- 1Xinjiang Key Laboratory of Desert Plant Roots Ecology and Vegetation Restoration, Xinjiang Institute of Ecology and Geography, Chinese Academy of Sciences, Urumqi, China
- 2National Engineering Technology Research Center for Desert-Oasis Ecological Construction, Xinjiang Institute of Ecology and Geography, Chinese Academy of Sciences, Urumqi, China
- 3Cele National Station of Observation and Research for Desert-Grassland Ecosystems, Xinjiang, China
- 4State Key Laboratory of Desert and Oasis Ecology, Xinjiang Institute of Ecology and Geography, Chinese Academy of Sciences, Urumqi, China
- 5Department of Soil Science, University of Manitoba, Winnipeg, MB, Canada
- 6Department of Botany, University of Narowal, Narowal, Pakistan
- 7School of Grassland Science, Beijing Forestry University, Beijing, China
- 8Hunan Institute of Agro-Environment and Ecology, Hunan Academy of Agricultural Science, Changsha, China
Soil nutrients are vital for plant growth and survival and present a crucial role in terrestrial function and productivity. However, little is known about the effect mechanism of groundwater table on soil nutrients in an arid desert ecological system. This study investigated the impacts of groundwater depth on the concentrations of soil organic carbon (C), available nitrogen (N), phosphorus (P), and potassium (K) at shallow groundwater depths (0.4, 0.8, 1.2, 1.8, and 2.2 m) and field deep groundwater depths (2.5, 4.5, and 11.0 m) in a desert-oasis ecotone in Central Asia in 2015 and 2016. Soil nitrate-N, inorganic-N, soil available P, and K concentrations were significantly affected by shallow and field deep groundwater. Groundwater depths did not alter soil ammonium-N concentration. Soil organic C concentration was influenced by field deep groundwater depth. Structural equation model showed that groundwater depth directly affected soil nitrate-N and K concentrations and indirectly altered the soil inorganic-N, soil organic C and available P concentrations in shallow groundwater. Moreover, groundwater depth directly influenced soil nitrate-N and soil organic C, available P and K concentrations and indirectly affected soil inorganic-N concentration in deep groundwater. Hence, groundwater depth should be considered one of the most critical environmental factors affecting soil nutrient variation in an arid desert. This study provides new insights into the soil nutrient variation under a declining groundwater depth in a hyper-arid ecosystem.
Introduction
Soil nutrients are essential for plant growth, survival, and distribution and present a crucial role in terrestrial functions and productivity. Soil organic carbon is substantial for maintaining soil fertility and represents soil quality, which impacts a wide range of soil physical, chemical, and biological properties (Cheng and An, 2015). Soil nitrogen (N), phosphorus (P), and potassium (K) are three crucial macroelements that individually or jointly affect plant productivity and play a considerable role in terrestrial functions (Hati et al., 2008; Quilchano et al., 2008; Liu et al., 2010; Li et al., 2016; Guan et al., 2017; Zhang et al., 2021a; Zhang et al., 2021b). Soil nutrient concentrations are influenced by lots of environmental factors, such as vegetation succession (Cheng and An, 2015), land utilization (Ross et al., 1999; Fang et al., 2012; Gelaw et al., 2014), N deposition (Ma et al., 2011), precipitation (Patil et al., 2010), and groundwater depth (Hefting et al., 2004; Rhymes et al., 2016).
Groundwater is the most critical limiting factor in an arid ecosystem (Zeng et al., 2006). Although Rhymes et al. (2016) reported that dune slacks are sensitive to slight variations in groundwater levels. Denitrification reduction led to an increase in soil nitrogen concentration with just a 10 cm decrease in groundwater depth. Lowered water depths will likely intensify biological effects, promote soil carbon decomposition, and potentially depress carbon sequestration in dune slack soils. Hefting et al. (2004) found that water table levels of 10 and 30 cm are the thresholds that characterize the soil microbial N cycle, leading to variations in nitrification, ammonification, and denitrification rates, which in turn alter the N dynamics. Furthermore, the Soil C, N, and P concentrations are significantly affected by groundwater depth in a desert ecosystem (Zhang et al., 2018b). Although we can find pieces of research information on the effects of the groundwater depth on soil nutrient concentrations, systematic studies on the variation of soil nutrient concentrations, especially C, N, P, and K, under reduced groundwater depth in an arid desert ecological system are rare.
In the arid region of Central Asia, the groundwater depth gradually deepened in the desert-oasis transition ecotone due to increasing human activity, indicating water resources shortage (Liu, 2007). The decline of groundwater depth remarkably affects soil water content, plant biomass accumulation, plant growth rate, plant photosynthesis, and plant water uptake, which further influences community structure and ecosystem composition (Nilsson et al., 1997; Li et al., 2010; Gui et al., 2013; Li et al., 2015). Zhang et al. (2018c) observed significant differences in soil nutrients among 2.5, 4.5 and 11.0 m groundwater depths in a desert ecosystem in an arid region. Topsoil P and K concentrations are positively altered by groundwater depths in the desert ecosystem (Zhang et al., 2021a). However, little is known about groundwater depth’s direct and indirect effect on soil nutrient concentrations in different groundwater depths. Therefore, we hypothesized the following: 1) Groundwater depth directly affects soil nutrients, such as ammonium-N, nitrate-N, and inorganic-N and organic C, available P and K concentrations, in the different environments. 2) Groundwater depth is a critical factor that affects the variation of soil nutrients in an arid desert ecological system.
Materials and methods
Study area
The study is conducted at the Cele National Station of Observation and Research for Desert and Grassland Ecosystem (Cele station; 37°00.77″N, 80°43.45″E) on the southern edge of the Taklamakan Desert in Xinjiang Uyghur Autonomous Region of China. Cele station is in the desert-oasis transition ecotone, which is occupied by dominant Alhagi sparsifolia (Zhang et al., 2021b). The vegetation coverage is 60%–80% (Zhang et al., 2018a), and the groundwater depth varies from 1.2 to 15.0 m. The study site’s elevation is 1,366 m, the mean annual temperature is 11.9°C, the mean potential annual evaporation is 2,600 mm, and the mean annual precipitation is only 35 mm (Gui et al., 2013; Liu et al., 2016; Zhang et al., 2021b). The temperatures can reach extreme levels, such as −31°C in the winter and 41.9°C in the summer. It is an arid region, with hot summers and cold winters. The soil is sandy, and the bulk density is 1.36 g/cm3 (Li et al., 2015; Liu et al., 2016). In 2015, the precipitation at Cele station was 34.2 mm, while in 2016, it was 43.4 mm.
