- 1Chair of Soil Physics, University Bayreuth, Bayreuth, Germany
- 2Research Area 1 “Landscape Functioning”, Leibniz Center for Agricultural Landscape Research (ZALF), Müncheberg, Germany
Climate scenarios predict more frequent and longer drought periods, potentially threatening agricultural yield. The water holding capacity of soils is crucial in controlling drought stress intensity for plants. Recently, amorphous silica was suggested to increase soil water holding capacity and availability. The objective of this study was to explore the potential impact of Si application to soils on the retention and flow of water in soils and their consequence on plant access to water under soil drying conditions. Two sandy soils were mixed with varying contents (0, 1 and 5% g/g) of some selected ASi amendments. The soil water retention and soil hydraulic conductivity were determined using evaporation measurement device implemented in a commercial device called HYPROP. For both soils, an application of ASi at rates of 1 or 5% increased the water holding capacity and soils treated with ASi maintained a higher hydraulic conductivity under soil drying conditions than the control soil. Simulation demonstrated that soils treated with ASi could longer sustain the transpirational demand of plants during a soil drying cycle. These first results confirm expected positive crop-growth effect of silica amendments on hydraulic properties of coarse-textured soils mainly by longer keeping up capillary flow during water extraction by plant roots.
Introduction
Ecosystem performance of terrestrial systems and crop production can be reduced by drought due to a reduction in plant available soil water (Michaelian et al., 2011; Fahad et al., 2017). On the continental and global scale, drought risks are suggested to threaten agricultural yield and ecosystem performance in the future due to climate change (Lehner et al., 2006; Allen et al., 2010; IPCC 2013). Water storage decreases during longer drought periods to a critical value at which water is no longer available for plants, initiating severe drought stress and wilting (Anjum et al., 2011). The water retention curve (WRC) describes the capacity of soils to hold water at different soil matric potentials (Saxton and Rawls 2006). Hence, the WRC is a key function controlling the plant available water content in soils. Storage of water within soil pores mainly depends on the pore size distribution of soils and the specific surface area of the soil particles.
Several soil amendments have been proposed to improve hydraulic properties of soil and ultimately plant access to water under soil drying conditions. Among different amendments, the amorphous silica (ASi, which is non-crystalline silicon dioxide) was recently shown to play an import role in improving soil water holding capacity (WHC) and plant available water in coarse textured soils treated with different ASi contents (Schaller et al., 2020a; Schaller et al., 2020b). These observations were attributed to high surface area, adsorption potential and internal porosity of the ASi particles (Schaller et al., 2020a). In line with these laboratory observations, an increased soil moisture during the whole growing season of wheat was reported in field trials for a sandy soil treated with ASi as potential soil amendment (Schaller et al., 2021b).
In addition to improve water holding capacity of soils, the application of ASi in soils was shown to impact hydraulic conductivity of coarse textured soils (Schaller et al., 2020a). As soil dries its hydraulic conductivities drops by several orders of magnitudes and may limit plant access to water (Gardner 1960). Application of ASi in soils was shown to maintain a higher hydraulic conductivity in coarse textured soil under drying conditions. The coarse textured soils mixed with different ASi contents shown an decreased hydraulic conductivity at saturation and an increased hydraulic conductivity under drying conditions compared to control soils (Schaller et al., 2020a).
Agricultural practices were shown to decrease the content of ASi in soils (Struyf et al., 2010; Vandevenne et al., 2012; Carey and Fulweiler 2016) due to yearly extractions of ASi by crop harvest (Vandevenne et al., 2012; Puppe et al., 2021), as many crop plants are Si accumulators (Katz et al., 2021). The natural ASi pool in soils includes phytogenic, zoogenic, microbial, and protozoic Si fractions (Sommer et al., 2006; Puppe et al., 2015). The phytogenic ASi pool consists of phytoliths and other amorphous forms of silica (Schaller et al., 2021a). This ASi is cycled in the soil by littler fall and litter decomposition. The ASi concentration in soils is between 0 and 6% in soils, with agricultural soils being limited to ASi concentration of 0–1% (Schaller et al., 2021a). Hence, agricultural soil are depleted in ASi with potential effects for water holding capacity, available water and hydraulic conductivity.
So far, the effects of ASi on water holding capacity, available water and hydraulic conductivity were only shown for one type of ASi-amendments and two soils (pure sand and a sandy clay loam). On the other side, the ultimate effect of ASi on plant access to water under drying conditions is still unclear. The objective of this study was to explore the potential impact of different ASi-fertilizer on the retention and flow of water in soils and their consequence on plant access to water under soil drying conditions. Additionally, we used model simulations to test if soils treated with ASi could longer sustain the transpirational demand of plants during a soil drying cycle. Our hypotheses were that (i) ASi increases the water holding capacity of soils and (ii) soils treated with ASi maintain a higher hydraulic conductivity under soil drying conditions for longer period of time than the control soil.
