- Department of Ecology and Conservation Biology, Texas A&M University, College Station, TX, United States
Grasslands and savannas in drylands have been and continue to be converted to woodlands through a phenomenon often described as woody plant encroachment. This conversion has profound implications for the ecosystem services that these landscapes provide, including water. In this paper, using examples from six case studies across drylands in the Great Plains and Chihuahuan Desert regions of the United States, we explore the ecohydrological changes that occurred following woody plant encroachment (WPE). In all cases, the increase in woody plant cover brought about modifications in connectivity, which led to profound ecohydrological changes at both the patch and landscape scales. At the wet end of the dryland spectrum (subhumid climates), increases in evapotranspiration following WPE led to reduced streamflows and groundwater recharge. In drier regions, woody plant encroachment did not alter evapotranspiration appreciably but did significantly alter hydrological connectivity because of changes to soil infiltrability. In semiarid climates where rainfall is sufficient to maintain cover in intercanopy areas concurrent with woody plant encroachment (thicketization), overall soil infiltrability was increased—translating to either decreased streamflows or increased streamflows, depending on soils and geology. In the driest landscapes, woody plant encroachment led to xerification, whereby intercanopy areas became bare and highly interconnected, resulting in higher surface runoff and, ultimately, higher groundwater recharge because of transmission losses in stream channels. On the basis of our review of the studies’ findings, we argue that the concept of ecohydrological connectivity provides a unifying framework for understanding these different outcomes.
Introduction
Grasslands and savannas represent important types of vegetation cover within drylands globally—particularly those under semiarid and subhumid climate regimes. Prior to the advent of mechanized agriculture, grasslands and savannas accounted for close to 40% of the Earth’s surface. Today, these landscapes cover only a little over 20% (Mishra and Young, 2020) and are considered an imperiled biome, threatened by agricultural conversion, overgrazing, altered fire regimes, and invasive plants (Wilcox et al., 2022). A prominent example of these proximate threats is woody plant encroachment (WPE)—the process by which grasslands and savannas are converted to woodlands or more densely wooded savannas.
The proliferation of trees and shrubs on rangelands is one of the most striking land-cover transformations of the last 100–200 years, and represents one of the gravest threats to grassland biomes (Sala and Maestre, 2014; Veldman et al., 2015). Much of this transformation has been driven by unsustainable livestock production and overgrazing. In the United States, especially during the late 1800s, overgrazing played a key role in disrupting longstanding fire regimes, which set the stage for the expansion of woody plants (Wilcox et al., 2018). Similar transitions took place in Australia and southern Africa with the arrival of Anglo-European settlers and their livestock (Archer et al., 2017). Although grazing management has improved in all these regions, many drylands remain chronically overgrazed, leading to vegetation regime shifts (Hoover et al., 2020). In addition to overgrazing, other factors contributing to WPE include the dissemination of woody-plant seeds by livestock, eradication of native browsers, increased atmospheric CO2 concentrations, and a warming climate (Archer et al., 2017).
The world’s grasslands and savannas are critically important for livestock production and the pastoral societies that depend on them (Yahdjian et al., 2015; Mishra and Young, 2020). Almost without exception, forage production will decline as woody plants increase (Archer et al., 2017)—as will, in many cases, species richness and biodiversity, especially those species endemic to more open ecosystems (Ratajczak et al., 2012; Archer and Predick, 2014). It is important to note that WPE is not necessarily synonymous with landscape degradation and/or desertification (Eldridge et al., 2011; Bestelmeyer et al., 2018). This is primarily true in the case of subhumid or relatively wet semiarid climates in which both canopy and intercanopy vegetation cover remains high year round (Basant et al., 2020). In some instances, WPE may even slow or reverse degradation or desertification that has already begun (Maestre et al., 2009). Woody plant encroachment that does not increase the extent of bare ground—either because the intercanopy patches remain vegetated with herbaceous plants and/or woody vegetation forms dense thickets—has been described as thicketization (Schreiner-McGraw et al., 2020). In contrast, WPE that does increase the extent of bare ground, eventuating in the degradation and heightened soil erosion described as xerification, is seen in more arid climates (Li et al., 2007). Heavy grazing by livestock can extend the amount of terrain affected by xerification (Webb et al., 2014).
In addition to causing declines in forage production and biodiversity, WPE can alter biogeochemical, energy, and water cycles in important but not always well-understood ways. In regions having annual rainfall exceeding 400 mm, above-ground carbon storage will generally increase with WPE. However, changes in below-ground carbon are less certain and are often unpredictable (Archer et al., 2017). With respect to climate, Wang et al. (2021) report that WPE can lead to subtle but important changes in temperatures in the Southern Great Plains. They found that daytime temperatures were lower and nighttime temperatures were higher following WPE.
With respect to the water cycle, WPE has the potential to affect primarily two contrasting processes: evapotranspiration and soil infiltrability. As the number and density of woody plants increase, interception and transpiration rates will rise, translating to higher evapotranspiration. At the same time, as woody cover increases, soil infiltrability will be altered through root action and the addition of organic matter (Leite et al., 2020). Historically, most scientific investigations of the mechanisms by which woody plants may alter groundwater recharge or streamflow in drylands have focused on evapotranspiration as the key mechanism (Bosch and Hewlett, 1982). This is true even of frameworks developed quite recently, largely overlooking the importance of changes to soil infiltrability (Huxman et al., 2005; Wilcox et al., 2006). Such frameworks emphasize how WPE may lead to higher evaporative losses in drylands when rainfall is greater than potential evapotranspiration for a period of time. Examples include Mediterranean climate regimes, where rainfall is “out of phase” with potential evapotranspiration; and areas where groundwater levels are shallow enough to be accessed by woody plants.
In the past several years, a number of studies have drawn attention to the fact that changes in soil infiltrability are important as well (Ilstedt et al., 2016; Basant et al., 2020; Leite et al., 2020). In general, infiltrability is higher under the canopy of woody plants than in the intercanopy, as woody roots form a network of macropores that enable preferential water flow, often promoting deep water percolation (Devitt and Smith, 2002; Tobella et al., 2014). In addition, through their canopies and through the accumulation of litter, woody plants provide mechanical protection against soil crusting from solar radiation and raindrop impact. By adding organic matter and promoting microbial activity, leaf litter acts as a soil amendment, enhancing soil structure and aggregate stability (Bochet et al., 1999). It also provides ideal conditions for soil fauna that—like woody roots—improve macroporosity and infiltrability (Marquart et al., 2020). Some woody plants have a strong legacy effect on soils. High infiltrability under canopies can last long after the plants have died or been removed (Cipriotti and Aguiar, 2017; Williams et al., 2019) and can even increase over time as roots decay (Wu et al., 2021).
As described in this paper, the two phenomena—evapotranspiration and soil infiltration—can have diametrically opposing influences on groundwater recharge and streamflows in drylands. For example, in regions where WPE leads to higher evapotranspiration, groundwater recharge and/or streamflows will decline as woody plant cover increases (Zou et al., 2016). However, for many drylands potential evapotranspiration (PET) greatly exceeds rainfall and there is relatively little “surplus water.” Thus, any changes in total evapotranspiration caused by WPE will be quite small. Instead, in these landscapes WPE will often lead to higher soil infiltrability, which—depending on soil and geologic conditions—can either reduce streamflows and groundwater recharge (Wilcox et al., 2008a), increase streamflows and groundwater recharge (Wilcox and Huang, 2010), or have minimal effect (Berg et al., 2016).
Although at first glance these outcomes appear so variable as to defy a unifying explanation, recent research suggests that such an explanation of the influences of WPE on the water cycle (and how those influences vary with scale) is provided by the concept of ecohydrological connectivity. As support for this assertion we offer the findings of six case studies carried out in the Southern Great Plains and Southwest regions of the United States and representing a climatic gradient that stretches from subhumid to arid. Each of these studies showed that WPE has altered groundwater recharge and/or streamflows in significant ways.
Connectivity as a unifying concept
Connectivity is broadly defined as the extent to which a system facilitates the transfer of matter and energy within itself (Keesstra et al., 2020). In the field of Earth and environmental sciences, this concept has been increasingly applied as an organizing principle for understanding environmental change and interactions across spatial scales (Peters et al., 2008; Okin et al., 2015; Iwaniec et al., 2021). At the same time, even within the hydrological sciences multiple definitions of the term exist (Bracken et al., 2013; Wohl, 2017). With respect to drylands, Okin et al. (2015) define connectivity as the extent to which materials can move, spread, or be redistributed from one place to another within the landscape—a definition similar to those proposed by others (Tetzlaff et al., 2007; Wohl, 2017). An essential characteristic of connectivity is that it functions spatially in multiple directions, including longitudinal (river channel), lateral (hillslope), and vertical (surface–subsurface) (Keesstra et al., 2018).