This study conducted simulation and field experiments in 2015 and 2016, respectively. The simulation experiment investigated the effect of shallow groundwater (0.4, 0.8, 1.2, 1.8, and 2.2 m) on soil nutrient variation. The field experiment determined the effect of deep groundwater (2.5, 4.5, and 11.0 m) on soil nutrient variation. A dominant phreatophytic species, A. sparsifolia, was grown in concrete soil containers (1 m × 1 m) with different artificial groundwater tables in April 2015. The groundwater table in each treatment was maintained at a fixed level by adding water through pipes weekly. More detailed information about the experimental layout is available in our previous publication (Li et al., 2015).
In the field experiment, various depths of groundwater were selected at three sites, including those with depths of 2.5 m (N37°01′18″, E80°42′29″), 4.5 m (N37°00′40″, E80°42′13″), and 11.0 m (N37°00′33″, E80°42′25″). Each of the three sites covered approximately 2 ha. The groundwater depths at these three sites did not change significantly between 2015 and 2016. More detailed information is available from previous studies (Liu, 2011; Gui et al., 2013; Zhang et al., 2018b; Zhang et al., 2021a).
Field sampling and chemical analysis
A previous study found no significant differences between the soil organic C, total and available N, and P concentrations beneath 50 cm soil depth around the A. sparsifolia at depths of 2.5, 4.5, and 11 m on the same research site (Liu, 2011). However, there were significant differences in soil organic C, total and available N, and P concentrations in the 0–20 cm under ground at various groundwater depths (Zhang et al., 2018b). Therefore, at each sampling stage, six soil samples in the shallow groundwater depths and nine soil samples in the deep groundwater depths around the canopy of A. sparsifolia were collected monthly from 0 to 20 cm depth using standardized collection protocols from June to October in each groundwater depth level in 2015 and 2016. Soil samples were air-dried, ground, and then passed through a 1-mm sieve. Soil organic carbon was determined by the K2Cr2O7 digestion method (Nelson and Sommers, 1975). We used 2 M KCl to extract fresh soil samples (10 g). Nitrate-N was measured with a continuous flow spectrophotometer using the cadmium reduction method and ammonium-N using the salicylate method (FIAstar 5000; Foss Tecator, Denmark). Inorganic N is composed of ammonium and nitrate (Lü et al., 2014). We measured the availability of P by colorimetry after extracting with 0.5 M NaHCO3 (Olsen et al., 1954). Available K was determined by using the flame photometer.
Data analysis
We performed all analyses with R software (version 4.0.3). Multiple comparisons of treatment means were conducted using the Tukey test. Linear relationships between groundwater and soil nutrients were analyzed using the “corrplot” package. Groundwater depth’s direct and indirect effects on soil nutrients were statistically separated and quantified by a piecewise structural equation modeling (piecewise SEM) study using the R package (Lefcheck, 2016). To determine whether the causal model missed essential links, we used the D-separation test of Piecewise SEM; p > 0.05 indicated that the model was valid (Shipley, 2002).
Results
Soil inorganic-N, C, P, and K concentrations affected by groundwater depth
In the shallow groundwater, soil nitrate-N and inorganic-N concentrations increased first and then decreased with the increasing groundwater depths (Figures 1A,E; Supplementary Figure S2). The concentrations of soil nitrate-N and inorganic-N at 0.8 and 1.2 m groundwater depths significantly elevated compared with 0.4, 1.8, and 2.2 m depths. Moreover, no significant differences in ammonium-N concentrations were observed among all groundwater depths (Figure 1C). The soil organic C concentration at 1.2 m groundwater depth was significantly greater than those at 1.8 and 2.2 m (Figure 2A). Moreover, no significant difference in soil available P concentrations was found among all groundwater depths (Figure 2C). Soil available K concentration at 0.4 and 0.8 m groundwater depth significantly raised compared with 1.8 and 2.2 m depths (Figure 2E; Supplementary Figure S4).
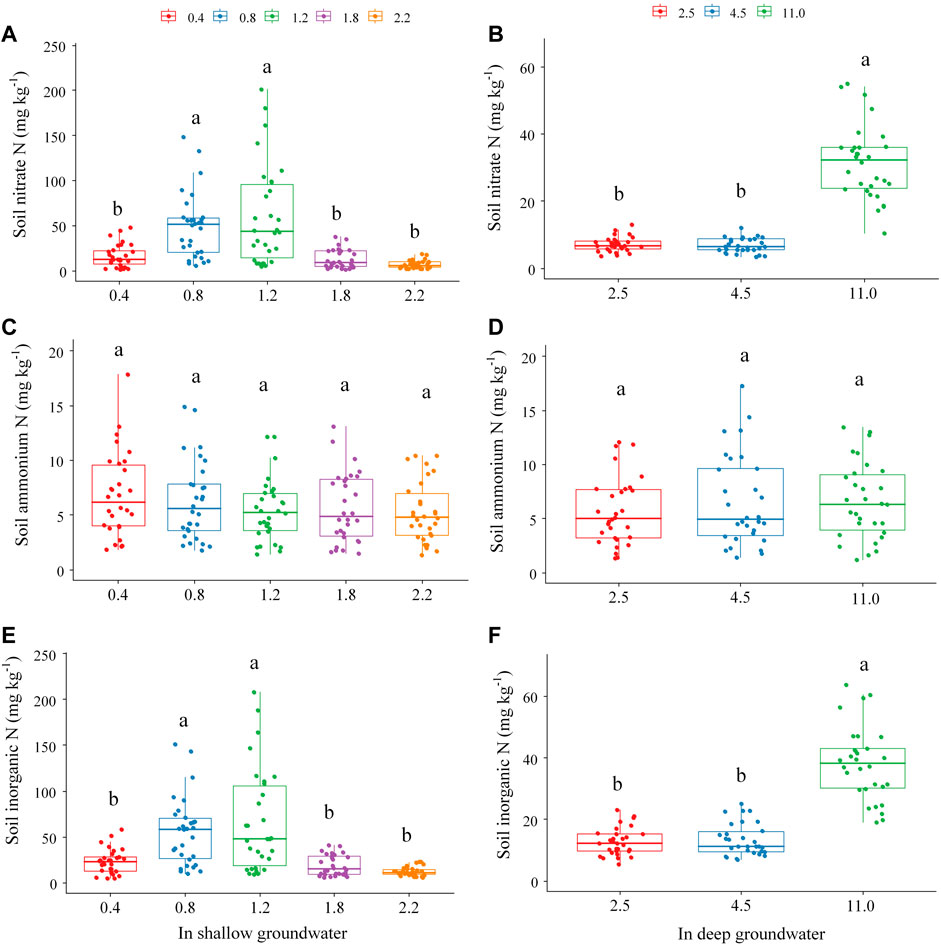
FIGURE 1. (A, C, E) represent variations of soil N concentration in shallow groundwater. (B, D, F) represent variations of soil N concentration in deep groundwater.