Materials and methods
Experimental design and analysis
A carbon-free pure quartz sand and sandy soil collected from a depth of 0–25 cm (Ap1 horizon) of ZALF experimental station Müncheberg (Germany) were selected to determine the effect of different ASi amendments on the retention and flow of water within the soil. The quartz sand had a particle size ranging between 100 and 200 μm, a pH of 5.7 (measured in CaCl2 solution), an electric conductivity of 4 μS cm−1, with no other mineral or organic matter. The sandy soil consisted of 74% sand, 21% silt and 5% clay, a pH of 5.3 (measured in CaCl2 solution), an electric conductivity of 20.5 μS cm−1 and 2.4% organic matter.
The air-dried soils were mixed with varying commercial Si amendments at rates of 0, 1, or 5% (weight). Table 1 shows the Si amendments used in this study and their specific surfaces area and their abbreviations throughout the text. The pure quartz sand was treated with ASi amendments of Sip50, Sip310, Zeo600, and CBK and the sandy soils with Sip50, Zeo600, CBK and Aerosil300. The pure quartz sand was not analyzed for the effect of Aerosil300 as this was already published (Schaller et al., 2020a). As Sipernat50 and Sipernat310 had a comparable effect on soil water holding capacity and conductivity, we used only Sipernat50 for analysis using the sandy soil. The soil mixture was prepared by mixing air-dried soils with different amendments.
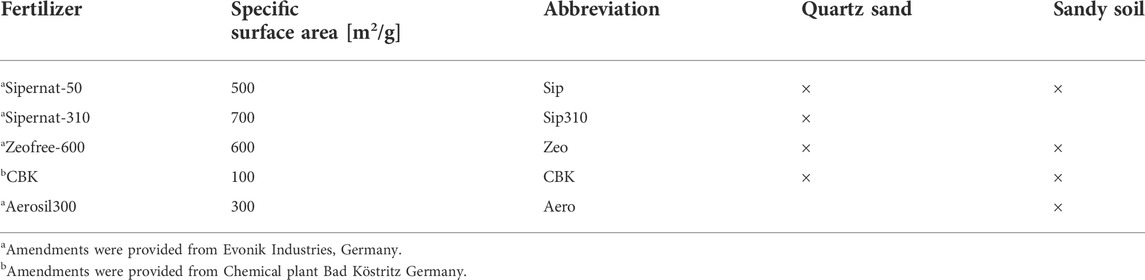
TABLE 1. List of the selected artificial Si amendments used in this study. These amendments were mixed with pure quartz sand and sandy soil.
Quantification of ASi amendments effects on soil hydraulic properties
The effect of additions of some selected Si amendments on water-related properties of soil, such as soil retention and soil hydraulic conductivity curves, was determined using an evaporation measurement device implemented in a commercial device called HYPROP (Meter Group, Germany) combined with the measurements of saturated hydraulic conductivity using a commercial device called Ksat (Meter Group, Germany). In brief, a soil cylinder 250 ml was filled uniformly with the prepared soil mixtures and pre-saturated by capillary rise for 48 