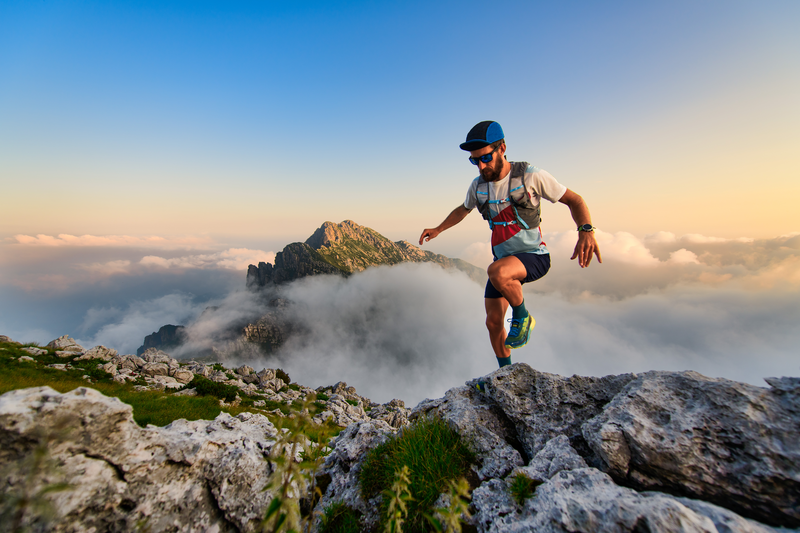
95% of researchers rate our articles as excellent or good
Learn more about the work of our research integrity team to safeguard the quality of each article we publish.
Find out more
REVIEW article
Front. Environ. Sci. , 23 August 2022
Sec. Environmental Economics and Management
Volume 10 - 2022 | https://doi.org/10.3389/fenvs.2022.938017
Global concerns over increasing carbon emissions, climate change, decreasing environmental quality, limited and uneven endowments of fossil fuels, rising energy demand, and volatile oil prices have inspired the move toward global energy system decarbonization. The challenges of achieving ambitious climate targets and sustainable development cannot be solved without the significant efforts of the Global South. Regarding net-zero emissions, the main issue for developing countries is avoiding future emissions from industrialization and lock-in into fossil fuel-intensive technologies. However, achieving strategic and ambitious climate targets in the Global South will require understanding the economic implications of decarbonization. This study’s objective and key contribution are to systematically review and provide a detailed assessment of the literature on decarbonization in the Global South to understand the multi-level economic implications of achieving net-zero emissions in the Global South. The assessment considers four broad themes–investment costs, employment impacts, economic growth, and other macroeconomic impacts (such as consumption, debt level, net savings, income and welfare, and trade balance, among others). Overall, the review finds that energy system decarbonization requires substantial investment outlay. Also, it is linked with job displacement in fossil energy sectors and job creation in green sectors. However, there is no unanimous evidence on net job creation in developing countries. Additionally, the GDP impacts are ambiguous; some studies find future GDP growth impacts, while others find GDP decline impacts of decarbonization scenarios. This paper also provides directions for future research.
A thriving global economy requires energy (Bhuiyan et al., 2022; Xie et al., 2022). In other words, all sectors directly or indirectly linked to economic growth and development necessitate using some form of energy. Energy demand has increased in both developed and developing countries in recent decades. While developed countries’ living standards have broadly improved, developing countries have lagged in matching energy supply with energy demand due to increasing living standards, population, urbanization, and industrialization (Bhattarai et al., 2022; Bhuiyan et al., 2022). In 2020, the total global primary energy consumption (PEC) was 557 Exajoules (EJ) (BP, 2021). Renewable energy consumption in 2020 was 32 EJ (or 6% of PEC), while fossil fuel consumption was 463 EJ (or 83% of PEC). The remainder was accounted for by nuclear energy and hydroelectricity (BP, 2021).
Global North and South countries continue to have significant energy access and use disparities. North America and Europe collectively account for 33.3% of global energy consumption (BP, 2021). In contrast, the Middle East, South and Central America, and Africa represent, respectively, 6.5, 4.7, and 3.3% of the world’s population (BP, 2021). In addition, energy-related carbon dioxide (CO2) emissions have risen sharply in recent decades due to the increasing energy demand and consumption of OECD member nations and emerging economies such as China, India, Brazil, and South Africa (Bhattarai et al., 2022).1 In 2020, energy-related CO2 emissions accounted for approximately 31.5 GtCO2, or more than 75% of total global CO2 emissions (Friedlingstein et al., 2022; IEA, 2021b; IEA, 2021a). Anthropogenic activities such as energy exploitation and consumption emissions have been linked to climate change (IPCC, 2014; Islam et al., 2021; Rehman et al., 2021; Irfan et al., 2022). In its current state, the global energy sector has weaknesses that manifest through decreasing environmental quality, limited and uneven global endowments of fossil fuels, rising global energy demand, and volatile oil prices (Tang et al., 2022; Xie et al., 2022). To avert further economic and environmental damage, a global transition from the conventional generation and consumption of nonrenewable energy sources toward renewable energy sources is needed (Delgado et al., 2020; Lallana et al., 2021).
The Paris Agreement provided a platform for Parties to pledge to reduce global warming by 2°C and even well below that, to 1.5°C, by mid-century (that is, by 2050) compared to pre-industrial times. The Agreement requires Parties to submit their intentions and strategies through Nationally Determined Contributions (NDC) (Lallana et al., 2021; Le Treut et al., 2021). Furthermore, in its 1.5°C Special Report, the Intergovernmental Panel on Climate Change (IPCC, 2018) stressed the need to reduce global CO2 emissions to net-zero by 2050 to attain the 1.5C target for global temperature increase. Recent studies, such as the United Nations Framework Convention on Climate Change (UNFCCC) Synthesis Report (UNFCC, 2021) and Johnstone et al. (2020), have shown that commitments made by countries through their NDCs are inadequate to attain the 2°C or 1.5°C targets; suggesting an energy system deep decarbonization is needed. Consequently, governments have been urged to boost their ambitions through periodic revised NDCs with incremental ambitions toward climate change mitigation.
Some countries and regions have responded by formulating mid-term and long-term low-carbon and net-zero emissions goals within the constraints of existing resources, technology, and economy (Bhattarai et al., 2022; UNFCCC, 2022). A cornerstone of these goals is increasing the share of renewables in the energy mix. The United States, Canada and Mexico were the first to respond to this call in 2016 by producing the first reported official mid-century strategies (Delgado et al., 2020). These actions by these three countries have motivated other countries (more than 51 by March 2022) that have submitted official low-emission or net-zero emission strategies (UNFCCC, 2022). For example, Colombia announced its ambition for climate neutrality by mid-century during the 2019 United Nations (UN) Climate Change Summit.
Furthermore, during the 26th UN Conference of Parties (COP26), Nigeria’s President pledged that the country would reach net-zero emission by 2060.2 Through ambitious energy and climate policies, the energy sector is critical to successful pathways to net-zero emissions by mid-century. The IEA (2021b) reports that mid-century low-carbon or net-zero emission targets require substantial expansion in clean energy innovations. Le Treut et al. (2021) note that there are currently practical solutions for low-carbon or net-zero energy systems by mid-century. The solutions include rapid growth of low-carbon electricity generation, reduction of fossil fuel and rapid retirement of fossil fuel power plants, renewable energy revolution, technological progress, electrification of energy end uses, and energy demand management focusing on energy-efficient equipment and processes (Hasanov et al., 2021b; Le Treut et al., 2021). This paper uses “decarbonization” to collectively refer to clean or low carbon energy sources and technologies. It also refers to deep decarbonization and net-zero emissions strategies such as renewable energy deployment.
Decarbonization commitments are undoubtedly linked to the economic considerations in two crucial ways. Firstly, the need to reduce emissions while sustaining the economic growth necessary to achieve other sustainable development goals (SDGs) and, secondly, the complex problem of allocating the emission reduction costs and extant emissions budget (Wang et al., 2021). Empirical studies analyzing the CO2-income (GDP) nexus broadly estimate their elasticity. A recent survey shows that the estimated elasticities range from a 0.3% reduction to a 2.5% increase in CO2 emissions for each 1% GDP increase (see Wang et al., 2021). This ambiguity has hampered policymakers’ ability to depend on empirical evidence in weighing choices amongst likely opposing social objectives. Recent ex-ante and ex-post analyses show that some countries have simultaneously decreased CO2 emissions without hindering economic growth (see Wang et al., 2021). While ex-ante analyses predict CO2 emissions based on modeling hypothesized causal links between CO2 emissions and decarbonization strategies, ex-post analyses use historical data to conduct analyses of the same phenomena under discussion.
Furthermore, the Environmental Kuznets curve (EKC) hypothesis is widely used to analyze emissions reduction and income growth in several economies (York et al., 2003; López-Menéndez et al., 2014; Ben Jebli and Kahia, 2020; Emenekwe et al., 2021). The EKC hypothesis suggests that emissions increase with economic growth up to a point where it starts declining. Simply put, individuals are willing to pay for environmental quality at a higher level of affluence (York et al., 2003; López-Menéndez et al., 2014). Thus, obtaining accurate ex-post estimates of CO2-GDP elasticities is essential in economic research since they often inform parameter values for calibrating models in ex-ante assessments.
Recent research has challenged the EKC hypothesis’ ability to explain emission reductions during growth phases. Firstly, empirical research on the EKC draws misleading conclusions about validating the hypothesis without checking whether the estimated income turning point falls within the data sample (Hasanov et al., 2021a). Secondly, many studies misinterpret income and income squared as CO2 emission elasticities. Hasanov et al. (2021a) investigate how units of measurement for independent variables affect estimated non-leading coefficients. They conclude that using estimates of non-leading terms to select the preferred specification of an estimated EKC relationship is inaccurate and should not be used since it could lead to misleading inferences. Third, several studies analyze CO2 emissions ad-hoc without a theoretical basis. Hasanov et al. (2021b) develop a theoretical framework that links CO2 to total factor productivity, renewable energy consumption, exports, imports, and income. The paper’s theoretical framework is only valid when the explanatory variables are considered. Empirical studies should theoretically justify their preferred specifications; else, their analyses are theoretically unfounded.
