- 1Discovery Bay Marine Laboratory-UWI, Queens Highway, Discovery Bay, Jamaica
- 2Centre for Marine Sciences, 1 Anguilla Close, University of the West Indies, Mona, Jamaica
- 3Port Royal Marine Laboratory-UWI, Port Royal, Jamaica
- 4Centre for Novel Agricultural Products (CNAP), Department of Biology, University of York, Heslington, YO, United Kingdom
- 5Department of Life Sciences, 2 Anguilla Close, University of the West Indies, Mona, Jamaica
The Sargassum spp. inundations across the Atlantic and Caribbean that began in 2011 have continued unabated, and new uses for the biomass are being continuously explored. Mangroves protect shorelines, store carbon, enhance water quality, and promote biodiversity. Their restoration can be hindered by poor soils associated with urbanized coastlines. Sargassum spp. application in the form of mulch, compost, and plant tonics has yielded positive results in a range of plants. As part of transforming the inundations to benefit communities, Sargassum spp. compost (SC) was assessed in mangrove seedling production for restoration. Pure SC was mixed with soil/sand medium, as different treatments, for the production of Rhizophora mangle seedlings in “wet” and “dry” nurseries. Plants in the “wet” nursery performed poorly, with 90–100% of plants in 50 and 100% SC, respectively, dying after 6 weeks. Seedlings in all SC treatments in the “dry” nursery survived with obvious and statistically significant treatment differences. Height and number of leaves indicated the best growth in the 75% SC treatment, while the control (0% SC) had the poorest growth. Seedling health, greatest in the control, was poorest in 50 and 100% SC. Elemental analysis of SC, seedlings, and soil/sand medium indicated that several elements (Na, K, Ca, As, and Se) found in high concentrations in the SC were low in the plants. Overall, low sequestration of elements by mangrove seedlings and the reported ability of mangrove soils to reduce element mobilization through chelation indicate the potential use of Sargassum spp. in soil amelioration for mangrove restoration without proportional contamination of the ecosystem. We see the potential use of nuisance Sargassum spp. blooms to support mangrove restoration, leading to increased benefits to coastal communities being affected by the inundations.
1 Introduction
Large floating mats of pelagic sargassum seaweed, comprising Sargassum natans I, S. natans VIII, and S. fluitans III (Schell et al., 2015), have been inundating shorelines across the Caribbean since 2011 (Wang et al., 2019). There is evidence that these annual blooms will continue into the foreseeable future, becoming the “new normal” (Wang et al., 2019; Salter et al., 2020; Machado et al., 2022). Although widely considered a nuisance (van Tussenbroek et al., 2017), many have come to regard the algal biomass as a resource (Milledge and Harvey, 2016; Chávez et al., 2020; Amador-Castro et al., 2021) with a plethora of projects exploring uses, which include soil amelioration (Desrochers et al., 2020). The effectiveness of Sargassum spp. in improving plant production (applied as mulch, compost, or liquid fertilizer) has been shown for several food crops (CARDI, 2015). However, there is a threat of increasing the salinity of the soil (CARDI, 2015), and although this may be ameliorated by washing the Sargassum spp. before and during composting (Eyras et al., 1998), this measure can be too expensive for some communities and also leaches nutrients (CARDI, 2015).
While there are examples of the use of Sargassum spp. seaweed for the restoration of dunes (Desrochers et al., 2020) and to enhance the actual growth of dune plants (Williams and Feagin, 2010; Thompson et al., 2020), there is no previous research study on the use of sargassum seaweed in mangrove restoration or rehabilitation. It is hypothesized that mangroves and other coastal plant species growing in poor soil conditions will benefit from the soil amelioration properties of Sargassum spp. (improved texture, water holding capacity, and increased nutrients), and it is also expected to be able to tolerate increased soil salinity. The production of compost from pelagic Sargassum spp. by mixing it with other plant materials (wood chips and food waste) has been reported (Sembera et al., 2018). However, there is yet no example of composting pure Sargassum spp., which would make use of larger quantities of the feedstock and facilitate an assessment of the effect of the pure compost on coastal plant species such as mangroves.
Mangrove ecosystem services are well known and include shoreline protection, water quality improvement, biodiversity support through the provision of a range of habitats, and carbon sequestration. Since 2006, over 200 benefits of mangroves have been documented (Lee et al., 2014) and later grouped into regulating, supporting, provisioning, and cultural ecosystem services (Kathiresan, 2012; Uddin et al., 2013; Webber et al., 2016; Mitra et al., 2018). There has also been increasing global recognition of mangrove importance in carbon sequestration (Alongi, 2012; Sandeman et al., 2018), with mangroves showing high rates of carbon capture and almost permanent storage in the forest sediment and living biomass of leaves, stems, and roots (Donato et al., 2011; Alongi, 2014).
Despite the ecological, economic, cultural, and climate regulation value of mangroves, these forests are being destroyed globally at rates of 1—2% per year (Giri et al., 2008; Hogarth, 2015); such that mangrove cover has declined by 35% over the last 2 decades due to human activities (Krauss et al., 2008). Mangrove forests have been threatened by mariculture (primarily shrimp farming) (Giri et al., 2008), excessive timber harvest (Kairo et al., 2001), deforestation for development (Valiela et al., 2001), hydrology changes (Turner and Lewis, 1997), and rising sea levels which force mangroves to recede to unsuitable land margins (Webber et al., 2016).
As is the case with many developing countries, Jamaica has lost almost 20% of mangroves in the last decade due to coastal development, land reclamation, and extreme weather events (Trench, 2021). Mangrove rehabilitation efforts have had increasing success but, in some cases, require severe interventions such as sediment replacement and slope reconstruction to restore hydrology as well as replanting of nursery-reared (hardened) seedlings (Nguyen et al., 2016; Trench, 2021). In some cases, the sediments used for mangrove rehabilitation sites are riverine sand, which does not provide high levels of organic matter as is naturally found in mangrove forests (Reef et al., 2010).
Urbanized coastlines (e.g., the shores of Kingston Harbor, Jamaica) provide a good example of mangrove areas that have experienced severe habitat modification and have little chance of restoration being facilitated by the natural recruitment of seedlings from adjacent forests. Such shorelines are said to be “propagule limited” (Lewis, 2009) and often require extensive replanting using nursery-reared seedlings for restoration efforts to succeed. Furthermore, soil conditions in such areas are often so altered that there is a need for sediment replacement or substantial amelioration for the introduced plants to survive. It was hypothesized that nursery propagation and out-planting of seedlings for severely altered urbanized areas may be enhanced by the use of natural soil ameliorants such as sargassum seaweed. This would also make use of the algal biomass associated with the pelagic Sargassum spp. inundations.