The connectivity concept is increasingly being employed by hydrologists and geomorphologists to better understand and describe water and sediment fluxes (Bracken et al., 2013; Bracken et al., 2015; Keesstra et al., 2018). It has been usefully applied by hydrologists in many environments, including wetlands (Cohen et al., 2016; Lane et al., 2018; Leibowitz et al., 2018), river systems (Castello et al., 2013; Jaeger et al., 2014; Goodrich et al., 2018), and arctic regions (Bring et al., 2016; Walvoord and Kurylyk, 2016)—at continental and even global scales (Peters et al., 2008; Good et al., 2015)—but it has been especially useful in clarifying ecosystem function and interaction in drylands (Turnbull and Wainwright, 2019; Saco et al., 2020; Calvo-Cases et al., 2021; Johnson et al., 2021). Okin et al. (2015) argue that connectivity serves as an “organizing principle to understand dryland structure and function at scales from individual plants to entire landscapes.” It has proved to be particularly valuable for understanding cross-scale interactions (Peters et al., 2006). For example, as discussed below, changes in infiltrability at the patch scale can have important implications for water fluxes at the landscape and even regional scales.
Depending on the context and the application, various modifiers have been applied to the basic term: hydrological connectivity (Bracken et al., 2013), ecohydrological connectivity (D'Odorico et al., 2010; Basant et al., 2020), eco-geomorphological connectivity (Calvo-Cases et al., 2021), stream connectivity (Goodrich et al., 2018), sediment connectivity (Bracken et al., 2015), etc. We believe that the term ecohydrological connectivity is appropriate when exploring how vegetation influences the water cycle, because of the intimate coupling between biota and the extent of connectedness of the landscape. As noted by Keesstra et al. (2018), biota can promote or impede connectivity. Plants, for example, reduce raindrop impact, modify soils through root activity and the addition of litter, and promote soil infiltrability. In drylands, the patchy nature of vegetation determines connectivity, which in turn controls source–sink dynamics—a critical factor for ecosystem functionality (Reid et al., 1999; Wilcox et al., 2003; Ludwig et al., 2005).
Case studies along a climate gradient
Using examples from six case studies carried out in the United States (Figure 1), we explore the ecohydrological changes that occur concomitant with WPE. Although these changes vary with location, depending on the particular conditions of climate, soils, geology, and topography, we demonstrate that the concept of ecohydrological connectivity provides a unifying framework for understanding and even predicting landscape-level ecohydrological responses to WPE.
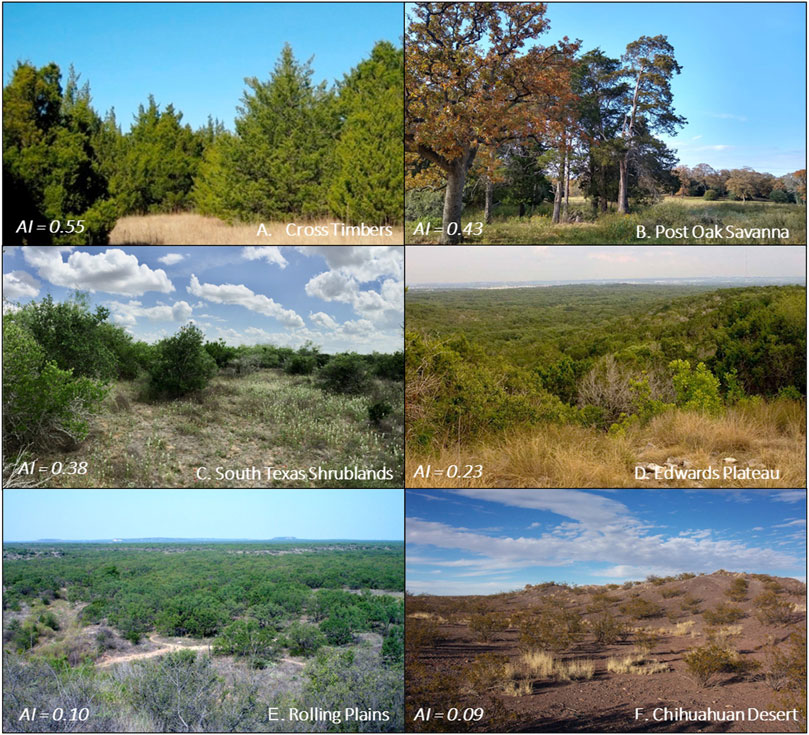
FIGURE 1. The six ecoregions represented by the case studies on which our evaluation is based: (A). Case Study 1--Cross Timbers of Oklahoma; (B). Case Study 2--Post Oak Savanna in Texas; (C). Case Study 3—South Texas Shrublands; (D). Case Study 4—Edwards Plateau of Texas; (E). Case Study 5—Rolling Plains of Texas; and (F). Case Study 6—Chihuahuan Desert of New Mexico.
These case studies, drawn from the Southern Great Plains and Southwest regions of the United States, span the climate spectrum of drylands from subhumid to arid (Figures 2, 3). These landscapes have been dramatically altered by WPE, beginning in the late 1800s—largely in response to a combination of overgrazing, droughts, and altered fire regimes (Bestelmeyer et al., 2018; Wilcox et al., 2018; Wilcox et al., 2022). Each of the six sites has been the focus of intensive studies addressing the ecohydrological ramifications of WPE. The case studies are discussed below roughly in the order of annual rainfall (from high to low).
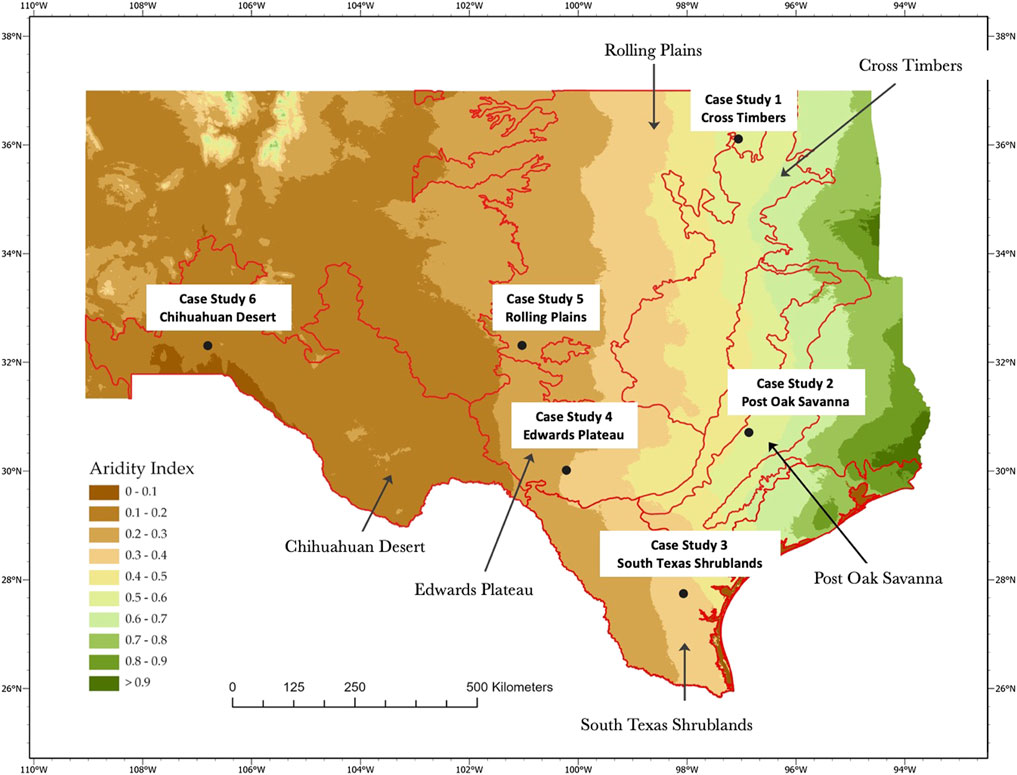
FIGURE 2. Locations of the six study sites within the ecoregions represented by the six case studies.