Our desktop review shows that empirical studies support not all countries’ long-term decarbonization commitments. Some countries are developing quantitative analyses to aid policymaking toward a just decarbonization process. Besides, several studies have analyzed the feasibility of decarbonization strategies in different countries (Le Treut et al., 2021). Most of these studies are based on bottom-up energy accounting models focusing on either the techno-economic feasibility, energy supply, and emissions impacts of the energy supply and energy demand sectors (such as power, transport, building, industry, etc.) (Emodi et al., 2017; Grande-Acosta and Islas-Samperio, 2017; Dioha et al., 2019; Islas-Samperio et al., 2019).
Moreover, policymakers seek to understand the long-term impacts of the decarbonization strategies beyond their techno-economic feasibility, energy supply and emissions impacts within the energy sector (Le Treut et al., 2021). Given the vast importance of energy on several dimensions of human activities and the economy, it is expected that shocks in the energy sector would extend to other sectors and affect macroeconomic outcomes. The impacts could be measured in terms of gross domestic product impacts, welfare, employment, and investment costs, among others. Recent efforts to analyze decarbonization’s multi-sectoral and macroeconomic impacts have been primarily based on bottom-up models. Some studies have used top-down models, such as computable general equilibrium (CGE) models, to analyze the energy, emissions and macroeconomic impacts of mid-century decarbonization strategies with differing outcomes (Schers, 2018; Delgado et al., 2020; Gupta et al., 2020). Additionally, recent advances in computational and analytical methods have enabled hybrid analyses using both soft- and hard-linking to get the best of both bottom-up and top-down models for the study of the multi-level impacts of decarbonization strategies (Winkler et al., 2017; Soummane et al., 2019; Lallana et al., 2021; Le Treut et al., 2021).
The structural transformation that will emanate from deep decarbonization strategies is likely to affect net jobs, but how smooth will labor mobility across sectors be? Is structural shift expressly guaranteed? How will countries ensure that the net job impact of decarbonization strategies is not harmful? Since decarbonization entails investment into new energy sources, the retirement of existing energy sources, and potentially the risk of stranded assets, writing-off retired assets, sourcing and incentivizing green investments could have significant macroeconomic implications. There is also the concern of sustaining growth and keeping the macroeconomy stable. The associated cost of energy system transformation is a core concern for policymakers tasked with formulating emissions reduction targets in line with the Paris Agreement’s mid-century climate mitigation strategies (Su et al., 2021). Thus, the most critical policy considerations are how this decarbonization relates to economic development and, specifically, what macroeconomic impacts this transition has (IPCC, 2014). Furthermore, considerable investments in carbon abatement technologies and a shift away from fossil-based technologies are crucial to keeping the global temperature increase well below 2°C. Consequently, rapid changes in investment trends are expected to have wide-reaching macroeconomic implications (Le Treut et al., 2021; Su et al., 2021).
Despite the growing number of studies on the decarbonization of energy systems, a dearth of systematic reviews of these studies exists. This article aims to fill the knowledge gap on the decarbonization of energy systems in selected countries of the Global South. Our classification of countries into the Global South is based on Fuhr’s (Fuhr, 2021) analyses of Global South development and emissions nexus. The focus on the Global South countries is twofold: firstly, Global South countries have contributed fewer emissions and, consequently, less to global warming than the Global North. Secondly, the Paris Agreement calls for common but differentiated efforts to see countries boost their climate change mitigation ambitions (Delgado et al., 2020). However, many low-income developing countries with low adaptive capacity are likely to suffer most from the consequences of climate change. Consequently, pursuing decarbonization may pose a threat to their crucial development objectives. However, research on this topic is relatively recent and fewer in the Global South (Fuhr, 2021).
In addition, we provide a multi-level macroeconomic reference point for policymakers and other key stakeholders involved in discussions on NDCs, long-term climate action plans, and the Sustainable Development Goals (SDGs) concerning decarbonizing energy systems. This reference point is valuable for developing economies which face the challenge of providing their citizens with adequate access to energy and confront considerable transition difficulties. While China is considered a Global South country, our preliminary search showed several studies focused on the decarbonization of China’s economy and specific sectors in the economy. The noteworthy point is that China is the only seemingly Global Southern country among the three largest global economies.3 This situation makes China an outlier, in our opinion. Thus, including China would result in a China-centric analysis that is not the intention of this review, so we drop China.
Systematic literature review (SLR) is a standard research methodology for focused studies (Page et al., 2021). This review method has been applied in recent literature reviews focused on environmental studies and climate change in general (Shaffril et al., 2018; Emodi et al., 2019; Bhattarai et al., 2022). The SLR is particularly valuable in analyzing the current state-of-the-art knowledge in a subject matter, detecting similarities and divergence within a subject matter, and classifying information topically. The SLR is also highly valuable in distinguishing the less investigated areas and subsequently creating direction for future research.
Based on the preceding discussions, the main objective of this study is to review the evidence in the ex-ante modeling literature on the multi-level macroeconomic implications of energy system decarbonization for selected Global South countries. The review of ex-post evidence is beyond the scope of the current review. The specific research questions are:
(1) To examine how the research field on macroeconomic effects of energy system decarbonization has evolved in terms of geographical locus and methods
(2) To identify the economy-wide investment costs of energy system decarbonization in the Global South
(3) To identify how will the structural changes emanating from decarbonization affect employment
(4) To identify the major macroeconomic effects (besides investment cost and employment impact) of energy system decarbonization pathways in the Global South
(5) To discuss the critical research and policy implications for the Global South developing countries
The rest of the paper is structured as follows. The second section presents the methodology applied in this study. The third section assesses the characteristics of the selected studies while highlighting the impacts of decarbonization on employment, macroeconomic indicators, and investment cost implication of strategies. The fourth section discusses findings from the studies, limitations of the review and areas for future research (and lessons for Global South developing countries). The last section concludes the study policy recommendations.
This section presents the methodology used in this study. The methodology used in this study is the systematic literature review (SLR) approach applied in other related literature reviews (see Shaffril et al., 2018; Emodi et al., 2019; Bhattarai et al., 2022). Therefore, this review summarizes the literature on the multi-economic implications of energy system decarbonization for selected Global South countries. Figure 1 shows the steps involved in the systematic review process: 1) defining the topic and identifying the research question(s); 2) using a keyword search string to identify relevant studies; 3) reading articles in detail to select studies for the review and structure a database; 4) extract data from the retrieved studies and place in the database; and 5) analyze, refine and summarize results for publication. A noteworthy point here is that the researchers used the PRISMA approach and the data sources, inclusion and exclusion criteria, review process, data extraction, and analysis.
This systematic review employed the PRISMA statement, which stands for Preferred Reporting Items for Systematic reviews and Meta-Analyses (Page et al., 2021). It is common practice to use PRISMA in environmental studies. In Shaffril et al. (Shaffril et al., 2018), the authors report two distinct advantages: first, it allows for systematic study by specifying specific research topics. Lastly, it attempts to filter through a large body of scientific material over an agreed-upon period to determine what should be included and what should be excluded. It also allows the researchers to conduct an exhaustive search of terms connected to the decarbonization of the energy system and its multi-level economic implications.
The three standard journal databases used in this study were Web of Science (WoS), Scopus, and Google Scholar. WoS is a comprehensive database with over 21,000 peer-reviewed journal publications, books, and proceedings from 256 fields, including environmental studies, social issues, and multidisciplinary social sciences.4 This ranking includes citations, papers, and citations per paper, which has been in place for some years, thanks to Clarivate Analytics. We also use the Scopus database, which boasts over 25,000 peer-reviewed journals from over 5,000 publishers worldwide. It is a rich abstract and citation database for environmental, social, and biological science topics.5 We also include the Google Scholar database, a potential source for relevant scientific and gray literature that may not have been published in the two preceding journal databases.
Table 1 presents information on the criteria considered for selecting papers for review. Regarding the literature type, peer-reviewed journal articles and gray literature of modelling/scenario-based studies are included for review. Therefore, we excluded ex-post analyses and non-modelling/scenario-based studies of both article journal and gray literature and review studies. The search process included only English-language publications to ease translation and avoid confusion. Regarding the review timeline, the researchers selected a period of 12 years to reflect the most recent state-of-the-art in knowledge (between 2010 and 2021).
Most importantly, only publications focusing on the Global South territories, excluding China, were selected. The researchers purposely excluded China to avoid a skewed review process. Our preliminary search showed that several articles focused on decarbonization (including deep decarbonization) of China’s economy and specific sectors in the economy. Thus, including China would result in a China-centric analysis, which is not the intention of this review. This review proceeds with selected Global South studies.
The review process was carried out in November 2021. Table 2 shows the applied keywords used during the search process.
The authors obtained 15,689 articles from the WoS database, 8,664 articles from the Scopus database and 7026 from the Google Scholar database. After careful exclusion of duplicates, 3,695 were identified. The next stage screened the articles based on titles and abstracts and excluded 3,471 articles out of the 3,695 articles from the previous stage. The articles were assessed for eligibility in the third stage. Here, the researchers excluded 192 articles because they were either literature review studies (91 items), missing documents (23 items), or out-of-scope for this review (79 articles, including non-scenario-based studies). This culminated in 31 studies selected for qualitative review (see Figure 2).
FIGURE 2. PRISM Flow Diagram of studies to be included in the systematic literature review. Source: Authors’ diagram based on PRISMA guideline (Page et al., 2021).
This section presents the findings from the assessed papers. Figure 3 shows an overview of the approach used to synthesize evidence from the literature review. First, the information is extracted about the study design. Secondly, the results include emissions and macroeconomic impacts. The latter is the focus of this study. Then the study proceeds with finding answers that address the stated objectives.
FIGURE 3. Pictorial depiction of the approach to literature review synthesis of evidence. Source: Authors’ diagram.