Rhizophora is the most common mangrove genus along Atlantic coasts (Cerón-Souza et al., 2021), and R. mangle is the most commonly used and easily propagated mangrove. R. mangle, which usually grows at the seaward edge of the forest, has been shown to be the most productive of the coastal mangrove species and has great ecosystem services due to the sheltered nursery-habitat (Mandal et al., 2019) created by its prop-roots hanging into the adjacent lagoon. In this context, our work aimed to determine whether R. mangle seedlings showed enhanced growth and optimal health with the use of pure Sargassum spp. compost (SC) under nursery conditions. The use of pure SC would also facilitate the identification of contaminants reported to be associated with pelagic Sargassum spp. blooms (Milledge et al., 2016; Chávez et al., 2020), in particular arsenic (Davis et al., 2021; Tonon et al., 2022).
2 Materials and Methods
2.1 Compost Production
Pure Sargassum spp. compost (SC) was produced by storage of fresh unwashed and unsorted Sargassum spp. from which all non-sargassum plants and debris had been removed. The sargassum biomass was collected from near-shore waters using a surf net at two locations in Jamaica: Hellshire coastline, St Catherine, and Fort Rocky, Palisadoes coastline (Kingston) in the summer months of 2019 and 2020, respectively. Care was taken to ensure that the collected material was free of beach sand. The Sargassum spp., which is consistently comprised of a mix of S. fluitans III, S. natans I, and S. natans VIII (Schell et al., 2015; Machado et al., 2022) in descending order of biomass, was placed in large black plastic bags which were stored unsecured (allowing air through but not rainwater) in an earthen pit for 3 months. The material was periodically ‘turned’ to effect mixing. The highest temperature recorded from the composting material during the day was ∼36°C.
2.2 Propagule Establishment
Similar-sized R. mangle propagules collected from mangrove forests along the north coast of Jamaica were soaked in fresh water for 24 h, measured and weighed, and then planted in a soil/sand mixture (in approximately 50:50 ratio) used as a potting medium in the mangrove nursery. Mangrove propagules (ten per treatment for wet nursery experiment and three per treatment for dry nursery experiment) were planted to the same depth in potting bags containing SC, soil/sand, or a mix based on the following five treatments: A- 100% SC; B- 75% SC with 25% soil/sand; C- 50% SC and soil/sand; D- 25% SC and 75% soil/sand; and E- 0% SC (a soil/sand only control).
The experiments were conducted at a mangrove nursery located at the Discovery Bay Marine Laboratory, north coast, Jamaica, in successive years for wet and dry nursery experiments. The wet nursery experiments were conducted in September 2019 and only maintained for approximately 6 weeks due to the death of all plants in selected treatments. The experiment was repeated under ‘dry’ nursery conditions approximately 1 year later.
During “wet” nursery conditions, the plants were exposed to normal seawater (35 psu) or brackish water salinity of 20 psu. The water level in the nursery enclosures was altered on 12 h cycles, and the enclosures in which the plants were kept were completely drained for 12 h before replacing the water for another 12 h. Thus, diurnal tides were simulated. Water levels were just enough to cover the soil during the “high tide”. Treatments with different SC and soil/sand ratios were segregated and kept in different enclosures in the ‘wet’ nursery so as not to have leachate from one treatment influencing another.
For the “dry” nursery experiment, no “tidal” fluctuations were introduced; however, plants were watered from above using a sprinkler type set up twice per day (06:30 and 17:00). Treatments were also segregated and kept far enough apart in the nursery so as not to have the leachate mixing across treatments.
The dry nursery experiment was established in September 2020 and terminated in June 2021 (40 weeks), and plants in all treatments were alive up to the end of the experiment. Seedlings were assessed each week for the number of leaves, number of nodes, height above soil level, and health status. Height, nodal distances, leaves, and biomass have proved to be useful mangrove seedling parameters (Gab-Alla et al., 2003; Hoppe-Speer et al., 2011). The health index was developed for our assessments to facilitate having an objective indication of seedling condition considering the potentially deleterious effects of the Sargassum spp. The health index was based on a numeric scale of 0—5 as follows:
0- Dead, no leaves, no green areas on stem, stem withered;
1- Surviving (plant has green color on stem) but no leaves;
2- Surviving, plant with few withered leaves, pale or yellow with curling, scarring, or spotting of leaves;
3- Poor health, possessing few leaves with evidence of thinning, and pale or yellow;
4- Good health, leaves not fully green but succulent (not thin or curled);
5- Best health possible, leaves fully green and succulent.
2.3 Elemental Analysis Using Inductively Coupled Plasma Mass Spectrometry (ICP-MS)
At the end of the dry nursery experiment, R. mangle seedlings were removed from the soil, thoroughly washed with fresh water, and sun-dried before roots and leaves were separated from the stems and ground separately for elemental analysis. Sun-drying was carried out over 48 h at temperatures between 30 and 35°C during the day and 20–24°C at night. Stems were not analyzed. Samples of the pure SC and the soil/sand potting medium (n = 3) were also sun-dried for elemental analysis, as indicated for the mangrove seedlings. All samples (∼0.2 g) were digested in a CEM MARS6 microwave digestion system in 20 ml Xpress vessels using concentrated sub-boiled nitric acid at 200°C for 10 min (CEM standard Xpress procedure for plant material). Following digestion, the samples were diluted with Milli-Q water to form an ∼ 20 ml mother solution and then subsampled to obtain an ∼ 1000x total dilution. The daughter samples were spiked to obtain a final concentration of 5 ppb for In and Re to act as internal standards. Standards were made from the Inorganic Ventures Environmental standard (IV-Stock-50) and were spiked to obtain 5 ppb In and Re final concentrations. The samples were analyzed on an Agilent 8900 QQQ-ICP-MS in standard He and O2 modes, depending on the element of interest.
2.4 Statistical Tests
Statistical tests were conducted using SPSS Version 22 to assess if there was a significant difference in plant parameters across the different treatments. p < 0.05 was accepted as significant for all parameters. Shapiro–Wilks analysis was used to test normality amongst all the parameters, and due to the assumptions of the data, where normality was achieved (number of leaves and number of nodes), the one-way ANOVA test of variance was conducted. Kruskal–Wallis analysis was used where normality was not achieved (height and health ranking). Kruskal–Wallis analysis was also used to assess significant differences in element concentration (in ppm) between treatments for roots and shoots, pure SC and soil/sand medium. Principal component analysis was used to group the 25 elements based on concentrations across treatments, pure SC and soil/sand medium.
3 Results
3.1 “Dry” Nursery Experiment
After 40 weeks, median height (p < 0.001), number of leaves (p = 0.045), and seedling health (p < 0.001) were found to vary significantly between treatments (Supplementary File S1). Height (Figure 1) indicated that the best seedling growth occurred in the 75% Sargassum spp. compost (SC) treatment, while the poorest growth was from the control (0% SC), followed by seedlings in 100% SC.