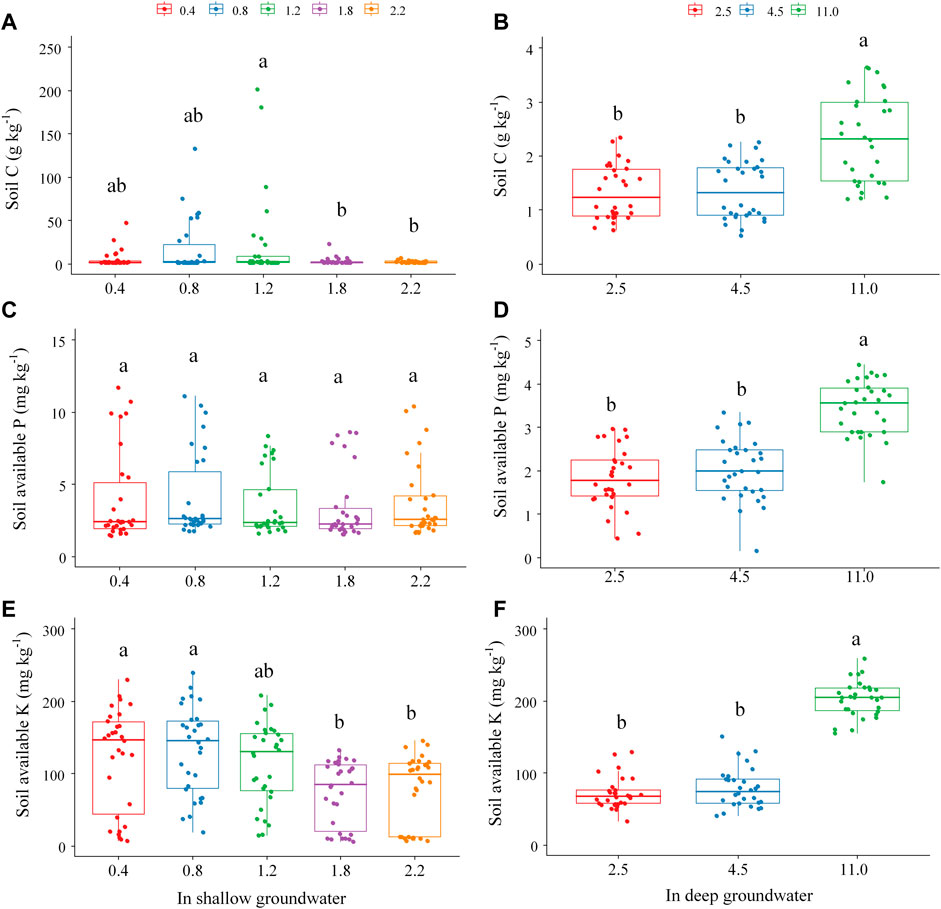
FIGURE 2. (A, C, E) represent variations of soil C, P, and K concentrations in shallow groundwater. (B, D, F) represent variations of soil C, P, and K concentrations in deep groundwater.
In the deep groundwater, soil nitrate-N and inorganic-N concentrations increased with increasing groundwater depths (Figures 1B,F; Supplementary Figure S3). The concentrations of soil nitrate-N and inorganic-N at 11.0 m groundwater depth significantly increased compared with 2.5 and 4.5 m depths. No significant differences in ammonium-N concentrations were observed among all groundwater depths during 2015 and 2016 (Figure 1D). The soil organic C, available P and K concentrations increased with increasing groundwater depths (Figures 2B,D,F; Supplementary Figure S5). Concentrations of soil organic C, available P and K at 11.0 m groundwater depth significantly enhanced compared with 2.5 and 4.5 m depths.
Relationships between groundwater and soil nutrients
In the shallow groundwater, depths were negatively related to soil nitrate-N, inorganic-N, and K concentrations (Figure 3A). Soil nitrate-N was positively associated with soil inorganic-N and soil organic C and available K concentrations. Soil ammonium-N was closely linked to soil inorganic-N, organic C, and available P concentrations. Soil inorganic-N was positively related to soil organic C and available K concentrations. Soil available P was significantly correlated with soil organic C and available K concentrations. Depth had a significant direct impact on soil nitrate-N and soil available K concentrations (Figure 4A). Soil inorganic-N was significantly affected by soil nitrate-N and soil ammonium-N. Soil organic C was significantly influenced by soil nitrate-N concentration. Soil available P was significantly determined by soil nitrate-N, ammonium-N, and Soil organic C concentrations. Soil available K was significantly altered by groundwater depth, soil ammonium-N, and available P concentrations.
