.1007/s10021-013-9679-7
Cable, J. M., Ogle, K., Williams, D. G., Weltzin, J. F., and Huxman, T. E. (2008). Soil texture drives responses of soil respiration to precipitation pulses in the sonoran desert: Implications for climate change. Ecosystems 11 (6), 961–979. doi:10.1007/s10021-008-9172-x
Carbone, M. S., Still, C. J., Ambrose, A. R., Dawson, T. E., Williams, A. P., Boot, C. M., et al. (2011). Seasonal and episodic moisture controls on plant and microbial contributions to soil respiration. Oecologia 167 (1), 265–278. doi:10.1007/s00442-011-1975-3
Chatterjee, A., and Jenerette, G. D. (2011). Changes in soil respiration Q10 during drying–rewetting along a semi-arid elevation gradient. Geoderma 163 (3-4), 171–177. doi:10.1016/j.geoderma.2011.04.003
Choi, R. T., Reed, S. C., and Tucker, C. L. (2022). Multiple resource limitation of dryland soil microbial carbon cycling on the Colorado Plateau. Ecology 103, e3671. doi:10.1002/ecy.3671
Conant, R. T., Dalla-Betta, P., Klopatek, C. C., and Klopatek, J. M. (2004). Controls on soil respiration in semiarid soils. Soil Biol. Biochem. 36 (6), 945–951. doi:10.1016/j.soilbio.2004.02.013
Cook, B. I., Mankin, J. S., Marvel, K., Williams, A. P., Smerdon, J. E., and Anchukaitis, K. J. (2020). Twenty‐first century drought projections in the CMIP6 forcing scenarios. Earth's. Future 8 (6). doi:10.1029/2019ef001461
Davidson, E. A., Belk, E., and Boone, R. D. (1998). Soil water content and temperature as independent or confounded factors controlling soil respiration in a temperate mixed hardwood forest. Glob. Change Biol. 4, 217–227. doi:10.1046/j.1365-2486.1998.00128.x
Demaria, E. M. C., Hazenberg, P., Scott, R. L., Meles, M. B., Nichols, M., and Goodrich, D. (2019). Intensification of the north American monsoon rainfall as observed from a long‐term high‐density gauge network. Geophys. Res. Lett. 46 (12), 6839–6847. doi:10.1029/2019gl082461
Feldman, A. F., Short Gianotti, D. J., Konings, A. G., McColl, K. A., Akbar, R., Salvucci, G. D., et al. (2018). Moisture pulse-reserve in the soil-plant continuum observed across biomes. Nat. Plants 4 (12), 1026–1033. doi:10.1038/s41477-018-0304-9
Ficklin, D. L., Null, S. E., Abatzoglou, J. T., Novick, K. A., and Myers, D. T. (2022). Hydrological intensification will increase the complexity of water resource management. Earth's. Future 10 (3), e2021EF002487. doi:10.1029/2021ef002487
Fierer, N., and Schimel, J. P. (2003). A proposed mechanism for the pulse in carbon dioxide production commonly observed following the rapid rewetting of a dry soil. Soil Sci. Soc. Am. J. 67 (3), 798–805. doi:10.2136/sssaj2003.0798
Filippa, G., Cremonese, E., Migliavacca, M., Galvagno, M., Forkel, M., Wingate, L., et al. (2016). Phenopix: A R package for image-based vegetation phenology. Agric. For. Meteorology 220, 141–150. doi:10.1016/j.agrformet.2016.01.006
Fowler, H. J., Lenderink, G., Prein, A. F., Westra, S., Allan, R. P., Ban, N., et al. (2021). Anthropogenic intensification of short-duration rainfall extremes. Nat. Rev. Earth Environ. 2 (2), 107–122. doi:10.1038/s43017-020-00128-6
Franzluebbers, A. J., Haney, R. L., Honeycutt, C. W., Schomberg, H. H., and Hons, F. M. (2000). Flush of carbon dioxide following rewetting of dried soil relates to active organic pools. Soil Sci. Soc. Am. J. 64 (2), 613–623. doi:10.2136/sssaj2000.642613x