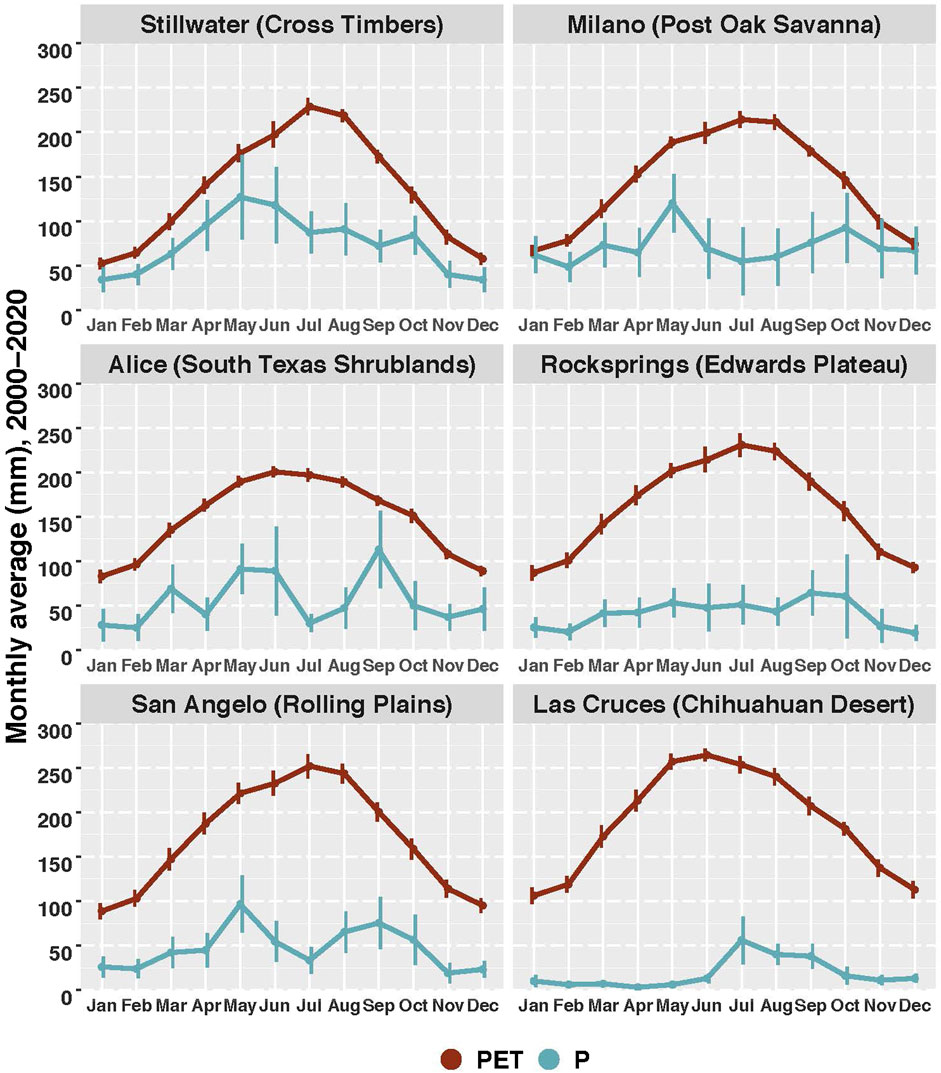
FIGURE 3. Mean values of potential evapotranspiraton (PET) and precipitation (P) for the period 2000–2020 in representative locations for each of the six case studies. PET was calculated using the MOD16 data set (Mu et al., 2011). Vertical bars represent 95% confidence interval.
Case Study 1: Cross Timbers—Proliferation of Eastern Red Cedar.
Overview. The states of the Central Great Plains, including Nebraska, Kansas, and Oklahoma, form the epicenter of WPE in the United States (Barger et al., 2011), largely because of the proliferation of eastern red cedar (Juniperus virginiana). According to recent assessments, areas dominated by this species are expanding by about 8% a year—which amounts to about 40 km2/year (Wang et al., 2017; Wang et al., 2018a). This expansion is especially pronounced in the Cross Timbers region of Oklahoma, a mosaic of upland deciduous forest, savanna, and tallgrass prairie that has been transformed by WPE. The climate is subhumid, with an average annual rainfall of around 900 mm and an aridity index (AI) of 0.5–0.6. Although the region is water limited for part of the year, it is on the wetter end of the “drylands” spectrum (Hoover et al., 2020) (Figure 2) and rainfall may exceed potential evapotranspiration during parts of the year (Figure 3).
Over the last several decades, an active research program within the Cross Timbers region has provided much of what we know concerning the ecohydrological implications of the proliferation of eastern red cedar in the Central Great Plains (Zou et al., 2018). In the past decade in particular, this research has provided detailed insights into the effects of encroachment of grasslands and savannas by eastern red cedar (Zou et al., 2014; Zou et al., 2015; Zou et al., 2016; Acharya et al., 2017a; Acharya et al., 2017b; Qiao et al., 2017). Once these areas have undergone encroachment, they essentially become closed-canopy woodlands dominated by eastern red cedar.
This body of work has brought to light such effects as drier soils, less groundwater recharge, and lower streamflow. For example, results from small catchments demonstrated that whereas runoff from grasslands was on average around 10% of precipitation, runoff from the juniper-encroached areas was only 2%. In addition, runoff dynamics were altered: whereas runoff from grasslands was typically generated as saturation overland flow, that from the juniper-encroached sites was generated as infiltration-excess overland flow (Qiao et al., 2017). These ecohydrological changes are being driven largely by higher transpiration and interception losses in the juniper woodlands (Caterina et al., 2014; Wang et al., 2018b; Torquato et al., 2020). Modeling exercises and historical streamflow records suggest that these smaller-scale changes will result in reduced streamflows at larger scales (Zou et al., 2016; Starks and Moriasi, 2017). Interestingly, soil infiltrability is much higher following encroachment but it does not seem to confer any hydrological benefits. Soils are consistently drier in the wooded areas (Zou et al., 2014).
Underlying mechanisms, landscape-scale responses, and changes in connectivity. Woody plant encroachment in and adjacent to the Cross Timbers region of Oklahoma has resulted in higher losses of water via evapotranspiration—owing to increases in both interception and transpiration by trees. These changes have reduced lateral as well as vertical ecohydrological connectivity, with a consequent diminishing of both groundwater recharge and streamflow generation. Modeling work and field observations suggest that these changes translate to larger-scale reductions in streamflows.
Case Study 2: Post Oak Savanna—Thicketization.
Overview. The second case study comes from the Post Oak Savanna (POS) ecoregion, which extends from northeastern to southwestern Texas (Figure 2). Like other oak savannas in the United States, it has been radically transformed by cultivation and subsequent abandonment, altered fire regimes, urbanization, and fragmentation (Hoagland et al., 1999). These factors are, of course, interrelated; but the net result is a highly fractured patchwork of pasturelands, open savannas, and dense woodlands (Figure 1). Many of the remaining woodlands are now thicketized, with a dense understory of eastern red cedar and yaupon (Ilex vomitoria). True savannas composed of native grasses and a scattering of trees are rare in the POS. Where they exist, the common grass species are big blue stem (Andropogon gerardi), indiangrass (Sorghastrum nutans), and switchgrass (Panicum virgatum). Pastures in the region consist mostly of introduced grasses such as bahaiagrass (Paspalum notatum) and bermudagrass (Cynodon spp.).
The other significant feature of the POS is that it overlies the recharge zone of the Carrizo–Wilcox aquifer, the third most important aquifer in Texas. Although relatively few field studies have evaluated the influence of vegetation on recharge rates in the Carrizo–Wilcox, modeling work by Keese et al. (2005) suggests that the influence is profound. In a comprehensive evaluation of groundwater recharge across the state of Texas, they found that in addition to soil and climate, vegetation had a marked influence on groundwater recharge rates. Their modeling experiments showed that where the dominant vegetation consists of deep-rooted trees, recharge is significantly lower than where shallow-rooted grasses dominate.
The modeling predictions of Keese et al. (2005) have recently been verified by a field study close to Milano, Texas (Basant et al., 2020), where average annual rainfall is around 930 mm/yr and the AI is between 0.5 and 0.6 (Figure 2). This site, like the Cross Timbers region (Case Study 1), sits at the wet end of the dryland spectrum (Figures 2, 3). In this study, the chloride mass balance approach was used to compare long-term recharge rates in thicketized woodlands with those in open pastures. The results were striking: rates of deep drainage (which is equivalent to recharge) in the thicketized woodlands were only around 1 mm/yr—compared with 127 mm/yr in the open pastures. While these results need to be verified at more locations within the POS, they strongly suggest that thicketization dramatically reduces groundwater recharge.
Underlying mechanisms, landscape-scale responses, and changes in connectivity. The dramatic declines in groundwater recharge observed in thicketized woodlands are driven by the higher evapotranspiration rates that accompany WPE and bring about a reduction in vertical connectivity. It is likely that this loss of connectivity would also result in lowered baseflows in the streams that are partially fed by the Carrizo–Wilcox aquifer.
Case Study 3: South Texas Shrublands—Increased Soil Infiltrability.
Overview. Our next example comes from a subtropical savanna in the South Texas Shrublands (Figure 2), where average annual rainfall is around 660 mm/yr (Figure 3) and the AI is between 0.3 and 0.4 (Figure 2). Predominant woody plants include Texas persimmon (Diospyros texana) and mesquite (Prosopis glandulosa). Common grass species in the intercanopy areas include purple aristida (Aristida purpurea), Texas grama (Bouteloua rigidiseta), and love grass (Eragrostis intermedia). The region is distinct in that it consists of an upland savanna surrounded by a low-lying “drainage-woodlands” area—the result of heavy encroachment by woody plants—that exhibits a banded vegetation pattern (see Figure 4). Woody plants have subsequently expanded into the upland areas as well.