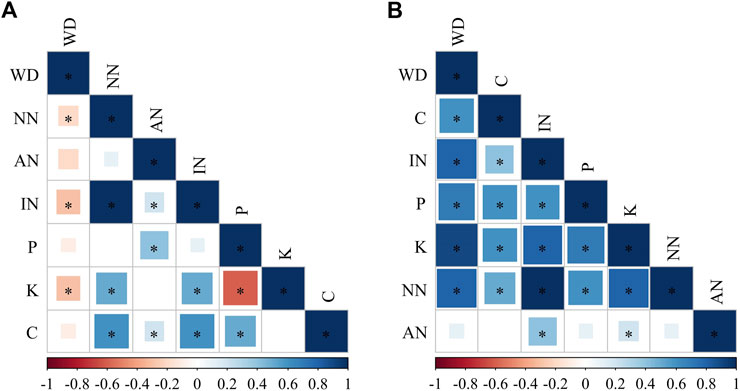
FIGURE 3. Relationships between groundwater depth and soil nutrients. Abbreviations of variables: WD, groundwater depth; C, soil organic carbon; NN, soil nitrate-N; AN, soil ammonium-N; IN, soil inorganic-N; P, soil available phosphorus; K, soil available potassium. (A) Relationships between groundwater depth and soil nutrients in the shallow groundwater; (B) Relationships between groundwater depth and soil nutrients in the deep natural groundwater. * indicates the correlation was significant at a 0.05 level.
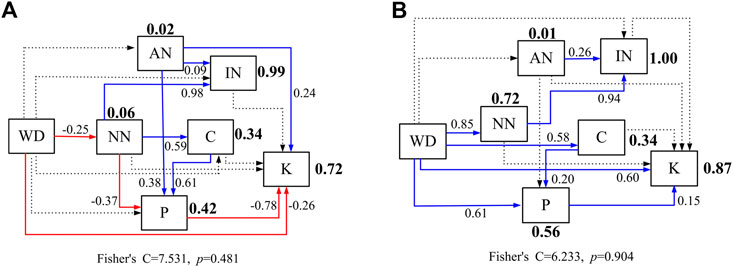
FIGURE 4. Controlling factor analysis of soil nutrients using the structural equation model. Solid lines indicate significant regressions (p < 0.05) and no significant regressions by dashed lines. Blue arrows denote positive relationships, and red arrows denote negative ones. Abbreviations of variables: WD, groundwater depth; C, soil organic carbon; NN, soil nitrate-N; AN, soil ammonium-N; IN, soil inorganic-N; P, soil available phosphorus; K, soil available potassium. (A) Relationships between groundwater depth and soil nutrients in the shallow groundwater; (B) Relationships between groundwater depth and soil nutrients in the deep natural groundwater.
In the deep groundwater, depths were positively related to soil nitrate-N and inorganic-N, organic C, and available P and K concentrations (Figure 3B). Soil organic C was positively associated with soil nitrate-N, soil inorganic-N, and soil available P and K concentrations. Soil inorganic-N was positively related to soil nitrate-N, soil ammonium-N, and soil available P and K concentrations. Soil available P was significantly correlated with soil nitrate-N and soil available K concentrations. Soil available K was positively influenced by soil nitrate-N and soil ammonium-N. A significant positive correlation was shown between soil nitrate-N and soil ammonium-N. Depth directly regulated soil nitrate-N and soil organic C, available P and K concentrations (Figure 4B). Soil inorganic-N was significantly altered by soil nitrate-N and soil ammonium-N. Soil available K was significantly determined by groundwater depth and soil available P concentration. Soil organic C was significantly affected by groundwater depth. Soil available P was significantly influenced by groundwater depth and soil organic C concentrations.
Discussion
Soil inorganic-N, C, P, and K concentrations affected by groundwater depth
Nitrification and denitrification are crucial in regulating nitrogen contents in ecosystems, whereas their rates are affected by a variety of factors, such as groundwater levels (Hefting et al., 2004; Rhymes et al., 2016), temperature (Reich and Oleksyn, 2004; Lü et al., 2014), precipitation (Zhu et al., 2017), and soil O2 levels (Burgin et al., 2010). Our results partly support the first hypothesis that groundwater affected soil nitrate-N and inorganic-N concentrations in the present study. Soil nitrate-N concentrations were the highest at 0.8 and 1.2 m groundwater depths in the simulation experiment in 2015 and 2016 (Figure 1A) may be due to soils at 0.8 and 1.2 m groundwater depths having the best moisture and aerobic conditions for nitrification (Li et al., 2015). This finding is consistent with the following statements: moderate soil moisture stimulates soil mineralization (Zhu et al., 2017); and soil water content is significantly influenced by groundwater depth at the same simulation experiment site (Li et al., 2015). In addition, Soil nitrate-N concentrations were greater at 11.0 m groundwater depths than at 2.5 and 4.5 m groundwater depths in the field experiment in 2015 and 2016 (Figure 1B). It is consistent with the report that drier slack dune soils inhibit denitrification, leading to increased nitrogen retention and greater eutrophication (Rhymes et al., 2016), and soil water content at 11.0 m groundwater depth was significantly lower than those at 2.5 and 4.5 m depths (Supplementary Figure S1).
However, soil nitrate-N concentration at 0.4 m groundwater depth was lower than that at 0.8 and 1.2 m because the soil was wetter and anaerobic conditions reduced the nitrification. This finding confirmed that net nitrification is inhibited by the scarcity of free oxygen in soil (Hefting et al., 2004). In contrast, soil nitrate-N concentrations at 1.8 and 2.2 m were lower than those at 0.8 and 1.2 m because the soils were too dry (Li et al., 2015), and moisture inhibited nitrification. This result was similar to the following finding: lower or higher soil moisture depressed soil mineralization, resulting in the variation of soil inorganic-N concentration (Zhu et al., 2017).
Conversely, no significant difference was found in ammonium-N concentrations in shallow (0.4–2.2 m) and deep (2.5–11.0 m) groundwater, which is consistent with the observation that being different to nitrate-N, ammonium-N was not influenced by soil water content (Song and Li, 2005). In addition, the variation trends of inorganic-N under different groundwater depths were similar to the changing trend of nitrate-N (Figures 1A,B,E,F). The inorganic-N concentration is the sum of nitrate and ammonium nitrogen. In this study, soil nitrate-N concentration in the simulation experiment was 10-fold greater than the ammonium-N concentrations (Figures 1A,C). Therefore, the changing trend of inorganic-N was attributed to the variation of nitrate-N. This finding is similar to the changes in soil N in the field experiment (Figures 1B,D). Hence, groundwater depth significant affected soil inorganic-N concentration, especially nitrate-N concentration, in different environments in an arid desert.