Garcia-Pichel, F., and Belnap, J. (1996). Microenvironments and microscale productivity of cyanobacterial desert crusts1. J. Phycol. 32 (5), 774–782. doi:10.1111/j.0022-3646.1996.00774.x
Gherardi, L. A., and Sala, O. E. (2015). Enhanced precipitation variability decreases grass- and increases shrub-productivity. Proc. Natl. Acad. Sci. U. S. A. 112 (41), 12735–12740. doi:10.1073/pnas.1506433112
Griffin-Nolan, R. J., Slette, I. J., and Knapp, A. K. (2021). Deconstructing precipitation variability: Rainfall event size and timing uniquely alter ecosystem dynamics. J. Ecol. 109 (9), 3356–3369. doi:10.1111/1365-2745.13724
Guerreiro, S. B., Fowler, H. J., Barbero, R., Westra, S., Lenderink, G., Blenkinsop, S., et al. (2018). Detection of continental-scale intensification of hourly rainfall extremes. Nat. Clim. Chang. 8 (9), 803–807. doi:10.1038/s41558-018-0245-3
Heisler-White, J. L., Knapp, A. K., and Kelly, E. F. (2008). Increasing precipitation event size increases aboveground net primary productivity in a semi-arid grassland. Oecologia 158 (1), 129–140. doi:10.1007/s00442-008-1116-9
Hoover, D. L., Hajek, O. L., Smith, M. D., Wilkins, K., Slette, I. J., and Knapp, A. K. (2022). Compound hydroclimatic extremes in a semi‐arid grassland: Drought, deluge, and the carbon cycle. Glob. Change Biol. 28 (8), 2611–2621. doi:10.1111/gcb.16081
Huxman, T. E., Snyder, K. A., Tissue, D., Leffler, A. J., Ogle, K., Pockman, W. T., et al. (2004). Precipitation pulses and carbon fluxes in semiarid and arid ecosystems. Oecologia 141 (2), 254–268. doi:10.1007/s00442-004-1682-4
Jenerette, G. D., Scott, R. L., and Huxman, T. E. (2008). Whole ecosystem metabolic pulses following precipitation events. Funct. Ecol. 22 (5), 924–930. doi:10.1111/j.1365-2435.2008.01450.x
Knapp, A. K., Beier, C., Briske, D. D., Classen, A. T., Luo, Y., Reichstein, M., et al. (2008). Consequences of more extreme precipitation regimes for terrestrial ecosystems. Bioscience 58 (9), 811–821. doi:10.1641/b580908
Knapp, A. K., Hoover, D. L., Wilcox, K. R., Avolio, M. L., Koerner, S. E., La Pierre, K. J., et al. (2015). Characterizing differences in precipitation regimes of extreme wet and dry years: Implications for climate change experiments. Glob. Chang. Biol. 21 (7), 2624–2633. doi:10.1111/gcb.12888
Kurc, S. A., and Small, E. E. (2004). Dynamics of evapotranspiration in semiarid grassland and shrubland ecosystems during the summer monsoon season, central New Mexico. Water Resour. Res. 40 (9). doi:10.1029/2004wr003068
Kuzyakov, Y., and Gavrichkova, O. (2010). Review: Time lag between photosynthesis and carbon dioxide efflux from soil: A review of mechanisms and controls: Time lag between photosynthesis and CO2 efflux from soil. Glob. Change Biol. 16 (12), 3386–3406. doi:10.1111/j.1365-2486.2010.02179.x
Leon, E., Vargas, R., Bullock, S., Lopez, E., Panosso, A. R., and La Scala, N. (2014). Hot spots, hot moments, and spatio-temporal controls on soil CO2 efflux in a water-limited ecosystem. Soil Biol. Biochem. 77, 12–21. doi:10.1016/j.soilbio.2014.05.029
Liu W, W. J., Li, L. F., Biederman, J. A., Hao, Y. B., Zhang, H., Kang, X. M., et al. (2017). Repackaging precipitation into fewer, larger storms reduces ecosystem exchanges of CO2 and H2O in a semiarid steppe. Agric. For. Meteorology 247, 356–364. doi:10.1016/j.agrformet.2017.08.029