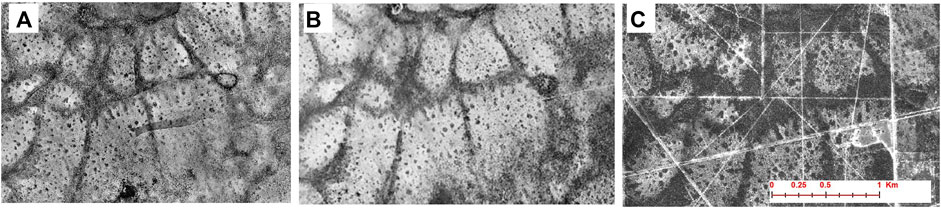
FIGURE 4. Aerial imagery showing changes over time in the study site for Case Study 3 (South Texas Shrublands) (A) 1930; (B) 1955; and (C) 1995. Note that woody vegetation is increasing with time and expanding from the drainage ways to the upland portions of the landscape.
Aerial photographs and travel accounts (Humphrey, 1958) from the early 20th century indicate that the two landforms were even more distinct in the past (Figure 4), when WPE was taking place mostly in the drainage-woodlands. Additional evidence confirming this early pattern of WPE was provided by Boutton et al. (1998), who found a greater accumulation of soil organic carbon as well as a higher mean age of woody plants in the drainage-woodlands than in the uplands. Additionally, on the basis of the mean residence time of 14C and δ13C in the soil organic carbon of the two landforms, Boutton et al. 1999) suggested that the process of WPE started around 150 years ago—roughly the time of the introduction of heavy grazing.
Basant et al. (2020), working at a location that had been ungrazed by domestic livestock for more than 40 years, tested the prevailing hypothesis that water from the uplands was subsidizing the drainage-woodlands. Over a 3-year period, they monitored runoff from large runoff plots on the uplands as well as soil water in both the uplands and the drainage-woodlands, and found that there was no overland flow from the uplands to the drainage areas.
In addition, when they measured soil infiltrability and compared it with that of an adjacent grazed area, they found much higher levels in the ungrazed study site—with the highest infiltrabilities occurring under the shrub clusters. Some 40 years ago, Weltz and Blackburn (1995) had monitored runoff over a 20-month period at the same site, from large (5 m × 7 m) plots. Their findings showed that at that time (soon after the removal of livestock), runoff was being generated from the upland areas; and that for rainfall events larger than 100 mm, runoff could be as high as 8% of rainfall. It is quite likely that 150 years ago, when the site was subjected to severe overgrazing, runoff from the uplands was much higher than it is today. On the basis of these findings, Basant et al. (2020) hypothesized that historical overgrazing amplified the runoff–runon process, resulting in significant subsidies of water from the uplands to the drainage areas. Then, the combination of relaxation of grazing pressure, increase in woody plants, and subsequent landscape recovery brought about the rise in soil infiltrability that made redistribution of water via surface runoff relatively rare.
It is likely that in addition to modifying soil infiltrability, woody plants have the effect of reducing groundwater recharge, but only slightly and primarily in wet years. Weltz and Blackburn (1995) found that for a relatively wet year (890 mm), deep drainage in areas that had been cleared of woody plants was 22 mm—whereas in areas not cleared of woody plants, no deep drainage at all was measured. Similarly, Moore et al. (2012) found that long-term recharge was only slightly affected by changes in woody plant cover: in areas cleared of woody plants, annual recharge was around 2 mm/yr, compared with zero where woody plants were not cleared.
Underlying mechanisms, landscape-scale responses, and changes in connectivity. In this landscape, the combination of WPE and the recovery of herbaceous plants that followed the decline in grazing led to increases in soil infiltrability, which in turn dramatically reduced lateral connectivity—with the result that the amount of runoff able to reach the low-lying drainage areas declined steeply. Although WPE also led to slight increases in evapotranspiration and deep drainage, the effect of these increases on total groundwater recharge is small.
Case Study 4: Edwards Plateau—Increasing Groundwater Recharge in Semiarid Karst Landscapes.
Overview. In the Edwards Plateau region of central Texas, average annual rainfall ranges from around 450–800 mm and the AI ranges from 0.2 to 0.4 (Figure 2). In spite of its semiarid climate, the Edwards Plateau is home to numerous perennially flowing springs, streams, and rivers. It is also the source area for the prolific and regionally important Edwards Aquifer, a major source of water for a number of urban areas as well as local agriculture. The explanation for this ecohydrological paradox is the underlying karst geology, which facilitates rapid and abundant groundwater recharge (Wilcox et al., 2007; Wilcox et al., 2008b).
The Edwards Plateau has undergone radical vegetation change as a result of historic overgrazing and subsequent WPE (Figure 1). Beginning in the late 1800s, pristine grasslands were rapidly converted to degraded and overgrazed rangelands; then, with the gradual reduction in grazing pressure (and the general lack of fire), the landscape has transitioned to woodlands dominated by live oak (Quercus fusiformis), Ashe juniper (Juniperus ashei), and redberry juniper (Juniperus pinchotii). A variety of short and mid-height grasses are common in the intercanopy areas.
Recognizing that such large-scale and dramatic changes in vegetation likely have an influence on streamflows (Wilcox, 2007), Wilcox and Huang (2010) explored the extent to which streamflows have changed in the Edwards Plateau. They analyzed long-term trends in streamflow, using data from four of the region’s major rivers. Using hydrograph separation techniques, they were able to determine the volumes of baseflows and stormflows—the logic being that while both baseflow and stormflow may be affected by changes in vegetation, the mechanisms by which they are affected are different. Baseflows will be affected by vegetation change that influences groundwater recharge, whereas stormflow will be affected by surface changes that influence overland flow.
Their findings were not only surprising but at first glance counterintuitive. Notwithstanding the prevailing view—in both lay and scientific circles—that woody plants were depleting spring flows, Wilcox and Huang (2010) found that baseflows had effectively doubled in the wake of WPE and overall recovery from overgrazing. It is in light of the known fact that vegetation (and woody plants in particular) enhance soil infiltrability that these results do make sense. For example, several studies have demonstrated that infiltrability under juniper trees is many times higher than in adjacent interspaces (Wilcox et al., 2008b; Leite et al., 2020).
Underlying mechanisms, landscape-scale responses, and changes in connectivity. In the Edwards Plateau region, the expansion of woody plant cover in combination with improved range condition has brought about an increase in soil infiltrability. In this case, even though the higher infiltrability has decreased lateral connectivity, at the same time—because of the high permeability of the karst substrate—it has substantially increased vertical connectivity. As a result, both groundwater recharge and baseflows in the streams and rivers have risen significantly.
Case Study 5: Rolling Plains of Texas—Diminishing Streamflows.
Overview. The North Concho River flows near the boundary between the Edwards Plateau and the Rolling Plains ecoregions in Texas—most of the river basin lying within the Rolling Plains (Figure 1). The average rainfall in this region is around 480 mm/yr and the AI is between 0.2 and 0.3 (Figure 2). The North Concho provides an interesting example of how changes in vegetation cover can translate to large-scale changes in streamflows. Since around 1960, mean annual streamflows have been 70% lower than they were in the first half of the 20th century—a change concurrent with a dramatic expansion of woody plants, in particular mesquite (Prosopis glandulosa) (Figure 1). Using the prevailing logic at the time, water planners attributed the decline in streamflows to increasing water use by mesquite; and with the goal of restoring streamflows, they implemented a large-scale brush removal program in the North Concho watershed. Between 2000 and 2005, more than one-third of the 3100-km2 watershed was cleared of mesquite. However, as reported in Wilcox et al. (2010), this large-scale effort did not result in any perceptible increase in streamflow.
In order to better understand what was driving streamflow in the North Concho, Wilcox et al. (2008a) conducted a detailed analysis of streamflow and precipitation records, going back to around 1915. What they found was that in this region streamflows make up a very small portion of the water budget and that most streamflow is generated from large rainfall events. In other words, most of the streamflow occurs as stormflows and is driven by infiltration-excess overland flow. But they also found that stormflows were much smaller and less frequent after 1960, despite the fact that average precipitation totals had not changed. These findings make sense in light of the extensive vegetation changes that took place over the preceding 100 years. As in the Edwards Plateau ecoregion (see Case Study 4), overgrazing dramatically altered vegetation cover in the Rolling Plains. Beginning around 1880 and lasting until around 1960, these rangelands were extremely heavily grazed. Then, when the numbers of grazing animals declined precipitously (Wilcox et al., 2012), vegetation cover—both herbaceous and woody—increased dramatically. Certainly, one result of the increase in tree cover has been higher soil infiltrability. For example, Wood et al. (1978) found that in previously heavily grazed rangelands in the Rolling Plains, infiltration rates were twice as high under mesquite canopies than in the intercanopy areas.