In previous studies, Gami et al. (2009) showed that soil organic C is affected by soil drainage and land management. Wang et al. (2000) reported that soil moisture substantially affects soil organic matter decomposition. Lower and higher soil moisture levels depress the organic matter decomposition rate, resulting in soil organic C concentration variation. Our study showed that groundwater depth significantly influenced the soil organic C concentration in shallow (0.4–2.2 m) and field deep (2.5–11.0 m) groundwater (Figures 2A,B). The soil organic C concentration at 1.2 m groundwater depths was greater higher than those at 1.8 and 2.2 m depths (Figure 2A). This finding is consistent with the observation that soil moisture level at 1.2 m groundwater depth may optimal for achieving the rate of organic matter decomposition (Li et al., 2015), resulting in higher soil organic C concentrations obtained at 1.2 m groundwater depth than at other groundwater levels. In addition, soil organic C concentration at 11.0 m groundwater depth was significantly greater than those at 2.5 and 4.5 m (Figure 2B). This finding is consistent with the results of a previous study, in which soil moisture was the most vital factor affecting the variation of soil organic C in the temperate grassland (Tao et al., 2013) and there was significantly lower soil water content at 11.0 m groundwater depth than at 2.5 and 4.5 m (Supplementary Figure S1). Hence, higher soil organic C retention and lower C loss were likely obtained in drier soil at 11.0 m groundwater depth.
Soil available P concentration depends on the dissolution of phosphate minerals and the release of fixed phosphorus (Vance et al., 2003). In the present study, no significant difference in soil P concentration was found among different stimulated shallow groundwater depths (Figure 2C), suggesting that groundwater depth had no noticeable impact on the dissolution of phosphate minerals and the release of fixed P in a short period. However, in the field deep groundwater experiment, soil organic P concentration at 11.0 m groundwater depth was significantly greater than those at 2.5 and 4.5 m (Figure 2D). It is attributed to the dissolution of phosphate minerals, and the release of fixed phosphorus might have occurred over a prolonged period. The recalcitrant soil P pools do not improve in the short term (Lehmann et al., 2001). This result is also consistent with the observation that the P density of paddy soil is influenced by groundwater table and soil drainage (Lin et al., 2009). In addition, we concluded that P concentration was greater at 11.0 m groundwater depth because the soil depth at 0–20 cm was drier, resulting in the P loss at 11.0 m groundwater depth was less than that at 2.5 and 4.5 m. It is also in accordance with the observation that at 11.0 m groundwater depth, soil water content was significantly lower than at 2.5 and 4.5 m (Supplementary Figure S1).
The release of soil available K concentration is significantly affected by soil moisture and groundwater (Wang et al., 2012; Chen et al., 2014; Chen et al., 2017). In this study, soil available K concentrations at 0.4 and 0.8 m groundwater depths were significantly greater than those at 1.8 and 2.2 m groundwater depths in the shallow groundwater depth (Figure 2E), which suggested that soil moisture levels at 0.4 and 0.8 m groundwater depths were suitable for the release of soil available K. This finding is consistent with the fact that soil moisture is positively related to soil available K, because changes in soil water affect the shrinkage and expansion of clay minerals, resulting in the variation of fixation and the release of soil K (Chen et al., 2014). In addition, soil available K concentration at 11.0 m groundwater depth was significantly higher than those at 2.5 and 4.5 m in deep groundwater (Figure 2F). This finding suggested that soil available K loss was likely lower at 11.0 groundwater depth than at other depths.
Importance of groundwater depth as an explanatory variable for soil nutrient variability
Soil nutrient concentration is determined by multiple factors, such as water levels (Hefting et al., 2004; Rhymes et al., 2016), temperature (Reich and Oleksyn, 2004; Lü et al., 2014), precipitation (Zhu et al., 2017), soil moisture content (Hefting et al., 2004; Lü et al., 2014), ages of plants (Chen et al., 2014), and human factors (Wang et al., 2012; Chen et al., 2017). In this study, our results confirm the second hypothesis that groundwater depth was one of the most critical environmental factors affecting soil nutrient variation in an arid desert (Figure 4; Supplementary Figure S6; Supplementary Table S1, S2). Groundwater depths had a substantial direct effect on soil nitrate-N (−0.25) and soil available K (−0.26) concentrations in stimulated shallow groundwater (Figure 4A), and soil nitrate-N (0.85), soil organic C (0.58), soil available P (0.56) and available K (0.60) concentrations in field deep groundwater (Figure 4B). Kalita and Kanwar (1993) reported that groundwater depth affects the nitrate-N concentration in groundwater. Nitrate-N concentration in groundwater decreases with increasing groundwater depth. This finding is related to the fact that the level of soil nitrate-N concentration that leached to the groundwater decreased with increasing depth. It is also in accordance with the fact that soil nitrate-N concentration was greatest at 1.2 m than those at 0.4 and 0.8 m depths (Figure 1A), and soil nitrate-N concentrations were greatest at 11.0 m groundwater depths than at 2.5 and 4.5 m (Figure 1B). Soil available K concentration was greatly determined by groundwater depth in field deep groundwater (Figures 3, 4B). This finding is consistent with our previous study that groundwater depth had a significantly positive impact on soil available P and K concentrations at 2.5–11.0 m depth (Zhang et al., 2018b), and groundwater depth significantly influenced the soil K concentrations at 2.25–3.26 m depth in a desert riparian ecosystem (Zhang et al., 2018c).
Groundwater depth had a significant impact on soil organic C (0.58) and available P (0.61) concentrations in field deep groundwater (Figure 4B). This finding was consistent with the result that soil C and P concentrations at 11.0 m groundwater depth were significantly greater than those at 4.5 m (Zhang et al., 2018b), and groundwater depth significantly influenced the soil organic C and available P concentrations at 2.25–3.26 m depth in a desert riparian ecosystem (Zhang et al., 2018c). In the present study, groundwater depth was the driving force for soil nitrate-N, soil organic C, and available P and K variations in deep groundwater (2.5–11.0 m), indicating groundwater depth was the most crucial factor affecting soil nutrient concentration at the south rim of the Taklamakan Desert.