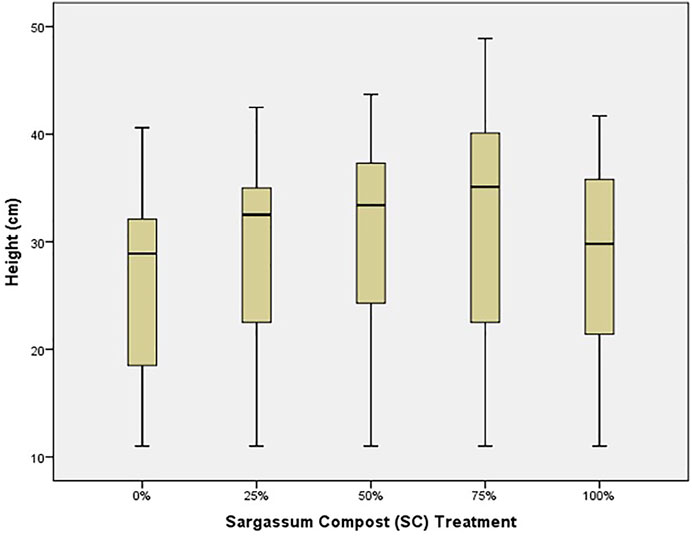
FIGURE 1. Median seedling height (bar), 25–75% range (box), and non-outlier range (whisker) for red mangrove seedlings in each treatment, indicating the % Sargassum spp. compost (SC).
Similarly, the number of leaves was greatest for seedlings in 75% SC treatments (Figure 2), and by the end of the experiment, lateral branching was observed only in that treatment. Seedling health index (Figure 3) was highest in the control and poorest in 100% SC, followed by the 50% SC treatment, which also showed poor health (comparable to 100% SC treatment). This was indicated by the curling of the leaves and dark spots along with yellowing/loss of color (Figure 4). Leaves also appeared thin (loss of succulence). The number of nodes did not vary significantly between treatments (p = 0.860).
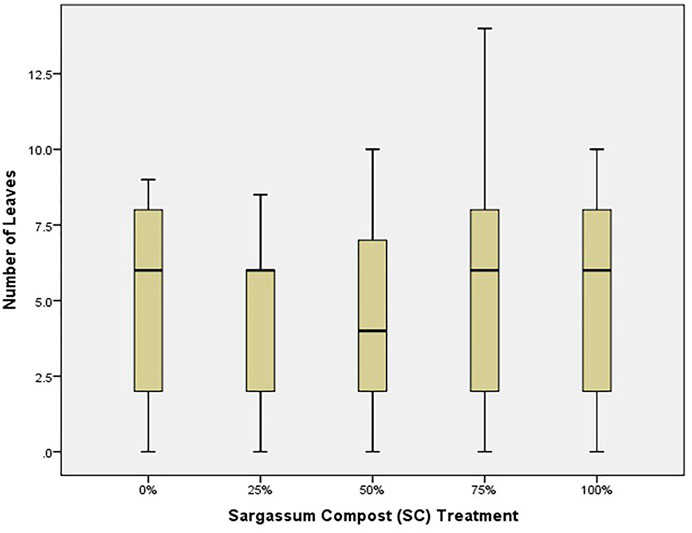
FIGURE 2. Median number of leaves (bar), 25–75% range (box), and non-outlier range (whisker) for red mangrove seedlings in each treatment, indicating the % Sargassum spp. compost (SC).
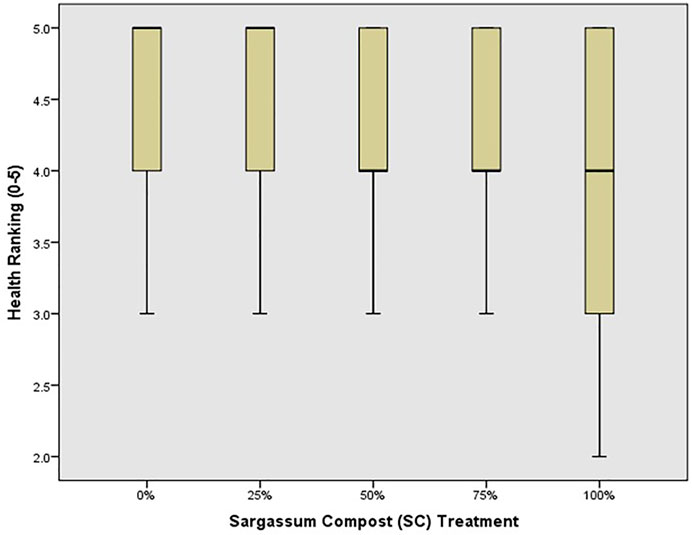
FIGURE 3. Median health rank (bar), 25–75% range (box), and non-outlier range (whisker) for red mangrove seedlings in each treatment, indicating the % Sargassum spp. compost (SC).
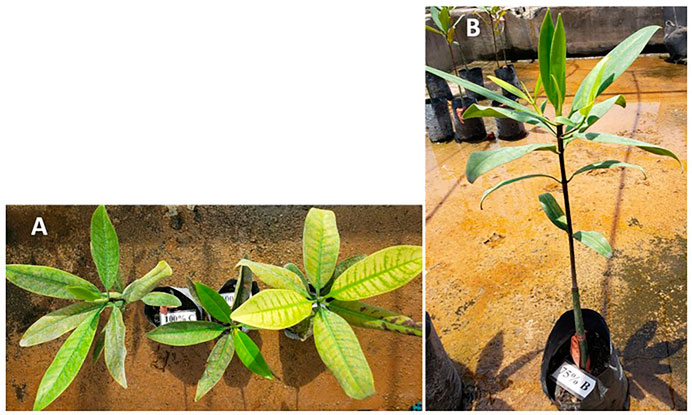
FIGURE 4. R. mangle seedlings showing leaf damage after growth in 100% SC (A) and seedlings in 75% SC showing lateral branching (B). Lateral branching observed at the end of the experiment.
3.2 “Wet” Nursery Experiments
All seedling parameters assessed in the wet nursery varied significantly between SC treatments (height, p < 0.001; number of leaves, p < 0.001; number of nodes, p < 0.001; health index, p < 0.001) (Supplementary File S2). However, the most noteworthy finding was the rapid demise of the plants under “wet” nursery conditions. Seedling survival in the “wet” nursery was especially poor for treatments of 100% and 50% SC. Complete loss of leaves and presumed death of the plants was seen after 3 weeks (Table 1). One plant of ten subsequently recovered in the 50% treatment, but there was no recovery of plants in the 100% treatment after 6 weeks. The control (0% SC), by contrast, showed 90% seedling survival.

TABLE 1. Mangrove seedling survival in ‘wet’ nursery conditions showing the death of the plants (0% survival) by week 3 in 100% Sargassum spp. compost (SC). The experiment was terminated after week 6 when one treatment had death of all plants.
The growth parameters (height, number of leaves, and number of nodes) and health rank assessed in the surviving ‘wet’ nursery plants showed a similar pattern across treatments. The number of leaves, number of nodes, and health were the greatest in the control (0% SC), while the 50 and 100% SC treatments showed the lowest values (Figure 5). Height was again similar with 50% and 100% SC treatments, showing the poorest growth, while plants in 75% SC treatment showed the greatest values (similar to the control and the 25% SC treatment).