Several studies have found that mesquite expansion does increase evapotranspiration by a few millimeters a year (Carlson et al., 1990; Saleh et al., 2009). But as argued by Wilcox et al. (2010), in systems of this nature—in which most streamflow is generated as infiltration-excess overland flow, with only a small percentage coming from deep drainage—changes in evapotranspiration have little bearing on either groundwater recharge or streamflows.
Underlying mechanisms, landscape-scale responses, and changes in connectivity. Like the situation described in Case Study 3, in the North Concho the improved range condition brought about by greatly lowered grazing pressure and the concomitant expansion of woody plants has led to higher soil infiltrability and less overland flow—hence a dramatic reduction in lateral connectivity that has resulted in much lower streamflows.
Case Study 6: Chihuahuan Desert—Increases in Surface Runoff and in Groundwater Recharge.
Overview. In the Chihuahuan Desert, where average annual precipitation is around 230 mm and the AI is <0.1 (Figure 2), large swaths of desert grassland have been converted to shrublands consisting primarily of creosote bush (Larrea tridentate). The initial transition from desert grassland to shrubland was most likely driven by overgrazing, but with increasing temperatures and changes in precipitation patterns—including the onset of periodic multi-year droughts—the process is continuing (Bestelmeyer et al., 2018). In addition, once the process has been initiated, self-reinforcing feedback loops may occur that accelerate the shift from grassland to shrubland (D'Odorico et al., 2012; Turnbull et al., 2012). As woody plants become dominant, water, soil, and nutrient resources are concentrated in the shrub clusters—at the expense of intercanopy areas, which gradually turn into bare ground (Schlesinger et al., 1990). With the consequent declines in surface roughness and soil infiltrability, the intercanopy areas become highly interconnected, which gives rise to much greater surface runoff than from desert grasslands. A recent modeling analysis suggests that in such shrublands, the increased surface runoff significantly augments groundwater recharge (Schreiner-McGraw et al., 2020), owing to the high transmission losses that occur in desert washes during runoff events (Schreiner-McGraw and Vivoni, 2018; Schreiner-McGraw et al., 2019). In this way, the conversion of desert grasslands to shrublands actually increases groundwater recharge.
Underlying mechanisms, landscape-scale responses, and changes in connectivity. At the drier end of the dryland spectrum (<300 mm rainfall/year), WPE leads to increasing bare ground, or xerification (Schreiner-McGraw et al., 2020). As noted above, the net result is dramatically higher lateral connectivity via a network of intercanopy patches, which greatly facilitates surface runoff and ultimately leads to higher groundwater recharge via transmission loss in stream channels.
A framework for understanding the ecohydrology of woody plant encroachment
As demonstrated by the case studies presented here, woody plant encroachment in dryland ecosystems does have profound ecohydrological consequences—at multiple scales—driven primarily by changes in evapotranspiration and/or soil infiltrability. The findings of these studies provide evidence for the existence of two thresholds in ecohydrological response to WPE: one occurring at an AI value of around 0.5, and the other at an AI value of about 0.1, which correspond roughly to the dividing lines between the three climate zones of subhumid, semiarid, and arid. We found that ecohydrological responses to WPE differed among the three zones and were related to changes in ecohydrological connectivity. These findings are conceptualized in Figure 5.
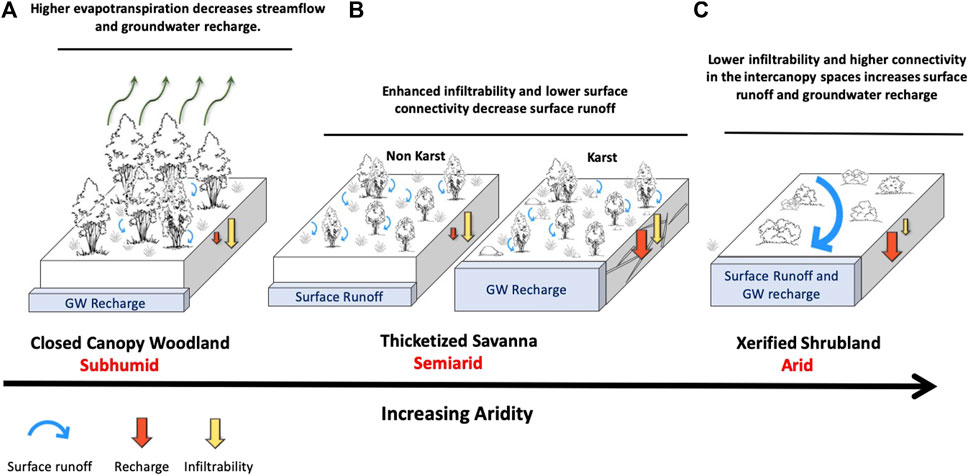
FIGURE 5. Conceptualized framework for understanding how woody plant encroachment modifies ecohydrological processes. (A). Closed Canopy Woodlands: In subhumid climates (aridity index 0.5–0.7) woody encroachment will result in higher evapotranspiration leading to lower streamflows and groundwater recharge. (B). Thicketized Savannas: in semiarid climates where rainfall is sufficient to maintain vegetated intercanopy areas (aridity index 0.2–0.5) woody encroachment enhances infiltrability (lower connectivity) resulting in less surface runoff. Where substrate is highly permeable such as karst landscapes, groundwater recharge may be increased. (C). Xerified shrublands: in arid climates (aridity index <0.2) woody encroachment increases landscape connectivity because of interconnected bare intercanopy patches. As a result surface runoff is increased as is groundwater recharge as a result of higher transmission losses in stream channels.
For subhumid climates, WPE results in the formation of closed-canopy woodlands and thereby significant increases in evapotranspiration, leading to reduced ecohydrological connectivity (both vertical and lateral). The result, as exemplified by the findings in the Cross Timbers region (Case Study 1) and in the Post Oak Savanna (Case Study 2), is lower groundwater recharge and streamflows. In both of these ecosystems, average annual precipitation is around 900 mm and the aridity index is between 0.5 and 0.6 (Figure 2); rainfall upon occasion may exceed PET, especially during the cooler periods of the year (Figure 3).
Three of the case studies (case studies 3, 4, and 5) are drawn from semiarid climates where annual rainfall ranges from around 400–700 mm and AI from 0.2 to 0.4. Because rainfall is much lower than PET during the entire year (Figure 3), WPE brings about only minor changes in evapotranspiration. At the same time, the higher vegetation cover resulting from WPE, in concert with recovery from historic overgrazing, has led to higher soil infiltrability. These conditions—an absence of overgrazing, sufficient rainfall to maintain vegetation cover in the intercanopy areas, and higher soil infiltrability—dramatically reduce lateral connectivity, which means lower surface runoff. In areas where subsurface vertical connectivity is poor, the consequence is lower streamflows, as seen from the South Texas Shrublands (Case Study 3) and the Rolling Plains (Case Study 5). In contrast, where subsurface connectivity is high, such as in the karst landscapes of the Edwards Plateau (Case Study 4), higher soil infiltrability means enhanced groundwater recharge and baseflows to streams and rivers. It is important to emphasize that in each of the ecoregions represented by these case studies, a gradual rebound from overgrazing took place concomitantly with WPE and there were strong interactions between the two. Together, they have contributed to increased vegetation cover on the landscape and higher soil infiltrability.
At the driest end of the climate gradient, where rainfall is less than 300 mm/yr and AI is around 0.1, WPE results in xerification. Intercanopy areas become bare and highly interconnected, leading to higher surface runoff. In areas where runoff is routed into highly transmissible stream channels (as in Case Study 6), groundwater recharge will be increased.
The concept of connectivity, then, provides a unifying framework for interpreting and making sense of findings that initially appear to be incongruent and inconsistent with respect to the ecohydrological effects of WPE. In drylands, changes in woody plant cover—by bringing about changes in evapotranspiration and/or soil infiltrability—fundamentally alter the ecohydrological connectivity of these landscapes. The directional change in connectivity can be lateral (overland flow, shallow subsurface flow) or vertical (deep drainage, groundwater recharge). The landscape-scale response to these changes will depend on the nature of the soil and rock substrate as well as the depth to groundwater.
Author contributions
BW Conceptualization and writing SB. Conceptualization, writing and figure preparation HO Conceptualization and writing and figure preparation PL. Conceptualization and writing.
Funding
This work was supported by USDA-NIFA awards 2019-68012-29819 and 2022-67019-36267.
Conflict of interest
The authors declare that the research was conducted in the absence of any commercial or financial relationships that could be construed as a potential conflict of interest.