h. Then, the saturated hydraulic conductivity of the soil was determined by Ksat using the falling head method. Same soil samples were analyzed with the HYPROP device by imposing an evaporation flux at the surface and monitoring the average soil water content and soil matric potentials at two locations (with intervals of 2.5 cm). This information was used to fit the soil water retention and hydraulic conductivity curves (Peters et al., 2015) according to the Peters–Durner–Iden (VG-PDI) model (Iden and Durner 2014) implemented in the program “Hyprop Fit”. The effect of Si on soil water availability for plants was quantified first by the conventional plant available water (
where
Modeling of Si amendments impacts on soil-plant-water relations
The potential impacts of Si amendments on soil-plant water relations were analyzed by solving water flow equation derived from the combination of the Darcy equation with the mass conservation principle (i.e., known as the Richards’ equation). Assuming that soil water flow towards a single root is radial symmetric, the Richards’ equation in radial coordinate (Van Lier et al., 2006) can be written as:
where
Model parameterization
Root water uptake was modeled assuming that the root and soil are concentric cylinders of radii
where
where
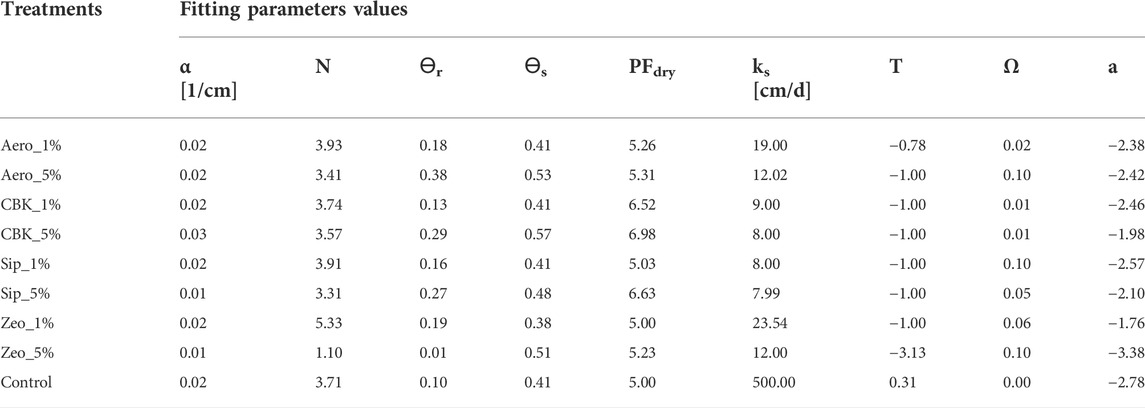
TABLE 2. The Peters–Durner–Iden (PDI) model parameters describing retention curve and hydraulic conductivity curve of sandy soil treated with different ASi contents.
Results
Silica amendments strongly increase the soil water holding capacity
The Hyprop-fitted soil water retention curves showed that ASi-amendments increased the water content at soil saturated conditions (Figure 1; Supplementary Figure S1 as supplementary information). The fitted PDI model parameters are given in Table 1. The sandy soil had an initial saturated water holding capacity of 0.41 cm3/cm3 (control without adding Si amendments). The water content at saturation increased due to addition of 5% ASi amendments (for 5% Aero-to 0.53 cm3/cm3, 5% CBK to 0.56 cm3/cm3, 5% Sip to 0.49 cm3/cm3, and 5% Zeo to 0.51 cm3/cm3). However, the addition of 1% of these amendments did not change saturated water content. At matric potential values lower than -80 cm, every Si-amendments at any concentration increased the water content of soil compared to the control. Similar effects were observed in pure quartz sand for the tested Si- amendments (Supplementary Figure S1 as supplementary information).