Although we did a series of research work through a 2-year simulation and field experiments, there are still three limitations in our manuscript. First, we just tested the topsoil nutrients at 2.5, 4.5, and 11.0 m depths in the present study because we found no significant differences between the soil organic C, total and available N, and P concentrations beneath 50 cm soil depth at depths of 2.5, 4.5, and 11.0 m on the same research site (Liu, 2011). Hence, this paper can not elucidate how groundwater influences different layers of soil nutrient concentrations. The soil nutrients at 0.2–11.0 m should be tested to reveal the influence of groundwater depth on different layers of soil nutrients in the future. Second, the soil particle size distribution, commonly used to classify soils and determine related soil properties, significantly affects soil water movement and soil erosion. As soil depth increased, soil particle size decreased in Northwestern China (Zhao et al., 2016). The fractal dimension values of soil particles ranged from 2.11 to 2.27 in the Cele Oasis at the southern rim of the Taklimakan Desert. The soil particle size in our study area is closely related to the soil organic matter contents (Gui et al., 2010). We believe the fine soil particles are essential in affecting soil C, N, P, and K concentrations under different groundwater depths. Hence, the mechanism of fine soil particles influencing soil nutrient cycles under different groundwater depths should be investigated in the future. Third, the effects of groundwater depth on soil nutrients may be over a long period and a complex process. Hence, more long-term data and environmental factors (temperature, precipitation, soil O2 levels, and microorganism) on soil nutrients in control and field experiments under different groundwater depths are needed to measure and elucidate the response mechanism of groundwater depth in the future. Despite the above limitations, our study synthesized the investigation of the changes in soil nutrients under varying groundwater depths in an arid desert ecosystem. Our results provide novel evidence that groundwater depth modulates different mechanisms affecting the concentrations of C, N, P, and K in the sandy soil of the desert in an arid region.
Conclusion
Groundwater depths significantly influenced soil nutrient concentrations in shallow (0.4–2.2 m) and field deep (2.5–11.0 m) groundwater. Soil nitrate-N and soil available K concentrations were directly affected by the groundwater depths in shallow and deep groundwater. However, groundwater depth hardly altered soil ammonium-N concentration in different groundwater depths. In the shallow groundwater, depths indirectly influenced soil inorganic-N, soil organic C, and available P concentrations. In the field deep groundwater, soil inorganic-N was indirectly affected by depth. Besides, depths directly influenced soil organic C and available P concentrations in the field deep groundwater. Hence, groundwater depth is one of the most critical factors affecting soil nutrients in an arid desert ecosystem. This study could help elucidate trends in C, N, P, and K concentrations in desert soil with different groundwater levels and their responses to declining groundwater depth in a desert. These findings would further enrich the existing soil heterogeneity studies and may contribute to the protection and restoration of desert plants in a hyper-arid desert ecosystem.
Data availability statement
The raw data supporting the conclusion of this article will be made available by the authors, without undue reservation.
Author contributions
BZ, XG, and FZ designed the study. BZ, ZZ, HY, and CL performed the experiments and collected the data. BZ and ZZ analyzed the data. BZ, QY, YG, HY, YL, CH, and GT interpreted the data and wrote the manuscript. All authors contributed to the article and approved the submitted version.
Funding
This study was supported by the National Natural Science Foundation of China (31500367), the Western Young Scholar Program-B of the Chinese Academy of Sciences (2018-XBQNXZB-018), the Project for High-level talent of Xinjiang Institute of Ecology and Geography (E215010201; E0502101), the Program of Joint Funds of the National Natural Science Foundation of China and the Government of Xinjiang Uygur Autonomous Region of China (U1903102), the Youth Innovation Promotion Association Foundation of the Chinese Academy of Sciences (2020435) and the Third Batch of Tianshan Talents Program of Xinjiang Uygur Autonomous Region (2021-2023).
Acknowledgments
We would like to thank Hanlin Luo, Shiming Li and Bo Wang for assistance in fieldwork at the Cele station and Mingfang Hu for assistance in soil elemental analysis. We are also grateful to the anonymous referees for reviewing this manuscript.
Conflict of interest
The authors declare that the research was conducted in the absence of any commercial or financial relationships that could be construed as a potential conflict of interest.
Publisher’s note
All claims expressed in this article are solely those of the authors and do not necessarily represent those of their affiliated organizations, or those of the publisher, the editors and the reviewers. Any product that may be evaluated in this article, or claim that may be made by its manufacturer, is not guaranteed or endorsed by the publisher.
Supplementary material
The Supplementary Material for this article can be found online at: https://www.frontiersin.org/articles/10.3389/fenvs.2022.939382/full#supplementary-material
References
Burgin, A. J., Groffman, P. M., and Lewis, D. N. (2010). Factors regulating denitrification in a riparian wetland. Soil Sci. Soc. Am. J. 74, 1826–1833. doi:10.2136/sssaj2009.0463
Chen, Q. C., Xu, F. L., Wang, W. L., and Cheng, Z. W. (2014). Seasonal dynamics of available K in Soil for different ages of Larix principis-rupprechtii in the northern foot of the Qinling. J. Plant Nutr. Fertil. 20 (5), 1243–1249.