This sub-section presents the findings related to emissions outcomes and multi-level economic impacts of energy system decarbonization scenarios from the reviewed studies. Thirty one papers are included for review covering 21 Global South countries (Table 3 and Figure 4). The list of studies included in the review is presented in Table 3. These studies were coded using the index number to differentiate the literature source when used in the review, and the numbers were placed in square brackets (e.g., [#“xy”], where “xy” represents the number allocated to an article in Table 3). The results provided a comprehensive analysis of the current research on the multi-level economic implications of energy system decarbonization in the Global South. A total of five studies focused on India [#2, #4, #9, #12, #17 ], four studies each focused on Malaysia [#6, #26, #30, #31] and South Africa [#3, #5, #11, #14], three focused on Brazil [#7, #8, #10], two studies each focused on Argentina [#21, #27], Colombia [#18, #24], Mexico [#20, #29], and Nigeria [#13, #19]. Furthermore, one study each focused on Algeria [#16], Egypt [#16], Libya [#16], Mauritania [#16], Morocco [#16], Sudan [#16], Tunisia [#16], Bolivia [#28], Ecuador [#22], Peru [#25], Qatar [#23], Thailand [#1], Saudi Arabia [#15].
FIGURE 4. The geographical scope of the publications identified in the systematic review. Source: Authors’ diagram using Microsoft Excel map builder. Note: Some publications cover more than one country.
The reviewed studies applied three broad categories of modelling: 1) Bottom-up model (e.g., LEAP model [Low Emissions Analysis Platform], IEEEE model [Integrated energy-engineering-environmental-economic system model], TIMES model [The Integrated MARKAL-EFOM-System], etc.); 2) Top-down models (e.g., IMACLIM-BRA model, SAGEM model [South African Green Economy Model, TERM-SA CGE model, etc.], AIM/CGE India model); and Hybrid models (e.g., LEAP + IMACLIM-ARG, LEAP + KLEM-KSA, AIM/End-use model + IMACLIM-IND). In general bottom-up models accounted for about 52% of the models, while the top-down and hybrid models accounted for 26 and 22% of the models, respectively. Furthermore, the research papers commonly applied the LEAP, IMACLIM, and the AIM/End-use models. The reviewed studies used quantitative data for the modelling exercises. Specifically, the studies used publicly available data. Where data is not publicly available, data from third-party providers are used. Reliability of data is a major issue in developing countries. However, no reviewed study described detailed data access, quality issues, or approaches to resolve them.
The 31 selected papers included in our systematic review were published between 2010 and 2021. One article was published in 2010 [#1] and 2011 [#2], two in 2014 [#3, #4], three in 2016 [#5–#7], four in 2018 [#8–#11], four in 2019 [#12–#15], four in 2020 [#16–#19], and twelve in 2021 [#20–#31]. The highest number of research was published in 2021. These reviewed papers are also representative of the Global South regions such as Sub-Saharan Africa, Asia (excluding China), Middle East and North Africa (MENA), and South and Central America (Figure 5).
FIGURE 5. Trends in decarbonization research published on selected Global South countries. Source: Authors’ diagram.
The 31 papers included in our systematic review were sourced from scientific journals and gray literature. Of this number, thirty studies were sourced from journals, while only one was sourced from gray literature (Figure 6). The journals with the most publications for review here are Energy Strategy review (6), Climate Policy (5), Energy Policy (4), and Journal of Cleaner Production (2).
FIGURE 6. Source of reviewed articles classified by literature type. Source: Authors’ diagram. Note: The bar chart blue indicates journal publications, while the only chart colored gray means that the publication is sourced from gray literature (McKinsey & Co.).
These studies developed scenarios that align with the policy options available to each country reviewed. Table 4 shows the broad-level scenario characteristics of the reviewed studies. Generally, the reviewed studies model a reference or Business-as-Usual (BAU) scenario and additional decarbonization or low emissions scenario. Typically, the BAU scenarios across the studies represent a current policy environment in which there is no change in climate/mitigation policy going into the future. Besides, most reviewed studies use the respective country’s nationally determined contributions (NDC) policies as the BAU. On the other hand, the number of low carbon or decarbonization scenarios modelled varies across studies, ranging from one to four. The scenario pathways are broadly categorized into the demand-side and supply-side (Table 4).
CO2 emissions tend to be strongly linked to economic growth (Fuhr, 2021; Islam et al., 2021; Rehman et al., 2021). While most Global South countries are low emitters, they are mainly developing countries targeting rapid economic growth. The concern of the deep decarbonization studies is finding economic pathways that synchronize the need for emission reduction with that of sustained economic growth. Thus, understanding how emissions can be reduced while achieving sustained economic growth through scenarios. Emission outcomes are categorized into the reference (business-as-usual) and alternate (decarbonization and/or deep decarbonization) scenarios. The business-as-usual (BAU) scenarios mirror the emission level that will follow counties’ current growth trajectory. There are often no deliberate efforts to reduce emissions in the BAU; thus, CO2 emissions grow for most countries. But, as Ouedraogo [#16] finds, BAU scenarios projections see Global South CO2 emissions increasing and not reaching the Paris Agreement’s net-zero target by 2050, growing between 5 and 12% annually. Thus, Dioha and Kumar [#19] find that in the BAU scenario for Nigeria, the total energy-related CO2 emissions rise from 76 MtCO2 in 2010 to 228 MtCO2 in 2030 and 572 MtCO2 in 2050.
The decarbonization scenarios show the emission reduction trajectory for countries given effort targets up to 2050. The surveyed studies all propose strategies for decarbonization, on the order of 10–70% below current levels or net-zero by 2050. The review revealed varying estimates toward the net-zero target from the analyses of low carbon scenarios. Dioha and Kumar [#19] find that total energy related GHG emissions in Nigeria relative to the BAU scenario will decrease by 27, 37, 46, and 77% for unconditional NDC (UCD), conditional NDC 1 (CD1), conditional NDC 2 (CD2), and emission tax (ETX) scenarios, respectively, by 2050. Similarly, total energy GHG emissions will reduce by 39% (from 398 MtCO2e in 2010 to 241 MtCO2e in 2050), and per capita emissions will decrease by 62% in South Africa [#5]. The model results indicate that the unemployment rate will decrease from 25 to 12%, just as the percentage of people living below the poverty line will decrease from 49 to 18% from 2010 to 2050. In North and Latin America, Martínez-Quintana [#29] finds a 211 MtCO2e reduction in 2030 from the baseline of 972 MtCO2e in Mexico. Idris et al. [#30] find that relative to the unconditional CO2 reduction commitment in the NDC in 2030 and 2050, the CO2 reduction was 79–82% of Malaysia’s total annual GHG emissions in the 2020 to 2030 period (equivalent to 325–450 GtCO2/year).
In summary, some studies find that the implementation will result in achieving the net-zero target before or by 2050 [#5, #8, #9, #12, #21, #22, #23, #28] or net-zero by 2060–2070 [#27]. On the other hand, other studies show that decarbonization scenarios achieve significant emissions reduction relative to the BAU scenario despite not reaching net zero by 2050 [#7, #8, #11, #13, #14, #16, #17, #24, #25, #26, #29, #30, #31]. On the other hand, La Rovere et al. [#10] find that GHG per capita emissions in 2030 will be less than half of those in 2005, and Brazilian NDC targets will be met up to 2030. However, if no additional mitigation strategies are implemented beginning in 2030, total GHG emissions will resume their upward trend, reaching 1,484 Mt CO2e in 2050. Additional mitigation helps reduce Brazilian GHG emissions in the New Brazil 1.5°C scenario, reaching 185 Mt CO2e by 2050, 87% lower than in the GPS scenario.
This sub-section concentrates on the critical multi-level economic implications of energy system decarbonization under review. These include the investment requirements/costs, employment potential and other macroeconomic implications.
16 out of 31 studies (or 52%) of studies under review considered the investment cost of the various decarbonization scenarios (Figure 7). The most common report measure of the costs in the reviewed studies is the US Dollar amount–nine studies [#6, #17, #16, #19, #20, #25, #21, #24, #28]. Others include the decarbonization investment as a share of total investment in the economy–one study, as a share of GDP–three studies [#4, #10, #27], profitability of investment–one study [#23], investment cost by technology, dispatch and operations and maintenance–three studies [#29, #30, #31]. Five of the studies provided more details of the investment requirement and implication as discussed in the preceding part.
Transitioning to a decarbonized energy system necessitates substantial changes in manufacturing methods and consumer preferences. Due to the capital-intensive nature of this change, all economic agents may incur a cost. Adopting innovative technology and resource-intensive methods increases carbon and energy efficiency, but may be more expensive than conventional practices in the short to medium term. Le Treut et al. [#21] find that the required investment to achieve deep decarbonization varies according to country-specific needs and the nature of transition needed. They find that the mean annual additional investment in power generation for Argentina from 2015 to 2050 amounts to between $6–$7 billion (Le Treut et al. [#21]). The study uses two decarbonization scenarios that differ based on technology strategy. Therefore, the investment includes $3.5 billion annually for wind power and solar PV in both deep decarbonization scenarios, $2.4 billion annually for gas-fired power in the deep decarbonization 1 (DD1) scenario and $ 3.7 billion per annum for nuclear plus hydropower in the deep decarbonization 1 (DD2) scenario.
La Rovere et al.‘s [#10] study on Brazil finds that the government plan scenario will require a total investment of $0.42 trillion in 2030 and $0.75 trillion in 2050, equivalent to 15.2% of GDP in 2030 and 15.8% by 2050. The study considered a government plan scenario that assumes the achievement of NDC targets up to 2030, the extension up to 2050, and a deep decarbonization scenario compatible with the global temperature target of 1.5°C. In La Rovere et al. [#7], the projected total investment spending on decarbonization measures would amount to some 1% of Brazil’s GDP by 2030. Mexico’s deep decarbonization scenario will require an additional capital investment of around $200 billion from 2020 to 2050 (almost a quarter of Brazil’s requirement) in generation capacity Buira et al. [#20]. This amounts to about $6.6 billion per annum, like the range for Argentina.