Publisher’s note
All claims expressed in this article are solely those of the authors and do not necessarily represent those of their affiliated organizations, or those of the publisher, the editors and the reviewers. Any product that may be evaluated in this article, or claim that may be made by its manufacturer, is not guaranteed or endorsed by the publisher.
References
Acharya, B. S., Halihan, T., Zou, C. B., and Will, R. E. (2017a). Vegetation controls on the spatio-temporal heterogeneity of deep moisture in the unsaturated zone: A hydrogeophysical evaluation. Sci. Rep. 7, 1499. doi:10.1038/s41598-017-01662-y
Acharya, B. S., Hao, Y. H., Ochsner, T. E., and Zou, C. B. (2017b). Woody plant encroachment alters soil hydrological properties and reduces downward flux of water in tallgrass prairie. Plant Soil 414, 379–391. doi:10.1007/s11104-016-3138-0
Archer, S. R., Anderson, E. M., Predick, K. I., Schwinning, S., Steidl, R. J., and Woods, S. R. (2017). “Woody plant encroachment: Causes and consequences,” in Rangeland systems: Processes, management, challenges. Editor D. D. Briske (Cham, Switzerland: Springer), 25–84.
Archer, S. R., and Predick, K. I. (2014). An ecosystem services perspective on brush management: Research priorities for competing land-use objectives. J. Ecol. 102, 1394–1407. doi:10.1111/1365-2745.12314
Barger, N. N., Archer, S. R., Campbell, J. L., Huang, C. Y., Morton, J. A., and Knapp, A. K. (2011). Woody plant proliferation in North American drylands: A synthesis of impacts on ecosystem carbon balance. J. Geophys. Res. 116, G00K07. doi:10.1029/2010jg001506
Basant, S., Wilcox, B. P., Leite, P. M., and Morgan, C. L. (2020). When savannas recover from overgrazing, ecohydrological connectivity collapses. Environ. Res. Lett. 15, 054001. doi:10.1088/1748-9326/ab71a1
Berg, M. D., Marcantonio, F., Allison, M. A., Mcalister, J., Wilcox, B. P., and Fox, W. E. (2016). Contrasting watershed-scale trends in runoff and sediment yield complicate rangeland water resources planning. Hydrol. Earth Syst. Sci. 20, 2295–2307. doi:10.5194/hess-20-2295-2016
Bestelmeyer, B. T., Peters, D. P. C., Archer, S. R., Browning, D. M., Okin, G. S., Schooley, R. L., et al. (2018). The grassland-shrubland regime shift in the southwestern United States: Misconceptions and their implications for management. Bioscience 68, 678–690. doi:10.1093/biosci/biy065
Bochet, E., Rubio, J. L., and Poesen, J. (1999). Modified topsoil islands within patchy Mediterranean vegetation in SE Spain. Catena 38, 23–44. doi:10.1016/s0341-8162(99)00056-9
Bosch, J. M., and Hewlett, J. D. (1982). A review of catchment experiments to determine the effect of vegetation changes on water yield and evapotranspiration. J. Hydrology 55, 3–23. doi:10.1016/0022-1694(82)90117-2
Bracken, L. J., Turnbull, L., Wainwright, J., and Bogaart, P. (2015). Sediment connectivity: A framework for understanding sediment transfer at multiple scales. Earth Surf. Process. Landf. 40, 177–188. doi:10.1002/esp.3635
Bracken, L. J., Wainwright, J., Ali, G. A., Tetzlaff, D., Smith, M. W., Reaney, S. M., et al. (2013). Concepts of hydrological connectivity: Research approaches, pathways and future agendas. Earth-Science Rev. 119, 17–34. doi:10.1016/j.earscirev.2013.02.001
Bring, A., Fedorova, I., Dibike, Y., Hinzman, L., Mard, J., Mernild, S. H., et al. (2016). Arctic terrestrial hydrology: A synthesis of processes, regional effects, and research challenges. J. Geophys. Res. Biogeosci. 121, 621–649. doi:10.1002/2015jg003131
Calvo-Cases, A., Arnau-Rosalen, E., Boix-Fayos, C., Estrany, J., Roxo, M. J., and Symeonakis, E. (2021). Eco-geomorphological connectivity and coupling interactions at hillslope scale in drylands: Concepts and critical examples. J. Arid Environ. 186, 104418. doi:10.1016/j.jaridenv.2020.104418
Carlson, D. H., Thurow, T. L., Knight, R. W., and Heitschmidt, R. K. (1990). Effect of honey mesquite on the water balance of Texas Rolling Plains rangeland. J. Range Manag. 43, 491–496. doi:10.2307/4002351
Castello, L., Mcgrath, D. G., Hess, L. L., Coe, M. T., Lefebvre, P. A., Petry, P., et al. (2013). The vulnerability of Amazon freshwater ecosystems. Conserv. Lett. 6, 217–229. doi:10.1111/conl.12008
Caterina, G. L., Will, R. E., Turton, D. J., Wilson, D. S., and Zou, C. B. (2014). Water use of Juniperus virginiana trees encroached into mesic prairies in Oklahoma, USA. Ecohydrology 7, 1124–1134.
Cipriotti, P. A., and Aguiar, M. R. (2017). Biotic and abiotic changes along a cyclic succession driven by shrubs in semiarid steppes from Patagonia. Plant Soil 414, 295–308. doi:10.1007/s11104-016-3131-7
Cohen, M. J., Creed, I. F., Alexander, L., Basu, N. B., Calhoun, A. J. K., Craft, C., et al. (2016). Do geographically isolated wetlands influence landscape functions? Proc. Natl. Acad. Sci. U. S. A. 113, 1978–1986. doi:10.1073/pnas.1512650113
D'odorico, P., Laio, F., Porporato, A., Ridolfi, L., Rinaldo, A., and Iturbe, I. R. (2010). Ecohydrology of terrestrial ecosystems. BioScience 60, 898–907. doi:10.1525/bio.2010.60.11.6
D'odorico, P., Okin, G. S., and Bestelmeyer, B. T. (2012). A synthetic review of feedbacks and drivers of shrub encroachment in arid grasslands. Ecohydrology 5, 520–530. doi:10.1002/eco.259
Devitt, D. A., and Smith, S. D. (2002). Root channel macropores enhance downward movement of water in a Mojave Desert ecosystem. J. Arid Environ. 50, 99–108. doi:10.1006/jare.2001.0853
Eldridge, D. J., Bowker, M. A., Maestre, F. T., Roger, E., Reynolds, J. F., and Whitford, W. G. (2011). Impacts of shrub encroachment on ecosystem structure and functioning: Towards a global synthesis. Ecol. Lett. 14, 709–722. doi:10.1111/j.1461-0248.2011.01630.x
Good, S. P., Noone, D., and Bowen, G. (2015). Hydrologic connectivity constrains partitioning of global terrestrial water fluxes. Science 349, 175–177. doi:10.1126/science.aaa5931
Goodrich, D. C., Kepner, W. G., Levick, L. R., and Wigington, P. J. (2018). Southwestern intermittent and ephemeral stream connectivity. J. Am. Water Resour. Assoc. 54, 400–422. doi:10.1111/1752-1688.12636
Hoagland, B. W., Butler, I. H., Johnson, F. L., and Gleen, S. (1999). “The cross Timbers,” in Savannas, barrens, and rock outcrop plant communities of North America. Editors R. C. Anderson, J. S. Fralish, and J. M. Baskin (New York: Cambridge Press), 231–248.