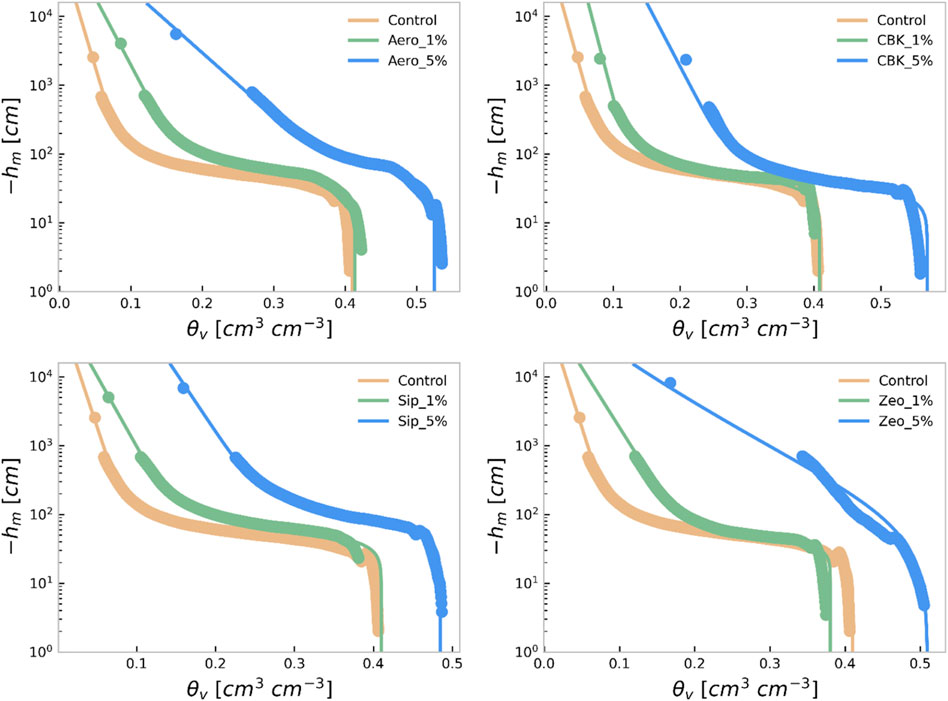
FIGURE 1. Different ASi amendments increase soil water holding capacity of sandy soil. The dots are the measured retention curves, and the lines are the estimated retention curves based on the PDI model. Under soil drying conditions, different ASi amendments increased soil water content (
The effect of ASi on water availability for plants was evaluated by estimating the plant available water (
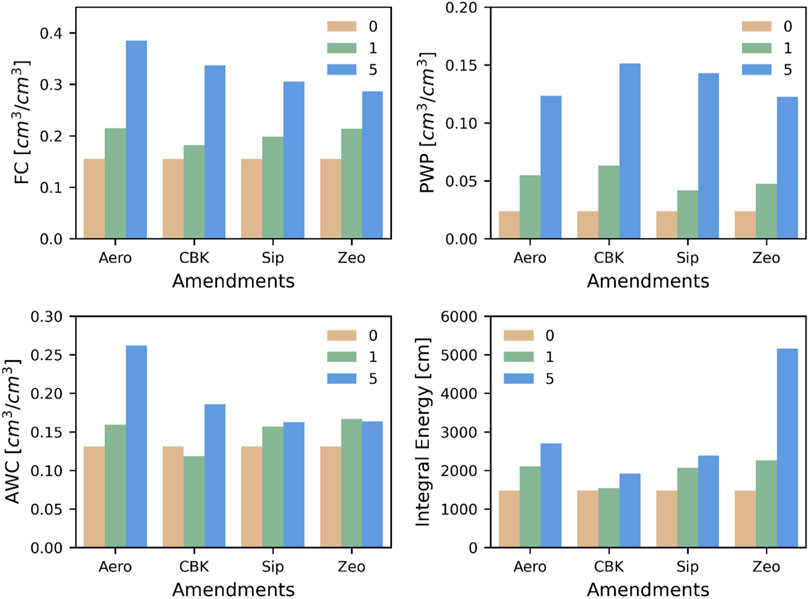
FIGURE 2. Different ASi amendments increase field capacity (FC), permanent wilting point (PWP), available water (AW) and integral energy of sandy soil. This data was extracted from the fitted soil retention curves (Figure 1).
The effect of ASi on water availability for plants was also evaluated by estimating the integral energy (
Silica amendments improve soil water flow
The estimated soil hydraulic conductivity curves of sandy soil and pure quartz sand are shown in Figure 3; Supplementary Figure S3 in the supplementary information, respectively. The application of ASi fertilizers reduced the hydraulic conductivity of soils at near saturation compared to the control soils (without any ASi). In both the quartz and the sandy soils, a lower hydraulic conductivity at the near-saturated zone was observed in treatments of 5% ASi compared to controls. As soil dried, the presence of ASi in both soils prevented a large drop in the hydraulic conductivity of soils and maintained a higher hydraulic conductivity than the control soils.
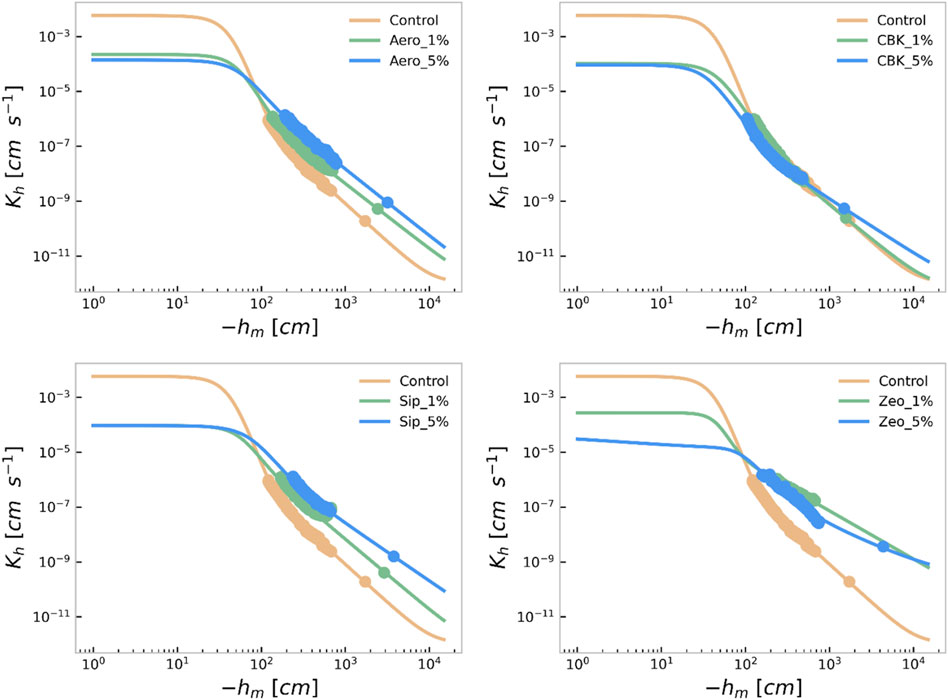
FIGURE 3. Different ASi amendments reduced the hydraulic conductivity of soils at near-saturated conditions, but it maintained a higher hydraulic conductivity as soil dried in sandy soil and pure quartz sand (Supplementary Figure S3 as supplementary information). The dots are the measured hydraulic conductivity curves, and the lines are the estimated curves based on the PDI model.