For Nigeria, Dioha and Kumar [#19] find that investments in the BAU are expected to rise at a cumulative average growth rate of 5.3% from $28 billion in 2010 to around $88 billion and $224 billion in 2030 and 2050, respectively. The study has four decarbonization scenarios, namely unconditional NDC (UCD), conditional NDC 1 (CD1), conditional NDC 2 (CD2), and Emission tax (ETX). Compared to BAU, the required investment for the scenarios UCD, CD1, CD2, and ETX grow by around 0, 2, 3, and 17%, respectively. However, by 2050, the investment in the mitigation scenarios will grow slightly slower than the BAU scenarios. This is because the mitigation scenarios reduce the cost of fuel, in the long run, thereby reducing the overall investment requirements. Conversely, Ouedraogo [#16] finds that the transformation costs of the NDC scenario will reach US$ 8 billion in 2030 for Egypt and $ 5 billion for Algeria.
The investment outlay required to achieve decarbonization is enormous, although varying across countries. However, some of such investments are found to pay back themselves. Applying net present value, Kazi et al. [#23] find that the green hydrogen economy will be a profitable option for industrial decarbonization in Qatar. The study also found other investment returns in general revenue by-product of the green hydrogen production (i.e., oxygen from electrolysis). Thus, direct profitability or return on investment is not the only value of decarbonization investment. The environmental impact could improve health; the investment can also have positive implications like new jobs and economic growth.
In Saudi Arabia, Soummane et al.’s [#15] projections show that real investments will grow from 801 billion in 2013 SAR (Saudi Arabia Riyal) to 1,394 billion in 2013 SAR in the BAU scenario between 2013 and 2030. This will result in real investments being approximately 74% higher in 2030 than in 2013. In the first decarbonization scenario, the low oil price scenario, real investment is projected to decline by 12.2% (to 725 billion in 2013 SAR) relative to the baseline scenario. The low oil price is characterized by a depressed global oil market that increases climate action. The second decarbonization scenario analyzed is the reformed scenario in which domestic energy tariffs are increased to match international levels and introduce energy efficiency initiatives. This scenario is projected to decrease real investment by 2.1% (to 808 billion in 2013 SAR) relative to the BAU scenario and 11.6% relative to the low oil price scenario.
A total of 8 out of 31 studies (or 26%) under review considered employment impacts of the various decarbonization scenarios (Figure 8). These articles indicated considerable employment benefits if any economy in the Global South attempts to decarbonize its economy.
The decarbonization strategy has substantial implications for the employment market. The influence is seen in the availability of new jobs in the energy sector. Reddy et al. [#4] find that the renewable energy sector accounted for 8% of India’s total employment in 2010. However, it is found that this will reach 87.82% by 2030 under the business as usual (BAU) scenario and 97% under the green economy (GE) scenario. Thus, the decarbonization strategy will add 10.5% of new jobs to the renewable energy sector in 2030. Solar thermal and solar water heating systems employ 0.76 million people, making them the most important renewable energy technology for employment. Hydroelectric power employs 0.275 million people followed by wind power with 0.243 million. Low-wage workers benefit from the green economy by having more employment opportunities and improving their standard of living over time.
According to Reddy et al. [#4], the renewable energy sector provides more construction, manufacturing, and installation employment per million Rupee investment and megawatt of power than conventional power sources such as coal and natural gas due to the labor-intensive nature of green energy investment. This is because green energy investment has labor-intensive characteristics. Le Treut et al. [#21] also estimate that job creation will increase in the deep decarbonization scenario; between 100,000 and 130,000 jobs will be created by 2050. The construction scenario will concentrate most of the net job creation in the deep decarbonization scenario with an average additional 90,000 to 144,000 FTE of non-relocatable jobs. Expansion of the low carbon capacities will create 4,000 to 5,000 new direct jobs. Also, Musango et al. [#3] find that the GE scenarios for South Africa will generate more jobs than the BAU scenario, which in 2030 will reach 28.347 million people, while the BAU2 generates jobs for 27.853 million people in the same year. In addition to the increase in employment volume. Altieri et al. [#5] find that, from 2010 to 2050, the wages for unskilled and low-skilled workers will rise by 45 and 160%, respectively, and the share of the population living below the poverty line will decrease from 49 to 18%. Total GHG emissions would decline by 39%, while per capita emissions would decrease by 62%. The model relies primarily on agriculture absorbing unskilled workers to reduce unemployment.
According to La Rovere et al. [#10], carbon tax revenue will decrease labor charges, increase job creation, and help keep employment by decarbonizing the economy. Besides, according to Gupta et al. [#12], there will be many financial and strategic losses. However, retraining and job creation for people in carbon-intensive industries could be one of the steps that facilitate a transition to a decarbonized economy. According to Bohlmann et al. [#14], the transition to low carbon energy will reduce employment in coal-producing areas while increasing employment in areas where non-coal electricity generation is expected to flourish. Thus, there is a rise in semi-skilled employment in all areas, except for coal-producing regions. In Saudi Arabia, Soummane et al.‘s [#15] projections show that the unemployment rate will increase to 5.6% in the BAU scenario between 2013 and 2030. The rate increases to 6.2 and 7.0% in the low oil price and reformed scenarios, respectively.
As earlier stated, the transformation and investment cost required to achieve a decarbonized economy could be enormous. Due to global climate mitigation policies, economic activities could suffer significant setbacks (IPCC, 2014). However, changes in major macroeconomic indicators at the national or regional level can be favorable or unfavorable, depending on possible productivity gains, implications on interregional trade, and new investment dynamics (Vrontisi et al., 2020). Our review identified critical macroeconomic indicators (besides investment cost and employment) common among the reviewed studies for brevity. These indicators include GDP growth rate, consumption, debt level, net savings and costs, income and welfare, security (of energy and food), trade balance, imports, and exports. A total of 17 studies (or 55%) under review considered the impacts of the various decarbonization scenarios on major macroeconomic indicators besides investment cost and employment (Table 5).
TABLE 5. Share of studies analyzed selected major macroeconomic indicators (besides investment cost and employment.
In South Africa, Musango et al.’s [#3] findings suggest that positive GDP impacts are expected by 2030. Specifically, real GDP in the green economy scenarios is projected to reach ZAR 2.867 billion in GE2% and ZAR 2.907 billion in GETS scenarios. These exceed the projections in the BAU scenarios, which were projected to be ZAR 2.850 billion in BAU and ZAR 2.879 billion in BAU2%. Furthermore, the study estimates that the projected per capita income ranges between ZAR 46,714 and ZAR 47,352 per person for the Green Economy scenarios. On the other hand, the BAU scenario per capita income projections in 2030 range from ZAR 46,432 to ZAR 46,895 per person. Also, in South Africa, Altieri et al. [#5] estimate that from 2010 to 2050, GDP will increase by more than 200%, averaging a 2.8% annual GDP growth during the period. Industry value added to GDP grows at an average annual rate of 2.7%. Agriculture grows at an average annual rate of 4.9%. Additionally, Bohlmann et al. [#14] project that GDP would be marginally lower, ranging from –0.014 and –0.031 for all scenarios) relative to the BAU scenario. They explain that the marginal decline in GDP stems from the coal exports cap for all scenarios.
In India, Mittal et al.’s [#9] economic modelling results show a 3.2% GDP loss in the 2°C scenario compared to the NDC scenario. In the NDC emission pathway, the mitigation cost in terms of GDP loss rises to 5.3% in 2050. The economic losses increase to 6% of GDP in the year 2050 in the 1.5°C scenario. The authors conclude that targeting the stringent 1.5°C goal would jeopardize India’s economic development. Furthermore, in India, Gupta et al.’s [#12] study suggests that, despite high resource requirements, the low-carbon scenarios can accommodate yearly economic growth of 5.8% from 2013 to 2050, which is on par or marginally higher than the BAU scenario.
Furthermore, they decompose sectoral economic activities and found that by 2030, the GDP shares of industries, agriculture and services decrease in the 2DegMG scenario compared to BAU due to the share of value-added mobilized by energy sectors being higher in the 2DegMG scenario because of the higher capital costs of the technologies required to achieve advanced mitigation targets. By 2050, however, the GDP share of industries also increases under the combined pressure of the increased capital intensity induced by the penetration of energy-efficient techniques. They also find marginal variability in real GDP and substantial changes in the share of trade balance in GDP.
In Gupta et al. [#17], the four scenarios analyzed for India show minor GDP variations, around 0.7% in 2050. Despite significant investment costs, they also find that the low-carbon pathways are compatible with Indian growth. In Malaysia, Colenbrander et al.’s [#6] and Idris et al.’s [#30] results suggest that early mitigation investment in climate change results in an increased economic growth rate. The economic growth rate is also estimated to outpace increases in emissions. In Brazil, Lefèvre et al. [#8] find small, aggregated GDP implications for decarbonization for the 2015–2030 period. The DD strategy induces additional emissions cuts with a minimal GDP loss (−0.14% compared to the GOV scenario) due to an extra carbon tax on the economy. On the other hand, La Rovere et al.’s [#10] results show that by 2030 and 2050, GDP will grow significantly in both GPS (2.75 trillion and 4.72 trillion in 2030 and 2050, respectively) and NB 1.5°C (2.69 trillion and 4.54 trillion in 2030 and 2050, respectively) scenarios. Besides, per capita GDP grows significantly from 7.75 thousand USD in 2005 to 12.06 in 2030 and 20.10 in 2050, respectively. For Argentina, Le Treut et al. [#21] find relatively small GDP implications of DDP scenarios relative to the BAU scenario. The net present value (NPV) of cumulated GDP is between 0.1 and 0.8% lower in the DD1 and DD2 scenarios than in the NDC (BAU) scenario.
In Saudi Arabia, Soummane et al.’s [#15] projections show that in the BAU scenario, the country’s real GDP will increase at an annual average growth rate of 2% between 2013 and 2030. This will result in real GDP being 42% higher in 2030 than in 2013. Real GDP is projected to decline by 1.4% relative to the baseline scenario in the first decarbonization scenario, the low oil price scenario. The second decarbonization scenario analyzed is the reformed scenario in which domestic energy tariffs are increased to match global levels and the introduction of energy efficiency initiatives. This scenario is projected to increase real GDP by 0.6% above the BAU scenario and 2% higher than the low oil scenario.