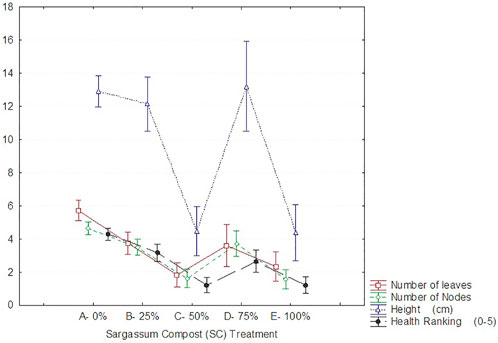
FIGURE 5. Change in seedling parameters; number of leaves, number of nodes, height, and health (means with ±95% CI) across SC treatment in the “wet” nursery averaged for all weeks.
3.3 Elemental Analysis
Elemental analysis of seedlings after the dry nursery experiments was reported for 25 elements (Table 2). Six elements (Na, Mg, K, Ca, As, and Se) found in high concentrations in the SC had minimal concentrations in the plants (roots or leaves) (Supplementary Figure S1). Most of the elements (Be, Al, V, Cr, Ni, Co., Mn, Fe, Ag, Ba, Pb, Th, and U) had high concentrations in the soil/sand medium compared to the SC; however, these were still low in the plants (Supplementary File S3). Most elements (19 of 25) displayed a general pattern of greater mean concentrations in the roots than in the leaves, while only four (Mg, Ca, Mn, and Ti) were higher in the leaves.
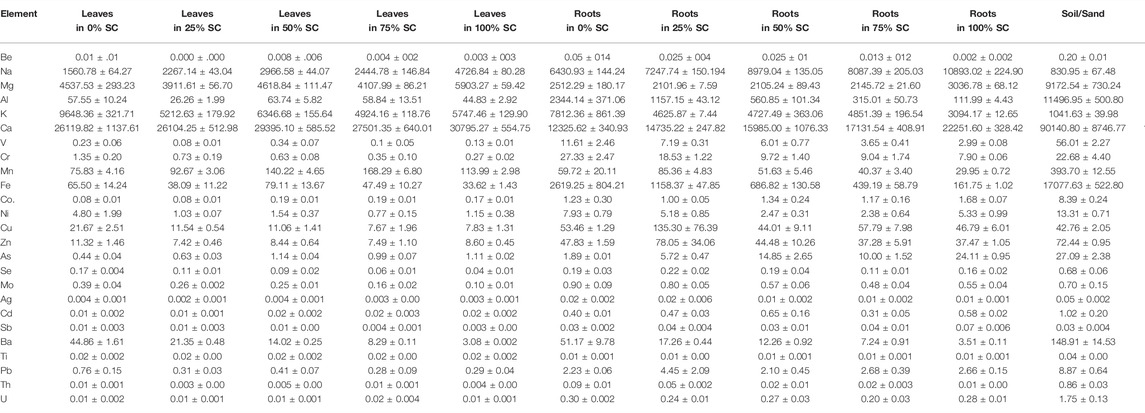
TABLE 2. Element concentration (mean with S.E.) in parts-per-million (ppm) for plant sections in all SC treatments, pure SC, and soil/sand potting mix.
Elements of interest, As and Na, had the greatest mean concentrations in the pure SC (202.43 ppm and 50,676.21 ppm, respectively); however, concentrations of these were much lower in the R. mangle seedlings (Table 2). Arsenic (As) concentrations in the R. mangle seedlings were less than 4% of concentrations in the SC for all except roots grown in 100% SC (which had 11.9% of As concentration in the SC). For Na, mean concentrations in the R. mangle seedlings grown in 100% SC were all lower than 17.7% of the SC concentration, except for roots in 100% SC, which had mean Na concentrations of 21.5% of the SC concentration.
Principal component analysis (PCA) indicated four groups (Figure 6). PCA also confirmed the groupings by the inclusion of elements found high in the pure SC (Mg, Na, K, Ca, As, and Se) in one cluster group. Ni, which was high in the soil/sand medium, low in SC, and with varied concentrations across R. mangle roots and leaves, was identified by the PCA as an outlier.
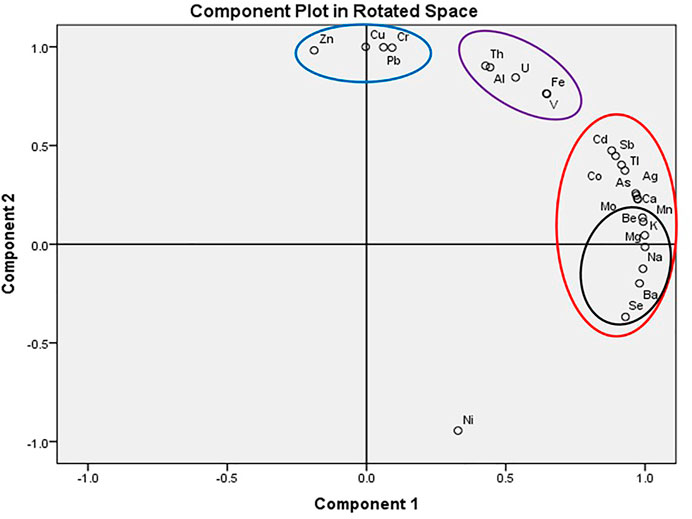
FIGURE 6. Principal Component analysis (PCA) showing grouping of elements according to concentrations in ppm.
4 Discussion
Since the Sargassum spp. influx across the Caribbean began in 2011, these algae have been assessed for efficacy in crop production with an indication of success; in particular, improved crop productivity and resistance against pests and harsh environmental conditions (CARDI, 2015; Desrochers et al., 2020). Mohanty et al. (2013) and Walsh (2019) used Sargassum spp. in compost form and examined its production for agriculture and horticulture applications. Liquid Sargassum spp. extract has also been used in foliar applications to achieve significant growth (Miranda et al., 2021). Pelagic Sargassum spp. have been shown to be rich in macro and micronutrients (Davis et al., 2021; Machado et al., 2022; Tonon et al., 2022), and so the production of commercial fertilizer from Sargassum spp. is well-established (Desrochers et al., 2020). Interestingly, the use of Sargassum spp. for mangrove restoration has not been previously explored. This is particularly relevant as we seek to achieve successful mangrove rehabilitation in urbanized areas which have less than ideal sediment conditions, and we saw the need to explore the utility of Sargassum for this application.
4.1 Impact of Sargassum spp. Compost (SC) on Mangrove Seedlings Under Different Nursery Conditions
The wet nursery results clearly demonstrated that the use of high concentrations of SC (100%) combined with waterlogged conditions resulted in very poor outcomes, quickly affecting plant survival. Rotting of the roots was observed concomitantly with the presence of a strong smell of hydrogen sulphide (H2S) gas. Rotting Sargassum spp. has been reported to produce H2S gas, which has resulted in a decline in natural mangrove and seagrass habitats (Oxenford et al., 2021). The finding of survival in the 50% SC being similarly poor to that of the 100% SC treatment is difficult to explain. The height of plants in 50% SC was also as low as in 100%, while 75% compost in the wet nursery yielded the greatest heights. The value of the ‘wet’ nursery experiments, however, was to demonstrate the deleterious effect of SC in water-logged soils and a similar propensity for H2S production as was observed with rotting of fresh Sargassum spp. in coastal areas.