Silica amendments mitigate water stress to plants: Simulation results
To better illustrate the effect of Si on plant access to water under soil drying conditions, we simulated root water uptake by considering a single root extracting water from soils treated with and without Aerosil300 at a concentration of 1 and 5%. In our simulation, the hydraulic properties of soil domain were parameterized based on the measured hydraulic properties of soils (Figure 1). Figure 4 shows an exemplary profile of soil matric potential at different time intervals during soil drying at a constant rate. With no Aerosil300 addition (Figure 4, left), the matric potential remained rather flat with minimal gradients for the first 50 h. Starting from 60 h on, a remarkable matric potential gradient developed close to the root. After 65.2 h, the matric potential dropped at the root surface and reached the predefined PWP. This drop is related to the radial nature of root water uptake requiring a greater flux at the vicinity of roots (radial geometry related) and also nonlinear reduction of hydraulic conductivity (soil hydraulic properties related) as soil dries (Gardner 1965). Adding 1% Aerosil300 in the sandy soil delayed the large drop in matric potential at the root surface and let water extraction from the soil 82.5 h before the soil matric potential reached the predefined PWP (Figure 4 right). The simulated matric potential at the soil-root interface (Figure 5) showed that by the addition of 1 and 5% Aerosil300, the matric potential drop across the soil towards the root surface was attenuated.
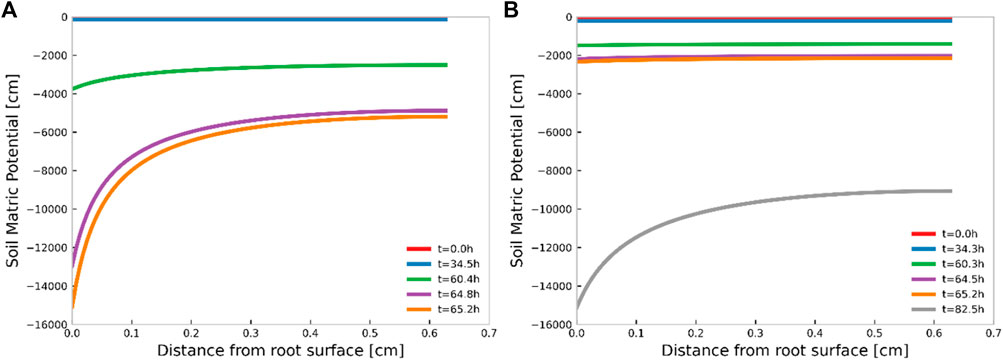
FIGURE 4. Simulated profiles of the matric potential plotted as a function of distance from root surface at different time steps for the case of sandy soil treated with (B) and without (A) an addition of 1% Aerosil300. A steady rate root water uptake is simulated till soil matric potential reached a critical value corresponding to PWP at the root surface in the soil. With the addition of 1% Aerosil300, root water extraction induced soil drying cycle lasted 17.3 h longer [t = 82.5 h, (B)] than for the control.
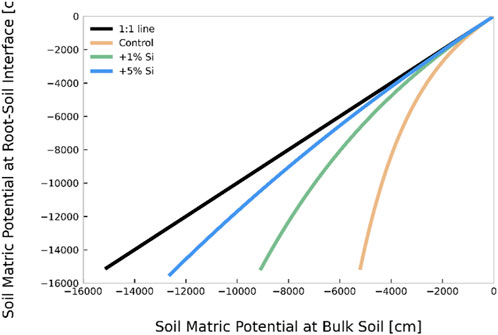
FIGURE 5. Matric potential at the root surface
Discussion
The objective of this study was to explore the potential impact of Si application to soils on the retention and flow of water in soils and their consequence on plant access to water under soil drying conditions. Our findings showed that i) increasing the ASi content in both soils resulted in a strong increase in the water holding capacity of soils, ii) soils treated with ASi maintained a higher hydraulic conductivity under soil drying conditions than the control soil, and iii) with the help of model simulation we showed soils treated with ASi could longer sustain the transpirational demand of plants during a soil drying cycle.