Chen, Y., Qi, Y. B., Wang, Y. Y., Zhang, L. L., and Liu, J. J. (2017). Spatial variability and factors affecting soil available potassium in the central Qinling-Daba Mountain area. Res. Environ. Sci. 30 (2), 257–266. doi:10.13198/j.issn.1001-6929.2017.01.47
Cheng, M., and An, S. S. (2015). Responses of soil nitrogen, phosphorous and organic matter to vegetation succession on the Loess Plateau of China. J. Arid. Land 7 (2), 216–223. doi:10.1007/s40333-014-0043-3
Fang, X., Xue, Z. J., Li, B. C., and An, S. S. (2012). Soil organic carbon distribution in relation to land use and its storage in a small watershed of the loess plateau, China. Catena 88, 6–13. doi:10.1016/j.catena.2011.07.012
Gami, S., Lauren, J., and Duxbury, J. (2009). Influence of soil texture and cultivation on carbon and nitrogen levels in soils of the eastern Indo-Gangetic Plains. Geoderma 153, 304–311. doi:10.1016/j.geoderma.2009.08.003
Gelaw, A., Singh, B., and Lal, R. (2014). Soil organic carbon and total nitrogen stocks under different land uses in a semi-arid watershed in Tigray, Northern Ethiopia. Agric. Ecosyst. Environ. 188, 256–263. doi:10.1016/j.agee.2014.02.035
Guan, F. Y., Xia, M. P., Tang, X. L., and Fan, S. H. (2017). Spatial variability of soil nitrogen, phosphorus and potassium contents in Moso bamboo forests in Yong'an City, China. Catena 150, 161–172. doi:10.1016/j.catena.2016.11.017
Gui, D. W., Lei, J. Q., Zeng, F. J., Mu, G, J., Zhu, J, T., Wang, H., et al. (2010). Characterizing variations in soil particle size distribution in oasis farmlands-a case study of the Cele Oasis. Math. Comput. Model. 51, 1306–1311. doi:10.1016/j.mcm.2009.10.035
Gui, D. W., Zeng, F. J., Liu, Z., and Zhang, B. (2013). Characteristics of the clonal propagation of Alhagi sparsifolia Shap. (Fabaceae) under different groundwater depths in Xinjiang, China. Rangel. J. 35, 355–362. doi:10.1071/RJ13004
Hati, K., Swarup, A., Mishra, B., Manna, M., Wanjari, R., Mandal, K., et al. (2008). Impact of long-term application of fertilizer, manure and lime under intensive cropping on physical properties and organic carbon content of an Alfisol. Geoderma 148, 173–179. doi:10.1016/j.geoderma.2008.09.015
Hefting, M., Clement, J. C., Dowrick, D., Cosandey, A. C., Bernal, S., Cimpianc, C., et al. (2004). Water table elevation controls on soil nitrogen cycling in riparian wetlands along a European climatic gradient. Biogeochemistry 67, 113–134. doi:10.1023/B:BIOG.0000015320.69868.33
Kalita, P. K., and Kanwar, R. S. (1993). Effect of water-table management practices on the transport of nitrate-N to shallow groundwater. Trans. ASAE 36 (2), 413–422. doi:10.13031/2013.28353
Lefcheck, J. S. (2016). PiecewiseSEM: Piecewise structural equation modelling in R for ecology, evolution, and systematics. Methods Ecol. Evol. 7, 573–579. doi:10.1111/2041-210X.12512
Lehmann, J., Günther, D., Socorroda Mota, M., Pereira, de Almeida, M., Zech, W., and Kaiser, K. (2001). Inorganic and organic soil phosphorus and sulfur pools in an Amazonian multistrata agroforestry system. Agroforest Syst. 53 (2), 113–124. doi:10.1023/A:1013364201542
Li, X. Y., Lin, L. S., Zhao, Q., Zhang, X. M., and Thomas, F. M. (2010). Influence of groundwater depth on species composition and community structure in the transition zone of Cele oasis. J. Arid. Land 2, 235–242. doi:10.3724/SP.J.1227.2010.00235
Li, C. J., Zeng, F. J., Zhang, B., Liu, B., Guo, Z. C., Gao, H. H., et al. (2015). Optimal root system strategies for desert phreatophytic seedlings in the search for groundwater. J. Arid. Land 7 (4), 462–474. doi:10.1007/s40333-015-0006-3
Li, Y., Niu, S. L., and Yu, G. R. (2016). Aggravated phosphorus limitation on biomass production under increasing nitrogen loading: a meta-analysis. Glob. Chang. Biol. 22, 934–943. doi:10.1111/gcb.13125
Lin, J. S., Shi, X. Z., Lu, X. X., Yu, D. S., Wang, H. J., Zhao, Y. C., et al. (2009). Storage and spatial variation of phosphorus in paddy soils of China. Pedosphere 19, 790–798. doi:10.1016/S1002-0160(09)60174-0
Liu, E. K., Yan, C. R., Mei, X. R., He, W. Q., Bing, S. H., Ding, L. P., et al. (2010). Long-term effect of chemical fertilizer, straw, and manure on soil chemical and biological properties in northwest China. Geoderma 158, 173–180. doi:10.1016/j.geoderma.2010.04.029
Liu, B., He, J. X., Zeng, F. J., Lei, J. Q., and Arndt, S. K. (2016). Life span and structure of ephemeral root modules of different functional groups from a desert system. New Phytol. 211, 103–112. doi:10.1111/nph.13880
Liu, M. (2007). Study on groundwater spatiotemporal distribution Law and its environmental effects in Hotan oasis. Master thesis. Xi'an: Xi'an University of Technology.
Liu, Z. (2011). Root distribution of Alhagi sparsifolia Shap. response to different water conditions. Master Thesis. Urumchi: Xinjiang Institute of Ecology and Geography, Chinese Academy of Sciences.
Lü, X. T., Dijkstra, F. A., Kong, D. L., Wang, Z. W., and Han, X. G. (2014). Plant nitrogen uptake drives responses of productivity to nitrogen and water addition in a grassland. Sci. Rep. 4, 4817. doi:10.1038/srep04817
Ma, L. N., Lü, X. T., Liu, Y., Guo, J. X., Zhang, N. Y., Yang, J. Q., et al. (2011). The effects of warming and nitrogen addition on soil nitrogen cycling in a temperate grassland, northeastern China. PLoS ONE 6 (11), e27645. doi:10.1371/journal.pone.0027645
Nelson, D. W., and Sommers, L. E. (1975). A rapid and accurate method for estimating organic carbon in soil. P. Indiana Acad. Sci. 84, 456–462.
Nilsson, C., Jansson, R., and Zinko, U. (1997). Long-term responses of river-margin vegetation to water-level regulation. Science 276 (5313), 798–800. doi:10.1126/science.276.5313.798
Olsen, S. R., Cole, C. V., Watanabe, F. S., and Dean, L. A. (1954). Estimation of available organic carbon in soil. P. Indiana Acad. Sci. 84, 456–462.