The positive response of red mangrove seedlings to 75% SC treatment in the dry nursery was best indicated by changes in seedling height. The gradual change in median height from the maximum in the 75% SC along the decreasing treatment percentages (50%, followed by 25% and then the control) indicated the positive effect of Sargassum spp. on R. mangle seedling growth. Growth in pure SC (100% treatment) was low, being marginally higher than the control, and was accompanied by the poorest health, showing that pure SC is deleterious to plant health and growth. There were indications of high concentrations of growth-inhibiting substances or other constituents that have deleterious effects on the growth and health of the plants in the undiluted compost. Several authors have reported the occurrence of heavy metals and substances such as phenols (sometimes in high concentrations) from Sargassum spp. (Milledge et al., 2016; Milledge and Harvey, 2016). Arsenic, in particular, has been measured from fresh and dried Sargassum spp. at concentrations above the accepted EPA values and with a dominance of the highly toxic inorganic form (Milledge et al., 2016; Davis et al., 2021; Tonon et al., 2022). The inhibitory effects from the use of the 100% SC treatment would support the recommendation that the SC be mixed with other plant material (Milledge et al., 2016) or used in lower concentrations with soil mixes. The use of 25–50% Sargassum spp. in compost trials by Walsh (2019) achieved good plant growth and low arsenic levels (4.2–7.2 ppm in the plants), which are within the EPA standards for such activity (Desrochers et al., 2020).
The experiment facilitated the assessment of the growth (and health) parameters normally used to assess seedlings within a mangrove nursery or after out-planting (Trench 2021). The effect of Sargassum spp. on the number of leaves was not as clear as seen for plant height in the dry nursery experiments. Leaf production and retention was, however, noticeably best in the 75% SC treatment, while the 50% treatment was the poorest. Currently, we do not have any clear explanation for the observed responses in 50% SC. Mangroves, especially the Rhizophora spp., have been reported to show necrosis and leaf loss (as well as low leaf production) in response to increasing and above-normal salinity (Aslam et al., 2011) and to other chemical stressors (Proffit and Develin, 1998). Other effects, such as leaf thickening (succulence), have also been reported for Rhizophora spp. grown in high salt conditions (Parida and Jha, 2010). While the leaf damage observed in our experiment was expected, the occurrence of thinning of the leaves (reduced thickness or loss of succulence), especially in the 100% treatment and in the 50% SC, would suggest that the plants were not responding to salt stress (Parida and Jha, 2010). The responses may be due to the effect of other (chemical) stressors. Heavy metals and other toxins (e.g., insecticides such as chlordecone) which are sequestered by Sargassum spp. after prolonged periods in the environment (Devault et al., 2022) could be toxic to the plants in high compost concentrations. Hence, the need for elemental analysis of the plants, compost, and soil used as a potting medium.
4.2 Partitioning of Elements Between Soil/Sand Medium, Sargassum spp. Compost, and Mangrove Seedlings
Our findings of high elemental concentrations associated with the soil/sand support the idea that mangrove sediments may act as an efficient metal trap (Silva et al., 1990; Saher and Asmat, 2017). Several researchers have also indicated that mangrove sediments can be the main reservoir for heavy metals when compared to the living biomass, the latter accounting for less than 1% of what was retained in the sediments. Others have reported that mangrove plants, especially Rhizophora spp., due to ion exchange at the roots, can actually regulate heavy metal uptake (Alongi et al., 2004; MacFarlane and Burchett, 2002; MacFarlane et al., 2007. The combined effect may account for the low elemental concentrations we observed in the plants.
We expected that seedling roots would have greater elemental concentrations than leaves based on direct contact with the source, and our results did indicate that, generally, mangrove seedling roots had higher concentrations of elements than the leaves. Mangroves are reported to be resistant to heavy metals (Machado and Lacerda, 2004) and tolerance, especially in R. mangle, as has been suggested by Walsh et al. (1979), due to the formation of non-toxic sulfides in the root or at the root surface. The plants may also employ detoxification at the tissue level and ion-exclusion at the roots, which is part of the same salt-exclusion mechanism (Medina, 1999) or a combination of these factors. Metal exclusion is therefore strongly suggested but also detoxification at the tissue level due to high tissue residues without proportional effect on the plant (Walsh et al., 1979). This was also seen in our experiments where for some elements in high concentrations in the soil/sand potting medium and with high root uptake in the control treatment, low concentrations were found in the leaves, and there was no obvious compromise to plant health. Furthermore, we observed that the only elements found to be higher in leaves than roots tended to be those naturally associated with plant leaves (e.g., Mg, Ca, and Mn).
Of the elements found to be high in pure SC (and low in plants), Na and As were deemed of concern because of their abundance in naturally occurring Sargassum spp. and their potential to cause poor outcomes and plant damage. While sodium and magnesium are common seawater elements (Cerón-Sousa et al., 2021), sodium was important to consider because salt stress can be very damaging even to coastal plants. According to Aslam et al. (2011), no toxic substance restricts plant growth more than excess salt. Mangrove seedlings have shown leaf damage in persistent hypersalinity (Krauss et al., 2008), a condition under which stunting and poor growth (sometimes leading to death) of mangroves has also been observed (Drexler and Ewel, 2001; Barreto, 2008). Arsenic is another element of concern because of its toxicity, potential to cause environmental contamination (Rodríguez-Martínez et al., 2020), and the fact that it has been consistently reported in high concentrations in pelagic Sargassum spp. associated with the blooms (Desrochers et al., 2020; Davis et al., 2021).
4.2.1 Sodium Contamination
Mangrove plants are said to be facultative halophytes, meaning they do not need to grow in saline soils and are found in areas with both high and fluctuating salinities (Parida and Jha, 2010). They employ various mechanisms to tolerate saline environments, including exclusion of salt at the roots, excretion of salts (usually by the leaves), and some tolerance of relatively high salt concentration in the tissues (Hogarth, 2015). Rhizophora spp. have concentric layers of hypodermal and endodermal cells (called a double-endodermis) in the roots that act as a barrier (Aslam et al., 2011). This genus is also known to move salt into older leaves which are then shed (Hoppe-Speer et al., 2011). Despite these mechanisms, Rhizophora is not able to survive in hypersaline soils and prefers the margins of the forest where a range from normal seawater (35 psu) to hyposaline and tidal flushing are experienced (Hoppe-Speer et al., 2011). The natural mangrove environment, however, is dynamic and species, therefore, tend to be tolerant to a range of conditions even for a short time. In less-than-ideal situations, these species may exhibit slower growth or appear less ‘healthy’ rather than be eliminated (Krauss et al., 2008). In the case of hypersalinity or high sodium, mangroves may also expend a lot of energy to remove salt, using large amounts of “photosynthetic energy” (Medina, 1999), exhibiting slower growth.