The improved water retention upon the ASi application was associated with an enhanced plant available water content. This enhancement can be explained by the water adsorption capacity of the added ASi (Schaller et al., 2020a). The ASi may impact soil water retention by increasing the capillary force holding water within soil pores (Tuller et al., 1999), which is directly related to the pore size distribution of soils (the smaller the pore size, the greater is the capillary force). In coarse-textured soils, such as those studied here, the application of ASi may shift the soil pore size distribution towards smaller pores due to the smaller size of newly added particles and by filling of larger pores by the amorphous silicate. In addition, the retention of water in the presence of ASi can also be affected by increased adsorptive forces acting on the liquid phase (Tuller et al., 1999) due to the large specific surface area and also internal porosity of ASi particles.
The effect of ASi amendments on water availability to plants was not only evaluated in terms of plant water content but also in terms of energy required for plants to extract a unit volume of water from soil by estimation of integral energy based on retention curve data (Minasny and McBratney 2003; Van Lier et al., 2006). The results showed more energy would be needed by plants to extract water from soils treated after ASi-amendments and this energy increases with ASi content in the soil. This indeed is related to the retention of water within soil with stronger capillary and adsorptive forces.
The measurements of Kh showed that under soil drying conditions, sandy soils treated with different ASi amendments maintain higher hydraulic conductivity than the control. This suggests application of ASi into sandy soil facilitates the flow of water within soil and possibly towards the plant roots under soil drying conditions. The latter effect was clearly illustrated from the simulated profile of matric potentials around a single root extracting water from soil during a drying cycle. Soils treated with 1% Aerosil 300 could sustain water extraction from soil under steady rate conditions for a longer time than the control soil. Indeed, as soil dries, its hydraulic conductivity drops by several orders of magnitude, limiting water flow within the soil. Therefore, a large gradient in water potential develops in the vicinity of roots in soil. The modeling results showed that mixing soils with 1 or 5% Aerosil 300 by resulting in a higher Kh under drying conditions delays the development of large gradients in matric potential in soil and, therefore, may favor root water uptake. This enhanced soil water availability and Kh during drying conditions may explain the better plant performance during drought after ASi-fertilization as found in other studies (Hattori et al., 2005; Chen et al., 2011; Ibrahim et al., 2018; Schaller et al., 2021b).
When the soil water potential approaches the PWP in coarse-textured soils such as those from ZALF (Müncheberg) or the pure sand, the liquid phase may become spatially discontinuous or fragmented (Tecon and Or 2017), reducing microbial activities and nutrient diffusion. The addition of ASi increasing the water content at PWP, may potentially increase microbial activities and diffusion of solutes and nutrients. We expect the largest effects of ASi-amendments on soil water relations for coarse-textured soils as the small particles of ASi may help to decrease the fraction of pore water that is draining freely due to gravity, whereas for fine-textured soil the ASi may rather clog the pores decreasing water availability.
Conclusion
Here we explored the impact of ASi amendments on soil hydraulic properties and effects on water movement in sandy soil under soil drying induced by plant roots. The results confirmed that sandy soils treated with ASi held higher plant available water and maintained a higher hydraulic conductivity under soil drying conditions than the control soil. The simulation results of root water uptake suggest that increased plant available water content and unsaturated hydraulic conductivity explain longer sustainment of plants’ transpirational demand during soil drying. Our findings contribute to the overall understanding of how ASi mitigates drought stress for plants by changing soil-plant-water relations via improving flow and retention of water within coarse-textured soils.
Data availability statement
The raw data supporting the conclusion of this article will be made available by the authors, without undue reservation.
Author contributions
JS had the idea. JS, MZ, and HG designed the experiments. MZ and BH conducted the experiments and did the measurements. BH and MZ did the modelling. MZ, JS, and HG wrote the manuscript. All authors discussed the results and commented on the manuscript.
Acknowledgments
We thank Gisela Ciesielski from the Institute of Soil Science and Site Ecology (Technische Universität Dresden, Germany) for carrying out the HYPROP-measurements of the sandy soil samples.
Conflict of interest
The authors declare that the research was conducted in the absence of any commercial or financial relationships that could be construed as a potential conflict of interest.
Publisher’s note
All claims expressed in this article are solely those of the authors and do not necessarily represent those of their affiliated organizations, or those of the publisher, the editors and the reviewers. Any product that may be evaluated in this article, or claim that may be made by its manufacturer, is not guaranteed or endorsed by the publisher.