Patil, R. H., Laegdsmand, M., Olesen, J. E., and Porter, J. R. (2010). Effect of soil warming and rainfall patterns on soil N cycling in Northern Europe. Agric. Ecosyst. Environ. 139, 195–205. doi:10.1016/j.agee.2010.08.002
Quilchano, C., Marañón, T., Pérez-Ramos, I., Noejovich, L., Valladares, F., and Zavala, M. (2008). Patterns and ecological consequences of abiotic heterogeneity in managed cork oak forests of Southern Spain. Ecol. Res. 23, 127–139. doi:10.1007/s11284-007-0343-6
Reich, P. B., and Oleksyn, J. (2004). Global patterns of plant leaf N and P in relation to temperature and latitude. Proc. Natl. Acad. Sci. U. S. A. 101, 11001–11006. doi:10.1073/pnas.0403588101
Rhymes, J., Jones, L., Wallace, H., Jones, T. G., Dunn, C., and Fenner, N. (2016). Small changes in water levels and groundwater nutrients alter nitrogen and carbon processing in dune slack soils. Soil Biol. Biochem. 99, 28–35. doi:10.1016/j.soilbio.2016.04.018
Ross, D., Tate, K., Scott, N., and Feltham, C. (1999). Land-use change: effects on soil carbon, nitrogen and phosphorus pools and fluxes in three adjacent ecosystems. Soil Biol. Biochem. 31, 803–813. doi:10.1016/S0038-0717(98)00180-1
Shipley, B. (2002). Cause and correlation in biology: a user's guide to path analysis, structural equations and causal inference. Cambridge: Cambridge University Press.
Song, H. X., and Li, S. X. (2005). Effects of root uptake function and soil water on NO3- -N and NH4+-N distribution. Sci. Agric. Sin. 38 (1), 96–101.
Tao, Z., Cidan, L. J., Zhang, S. H., Xie, C. J., Lin, P. S., Liao, Q., et al. (2013). Controls over soil organic carbon content in grasslands. Acta eco. Sin. 33 (9), 2684–2694. doi:10.5846/stxb201201150090
Vance, C. P., Uhde-Stone, C., and Allan, D. L. (2003). Phosphorus acquisition and use: critical adaptations by plants for securing a nonrenewable resource. New Phytol. 157, 423–447. doi:10.1046/j.1469-8137.2003.00695.x
Wang, Y., Amundson, R., and Niu, X. F. (2000). Seasonal and altitudinal variation in decomposition of soil organic matter inferred from radiocarbon measurements of soil CO2 flux. Glob. Biogeochem. Cycles 14 (1), 199–211. doi:10.1029/1999GB900074
Wang, Z., Lu, J. W., Zhang, W. J., and Li, X. K. (2012). Influential factors on soil available potassium evaluation in agriculture. Soils 44 (6), 898–904.
Zeng, F. J., Bleby, T. M., Landman, P. A., Adams, M. A., and Arndt, S. K. (2006). Water and nutrient dynamics in surface roots and soils are not modified by short-term flooding of phreatophytic plants in a hyperarid desert. Plant Soil 279, 129–139. doi:10.1007/s11104-005-0498-2
Zhang, B., Gui, D. W., Gao, X. P., Shareef, M., Li, L., and Zeng, F. J. (2018a). Controlling soil factor in plant growth and salt tolerance of leguminous plant alhagi sparsifolia shap. in saline deserts, northwest China. Contemp. Probl. Ecol. 11, 111–121. doi:10.1134/S199542551801002X
Zhang, B., Gao, X. P., Li, L., Lu, Y., Shareef, M., Huang, C. B., et al. (2018b). Groundwater depth affects phosphorus but not carbon and nitrogen concentrations of a desert phreatophyte in northwest China. Front. Plant Sci. 9, 338. doi:10.3389/fpls.2018.00338
Zhang, X. L., Guan, T. Y., Zhou, J. H., Cai, W. T., Gao, N. N., Du, H., et al. (2018c). Groundwater depth and soil properties are associated with variation in vegetation of a desert riparian ecosystem in an arid area of China. Forests 9, 34. doi:10.3390/f9010034
Zhang, B., Tang, G. L., Yin, H., Zhao, S. L., Shareef, M., Liu, B., et al. (2021a). Groundwater depths affect phosphorus and potassium resorption but not their utilization in a desert phreatophyte in its hyper-arid environment. Front. Plant Sci. 12, 665168. doi:10.3389/fpls.2021.665168
Zhang, B., Tang, G. L., Luo, H. L., Yin, H., Zhang, Z. H., Xue, J., et al. (2021b). Topsoil nutrients drive leaf carbon and nitrogen concentrations of a desert phreatophyte in habitats with different shallow groundwater depths. Water 13, 3093. doi:10.3390/w13213093
Zhao, Y., Feng, Q., and Yang, H. D. (2016). Soil salinity distribution and its relationship with soil particle size in the lower reaches of Heihe River, Northwestern China. Environ. Earth Sci. 75, 810. doi:10.1007/s12665-016-5603-8
Keywords: soil nutrient, groundwater drawdown, sandy soil, human activity, soil heterogeneity, desert ecosystem
Citation: Zhang B, Zeng F, Gao X, Shareef M, Zhang Z, Yu Q, Gao Y, Li C, Yin H, Lu Y, Huang C and Tang G (2022) Groundwater depth alters soil nutrient concentrations in different environments in an arid desert. Front. Environ. Sci. 10:939382. doi: 10.3389/fenvs.2022.939382
Received: 09 May 2022; Accepted: 20 July 2022;
Published: 22 August 2022.
Edited by:
Ning Chen, Lanzhou University, ChinaReviewed by:
Guohua Wang, Shanxi Normal University, ChinaYafeng Zhang, Northwest Institute of Eco-Environment and Resources (CAS), China
Copyright © 2022 Zhang, Zeng, Gao, Shareef, Zhang, Yu, Gao, Li, Yin, Lu, Huang and Tang. This is an open-access article distributed under the terms of the Creative Commons Attribution License (CC BY). The use, distribution or reproduction in other forums is permitted, provided the original author(s) and the copyright owner(s) are credited and that the original publication in this journal is cited, in accordance with accepted academic practice. No use, distribution or reproduction is permitted which does not comply with these terms.
*Correspondence: Bo Zhang, zhangbo@ms.xjb.ac.cn; Gangliang Tang, tanggangliang@ms.xjb.ac.cn