Liu, W., Zhang, Z., and Wan, S. (2009). Predominant role of water in regulating soil and microbial respiration and their responses to climate change in a semiarid grassland. Glob. Change Biol. 15 (1), 184–195. doi:10.1111/j.1365-2486.2008.01728.x
Liu Z, Z., Zhang, Y., Fa, K., Qin, S., and She, W. (2017). Rainfall pulses modify soil carbon emission in a semiarid desert. Catena 155, 147–155. doi:10.1016/j.catena.2017.03.011
López-Ballesteros, A., Serrano-Ortiz, P., Sánchez-Cañete, E. P., Oyonarte, C., Kowalski, A. S., Pérez-Priego, Ó., et al. (2016). Enhancement of the net CO2release of a semiarid grassland in SE Spain by rain pulses: Rain Pulses Enhance Net CO2Release. J. Geophys. Res. Biogeosci. 121 (1), 52–66. doi:10.1002/2015jg003091
Luo, Y., and Zhou, X. (2006). “Chapter 5 - controlling factors,” in Soil respiration and the environment. Editors Y. Luo, and X. Zhou (Burlington: Academic Press), 79–105.
McCabe, G. J., Legates, D. R., and Lins, H. F. (2010). Variability and trends in dry day frequency and dry event length in the southwestern United States. J. Geophys. Res. 115 (D7), D07108. doi:10.1029/2009jd012866
Moustakis, Y., Papalexiou, S. M., Onof, C. J., and Paschalis, A. (2021). Seasonality, intensity, and duration of rainfall extremes change in a warmer climate. Earth's. Future 9 (3), ef001824. doi:10.1029/2020ef001824
Moyano, F. E., Manzoni, S., and Chenu, C. (2013). Responses of soil heterotrophic respiration to moisture availability: An exploration of processes and models. Soil Biol. Biochem. 59, 72–85. doi:10.1016/j.soilbio.2013.01.002
Niu, F., Chen, J., Xiong, P., Wang, Z., Zhang, H., and Xu, B. (2019). Responses of soil respiration to rainfall pulses in a natural grassland community on the semi-arid Loess Plateau of China. Catena 178, 199–208. doi:10.1016/j.catena.2019.03.020
Noy-Meir, I. (1973). Desert ecosystems: Environment and producers. Annu. Rev. Ecol. Syst. 4 (1), 25–51. doi:10.1146/annurev.es.04.110173.000325
Ogle, K., and Reynolds, J. F. (2004). Plant responses to precipitation in desert ecosystems: Integrating functional types, pulses, thresholds, and delays. Oecologia 141 (2), 282–294. doi:10.1007/s00442-004-1507-5
Orchard, V. A., and Cook, F. J. (1983). Relationship between soil respiration and soil moisture. Soil Biol. Biochem. 15 (4), 447–453. doi:10.1016/0038-0717(83)90010-x
Osborne, B. B., Bestelmeyer, B. T., Currier, C. M., Homyak, P. M., Throop, H. L., Young, K., et al. (2022). The consequences of climate change for dryland biogeochemistry. New Phytol. doi:10.1111/nph.18312
Polade, S. D., Pierce, D. W., Cayan, D. R., Gershunov, A., and Dettinger, M. D. (2014). The key role of dry days in changing regional climate and precipitation regimes. Sci. Rep. 4, 4364. doi:10.1038/srep04364
Porporato, A., D’odorico, P., Laio, F., Ridolfi, L., and Rodriguez-Iturbe, I. (2002). Ecohydrology of water-controlled ecosystems. Adv. Water Resour. 25 (8-12), 1335–1348. doi:10.1016/s0309-1708(02)00058-1
Post, A. K., and Knapp, A. K. (2021). How big is big enough? Surprising responses of a semiarid grassland to increasing deluge size. Glob. Chang. Biol. 27 (6), 1157–1169. doi:10.1111/gcb.15479