4.2.2 Arsenic Contamination
Holopelagic Sargassum spp., like many brown seaweeds, contain concerning concentrations of arsenic (Rodríguez-Martínez et al., 2020). In our experiments, the poor health of the seedlings in 100% SC treatment was thought to be due to increased levels of contaminants such as arsenic. The indicators of poor health, which included spotting (brown), curling, and yellowing of the leaves, were very evident in the 100% Sargassum spp. compost plants. However, while elemental analysis indicated the greatest mean As concentration (202.43 ppm) in the pure SC, the only plant tissue with concentrations greater than 20% (24.1 ppm) was the roots of seedlings grown in the 100% pure SC treatment. This indicates the lack of uptake and sequestration by the R. mangle seedlings and the possible utility of Sargassum spp. in nursery propagation of this plant. Furthermore, application on a wider scale (out-planting) may have positive outcomes.
5 Application
Collecting and drying or composting of Sargassum spp. in tropical coastal communities with high insolation (and low rainfall) is a relatively low-cost activity that produces material with potential for several applications. In this context, composting has the greatest potential for soil amelioration as both the properties (texture and water holding capacity) and nutrient content of the soil or sand are enhanced (Pan et al., 2012; Adugna, 2016). Desrochers et al. (2020) have indicated that using seaweed as compost in coastal areas is one of the simplest, cheapest, and most practical methods of making use of the large algal biomass. In line with this, our experiments show that in appropriate proportions, pure SC does enhance mangrove seedling growth with height and, to a lesser extent, leaf production, being good indicators.
While potentially toxic elements found naturally in or sequestered by Sargassum spp. remain a concern, for mangroves there is evidence that especially R. mangle has developed strategies to minimize uptake. These attributes of mangroves have actually been proposed for application to mitigate metal pollution in coastal areas (Machado and Lacerda, 2004). Therefore, there is potential for the use of the algal biomass in nuisance pelagic Sargassum spp. blooms to support mangrove rehabilitation under conditions where the application is in relatively dry areas (e.g., along the urbanized shorelines such as Kingston Harbor, Jamaica) and under controlled applications where the ameliorant can be contained in the immediate planting area. This could ensure better success of rehabilitation efforts in such areas and make use of the algal biomass, leading to the benefit of impoverished coastal communities who are ultimately most affected by Sargassum spp. inundations and mangrove loss.
Data Availability Statement
The original contributions presented in the study are included in the article/Supplementary Material; further inquiries can be directed to the corresponding author.
Author Contributions
CT: investigation, formal analysis, visualization, and writing—review and editing; S-LT: investigation, formal analysis, and writing—review and editing; DT: investigation, formal analysis, and writing—review and editing; G-MM: investigation, formal analysis, and writing—review and editing; PF: investigation, formal analysis, and writing—review and editing; HS: formal analysis and writing—review and editing; CBM: formal analysis and writing—review and editing; DW: formal analysis and writing—review and editing; TT: conceptualization, funding acquisition, investigation, formal analysis, visualization, and writing—review and editing. MW: conceptualization, funding acquisition, investigation, formal analysis, visualization, and writing—review and editing.
Funding
This work was supported by the Economic and Social Research Council [Grant number ES/T002964/1].
Acknowledgments
The authors are grateful to the following DBML interns, Javel Noble and Kadian McCalla as well as colleagues of the International Centre for Environment and Nuclear Sciences (ICENS) and Natural Products Institute (NPI) at the University of the West Indies (Mona) for assistance with sample processing and storage.
Conflict of Interest
The authors declare that the research was conducted in the absence of any commercial or financial relationships that could be construed as a potential conflict of interest.
Publisher’s Note
All claims expressed in this article are solely those of the authors and do not necessarily represent those of their affiliated organizations, or those of the publisher, the editors, and the reviewers. Any product that may be evaluated in this article, or claim that may be made by its manufacturer, is not guaranteed or endorsed by the publisher.
Supplementary Material
The Supplementary Material for this article can be found online at: https://www.frontiersin.org/articles/10.3389/fenvs.2022.932293/full#supplementary-material
References
Adugna, G. (2016). A review on impact of compost on soil properties, water use and crop productivity. Acad. Res. J. Agric. Sci. Res. 4, 93–104. doi:10.14662/ARJASR2016.010
Alongi, D. M. (2014). Carbon cycling and storage in mangrove forests. Ann. Rev. Mar. Sci. 6, 195–219. doi:10.1146/annurev-marine-010213-135020
Alongi, D. M. (2012). Carbon sequestration in mangrove forests. Carbon Manag. 3, 313–322. doi:10.4155/cmt.12.20
Alongi, D. M., Wattayakorn, G., Boyle, S., Tirendi, F., Payn, C., and Dixon, P. (2004). Influence of roots and climate on mineral and trace element storage and flux in tropical mangrove soils. Biogeochemistry 69, 105–123. doi:10.1023/b:biog.0000031043.06245.af
Amador-Castro, F., García-Cayuela, T., Alper, H. S., Rodriguez-Martinez, V., and Carrillo-Nieves, D. (2021). Valorization of pelagic Sargassum sp. biomass into sustainable applications: Current trends and challenges. J. Environ. Manag. 283, 112013. doi:10.1016/j.jenvman.2021.112013
Aslam, R., Bostan, N., Nabgha-e-Amen, M., and Safdar, W. (2011). A critical review on halophytes: Salt tolerant plants. J. Med. Plants Res. 5, 7108–7118. doi:10.5897/JMPRx11.009
Barreto, M. B. (2008). “Diagnostics about the state of mangroves in Venezuela: Case studies from the national park morrocoy and wildlife refuge cuare,” in Mangroves and halophytes: Restoration and utilisation. Editors P. H. Lieth, D. M. G. Sucre, and B. Herzog (Netherlands: Springer), 51–64.
CARDI (2015). Sargassum seaweed and its use in crop and livestock production: Possible agri-business opportunities. Policy Brief 2015 Available at: http://www.cardi.org/Sargassum-Seaweed-and-its-use-incrop-and-livestock-production-CARDI-Policy-Brief.pdf (accessed February 23, 2022).
Cerón-Souza, I., Barreto, M. B., Barreto-Pittol, E., Silva, A., Feliner, G. N., and Medina, E. (2021). Rhizophora zonation, salinity, and nutrients in the western atlantic. Biotropica 53, 384–396. doi:10.1111/btp.12924
Chávez, V., Uribe-Martínez, A., Cuevas, E., Rodríguez-Martínez, R. E., van Tussenbroek, B. I., Francisco, V., et al. (2020). Massive influx of pelagic sargassum spp. on the coasts of the Mexican caribbean 2014–2020: Challenges and opportunities. Water 12, 2908. doi:10.3390/w12102908
Davis, D., Simister, R., Campbell, S., Marston, M., Bose, S., McQueen-Mason, S. J., et al. (2021). Biomass composition of the golden tide pelagic seaweeds Sargassum fluitans and S. natans (morphotypes I and VIII) to inform valorisation pathways. Sci. Total Environ. 762, 143134. doi:10.1016/j.scitotenv.2020.143134
Desrochers, A., Cox, S., Oxenford, H., and van Tussenbroek, B. (2020). Sargassum sp. uses guide: A resource for caribbean researchers, entrepreneurs and policy makers. CERMES Tech. Rep. 97, 172.