Gupta et al. [#12] compared the BAU and 2DegMG scenarios by 2050 in India while assuming favorable investment and exchange rate policies. The results show that the low emission pathway offers a 35-GDP point significant reduction in foreign debt by increasing the trade balance at the consumption cost of 1% of GDP while slightly improving activity. In the event of high growth, the low-carbon option achieves close to maintaining foreign debt at its base-year level (+4 GDP points only by 2050). Conversely, the BAU and low-growth low-carbon trajectories lead to dramatic increases in foreign debt (by +90 and +158 GDP points by 2050, respectively) via increased trade deficits. In Argentina, Le Treut et al. [#21] found that national debt increases marginally to 0.6 and 1.6% of GDP due to the DDP scenarios relative to the BAU scenario.
Also, in South Africa, Altieri et al. [#5] project that people living below the poverty line will decrease from 49 to 18% in 2050. Gupta et al. [#12] compare the BAU and 2DegMG scenarios by 2050 while assuming favorable investment and exchange rate policies. The result shows that transitioning from fossil fuel-based energy systems would result in a consumption cost equivalent to 1% of GDP. In Gupta et al. [#17], transitioning from fossil fuel-based energy systems would cause the share of household consumption to decrease by 4.4–5.0 percentage points in 2030 and by 1.6–1.9 percentage points in 2050 in the scenarios. In Brazil, Lefèvre et al. [#8] find small, aggregated GDP implications for decarbonization for the 2015–2030 period. The decarbonization scenario induces significant increases in general price levels due to additional carbon tax on the economy.
In Argentina, Le Treut et al. [#21] find minimal consumption impact due to decreased residential energy consumption and slightly weaker purchasing power in DD scenarios because of increased consumer prices. Additionally, Babatunde et al. [#26] note that by 2050, energy decarbonization would result in a 15% increase in welfare. In Saudi Arabia, Soummane [#15] projects that real private (public) consumption will grow by approximately 74% (38%) between 2013 and 2030 in the BAU scenario. In the low oil price scenario, private consumption increases by 7% (equal to 1,491 billion in 2013 SAR) relative to the BAU scenario, while public consumption declines by 12.2% (equal to 762 billion in 2013 SAR) between 2013 and 2030. Furthermore, in the reformed scenario, private consumption grows by 8% (equal to 1,505 billion in 2013 SAR) relative to the BAU scenario, while public consumption declines by 10.1% (equal to 780 billion in 2013 SAR) between 2013 and 2030. However, private and public consumption will increase by 0.9 and 2.4%, respectively, relative to the low oil price scenario.
In South Africa, Van Zyl et al. [#11] project that the cumulative net savings associated with achieving the CERC “fair share” emissions pathway, assuming the moderate use of low carbon power generation measures, would reach $5.3 billion by 2030. They also state that net savings could reach $46.8 billion by 2030 if power generation moves towards full decarbonization. In Gupta et al. [#17], the four scenarios for India indicate that transitioning from fossil fuel-based energy systems would result in foreign exchange savings to the tune of $1 trillion from 2012 to 2050 for oil imports. Ouedraogo [#16] analyzes the energy and economic impacts of transition pathways for seven North African countries to meet its (intended) nationally determined contributions ((I)NDCs) under the Paris Agreement. Their modeling results reveal negative costs of reduced GHG emissions, suggesting that the overall direct benefits of implementing the (I)NDCs exceed the costs. Thus, applying the (I)NDC scenario supports GHG savings of 1960 MtCO2e at a negative cost of US$ −199 tCO2e in 2030.
In South Africa, Bohlmann et al. [#14] project a decline in coal exports in all scenarios relative to the BAU, further resulting in marginal GDP declines, as pointed out previously. In Brazil, Lefèvre et al.’s [#8] results show that aggregated industry exports and outputs are 1.8 and 1.5% lower, respectively, in the DD than in the GOV scenario, owing to the negative impact of oil revenues on oil revenues and the exchange rate in the long run. They conclude that DD is not a winning scenario for all. Soummane [#15] projects exports to increase by 38.3% in the BAU scenario. Real exports will grow by 0.8% (equal to 2,059 billion in 2013 SAR) relative to the BAU scenario in the low oil price scenario. Furthermore, in the reformed scenario, real exports grow by 0.3% (equal to 2,049 billion in 2013 SAR) relative to the BAU scenario and decline by 0.5% relative to the low oil price scenario between 2013 and 2030.
In South Africa, Altieri et al. [#5] account for energy security; the study finds that demand-side energy poverty decreases as households move into the middle-income category. On the supply side, 99% of households will have electricity connections in 2050. On the other hand, La Rovere et al.’s [#10] results show that by 2030 and 2050, per capita GDP will grow significantly, evolving from 7.75 thousand USD in 2005 to 12.06 in 2030 and 20.10 in 2050, respectively, allowing for both energy and food security. In Argentina, Le Treut et al. [#21] found projects that decreased residential energy consumption linked to increased consumer prices in the DDP scenarios relative to the BAU scenario. In Peru, Ugarte et al. [#25] show that Between 2020 and 2050, the DDP initiatives have the possibility of averting the destruction of 4 million hectares of primary forests.
In contrast, the stock of secondary forests will be reduced by 1.4 million hectares by 2050. The DDP initiatives focus their effects on agriculture and grassland. Due to the measures, the potential deforestation by farmland is decreased by 61%, on average (3.8 million hectares preserved), between 2018 and 2050. In the case of livestock, the influence of the forestry revenues took all incentives to convert forestland into grassland, preventing the conversion of 322 thousand hectares. Furthermore, the reduction in the transformation of primary forests to agriculture suggests a decline in the area harvested for crops; this will result in a significant fall in rice production and consequently an increase in the volume of imports; the latter could be related to a loss of food security.
In India, Gupta et al.’s [#12] study find that there will be substantial changes in the share of trade balance in GDP, by 2050, in the 2DegMG scenario, compared to the BAU scenario. However, the study did not concretely indicate the significant changes. Additionally, the study suggests that there will be an increase in the trade balance, which will help to reduce foreign debt significantly. On the other hand, Gupta et al. [#17] find that low carbon pathways will decrease the deficit in the trade balance by up to 5 percentage points in 2030 and 1.8 percentage points in 2050, compared to BAU. Soummane [#15] projects that trade balance contribution to GDP will decrease from 24.6% in 2013 to 18.1% in 2030 in the BAU scenario. The trade balance in the low oil price scenario will decline by 8.1 percentage points (equal to 10%) relative to the BAU scenario. Furthermore, in the reformed scenario, the trade balance share of GDP decreases by 9.5 percentage points (equal to 8.7%) relative to the BAU scenario and declines by 1.3 percentage points relative to the low oil price scenario between 2013 and 2030.
Finally, Villamor et al. [#22] find that DDP scenarios are challenging but do not compromise socio-economic development in Ecuador.
The discussion of results is separated into three parts. Section 4.1 discusses the major findings, while Section 4.2 Section 4.3 Sections 4.4 outline the lessons learned, the limitations of this review, and the recommendations for further research in the Global South and macroeconomics of energy system decarbonization, respectively.
The study’s main findings are in four-folds, covering emissions reduction targets, investment cost, employment, and other macroeconomic implications. GHG emissions are often linked with economic growth as more advanced economies emit more GHG. However, it was found from the reviewed studies that while retaining economic growth targets, developing countries of the Global South can achieve emission reduction goals but at different periods. It is found that for some counties, a net-zero target can be achieved by 2050 or earlier [#5, #8–#9, #12, #21–#23, #28], for others, it can be achieved by 2060–2070 [#27].
The investment cost required to achieve a decarbonized economy could be enormous. They vary according to country-specific needs and the nature of the transition needed. Argentina will require between $6–$7 billion per annum from 2015 to 2050; Brazil requires $200 billion, Mexico needs up to $750 billion, while Nigeria requires $ 288 billion over 40 years [#10, #19, #20, #21]. However, Kazi et al. [#23] find strong potential for such investment to pay back themselves. In addition to having a positive return, the investment guarantees jobs and strengthens economic growth potential. Also, direct profitability or return on investment is not the only value of decarbonization investment. The environmental impact could improve health; the investment can also have positive implications like new jobs and economic growth.
The emission reduction strategies seem to be compatible with economic growth. Investment in renewable energy positively influences the volume of jobs (inverse effect on unemployment) and the wage structure. Reddy et al. [#4] find an additional 10.5% of new jobs will be added to the renewable energy sector in 2030 due to the decarbonization strategy in India. Altieri et al. [#5] also find that wages for unskilled labor will increase by 45% from 2010 to 2050. Low-skilled labor wages will increase by 160% as the unemployment rate decreases from 25 to 12%.
Economic growth could be positive, as in the case of South Africa, or negative for some scenarios like in India, or negative for all scenarios relative to BAU [#5, #9, #14]. Altieri et al. [#5] found that from 2010 to 2050, GDP will increase by more than 200%, averaging 2.8% annual GDP growth from the period in South Africa, while Mittal et al.’s [#9] economic modeling results show that 3.2% GDP loss occurs in the 2°C scenario compared to the NDC scenario. However, Bohlmann et al. [#14] project that GDP would be marginally lower, ranging from −0.014 and −0.031 for all scenarios relative to the BAU scenario. They explain that the marginal decline in GDP stems from the coal exports cap for all scenarios. The implication is that decarbonization strategies must be carefully formulated according to the economic context and the growth and sustainable development target.
Developing countries in the global south face considerable challenges in setting up energy systems for deep decarbonization. The first set of issues concerns the cost implication of setting up energy system decarbonization. The challenge is even more so given that oil accounts for 60% of total export for at least 20 Global South countries. Most of these countries have a sizable undeveloped but aspirational population, emphasizing the country’s development needs. Thus, energy consumption will undoubtedly increase because of population growth and a desire for socio-economic development (Dioha et al., 2019). Making choices regarding addressing unmet energy requirements while preserving the environment is undoubtedly complex.