Hoover, D. L., Bestelmeyer, B., Grimm, N. B., Huxman, T. E., Reed, S. C., Sala, O., et al. (2020). Traversing the wasteland: A framework for assessing ecological threats to drylands. Bioscience 70, 35–47. doi:10.1093/biosci/biz126
Huxman, T. E., Wilcox, B. P., Breshears, D. D., Scott, R. L., Snyder, K. A., Small, E. E., et al. (2005). Ecohydrological implications of woody plant encroachment. Ecology 86, 308–319. doi:10.1890/03-0583
Ilstedt, U., Tobella, A. B., Bazie, H. R., Bayala, J., Verbeeten, E., Nyberg, G., et al. (2016). Intermediate tree cover can maximize groundwater recharge in the seasonally dry tropics. Sci. Rep. 6, 21930. doi:10.1038/srep21930
Iwaniec, D. M., Gooseff, M., Suding, K. N., Johnson, D. S., Reed, D. C., Peters, D. P. C., et al. (2021). Connectivity: Insights from the US long term ecological research network. Ecosphere 12, 30. doi:10.1002/ecs2.3432
Jaeger, K. L., Olden, J. D., and Pelland, N. A. (2014). Climate change poised to threaten hydrologic connectivity and endemic fishes in dryland streams. Proc. Natl. Acad. Sci. U. S. A. 111, 13894–13899. doi:10.1073/pnas.1320890111
Johnson, J. C., Williams, C. J., Guertin, D. P., Archer, S. R., Heilman, P., Pierson, F. B., et al. (2021). Restoration of a shrub-encroached semi-arid grassland: Implications for structural, hydrologic, and sediment connectivity. Ecohydrology 14, 20. doi:10.1002/eco.2281
Keese, K. E., Scanlon, B. R., and Reedy, R. C. (2005). Assessing controls on diffuse groundwater recharge using unsaturated flow modeling. Water Resour. Res. 41. doi:10.1029/2004wr003841
Keesstra, S. D., Bagarello, V., Ferro, V., Finger, D., and Parsons, A. J. (2020). Connectivity in hydrology and sediment dynamics. Land Degrad. Dev. 31, 2525–2528. doi:10.1002/ldr.3401
Keesstra, S., Nunes, J. P., Saco, P., Parsons, T., Poeppl, R., Masselink, R., et al. (2018). The way forward: Can connectivity be useful to design better measuring and modelling schemes for water and sediment dynamics? Sci. Total Environ. 644, 1557–1572. doi:10.1016/j.scitotenv.2018.06.342
Lane, C. R., Leibowitz, S. G., Autrey, B. C., Leduc, S. D., and Alexander, L. C. (2018). Hydrological, physical, and chemical functions and connectivity of non-floodplain wetlands to downstream waters: A review. J. Am. Water Resour. Assoc. 54, 346–371. doi:10.1111/1752-1688.12633
Leibowitz, S. G., Wigington, P. J., Schofield, K. A., Alexander, L. C., Vanderhoof, M. K., and Golden, H. E. (2018). Connectivity of streams and wetlands to downstream waters: An integrated systems framework. J. Am. Water Resour. Assoc. 54, 298–322. doi:10.1111/1752-1688.12631
Leite, P. a. M., Wilcox, B. P., and Mcinnes, K. J. (2020). Woody plant encroachment enhances soil infiltrability of a semiarid karst savanna. Environ. Res. Commun. 2, 115005. doi:10.1088/2515-7620/abc92f
Li, J., Okin, G. S., Alvarez, L., and Epstein, H. (2007). Quantitative effects of vegetation cover on wind erosion and soil nutrient loss in a desert grassland of southern New Mexico, USA. Biogeochemistry 85, 317–332. doi:10.1007/s10533-007-9142-y
Ludwig, J. A., Wilcox, B. P., Breshears, D. D., Tongway, D. J., and Imeson, A. C. (2005). Vegetation patches and runoff-erosion as interacting ecohydrological processes in semiarid landscapes. Ecology 86, 288–297. doi:10.1890/03-0569
Maestre, F. T., Bowker, M. A., Puche, M. D., Hinojosa, M. B., Martinez, I., Garcia-Palacios, P., et al. (2009). Shrub encroachment can reverse desertification in semi-arid Mediterranean grasslands. Ecol. Lett. 12, 930–941. doi:10.1111/j.1461-0248.2009.01352.x
Marquart, A., Eldridge, D. J., Geissler, K., Lobas, C., and Blaum, N. (2020). Interconnected effects of shrubs, invertebrate-derived macropores and soil texture on water infiltration in a semi-arid savanna rangeland. Land Degrad. Dev. 31, 2307–2318. doi:10.1002/ldr.3598
Mishra, N. B., and Young, K. R. (2020). “Savannas and grasslands,” in The handbook of natural resources: Terrestrial ecosystems and biodiversity. Editor Y. Wang. 2nd ed (Taylor & Francis Group).
Moore, G. W., Barre, D. A., and Owens, M. K. (2012). Does shrub removal increase groundwater recharge in southwestern Texas semiarid rangelands? Rangel. Ecol. Manag. 65, 1–10. doi:10.2111/rem-d-11-00055.1
Mu, Q. Z., Zhao, M. S., and Running, S. W. (2011). Improvements to a MODIS global terrestrial evapotranspiration algorithm. Remote Sens. Environ. 115, 1781–1800. doi:10.1016/j.rse.2011.02.019
Okin, G. S., Moreno-De Las Heras, M., Saco, P. M., Throop, H. L., Vivoni, E. R., Parsons, A. J., et al. (2015). Connectivity in dryland landscapes: Shifting concepts of spatial interactions. Front. Ecol. Environ. 13, 20–27. doi:10.1890/140163
Peters, D. P. C., Bestelmeyer, B. T., Herrick, J. E., Fredrickson, E. L., Monger, H. C., and Havstad, K. M. (2006). Disentangling complex landscapes: New insights into arid and semiarid system dynamics. BioScience 56, 491–501. doi:10.1641/0006-3568(2006)56[491:dclnii]2.0.co;2
Peters, D. P. C., Groffman, P. M., Nadelhoffer, K. J., Grimm, N. B., Coffins, S. L., Michener, W. K., et al. (2008). Living in an increasingly connected world: A framework for continental-scale environmental science. Front. Ecol. Environ. 6, 229–237. doi:10.1890/070098
Qiao, L., Zou, C. B., Stebler, E., and Will, R. E. (2017). Woody plant encroachment reduces annual runoff and shifts runoff mechanisms in the tallgrass prairie, USA. Water Resour. Res. 53, 4838–4849. doi:10.1002/2016wr019951
Ratajczak, Z., Nippert, J. B., and Collins, S. L. (2012). Woody encroachment decreases diversity across North American grasslands and savannas. Ecology 93, 697–703. doi:10.1890/11-1199.1
Reid, K. D., Wilcox, B. P., Breshears, D. D., and Macdonald, L. (1999). Runoff and erosion in a pinon-juniper woodland: Influence of vegetation patches. Soil Sci. Soc. Am. J. 63, 1869–1879. doi:10.2136/sssaj1999.6361869x
Saco, P. M., Rodriguez, J. F., Moreno-De Las Heras, M., Keesstra, S., Azadi, S., Sandi, S., et al. (2020). Using hydrological connectivity to detect transitions and degradation thresholds: Applications to dryland systems. Catena 186, 104354. doi:10.1016/j.catena.2019.104354
Sala, O. E., and Maestre, F. T. (2014). Grass-woodland transitions: Determinants and consequences for ecosystem functioning and provisioning of services. J. Ecol. 102, 1357–1362. doi:10.1111/1365-2745.12326
Saleh, A., Wu, H., Brown, C. S., Teagarden, F. M., Mcwilliams, S. M., Hauck, L. M., et al. (2009). Effect of brush control on evapotranspiration in the North Concho River watershed using the eddy covariance technique. J. Soil Water Conservation 64, 336–349. doi:10.2489/jswc.64.5.336
Schlesinger, W. H., Reynolds, J. F., Cunningham, G. L., Huenneke, L. F., Jarrell, W. M., Virginia, R. A., et al. (1990). Biological feedbacks in global desertification. Science 247, 1043–1048. doi:10.1126/science.247.4946.1043
Schreiner-Mcgraw, A. P., Ajami, H., and Vivoni, E. R. (2019). Extreme weather events and transmission losses in arid streams. Environ. Res. Lett. 14. doi:10.1088/1748-9326/ab2949
Schreiner-Mcgraw, A. P., Vivoni, E. R., Ajami, H., Sala, O. E., Throop, H. L., and Peters, D. C. (2020). Woody plant encroachment has a larger impact than climate change on dryland water budgets. Sci. Rep. 10, 8112. doi:10.1038/s41598-020-65094-x
Schreiner-Mcgraw, A. P., and Vivoni, E. R. (2018). On the sensitivity of hillslope runoff and channel transmission losses in arid piedmont slopes. Water Resour. Res. 54, 4498–4518. doi:10.1029/2018wr022842
Starks, P. J., and Moriasi, D. N. (2017). Impact of Eastern redcedar encroachment on stream discharge in the North Canadian River basin. J. Soil Water Conservation 72, 12–25. doi:10.2489/jswc.72.1.12
Tetzlaff, D., Soulsby, C., Bacon, P. J., Youngson, A. F., Gibbins, C., and Malcolm, I. A. (2007). Connectivity between landscapes and riverscapes - a unifying theme in integrating hydrology and ecology in catchment science? Hydrol. Process. 21, 1385–1389. doi:10.1002/hyp.6701
Tobella, A. B., Reese, H., Almaw, A., Bayala, J., Malmer, A., Laudon, H., et al. (2014). The effect of trees on preferential flow and soil infiltrability in an agroforestry parkland in semiarid Burkina Faso. Water Resour. Res. 50, 3342–3354. doi:10.1002/2013wr015197
Torquato, P. R., Will, R. E., Zhang, B., and Zou, C. B. (2020). Stand-level transpiration increases after eastern redcedar (juniperus virginianaL.) encroachment into the midstory of oak forests. Forests 11.