Supplementary material
The Supplementary Material for this article can be found online at: https://www.frontiersin.org/articles/10.3389/fenvs.2022.935012/full#supplementary-material
References
Allen, C. D., Macalady, A. K., Chenchouni, H., Bachelet, D., McDowell, N., Vennetier, M., et al. (2010). A global overview of drought and heat-induced tree mortality reveals emerging climate change risks for forests. For. Ecol. Manage. 259, 660–684. doi:10.1016/j.foreco.2009.09.001
Anjum, S. A., Xie, X.-y., Wang, L.-c., Saleem, M. F., Man, C., and Lei, W. (2011). Morphological, physiological and biochemical responses of plants to drought stress. Afr. J. Agric. Res. 6, 2026–2032. doi:10.5897/AJAR10.027
Assouline, S., and Or, D. (2014). The concept of field capacity revisited: Defining intrinsic static and dynamic criteria for soil internal drainage dynamics. Water Resour. Res. 50, 4787–4802. doi:10.1002/2014wr015475
Carey, J. C., and Fulweiler, R. W. (2016). Human appropriation of biogenic silicon–the increasing role of agriculture. Funct. Ecol. 30, 1331–1339. doi:10.1111/1365-2435.12544
Carminati, A., Schneider, C. L., Moradi, A. B., Zarebanadkouki, M., Vetterlein, D., Vogel, H. J., et al. (2011). How the rhizosphere may favor water availability to roots. Vadose Zone J. 10, 988–998. doi:10.2136/vzj2010.0113
Celia, M. A., Bouloutas, E. T., and Zarba, R. L. (1990). A general mass-conservative numerical solution for the unsaturated flow equation. Water Resour. Res. 26, 1483–1496. doi:10.1029/wr026i007p01483
Chen, W., Yao, X., Cai, K., and Chen, J. (2011). Silicon alleviates drought stress of rice plants by improving plant water status, photosynthesis and mineral nutrient absorption. Biol. Trace Elem. Res. 142, 67–76. doi:10.1007/s12011-010-8742-x
Fahad, S., Bajwa, A. A., Nazir, U., Anjum, S. A., Farooq, A., Zohaib, A., et al. (2017). Crop production under drought and heat stress: Plant responses and management options. Front. Plant Sci. 8, 1147. doi:10.3389/fpls.2017.01147
Gardner, W. (1965). Dynamic aspects of soil-water availability to plants. Annu. Rev. Plant Physiol. 16, 323–342. doi:10.1146/annurev.pp.16.060165.001543
Gardner, W. R. (1960). Dynamic aspects of water availability to plants. Soil Sci. 89, 63–73. doi:10.1097/00010694-196002000-00001
Hattori, T., Inanaga, S., Araki, H., An, P., Morita, S., Luxova, M., et al. (2005). Application of silicon enhanced drought tolerance in Sorghum bicolor. Physiol. Plant. 123, 459–466. doi:10.1111/j.1399-3054.2005.00481.x
Ibrahim, M. A., Merwad, A.-R. M., and Elnaka, E. A. (2018). Rice (Oryza Sativa L.) tolerance to drought can be improved by silicon application. Commun. Soil Sci. Plant Anal. 49, 945–957. doi:10.1080/00103624.2018.1448856
Iden, S. C., and Durner, W. (2014). Comment on “Simple consistent models for water retention and hydraulic conductivity in the complete moisture range” by A. Peters. Water Resour. Res. 50, 7530–7534. doi:10.1002/2014wr015937
IPCC (2013). Climate change 2013: The physical science basis. Contribution of working Group I to the fifth assessment report of the intergovernmental panel on climate change. Cambridge, United Kingdom and New York, NY, USA: Cambridge University Press.