To reduce GHG emissions, developing countries must make decisions that would have a long-term impact, such as new technologies with significant socio-economic implications. Thus, decisions must be made with a firm grasp of future energy demand, supply, GHG emissions, and socio-economic implications. This review draws lessons from the reviewed Global South countries’ decarbonization research. While the implications of energy system decarbonization in the reviewed studies vary from one to another, there are common aspects of decarbonization that can be extracted and used as insights to ensure a just energy system decarbonization for developing countries (including fossil fuel-dependent countries).
Decarbonizing the energy system is a lengthy, diverse, and complex process. It poses a risk to the economy for net oil exporters because of its trade balance implication. A net oil importer could also have the challenge of stranded assets to consider. As a result, successful decarbonization entails researching to ascertain the effects on the oil sector and the economy. This review examines the ex-ante evidence of the impacts of decarbonization on GDP, welfare, trade, and employment in a sample of Global South nations. However, it should be regarded as a preliminary study that only addresses the issue’s surface. Additional extensive and in-depth studies on this subject should be conducted to provide country-specific context for what might be faced during decarbonization.
The three main approaches were adopted by studies reviewed, each having a unique value in understanding pathways to deep decarbonization. However, Winkler et al. (2017) assert that models combining bottom-up and top-down models can better address frame technological change, economy-wide interactions, costs, emission reductions, and broader socio-economic implications of mitigation. Deep decarbonization will require technical modifications. These changes have broader socio-economic implications for various economic agents. A granular understanding of the interactions between economic agents is essential. Therefore, a bottom model linked with a top-down model might hold the most promise for the Global South developing countries.
Addressing the rising energy demand requires large-scale renewable energy and energy storage deployment. These will require significant investment, particularly early in the decarbonization agenda. The potential of a country to attract renewable energy investment is a crucial measure of its decarbonization readiness. For most countries, increasing the amount of renewable energy in the energy mix would fulfill both energy security and climate goals. Renewable energy sources attract investment and demonstrate a country’s preparation for the energy revolution. Due to the enormous investments required to create renewable energy, the government cannot simply support them from its budget. Complementary sources of investment, such as private sector investment, are needed.
The labor market will be significantly impacted by decarbonization. As a result, labor market policy should take proactive measures to assist those affected by changes in labor market demand due to energy system decarbonization. Policies should prioritize growing employment opportunities and improving employability and training (International Labour Organization, 2017). Employment services should be established to give displaced workers information about available options, job matching services, and training.
For several Global South countries, oil is an important export commodity and energy source. Thus, the oil phase-out will significantly impact the economy. Development at the national level and in oil-dependent regions depends on oil income, accounting for around 80% of the federal government (including foreign exchange) revenues (CBN, 2019). Due to the oil phase-out associated with the decarbonization goal, the country and its oil-producing regions will confront a liquidity shortage. Simultaneously, the country’s government’s unwillingness to embrace the energy transition will undoubtedly result in future economic troubles as oil’s market value as a commodity and source of energy declines. The transition from oil to renewable energy sources will also require significant finance. The federal and regional governments may establish a specific fund to assist oil-dependent regions in transition as a precautionary measure.
This review study represents one of the first attempts at synthesizing the literature on multi-level economic implications of energy system decarbonization. The first limitation is that this review focused only on ex-post modelling-/scenario-based studies. While this is important for evidence-based climate policymaking, it may leave substantial evidence base on non-modelling-/scenario-based studies. On the one hand, the focus on the Global Southern countries presents high-level evidence that may mask country-specific evidence. On the other hand, it does not provide a global evidence base. However, the focus on the Global South is an initial attempt to synthesize the existing evidence given the dearth of country-based studies focused on the multi-level economic implications of deep decarbonization in developing regions, especially Sub-Saharan Africa.
Much is unknown about how energy system deep decarbonization affects different economic indicators. Consequently, several areas of research need to be given attention. The suggestion for future research is threefold. First, this review focused only on modelling-/scenario-based studies. We believe this decision is vital for evidence-based climate policymaking. However, there is room for future review studies to account for both ex-post analyses and non-modelling-/scenario-based studies and consider reviewing other systematic review studies to synthesize the state-of-the-art knowledge.
Secondly, the current review focused on countries in the Global South, given the dearth of modelling-/scenario-based studies focused on individual countries. Future studies can extend the literature with country-based systematic reviews, given the small but growing body of country-based studies.
Thirdly, from a methodology perspective, modeling studies, we find that the current assessment of macroeconomic impacts in our review exhibits critical gaps because they do not account for the costs of associate climate damages and the substantial co-benefits emanating from reduced negative externalities such as air and noise pollution, among others, which could offset the small economic costs. Thus, future modeling studies should attempt to estimate such net benefits. Also, future modeling studies should decompose investments into those that tend to provide more employment and those that care to reduce emissions and how the two investments can combine to bring the optimum result of emission reduction and job creation. Also, future studies should consider the potential sources of the investment and fiscal space to accommodate the required investments. Additionally, future modeling studies should capture the real-world transition costs in labor and capital markets.
Finally, researchers should focus on developing countries developing long-term low emission development strategies but lack the existing modeling studies for sound policymaking. We believe that any modeling study based on the multi-level economic implications of decarbonization pathways should consider the results of this review as a basis for expanding future quantitative and qualitative studies of the socio-economic implications of energy system decarbonization.
The challenges of achieving ambitious climate targets and sustainable development cannot be solved without the significant efforts of the Global South. Regarding net-zero, the main issue for developing countries is avoiding future emissions from industrialization and lock-in into fossil fuel-intensive technologies. However, strategies toward ambitious climate targets in the Global South depend on the decarbonization of economic sectors and their macroeconomic impacts. A small but growing number of scenario-based studies focused on the macroeconomic impacts of several decarbonization scenarios in the Global South. However, to the best of our knowledge, no study has attempted to synthesize the evidence base of these studies through a focused literature review. To fill this gap, this study examined multi-level macroeconomic implications of decarbonization in selected Global South countries through a systematic literature review of journal publications and gray literature drawn from three databases, specifically, Web of Science, Scopus, and Google Scholar. This study’s inclusion and exclusion criteria produced 31 papers for final review.
The results identified three main themes–investment costs, employment impacts, and other macroeconomic impacts (besides the previous two), including GDP levels, GDP growth rate, consumption rate, debt level, net savings, income and welfare, and trade balance, among others. Overall, the review finds that energy system decarbonization requires substantial investment outlay. Deep decarbonization will require strategic investment in the energy transition. Most countries will require an investment of up to $174 billion over 30 years. For some, the needed investment could go as high as US$450 billion. Apart from the fact that these investments will lead to economic gains beyond climate mitigation, the opportunity cost of allowing the climate to continue deteriorating, if estimated, is likely to be a far higher financial cost of mitigation.
Also, it is linked with job displacement in fossil energy sectors and job creation in green sectors. However, there is no unanimous evidence on net job creation. Additionally, the GDP impacts are ambiguous; some studies find future GDP growth impacts, while others find GDP decline impacts of decarbonization scenarios. The studies reviewed show that decarbonization can be compatible with the economic growth objectives of the Global South developing countries. The strategies to achieve emission reduction while growing the economy will require investment but so will economic growth plans without deep decarbonization pathways. Moreover, the additional jobs, economic growth potential, and environmental benefits that can come from such investment are all incentives for countries of the Global South to prioritize. However, in doing this, careful formulation of the deep decarbonization strategies is essential to optimize net gains while eliminating (or limiting) potential economic losses.
The key contribution of this study is that it is, to the best of our knowledge, the first to review the state-of-the-art in the literature on the multi-level macroeconomic implications of decarbonization strategies in the Global South countries, with a focus on the investment costs, employment impacts, economic growth, among other macroeconomic implications. This study is necessary to provide a broader perspective on the macroeconomic implications of decarbonization planning and policy aspects in developing countries. Recommendations are provided for future studies, particularly from a modeling perspective. A bottom-up model coupled with a hybrid top-down model holds more promise to answer the economic interaction questions of most global south countries. The key lessons from this review that will inform deep decarbonization (DD) modeling analysis for fossil fuel-based economies are fivefold. Firstly, a DD analysis for developing countries must cover wide-ranging implications of energy system decarbonization on the overall economy and oil industry while building on the results of this review. Secondly, a DD analysis must consider the implication for net green jobs and economic diversification. Third, a DD analysis must consider sources of finance for investment in decarbonization strategies such as renewable energy technologies and energy efficiency. Fourthly, the active labor market, skill development, and social protection policies that create just transition are key considerations. Lastly, the potential for national and regional energy transition funds should be accounted for.
CE, CO, UN, NE, OD, and EA contributed to the conception and design of the study. CE, UN, NE, OD, and EA organized the database. CE performed the statistical analysis. CE and UN wrote the first draft of the manuscript. CE, CO, UN, NE, OD, and EA wrote sections of the manuscript. All authors contributed to manuscript revision and read and approved the submitted version.
The authors declare that the research was conducted in the absence of any commercial or financial relationships that could be construed as a potential conflict of interest.
All claims expressed in this article are solely those of the authors and do not necessarily represent those of their affiliated organizations, or those of the publisher, the editors and the reviewers. Any product that may be evaluated in this article, or claim that may be made by its manufacturer, is not guaranteed or endorsed by the publisher.