Devault, D. A., Massat, F., Baylet, A., Dolique, F., and Lopez, P. J. (2022). Arsenic and chlordecone contamination and decontamination toxicokinetics in Sargassum sp. Environ. Sci. Pollut. Res. 29, 6–16. doi:10.1007/s11356-020-12127-7
Donato, D., Kauffman, J., Murdiyarso, D., Kurnianto, S., Stidham, M., and Kanninen, M. (2011). Mangroves among the most carbon-rich forests in the tropics. Nat. Geosci. 4, 293–297. doi:10.1038/ngeo1123
Drexler, J. Z., and Ewel, K. C. (2001). Effect of the 1997–1998 ENSO-related drought on hydrology and salinity in a micronesian wetland complex. Estuaries 24, 347–356. doi:10.2307/1353237
Eyras, M., Rostagn, C., and Defosse, G. (1998). Biological evaluation of Sargassum composting. Compost Sci. Util. 6, 74–81. doi:10.1080/1065657X.1998.10701943
Gab-Alla, A., Khafagi, I., Salama, W., and Fouda, M. (2003). Production of nursery-reared seedlings of the gray mangrove Avicennia marina under laboratory conditions. Egypt. J. Biol. 5, 55–61.
Giri, C., Zhu, Z., Tieszen, L., Singh, A., Gillette, S., and Kelmelis, J. (2008). Mangrove forest distributions and dynamics (1975–2005) of the tsunami-affected region of asia. J. Biogeogr. 35, 519–528. doi:10.1111/j.1365-2699.2007.01806.x
Hogarth, P. (2015). The biology of mangroves and seagrasses. 3rd Ed. United Kingdom: Oxford University Press.
Hoppe-Speer, S. C., Adams, J. B., Rajkaran, A., and Bailey, D. (2011). The response of the red mangrove Rhizophora mucronata Lam. to salinity and inundation in South Africa. Aquat. Bot. 95, 71–76. doi:10.1016/j.aquabot.2011.03.006
Kairo, J., Dahdouh-Guebas, F., Bosire, J., and Koedam, N. (2001). Restoration and management of mangrove systems - a lesson for and from the east african region. South Afr. J. Bot. 67, 383–389. doi:10.1016/s0254-6299(15)31153-4
Kathiresan, K. (2012). Importance of mangrove ecosystem. Int. J. Mar. Sci. 2, 70–89. doi:10.5376/ijms.2012.02.0010
Krauss, K. W., Lovelock, C. E., McKee, K. L., López-Hoffman, L., Ewe, S. M., and Sousa, W. P. (2008). Environmental drivers in mangrove establishment and early development: A review. Aquat. Bot. 89, 105–127. doi:10.1016/j.aquabot.2007.12.014
Lee, S. Y., Primavera, J. H., Dahdouh-Guebas, F., McKee, K., Bosire, J. O., Cannicci, S., et al. (2014). Ecological role and services of tropical mangrove ecosystems: A reassessment. Glob. Ecol. Biogeogr. 23, 726–743. doi:10.1111/geb.12155
Lewis, R. R. (2009). “Methods and criteria for successful mangrove forest restoration,” in Coastal wetlands: An integrated ecosystem approach (Amsterdam, Netherlands), 787–800.
MacFarlane, G. R., and Burchett, M. D. (2002). Toxicity, growth and accumulation relationships of copper, lead and zinc in the grey mangrove Avicennia marina (Forsk.) Vierh. Mar. Environ. Res. 54, 65–84. doi:10.1016/s0141-1136(02)00095-8
MacFarlane, G. R., Koller, C. E., and Blomberg, S. P. (2007). Accumulation and partitioning of heavy metals in mangroves: A synthesis of field-based studies. Chemosphere 69, 1454–1464. doi:10.1016/j.chemosphere.2007.04.059
Machado, C. B., Maddix, G., Thomas, S., Burton, J., Langer, S., Larson, T., et al. (2022). Pelagic sargassum events in Jamaica: Morphotype abundance, provenance, and influence of sample preparation on biochemical composition. Sci. Total Environ. 817, 152761. doi:10.1016/j.scitotenv.2021.152761
Machado, W., and Lacerda, L. D. (2004). “Overview of the biogeochemical controls and concerns with trace metal accumulation in mangrove sediments,” in Environmental geochemistry in tropical and subtropical environments (Berlin, Heidelberg: Springer), 319–334. doi:10.1007/978-3-662-07060-4_22
Mandal, A., Smith, R-A., Edwards, T., Kinlocke, R., Mitchell, S., Webber, M., et al. (2019). Forces of nature: Assessment of the economic valuation of coastal protection services provided by mangroves in Jamaica. The world bank-PROFOR-world bank caribbean office.
Medina, E. (1999). “Mangrove physiology: The challenge of salt, heat, and light stress under recurrent flooding,” in Ecosistemas de manglar en América tropical. Editors A. Yáñez-Arancibia, and A. L. Lara-Domínguez (México: Instituto de Ecología A.C), 109–126.
Milledge, J. J., and Harvey, P. J. (2016). Golden tides: Problem or golden opportunity? The valorisation of sargassum from beach inundations. J. Mar. Sci. Eng. 4, 60. doi:10.3390/jmse4030060
Milledge, J. J., Nielsen, B. V., and Bailey, D. (2016). High-value products from macroalgae: The potential uses of the invasive brown seaweed, Sargassum muticum. Rev. Environ. Sci. Biotechnol. 15, 67–88. doi:10.1007/s11157-015-9381-7
Miranda, J. L. L., Celis, L. B., Estévez, M., Chávez, V., Van Tussenbroek, B. I., Uribe-Martínez, A., et al. (2021). Commercial potential of pelagic sargassum spp. in Mexico. Front. Mar. Sci. 8, 768470. doi:10.3389/fmars.2021.768470
Mitra, A., Zaman, S., and Gobato, R. (2018). Indian sundarban mangroves: A potential carbon scrubbing system. Parana J. Sci. Educ. 4, 7–29.
Mohanty, D., Adhikary, S., Chattopadhyay, G., Prakasam, V., Gopamma, D., Ramoji, G., et al. (2013). Seaweed liquid fertilizer (SLF) and its role in agriculture productivity. Ecoscan III 23–26, 1–10.