Poulter, B., Frank, D., Ciais, P., Myneni, R. B., Andela, N., Bi, J., et al. (2014). Contribution of semi-arid ecosystems to interannual variability of the global carbon cycle. Nature 509 (7502), 600–603. doi:10.1038/nature13376
Roby, M. C., Scott, R. L., Barron-Gafford, G. A., Hamerlynck, E. P., and Moore, D. J. P. (2019). Environmental and vegetative controls on soil CO2 efflux in three semiarid ecosystems. Soil Syst. 3 (1), 6. doi:10.3390/soilsystems3010006
Roby, M. C., Scott, R. L., and Moore, D. J. P. (2020). High vapor pressure deficit decreases the productivity and water use efficiency of rain-induced pulses in semiarid ecosystems. J. Geophys. Res. Biogeosci. 125 (10), jg005665. doi:10.1029/2020jg005665
Rousk, J., and Brangarí, A. C. (2022). Do the respiration pulses induced by drying–rewetting matter for the soil–atmosphere carbon balance? Glob. Change Biol. 28, 3486–3488. doi:10.1111/gcb.16163
Savage, K., Davidson, E. A., Richardson, A. D., and Hollinger, D. Y. (2009). Three scales of temporal resolution from automated soil respiration measurements. Agric. For. Meteorology 149 (11), 2012–2021. doi:10.1016/j.agrformet.2009.07.008
Schwinning, S., and Sala, O. E. (2004). Hierarchy of responses to resource pulses in arid and semi-arid ecosystems. Oecologia 141 (2), 211–220. doi:10.1007/s00442-004-1520-8
Sloat, L. L., Gerber, J. S., Samberg, L. H., Smith, W. K., Herrero, M., Ferreira, L. G., et al. (2018). Increasing importance of precipitation variability on global livestock grazing lands. Nat. Clim. Chang. 8, 214–218. doi:10.1038/s41558-018-0081-5
Sponseller, R. A. (2007). Precipitation pulses and soil CO2flux in a Sonoran Desert ecosystem. Glob. Chang. Biol. 13 (2), 426–436. doi:10.1111/j.1365-2486.2006.01307.x
Thomey, M. L., Collins, S. L., Vargas, R., Johnson, J. E., Brown, R. F., Natvig, D. O., et al. (2011). Effect of precipitation variability on net primary production and soil respiration in a chihuahuan desert grassland: Precipitation variability in desert grassland. Glob. Change Biol. 17 (4), 1505–1515. doi:10.1111/j.1365-2486.2010.02363.x
Unger, S., Máguas, C., Pereira, J. S., David, T. S., and Werner, C. (2010). The influence of precipitation pulses on soil respiration – assessing the “Birch effect” by stable carbon isotopes. Soil Biol. Biochem. 42 (10), 1800–1810. doi:10.1016/j.soilbio.2010.06.019
Vargas, R., Collins, S. L., Thomey, M. L., Johnson, J. E., Brown, R. F., Natvig, D. O., et al. (2012). Precipitation variability and fire influence the temporal dynamics of soil CO2 efflux in an arid grassland. Glob. Chang. Biol. 18 (4), 1401–1411. doi:10.1111/j.1365-2486.2011.02628.x
Vargas, R., Sánchez-Cañete, P., E., Serrano-Ortiz, P., Curiel Yuste, J., Domingo, F., López-Ballesteros, A., et al. (2018). Hot-Moments of soil CO2 efflux in a water-limited grassland. Soil Syst. 2 (3), 47. doi:10.3390/soilsystems2030047
Wang, B., Zha, T. S., Jia, X., Wu, B., Zhang, Y. Q., and Qin, S. G. (2014). Soil moisture modifies the response of soil respiration to temperature in a desert shrub ecosystem. Biogeosciences 11 (2), 259–268. doi:10.5194/bg-11-259-2014
Wang, Y., Li, X., Zhang, C., Wu, X., Du, E., Wu, H., et al. (2019). Responses of soil respiration to rainfall addition in a desert ecosystem: Linking physiological activities and rainfall pattern. Sci. Total Environ., 650(Pt 2), 3007–3016. doi:10.1016/j.scitotenv.2018.10.057