Katz, O., Puppe, D., Kaczorek, D., Prakash, N. B., and Schaller, J. (2021). Silicon in the soil–plant continuum: Intricate feedback mechanisms within ecosystems. Plants 10, 652. doi:10.3390/plants10040652
Lehner, B., Döll, P., Alcamo, J., Henrichs, T., and Kaspar, F. (2006). Estimating the impact of global change on flood and drought risks in europe: A continental, integrated analysis. Clim. Change 75, 273–299. doi:10.1007/s10584-006-6338-4
Michaelian, M., Hogg, E. H., Hall, R. J., and Arsenault, E. (2011). Massive mortality of aspen following severe drought along the southern edge of the Canadian boreal forest. Glob. Chang. Biol. 17, 2084–2094. doi:10.1111/j.1365-2486.2010.02357.x
Minasny, B., and McBratney, A. (2003). Integral energy as a measure of soil-water availability. Plant Soil 249, 253–262. doi:10.1023/a:1022825732324
Peters, A., Iden, S. C., and Durner, W. (2015). Revisiting the simplified evaporation method: Identification of hydraulic functions considering vapor, film and corner flow. J. Hydrol. X. 527, 531–542. doi:10.1016/j.jhydrol.2015.05.020
Puppe, D., Ehrmann, O., Kaczorek, D., Wanner, M., and Sommer, M. (2015). The protozoic Si pool in temperate forest ecosystems—quantification, abiotic controls and interactions with earthworms. Geoderma 243, 196–204. doi:10.1016/j.geoderma.2014.12.018
Puppe, D., Kaczorek, D., Schaller, J., Barkusky, D., and Sommer, M. (2021). Crop straw recycling prevents anthropogenic desilication of agricultural soil-plant systems in the temperate zone – results from a long-term field experiment in NE Germany. Geoderma 403, 115187. doi:10.1016/j.geoderma.2021.115187
Saxton, K. E., and Rawls, W. J. (2006). Soil water characteristic estimates by texture and organic matter for hydrologic solutions. Soil Sci. Soc. Am. J. 70, 1569–1578. doi:10.2136/sssaj2005.0117
Schaller, J., Cramer, A., Carminati, A., and Zarebanadkouki, M. (2020a). Biogenic amorphous silica as main driver for plant available water in soils. Sci. Rep. 10, 2424. doi:10.1038/s41598-020-59437-x
Schaller, J., Frei, S., Rohn, L., and Gilfedder, B. S. (2020b). Amorphous silica controls water storage capacity and phosphorus mobility in soils. Front. Environ. Sci. 8, 1–12. doi:10.3389/fenvs.2020.00094
Schaller, J., Puppe, D., Kaczorek, D., Ellerbrock, R., and Sommer, M. (2021a). Silicon cycling in soils revisited. Plants 10, 295. doi:10.3390/plants10020295
Schaller, J., Scherwietes, E., Gerber, L., Vaidya, S., Kaczorek, D., Pausch, J., et al. (2021b). Silica fertilization improved wheat performance and increased phosphorus concentrations during drought at the field scale. Sci. Rep. 11, 20852–20912. doi:10.1038/s41598-021-00464-7
Sommer, M., Kaczorek, D., Kuzyakov, Y., and Breuer, J. (2006). Silicon pools and fluxes in soils and landscapes - a review. Z. Pflanzenernahr. Bodenk. 169, 310–329. doi:10.1002/jpln.200521981
Struyf, E., Smis, A., Van Damme, S., Garnier, J., Govers, G., Van Wesemael, B., et al. (2010). Historical land use change has lowered terrestrial silica mobilization. Nat. Commun. 1, 129. doi:10.1038/ncomms1128
Tecon, R., and Or., D. (2017). Biophysical processes supporting the diversity of microbial life in soil. Fems Microbiol. Rev. 41, 599–623. doi:10.1093/femsre/fux039
Tuller, M., Or, D., and Dudley, L. M. (1999). Adsorption and capillary condensation in porous media: Liquid retention and interfacial configurations in angular pores. Water Resour. Res. 35, 1949–1964. doi:10.1029/1999wr900098
Van Lier, Q. d. J., Metselaar, K., and Van Dam, J. C. (2006). Root water extraction and limiting soil hydraulic conditions estimated by numerical simulation. Vadose Zone J. 5, 1264–1277. doi:10.2136/vzj2006.0056
Vandevenne, F., Struyf, E., Clymans, W., and Meire, P. (2012). Agricultural silica harvest: Have humans created a new loop in the global silica cycle? Front. Ecol. Environ. 10, 243–248. doi:10.1890/110046
Keywords: available plant water, drought stress, HYPROP, field capacity, permanent wilting point, soil hydraulic conductivity, soil water retention, water holding capacity
Citation: Zarebanadkouki M, Hosseini B, Gerke HH and Schaller J (2022) Amorphous silica amendment to improve sandy soils’ hydraulic properties for sustained plant root access under drying conditions. Front. Environ. Sci. 10:935012. doi: 10.3389/fenvs.2022.935012
Received: 03 May 2022; Accepted: 18 July 2022;
Published: 10 August 2022.
Edited by:
Ilan Stavi, Dead Sea and Arava Science Center, IsraelCopyright © 2022 Zarebanadkouki, Hosseini, Gerke and Schaller. This is an open-access article distributed under the terms of the Creative Commons Attribution License (CC BY). The use, distribution or reproduction in other forums is permitted, provided the original author(s) and the copyright owner(s) are credited and that the original publication in this journal is cited, in accordance with accepted academic practice. No use, distribution or reproduction is permitted which does not comply with these terms.
*Correspondence: Jörg Schaller, Sm9lcmcuU2NoYWxsZXJAemFsZi5kZQ==