1OECD stands for Organization for Economic Cooperation and Development
2See https://unfccc.int/sites/default/files/resource/Nigeria_LTS1.pdf
3https://www.bloomberg.com/news/articles/2022-02-11/when-will-china-be-the-world-s-biggest-economy-maybe-never
4See WoS information here: https://clarivate.com/webofsciencegroup/solutions/web-of-science-core-collection/
5See Scopus information here: https://service.elsevier.com/app/answers/detail/a_id/11274/supporthub/scopus/
Altieri, K. E., Trollip, H., Caetano, T., Hughes, A., Merven, B., and Winkler, H. (2016). Achieving development and mitigation objectives through a decarbonization development pathway in South Africa. Clim. Policy 16, S78–S91. doi:10.1080/14693062.2016.1150250
Babatunde, K. A., Said, F. F., Nor, N. G. M., Begum, R. A., and Mahmoud, M. A. (2021). Coherent or conflicting? Assessing natural gas subsidy and energy efficiency policy interactions amid CO2 emissions reduction in Malaysia electricity sector. J. Clean. Prod. 279, 123374. doi:10.1016/j.jclepro.2020.123374
Ben Jebli, M., and Kahia, M. (2020). The interdependence between CO2 emissions, economic growth, renewable and non-renewable energies, and service development: evidence from 65 countries. Clim. Change 162 (2), 193–212. doi:10.1007/s10584-020-02773-8
Bhattarai, U., Maraseni, T., and Apan, A. (2022). Assay of renewable energy transition: a systematic literature review. Sci. Total Environ. 833, 155159. doi:10.1016/j.scitotenv.2022.155159
Bhuiyan, M. A., Zhang, Q., Khare, V., Mikhaylov, A., Pinter, G., and Huang, X. (2022). Renewable energy consumption and economic growth nexus—a systematic literature review. Front. Environ. Sci. 10, 1–21. doi:10.3389/fenvs.2022.878394
Bohlmann, H. R., Horridge, J. M., Inglesi-Lotz, R., Roos, E. L., and Stander, L. (2019). Regional employment and economic growth effects of South Africa’s transition to low-carbon energy supply mix. Energy Policy 128, 830–837. doi:10.1016/j.enpol.2019.01.065
Buira, D., Tovilla, J., Farbes, J., Jones, R., Haley, B., and Gastelum, D. (2021). A whole-economy deep decarbonization pathway for Mexico. Energy Strategy Rev. 33, 100578. doi:10.1016/j.esr.2020.100578
CBN (2019). Central bank of Nigeria annual statistical bulletin. Available at: https://www.cbn.gov.ng/documents/Statbulletin.asp (Accessed November 15, 2021).
Colenbrander, S., Gouldson, A., Sudmant, A. H., Papargyropoulou, E., Chau, L. W., and Ho, C. S. (2016). Exploring the economic case for early investment in climate change mitigation in middle-income countries: a case study of johor bahru, Malaysia. Clim. Dev. 8 (4), 351–364. doi:10.1080/17565529.2015.1040367
Delgado, R., Wild, T. B., Arguello, R., Clarke, L., and Romero, G. (2020). Options for Colombia’s mid-century deep decarbonization strategy. Energy Strategy Rev. 32, 100525. doi:10.1016/j.esr.2020.100525
Dioha, M. O., and Kumar, A. (2020). Exploring the energy system impacts of Nigeria’s Nationally Determined Contributions and low-carbon transition to mid-century. Energy Policy 144, 111703. doi:10.1016/j.enpol.2020.111703
Dioha, M. O., Emodi, N. V., and Dioha, E. C. (2019). Pathways for low carbon Nigeria in 2050 by using NECAL2050. Renew. Energy Focus 29, 63–77. doi:10.1016/j.ref.2019.02.004
Emenekwe, C. C., Onyeneke, R. U., and Nwajiuba, C. U. (2021). Financial development and carbon emissions in sub - saharan Africa. Environ. Sci. Pollut. Res. 29, 19624–19641. doi:10.1007/s11356-021-17161-7
Emodi, N. V., Emodi, C. C., Murthy, G. P., and Emodi, A. S. A. (2017). Energy policy for low carbon development in Nigeria: a LEAP model application. Renew. Sustain. Energy Rev. 68, 247–261. doi:10.1016/j.rser.2016.09.118
Emodi, N. V., Chaiechi, T., and Beg, A. B. M. R. A. (2019). The impact of climate variability and change on the energy system: a systematic scoping review. Sci. Total Environ. 676, 545–563. doi:10.1016/j.scitotenv.2019.04.294
Friedlingstein, P., Jones, M. W., O’Sullivan, M., Andrew, R. M., Bakker, D. C. E., Hauck, J., et al. (2022). Global carbon budget 2021. Earth Syst. Sci. Data 14 (4), 1917–2005. doi:10.5194/essd-14-1917-2022
Fuhr, H. (2021). The rise of the Global South and the rise in carbon emissions. Third World Q. 42 (11), 2724–2746. doi:10.1080/01436597.2021.1954901
Grande-Acosta, G., and Islas-Samperio, J. (2017). Towards a low-carbon electric power system in Mexico. Energy Sustain. Dev. 37, 99–109. doi:10.1016/j.esd.2017.02.001
Gupta, D., Ghersi, F., Vishwanathan, S. S., and Garg, A. (2019). Achieving sustainable development in India along low carbon pathways: macroeconomic assessment. World Dev. 123, 104623. doi:10.1016/j.worlddev.2019.104623
Gupta, D., Ghersi, F., Vishwanathan, S. S., and Garg, A. (2020). Macroeconomic assessment of India’s development and mitigation pathways. Clim. Policy 20 (7), 779–799. doi:10.1080/14693062.2019.1648235
Hasanov, F. J., Hunt, L. C., and Mikayilov, J. I. (2021a). Estimating different order polynomial logarithmic environmental Kuznets curves. Environ. Sci. Pollut. Res. 28 (31), 41965–41987. doi:10.1007/s11356-021-13463-y
Hasanov, F. J., Khan, Z., Hussain, M., and Tufail, M. (2021b). Theoretical framework for the carbon emissions effects of technological progress and renewable energy consumption. Sustain. Dev. 29, 810–822. doi:10.1002/sd.2175
Hickman, R., Ashiru, O., and Banister, D. (2011). Transitions to low carbon transport futures: strategic conversations from london and Delhi. J. Transp. Geogr. 19 (6), 1553–1562. doi:10.1016/j.jtrangeo.2011.03.013
Idris, M. N., Hashim, H., Leduc, S., Yowargana, P., Kraxner, F., and Woon, K. S. (2021). Deploying bioenergy for decarbonizing Malaysian energy sectors and alleviating renewable energy poverty. Energy 232, 120967. doi:10.1016/j.energy.2021.120967
Idris, M. N., Leduc, S., Yowargana, P., Hashim, H., and Kraxner, F. (2021). Spatio-temporal assessment of the impact of intensive palm oil-based bioenergy deployment on cross-sectoral energy decarbonization. Appl. Energy 285, 116460. doi:10.1016/j.apenergy.2021.116460
IEA (2021a). Global energy and CO2 emissions in 2020. Availabe at: https://www.iea.org/reports/global-energy-review-2020/global-energy-and-co2-emissions-in-2020 (Accessed December 20, 2021).
IEA (2021b). Net zero by 2050: a roadmap for the global energy sector. Paris, France: International Energy Agency, 1–224. Availabe at: www.iea.org.
International Labour Organization (2017). How to measure and model social and employment outcomes of climate and sustainable development policies. Geneva, Switzerland: Green Jobs Assessment Institutions Network.
IPCC (2014). Climate change 2014: synthesis report. Contribution of working groups I, II and III to the fifth assessment Report of the intergovernmental Panel on climate change. Editors R. K. Pachauri, and L. Meyer, Core-Writing-Team (Geneva, Switzerland: Intergovernmental Panel on Climate Change IPCC).