Xu, L., Baldocchi, D. D., and Tang, J. (2004). How soil moisture, rain pulses, and growth alter the response of ecosystem respiration to temperature: RAIN, growth, and respiration. Glob. Biogeochem. Cycles 18 (4), gb002281. doi:10.1029/2004gb002281
Yan, D., Scott, R. L., Moore, D. J. P., Biederman, J. A., and Smith, W. K. (2019). Understanding the relationship between vegetation greenness and productivity across dryland ecosystems through the integration of PhenoCam, satellite, and eddy covariance data. Remote Sens. Environ. 223, 50–62. doi:10.1016/j.rse.2018.12.029
Yan, L., Chen, S., Huang, J., and Lin, G. (2011). Water regulated effects of photosynthetic substrate supply on soil respiration in a semiarid steppe. Glob. Change Biol. 17 (5), 1990–2001. doi:10.1111/j.1365-2486.2010.02365.x
Yan, L., Chen, S., Xia, J., and Luo, Y. (2014). Precipitation regime shift enhanced the rain pulse effect on soil respiration in a semi-arid steppe. PloS One 9 (8), e104217. doi:10.1371/journal.pone.0104217
Yan, Z., Bond-Lamberty, B., Todd-Brown, K. E., Bailey, V. L., Li, S., Liu, C., et al. (2018). A moisture function of soil heterotrophic respiration that incorporates microscale processes. Nat. Commun. 9 (1), 2562–2610. doi:10.1038/s41467-018-04971-6
Zhang, F., Biederman, J. A., Pierce, N., Potts, D., Devine, C., Yanbin, H., et al. (2022). Precipitation temporal repackaging into fewer, larger storms delayed seasonal timing of peak photosynthesis in a semi-arid grassland. Funct. Ecol. 36, 646–658. doi:10.1111/1365-2435.13980
Zhang, F., Biederman, J. A., Dannenberg, M. P., Yan, D., Reed, S. C., and Smith, W. K. (2021). Five decades of observed daily precipitation reveal longer and more variable drought events across much of the western United States. Geophys. Res. Lett. 48 (7), e2020GL092293. doi:10.1029/2020gl092293
Zhang, X., Niu, G. Y., Elshall, A. S., Ye, M., Barron‐Gafford, G. A., and Pavao-Zuckerman, M. (2014). Assessing five evolving microbial enzyme models against field measurements from a semiarid savannah—what are the mechanisms of soil respiration pulses? Geophys. Res. Lett. 41 (18), 6428–6434. doi:10.1002/2014gl061399
Keywords: soil respiration, soil efflux, rainfall intensification, soil moisture, pulse, semiarid
Citation: Roby MC, Scott RL, Biederman JA, Smith WK and Moore DJP (2022) Response of soil carbon dioxide efflux to temporal repackaging of rainfall into fewer, larger events in a semiarid grassland. Front. Environ. Sci. 10:940943. doi: 10.3389/fenvs.2022.940943
Received: 10 May 2022; Accepted: 31 August 2022;
Published: 21 September 2022.
Edited by:
Anping Chen, Colorado State University, United StatesReviewed by:
Alison Post, Northern Arizona University, United StatesQiang Yu, Chinese Academy of Agricultural Sciences (CAAS), China
Copyright © 2022 Roby, Scott, Biederman, Smith and Moore. This is an open-access article distributed under the terms of the Creative Commons Attribution License (CC BY). The use, distribution or reproduction in other forums is permitted, provided the original author(s) and the copyright owner(s) are credited and that the original publication in this journal is cited, in accordance with accepted academic practice. No use, distribution or reproduction is permitted which does not comply with these terms.
*Correspondence: Matthew C. Roby, matthew.roby@usda.gov