Turnbull, L., and Wainwright, J. (2019). From structure to function: Understanding shrub encroachment in drylands using hydrological and sediment connectivity. Ecol. Indic. 98, 608–618. doi:10.1016/j.ecolind.2018.11.039
Turnbull, L., Wilcox, B. P., Belnap, J., Ravi, S., D'odorico, P., Childers, D., et al. (2012). Understanding the role of ecohydrological feedbacks in ecosystem state change in drylands. Ecohydrology 5, 174–183. doi:10.1002/eco.265
Veldman, J. W., Buisson, E., Durigan, G., Fernandes, G. W., Le Stradic, S., Mahy, G., et al. (2015). Toward an old-growth concept for grasslands, savannas, and woodlands. Front. Ecol. Environ. 13, 154–162. doi:10.1890/140270
Walvoord, M. A., and Kurylyk, B. L. (2016). Hydrologic impacts of thawing permafrost-A review. Vadose Zone J. 15, 010010. doi:10.2136/vzj2016.01.0010
Wang, J. E., Xiao, X. M., Qin, Y. W., Dong, J. W., Geissler, G., Zhang, G. L., et al. (2017). Mapping the dynamics of eastern redcedar encroachment into grasslands during 1984-2010 through PALSAR and time series Landsat images. Remote Sens. Environ. 190, 233–246. doi:10.1016/j.rse.2016.12.025
Wang, J., Xiao, X. M., Basara, J., Wu, X. C., Bajgain, R., Qin, Y. W., et al. (2021). Impacts of juniper woody plant encroachment into grasslands on local climate. Agric. For. Meteorology 307, 108508. doi:10.1016/j.agrformet.2021.108508
Wang, J., Xiao, X. M., Zhang, Y., Qin, Y. W., Doughty, R. B., Wu, X. C., et al. (2018b). Enhanced gross primary production and evapotranspiration in juniper-encroached grasslands. Glob. Chang. Biol. 24, 5655–5667. doi:10.1111/gcb.14441
Wang, J., Xiao, X., Qin, Y., Droughty, R. B., Dong, J., and Zou, Z. (2018a). Characterizing the encroachment of juniper forests into sub-humid and semi-arid prairies from 1984 to 2010 using PALSA and Landsat data. Remote Sens. Environ. 205, 166–179. doi:10.1016/j.rse.2017.11.019
Webb, N. P., Herrick, J. E., and Duniway, M. C. (2014). Ecological site-based assessments of wind and water erosion: Informing accelerated soil erosion management in rangelands. Ecol. Appl. 24, 1405–1420. doi:10.1890/13-1175.1
Weltz, M. A., and Blackburn, W. H. (1995). Water budget for south Texas rangelands. J. Range Manag. 48, 45–52. doi:10.2307/4002503
Wilcox, B. P., Birt, A., Archer, S. R., Fuhlendorf, S. D., Kreuter, U. P., Sorice, M. G., et al. (2018). Viewing woody-plant encroachment through a social–ecological lens. BioScience 68, 691–705. doi:10.1093/biosci/biy051
Wilcox, B. P., Breshears, D. D., and Allen, C. D. (2003). Ecohydrology of a resource-conserving semiarid woodland: Effects of scale and disturbance. Ecol. Monogr. 73, 223–239. doi:10.1890/0012-9615(2003)073[0223:eoarsw]2.0.co;2
Wilcox, B. P. (2007). Does rangeland degradation have implications for global streamflow? Hydrol. Process. 21, 2961–2964. doi:10.1002/hyp.6856
Wilcox, B. P., Fuhlendorf, S. D., Walker, J. W., Twidwell, D., Wu, X. B., Goodman, L. E., et al. (2022). Saving imperiled grassland biomes by recoupling fire and grazing: A case study from the Great Plains. Front. Ecol. Environ. 20, 179–186. doi:10.1002/fee.2448
Wilcox, B. P., Huang, Y., and Walker, J. W. (2008a). Long-term trends in streamflow from semiarid rangelands: Uncovering drivers of change. Glob. Change Biol. 14, 1676–1689. doi:10.1111/j.1365-2486.2008.01578.x
Wilcox, B. P., and Huang, Y. (2010). Woody plant encroachment paradox: Rivers rebound as degraded grasslands convert to woodlands. Geophys. Res. Lett. 37, L07402. doi:10.1029/2009gl041929
Wilcox, B. P., Owens, M. K., Dugas, W. A., Ueckert, D. N., and Hart, C. R. (2006). Shrubs, streamflow, and the paradox of scale. Hydrol. Process. 20, 3245–3259. doi:10.1002/hyp.6330
Wilcox, B. P., Sorice, M. G., Angerer, J., and Wright, C. L. (2012). Historical changes in stocking densities on Texas rangelands. Rangel. Ecol. Manag. 65, 313–317. doi:10.2111/rem-d-11-00119.1
Wilcox, B. P., Taucer, P. I., Munster, C. L., Owens, M. K., Mohanty, B. P., Sorenson, J. R., et al. (2008b). Subsurface stormflow is important in semiarid karst shrublands. Geophys. Res. Lett. 35, L10403. doi:10.1029/2008gl033696
Wilcox, B. P., Walker, J. W., and Heilman, J. L. (2010). Commentary on "Effect of brush control on evapotranspiration in the North Concho River watershed using the eddy covariance technique" by Saleh et al. (2009). J. Soil Water Conservation 65, 83A–84A. doi:10.2489/jswc.65.4.83a
Wilcox, B. P., Wilding, L. P., and Woodruff, C. M. (2007). Soil and topographic controls on runoff generation from stepped landforms in the Edwards Plateau of Central Texas. Geophys. Res. Lett. 34, L24S24. doi:10.1029/2007gl030860
Williams, C. J., Pierson, F. B., Kormos, P. R., Al-Hamdan, O. Z., Nouwakpo, S. K., and Weitz, M. A. (2019). Vegetation, hydrologic, and erosion responses of sagebrush steppe 9 Yr following mechanical tree removal. Rangel. Ecol. Manag. 72, 47–68. doi:10.1016/j.rama.2018.07.004
Wohl, E. (2017). Connectivity in rivers. Prog. Phys. Geogr. Earth Environ. 41, 345–362. doi:10.1177/0309133317714972
Wood, M. K., Blackburn, W. H., Eckert, J., and Peterson, F. F. (1978). Interrelations of the physical properties of coppice dune and vesicular dune interspace soils with grass seedling emergence. J. Range Manag. 31, 189–192. doi:10.2307/3897177
Wu, G. L., Cui, Z., and Huang, Z. (2021). Contribution of root decay process on soil infiltration capacity and soil water replenishment of planted forestland in semi-arid regions, 404.Geoderma
Yahdjian, L., Sala, O. E., and Havstad, K. M. (2015). Rangeland ecosystem services: Shifting focus from supply to reconciling supply and demand. Front. Ecol. Environ. 13, 44–51. doi:10.1890/140156
Zou, C. B., Caterina, G. L., Will, R. E., Stebler, E., and Turton, D. (2015). Canopy interception for a tallgrass prairie under juniper encroachment. Plos One 10, e0141422. doi:10.1371/journal.pone.0141422
Zou, C. B., Qiao, L., and Wilcox, B. P. (2016). Woodland expansion in central Oklahoma will significantly reduce streamflows – A modelling analysis. Ecohydrology 9, 807–816. doi:10.1002/eco.1684
Zou, C. B., Turton, D. J., Will, R. E., Engle, D. M., and Fuhlendorf, S. D. (2014). Alteration of hydrological processes and streamflow with juniper (Juniperus virginiana) encroachment in a mesic grassland catchment. Hydrol. Process. 28, 6173–6182. doi:10.1002/hyp.10102
Keywords: ecohydrology, rangelands, runoff, groundwater recharge, dryland
Citation: Wilcox BP, Basant S, Olariu H and Leite PAM (2022) Ecohydrological connectivity: A unifying framework for understanding how woody plant encroachment alters the water cycle in drylands. Front. Environ. Sci. 10:934535. doi: 10.3389/fenvs.2022.934535
Received: 02 May 2022; Accepted: 31 August 2022;
Published: 28 September 2022.
Edited by:
Lindsay Beaumont Hutley, Charles Darwin University, AustraliaReviewed by:
Mohd Yawar Ali Khan, King Abdulaziz University, Saudi ArabiaMandy Slate, University of Colorado Boulder, United States
Copyright © 2022 Wilcox, Basant, Olariu and Leite. This is an open-access article distributed under the terms of the Creative Commons Attribution License (CC BY). The use, distribution or reproduction in other forums is permitted, provided the original author(s) and the copyright owner(s) are credited and that the original publication in this journal is cited, in accordance with accepted academic practice. No use, distribution or reproduction is permitted which does not comply with these terms.
*Correspondence: Bradford P. Wilcox, YndpbGNveEB0YW11LmVkdQ==