IPCC (2018). “Summary for policymakers,” in Global Warming of 1.5°C. An IPCC Special Report on the impacts of global warming of 1.5°C above pre-industrial levels and related global greenhouse gas emission pathways, in the context of strengthening the global response to the threat of climate change. Editors V. Masson-Delmotte, P. Zhai, H.-O. Pörtner, D. Roberts, J. Skea, P. R. Shuklaet al. (Cambridge, United Kingdom: Cambridge University Press), 3–24. doi:10.1017/9781009157940.001
Irfan, M., Elavarasan, R. M., Ahmad, M., Mohsin, M., Dagar, V., and Hao, Y. (2022). Prioritizing and overcoming biomass energy barriers: application of AHP and G-TOPSIS approaches. Technol. Forecast. Soc. Change 177, 121524. doi:10.1016/j.techfore.2022.121524
Islam, M. M., Khan, M. K., Tareque, M., Jehan, N., and Dagar, V. (2021). Impact of globalization, foreign direct investment, and energy consumption on CO2 emissions in Bangladesh: does institutional quality matter? Environ. Sci. Pollut. Res. 28 (35), 48851–48871. doi:10.1007/s11356-021-13441-4
Islas-Samperio, J. M., Manzini, F., and Grande-Acosta, G. K. (2019). Toward a low-carbon transport sector in Mexico. Energies 13 (1), 84. doi:10.3390/en13010084
Johnstone, P., Rogge, K. S., Kivimaa, P., Fratini, C. F., Primmer, E., and Stirling, A. (2020). Waves of disruption in clean energy transitions: sociotechnical dimensions of system disruption in Germany and the United Kingdom. ENERGY Res. Soc. Sci. 59, 101287. doi:10.1016/j.erss.2019.101287
Kazi, M. K., Eljack, F., El-Halwagi, M. M., and Haouari, M. (2021). Green hydrogen for industrial sector decarbonization: costs and impacts on hydrogen economy in Qatar. Comput. Chem. Eng. 145, 107144. doi:10.1016/j.compchemeng.2020.107144
La Rovere, E. L., Gesteira, C., Grottera, C., and Wills, W. (2016). “Pathways to a low carbon economy in Brazil,” in Brazil in the anthropocene: conflicts between predatory development and environmental policies (New York, NY: Routledge/Taylor & Francis), 242–266. doi:10.4324/9781315544069
La Rovere, E. L., Grottera, C., and Wills, W. (2018). Overcoming the financial barrier to a low emission development strategy in Brazil. Int. Econ. 155, 61–68. doi:10.1016/j.inteco.2017.12.004
Lallana, F., Bravo, G., Le Treut, G., Lefèvre, J., Nadal, G., and Di Sbroiavacca, N. (2021). Exploring deep decarbonization pathways for Argentina. Energy Strategy Rev. 36, 100670. doi:10.1016/j.esr.2021.100670
Le Treut, G., Lefèvre, J., Lallana, F., and Bravo, G. (2021). The multi-level economic impacts of deep decarbonization strategies for the energy system. Energy Policy 156, 112423. doi:10.1016/j.enpol.2021.112423
Lefèvre, J., Wills, W., and Hourcade, J. C. (2018). Combining low-carbon economic development and oil exploration in Brazil? An energy–economy assessment. Clim. Policy 18 (10), 1286–1295. doi:10.1080/14693062.2018.1431198
Lopez, G., Aghahosseini, A., Bogdanov, D., Mensah, T. N. O., Ghorbani, N., Caldera, U., et al. (2021). Pathway to a fully sustainable energy system for Bolivia across power, heat, and transport sectors by 2050. J. Clean. Prod. 293, 126195. doi:10.1016/j.jclepro.2021.126195
López-Menéndez, A. J., Pérez, R., and Moreno, B. (2014). Environmental costs and renewable energy: re-Visiting the environmental Kuznets curve. J. Environ. Manag. 145, 368–373. doi:10.1016/j.jenvman.2014.07.017
Martínez-Quintana, M. A., Martín-del-Campo, C., and Cruz-Mendoza, G. (2021). A power optimization model for the long-term planning scenarios: case study of Mexico’s power system decarbonization. Can. J. Chem. Eng. 99 (4), 884–897. doi:10.1002/cjce.23986
Mittal, S., Liu, J. Y., Fujimori, S., and Shukla, P. R. (2018). An assessment of near-to-mid-term economic impacts and energy transitions under “2°C” and “1.5 °C” scenarios for India. ENERGIES 11 (9), 2213. doi:10.3390/en11092213
Musango, J. K., Brent, A. C., and Bassi, A. M. (2014). Modelling the transition towards a green economy in South Africa. Technol. Forecast. Soc. Change 87, 257–273. doi:10.1016/j.techfore.2013.12.022
Ouedraogo, N. S. (2017). Africa energy future: alternative scenarios and their implications for sustainable development strategies. Energy Policy 106, 457–471. doi:10.1016/j.enpol.2017.03.021
Ouedraogo, N. S. (2020). Transition pathways for North Africa to meet its (intended) nationally determined contributions ((I)NDCs) under the Paris agreement: a model-based assessment. Clim. Policy 20 (1), 71–94. doi:10.1080/14693062.2019.1685449
Page, M. J., McKenzie, J. E., Bossuyt, P. M., Boutron, I., Hoffmann, T. C., Mulrow, C. D., et al. (2021). The PRISMA 2020 statement:an updated guideline for reporting systematic reviews. BMJ 372, n71. doi:10.1136/bmj.n71
Phdungsilp, A. (2010). Integrated energy and carbon modeling with a decision support system: policy scenarios for low-carbon city development in Bangkok. Energy Policy 38 (9), 4808–4817. doi:10.1016/j.enpol.2009.10.026
Reddy, B. S. (2014). India’s energy system transition - survival of the greenest. Renew. Energy 92, 293–302. doi:10.1016/j.renene.2016.02.027
Rehman, A., Ma, H., Ozturk, I., Murshed, M., and Dagar, V. (2021). The dynamic impacts of CO2 emissions from different sources on Pakistan’s economic progress: a roadmap to sustainable development. Environ. Dev. Sustain. 23 (12), 17857–17880. doi:10.1007/s10668-021-01418-9
Schers, J. (2018). Economic growth, unemployment and skills in South Africa : an Analysis of different recycling schemes of carbon tax revenue. Paris, France: Economics and Finance Université Paris saclay COmUE. NNT: 2018SACLA039f. Available at: https://tel.archives-ouvertes.fr/tel-02293182/document (Accessed December 21, 2021).
Shaffril, H. A. M., Krauss, S. E., and Samsuddin, S. F. (2018). A systematic review on Asian’s farmers’ adaptation practices towards climate change. Sci. Total Environ. 644, 683–695. doi:10.1016/j.scitotenv.2018.06.349
Simsek, Y., Sahin, H., Lorca, Á., Santika, W. G., Urmee, T., and Escobar, R. (2020). Comparison of energy scenario alternatives for Chile: towards low-carbon energy transition by 2030. Energy 206, 118021. doi:10.1016/j.energy.2020.118021
Soummane, S., Ghersi, F., and Lefevre, J. (2019). Macroeconomic pathways of the Saudi economy: the challenge of global mitigation action versus the opportunity of national energy reforms. Energy Policy 130, 263–282. doi:10.1016/j.enpol.2019.03.062
Su, X., Ghersi, F., Teng, F., Le Treut, G., and Liang, M. (2021). The economic impact of a deep decarbonisation pathway for China: a hybrid model analysis through bottom-up and top-down linking. Mitig. Adapt. Strateg. Glob. Chang. 27 (1), 11. doi:10.1007/s11027-021-09979-w
Tang, C., Irfan, M., Razzaq, A., and Dagar, V. (2022). Natural resources and financial development: role of business regulations in testing the resource-curse hypothesis in ASEAN countries. Resour. Policy 76, 102612. doi:10.1016/j.resourpol.2022.102612
Ugarte, D. D. L. T., Collado, M., Requejo, F., Gomez, X., and Heros, C. (2021). A deep decarbonization pathway for Peru’s rainforest. Energy Strategy Rev. 36, 100675. doi:10.1016/j.esr.2021.100675
UNFCC (2021). Nationally determined contributions under the Paris agreement: Synthesis report by the secretariat. Available at: https://unfccc.int/sites/default/files/resource/cma2021_08E.pdf (Accessed March 3, 2021).
UNFCCC (2022). The Paris agreement: communication of long-term strategies. Available at: https://unfccc.int/process/the-paris-agreement/long-term-strategies (Accessed March 3, 2021).
Van Zyl, H., Lewis, Y., Kinghorn, J., and Reeler, J. (2018). The cost of achieving South Africa’s ‘fair share’ of global climate change mitigation. Clim. Policy 18 (10), 1327–1339. doi:10.1080/14693062.2018.1437019
Villamar, D., Soria, R., Rochedo, P., Szklo, A., Imperio, M., Carvajal, P., et al. (2021). Long-term deep decarbonisation pathways for Ecuador: insights from an integrated assessment model. Energy Strategy Rev. 35, 100637. doi:10.1016/j.esr.2021.100637
Vrontisi, Z., Fragkiadakis, K., Kannavou, M., and Capros, P. (2020). Energy system transition and macroeconomic impacts of a European decarbonization action towards a below 2°C climate stabilization. Clim. CHANGE 162 (4), 1857–1875. doi:10.1007/s10584-019-02440-7
Wang, R., Assenova, V. A., and Hertwich, E. G. (2021). Energy system decarbonization and productivity gains reduced the coupling of CO2 emissions and economic growth in 73 countries between 1970 and 2016. One Earth 4, 1614–1624. doi:10.1016/j.oneear.2021.10.010
Winkler, H., Delgado, R., Palma-Behnke, R., Pereira, A., Vásquez Baos, T., Moyo, A., et al. (2017). Information for a developmental approach to mitigation: linking sectoral and economy-wide models for Brazil, Chile, Colombia, Peru and South Africa. Clim. Dev. 9 (6), 559–570. doi:10.1080/17565529.2016.1174660
Xie, M., Irfan, M., Razzaq, A., and Dagar, V. (2022). Forest and mineral volatility and economic performance: evidence from frequency domain causality approach for global data. Resour. Policy 76, 102685. doi:10.1016/j.resourpol.2022.102685
York, R., Rosa, E. A., and Dietz, T. (2003). STIRPAT, IPAT and ImPACT: analytic tools for unpacking the driving forces of environmental impacts. Ecol. Econ. 46, 351–365. doi:10.1016/S0921-8009(03)00188-5
Younis, A., Benders, R., Delgado, R., Lap, T., Gonzalez-Salazar, M., Cadena, A., et al. (2021). System analysis of the bio-based economy in Colombia: a bottom-up energy system model and scenario analysis. Biofuel. Bioprod. Biorefin. 15 (2), 481–501. doi:10.1002/bbb.2167
Deb Debt (including foreign and national, and budget balance)
Exp Exports
GDP Gross domestic product
Other Overall benefits
Sav National savings, foreign exchange
Sec Food, energy, forest land
Trd Trade balance
Wel Welfare (income, poverty, inflation, public/private consumption)
Keywords: deep decarbonization, long-term climate strategies, macroeconomic effects, systematic literature review, global south, Nigeria
Citation: Emenekwe CC, Okereke C, Nnamani UA, Emodi NV, Diemuodeke OE and Anieze EE (2022) Macroeconomics of decarbonization strategies of selected global south countries: A systematic review. Front. Environ. Sci. 10:938017. doi: 10.3389/fenvs.2022.938017
Received: 06 May 2022; Accepted: 19 July 2022;
Published: 23 August 2022.
Edited by:
Fakhri J. Hasanov, King Abdullah Petroleum Studies and Research Center (KAPSARC), Saudi ArabiaReviewed by:
Javier Bustos-Salvagno, Universidad del Desarrollo, ChileCopyright © 2022 Emenekwe, Okereke, Nnamani, Emodi, Diemuodeke and Anieze. This is an open-access article distributed under the terms of the Creative Commons Attribution License (CC BY). The use, distribution or reproduction in other forums is permitted, provided the original author(s) and the copyright owner(s) are credited and that the original publication in this journal is cited, in accordance with accepted academic practice. No use, distribution or reproduction is permitted which does not comply with these terms.
*Correspondence: Chukwuemeka Chinonso Emenekwe, Y2h1a3d1ZW1la2EuZW1lbmVrd2VAZnVuYWkuZWR1Lm5n
Disclaimer: All claims expressed in this article are solely those of the authors and do not necessarily represent those of their affiliated organizations, or those of the publisher, the editors and the reviewers. Any product that may be evaluated in this article or claim that may be made by its manufacturer is not guaranteed or endorsed by the publisher.
Research integrity at Frontiers
Learn more about the work of our research integrity team to safeguard the quality of each article we publish.