Nguyen, T. P., Van Tam, N., and Parnell, K. E. (2016). Community perspectives on an internationally funded mangrove restoration project: Kien Giang province, Vietnam. Ocean Coast. Manag. 119, 146–154. doi:10.1016/j.ocecoaman.2015.10.008
Oxenford, H. A., Cox, S. A., van Tussenbroek, B. I., and Desrochers, A. (2021). Challenges of turning the sargassum crisis into gold: Current constraints and implications for the caribbean. Phycology 1, 27–48. doi:10.3390/phycology1010003
Pan, I., Dam, B., and Sen, S. K. (2012). Composting of common organic wastes using microbial inoculants. Biotech 2, 127–134. doi:10.1007/s13205-011-0033-5
Parida, A. K., and Jha, B. (2010). Salt tolerance mechanisms in mangroves: A review. Trees 24, 199–217. doi:10.1007/s00468-010-0417-x
Reef, R., Feller, I. C., and Lovelock, C. E. (2010). Nutrition of mangroves. Tree Physiol. 30, 1148–1160. doi:10.1093/treephys/tpq048
Rodríguez-Martínez, R. E., Roy, P. D., Torrescano-Valle, N., Cabanillas-Terán, N., Carrillo-Domínguez, S., Collado-Vides, L., et al. (2020). Element concentrations in pelagic Sargassum sp. along the Mexican Caribbean coast in 2018-2019. PeerJ 8, e8667. doi:10.7717/peerj.8667
Saher, N. U., and Asmat, S. S. (2017). Evaluation of heavy metals contamination in mangrove sediments and their allied fiddler crab species (Austruca sindensis (alcock, 1900) from Hawks bay, Karachi, Pakistan. Pak. Int. J. Biol. Biotechnol. 14, 411–417.
Salter, M. A., Rodríguez-Martínez, R. E., Álvarez-Filip, L., Jordán-Dahlgren, E., and Perry, C. T. (2020). Pelagic Sargassum as an emerging vector of high rate carbonate sediment import to tropical Atlantic coastlines. Glob. Planet. Change 195, 103332. doi:10.1016/j.gloplacha.2020.103332
Schell, J. M., Goodwin, D. S., and Siuda, A. N. (2015). Recent sargassum inundation events in the caribbean: Shipboard observations reveal dominance of a previously rare form. Oceanogr. Wash. D. C). 28, 8–10. doi:10.5670/oceanog.2015.70
Sembera, J. A., Meier, E. J., and Waliczek, T. M. (2018). Composting as an alternative management strategy for sargassum drifts on coastlines. HortTechnology 28, 80–84. doi:10.21273/horttech03836-17
Silva, C. A. R., Lacerda, L. D., and Rezende, C. E. (1990). Metals reservoir in a red mangrove forest. Biotropica 22, 339–345. doi:10.2307/2388551
Thompson, T. M., Young, B. R., and Baroutian, S. (2020). Pelagic sargassum for energy and fertiliser production in the caribbean: A case study on Barbados. Renew. Sustain. Energy Rev. 118, 109564. doi:10.1016/j.rser.2019.109564
Tonon, T., Machado, C. B., Webber, M., Webber, D., Smith, J., Pilsbury, A., et al. (2022). Biochemical and elemental composition of pelagic sargassum biomass harvested across the caribbean. Phycology 2, 204–215. doi:10.3390/phycology2010011
Trench, C. (2021). Hydrological restoration approaches for degraded mangrove forests in Jamaica. PhD Thesis. UWI (Mona): Department of Life Sciences.
Turner, R., and Lewis, R. (1997). Hydrologic restoration of coastal wetlands. Wetl. Ecol. Manag. 4, 65–72. doi:10.1007/bf01876229
Uddin, M. S., van Steveninck, E. D. R., Stuip, M., and Shah, M. A. R. (2013). Economic valuation of provisioning and cultural services of a protected mangrove ecosystem: A case study on sundarbans reserve forest, Bangladesh. Ecosyst. Serv. 5, 88–93. doi:10.1016/j.ecoser.2013.07.002
Valiela, I., Bowen, J., and York, J. (2001). Mangrove forests: One of the world's threatened major tropical environments. Bioscience 51, 807–815. doi:10.1641/0006-3568(2001)051[0807:mfootw]2.0.co;2
van Tussenbroek, B. I., Hernandez Arana, H. A., Rodriguez-Martinez, R. E., Espinoza-Avalos, J., Canizales-Flores, H. M., Gonzalez-Godoy, C. E., et al. (2017). Severe impacts of brown tides caused by Sargassum sp. spp. on near-shore Caribbean seagrass communities. Mar. Pollut. Bull. 122, 272–281. doi:10.1016/j.marpolbul.2017.06.057
Walsh, G. E., Ainsworth, K. A., and Rigby, R. (1979). Resistance of red mangrove (Rhizophora mangle L.) seedlings to lead, cadmium, and mercury. Biotropica 11, 22–27. doi:10.2307/2388167
Walsh, K. T. (2019). Examining the quality of a compost product derived from Sargassum (Sargassum fluitans and Sargassum natans). San Marcos, TX: MS Thesis- Texas State University.
Wang, M., Hu, C., Barnes, B. B., Mitchum, G., Lapointe, B., and Montoya, J. P. (2019). The great Atlantic Sargassum sp. belt. Science 365, 83–87. doi:10.1126/science.aaw7912
Webber, M., Calumpong, H., Ferreira, F., Granek, E., Green, S., Ruwa, R., et al. (2016). The first global integrated marine assessment: World ocean assessment I. Cambridge University Press, Mangroves, 877–886. Available at: http://www.un.org/depts/los/global_reporting/WOA_RPROC/Chapter_48.pdf.
Keywords: Sargassum compost, urbanized coastlines, mangrove seedlings, element analysis, Caribbbean
Citation: Trench C, Thomas S-L, Thorney D, Maddix G-M, Francis P, Small H, Machado CB, Webber D, Tonon T and Webber M (2022) Application of Stranded Pelagic Sargassum Biomass as Compost for Seedling Production in the Context of Mangrove Restoration. Front. Environ. Sci. 10:932293. doi: 10.3389/fenvs.2022.932293
Received: 29 April 2022; Accepted: 22 June 2022;
Published: 06 September 2022.
Edited by:
Bernardo Duarte, Center for Marine and Environmental Sciences (MARE), PortugalReviewed by:
Carina Rafaela Faria Da Costa Félix, Polytechnic Institute of Leiria, PortugalAmany mohamed El-sikaily, Natioal instittut of oceanograpphy and fisheries, Egypt, Egypt
Copyright © 2022 Trench, Thomas, Thorney, Maddix, Francis, Small, Machado, Webber, Tonon and Webber. This is an open-access article distributed under the terms of the Creative Commons Attribution License (CC BY). The use, distribution or reproduction in other forums is permitted, provided the original author(s) and the copyright owner(s) are credited and that the original publication in this journal is cited, in accordance with accepted academic practice. No use, distribution or reproduction is permitted which does not comply with these terms.
*Correspondence: Thierry Tonon, dGhpZXJyeS50b25vbkB5b3JrLmFjLnVr