- 1Collaborative Innovation Center for Vessel Pollution Monitoring and Control, College of Environmental Sciences and Engineering, Dalian Maritime University, Dalian, China
- 2State Key Laboratory of Urban Water Resources and Environments (SKLURE), School of Environment, Harbin Institute of Technology, Harbin, China
A novel bioelectrochemical reactor assembled with cooperative cathodes of chemical cathode and bio-cathode (BERCC) and excess sludge as the anodic substrate obtained continuous and effective Cr(VI) reduction. Cooperative cathodes in BERCC stimulated the growth of electrochemically active microorganisms such as Geobacter sp. and Shewanella sp. in the anodic biofilm and produced 8.21 ± 0.64 mg C/(L·h) more electrons than the dual chemical cathodes in the bioelectrochemical reactor with dual chemical cathodes, which enhanced the electrons for electricity generation and Cr(VI) reduction by approximately 58.3% and 56.1 ± 5.6%, respectively.
1 Introduction
The microbial electrochemical system (MES) is an emerging platform technology that combines electrochemical systems with microbial processes. In a typical MES with a dual chamber for Cr(VI) reduction, the initial high concentrations of Cr(VI) and H+ can lead to a high Cr(VI) reduction rate at the early stages of the reaction process (Gupta et al., 2017). As the reaction progresses, the consumption of Cr(VI) and H+ in the cathodic chamber results in a decline in the motivation force from the cathodic reaction, which reduces the activity of electrochemically active microorganisms (EAMs) in the anodic chamber and has a negative influence on electricity generation (Xafenias et al., 2014; Li et al., 2021; More and Gupta, 2021). Similar changes have shown that the MES faces a decline in Cr(VI) reduction efficiency after cycles of operation, which limits the continuous and effective Cr(VI) reduction in the MES (Huang et al., 2021; Song et al., 2016; An et al., 2014).
Using a high organic concentration of anodic substrate is one solution that supplies abundant electrons for continuous and effective Cr(VI) reduction in the MES. Sludge has been reported to supply sufficient electrons for stable and high-flow electron transfer in the MES (Jiang et al., 2009; Meng et al., 2019) and can thus create the condition of sufficient electron donors for stable and high-flow electron transfer. Maintaining high activity of EAMs is the other solution that supplies electrons for continuous and effective Cr(VI) reduction in the MES, which results in high performance of electricity generation. The continuous Cr(VI) reduction rate and electricity generation can be kept stable by the cooperative cathodes of the bio-cathode coupled to traditional dual-chamber MES, as has been shown in a previous study by Xie et al. (2011). In addition, bio-cathode could lower the cost of construction and operation of reactors (He and Angenent 2006; Rabaey and Keller 2008). Hence, coupling of the bio-cathode and chemical cathode can be regarded as the appropriate cooperative cathodes to breakthrough the limitations of continuous and effective Cr(VI) reduction in the MES, which has rarely been reported.
To obtain abundant electrons for continuous and effective Cr(VI) reduction in the MES, a novel BER assembled with cooperative cathodes of chemical cathode and bio-cathode was constructed, as shown in Figure 1A. The performances of the BER with cooperative cathodes (BERCC) and BER with dual chemical cathodes (BERDC) were compared to substantiate the feasibility of maintaining high efficiency by cooperative cathodes. Furthermore, the distribution of electron flux and the structure of the microbial community within BERDC and BERCC were analyzed. This study provides an alternative technology for the continuous and efficient remediation of Cr(VI)-contaminated wastewater and the treatment of excess sludge.
2 Materials and methods
The bioelectrochemical reactor with cooperative cathodes (BERCC) consists of a cylindrical anodic chamber (Φ 80 mm × 90 mm) and two cubic cathodic chambers (60 mm × 70 mm × 90 mm each) (Figure 1A). A proton-exchange membrane (PEM) (Nafion™ 117, DuPont Co., United States) separated the anodic chamber from the chemical cathodic chamber to prevent the Cr3+ produced in the chemical cathodic chamber from entering the anodic chamber, and the cation exchange membrane (CEM) (AMI-7001, Ultrex, United States) separated the anodic chamber from the bio-cathodic chamber for low cost. All of the anodes and cathodes were made of graphite fiber brushes (Φ 70 mm × 70 mm for the anode and Φ 60 mm × 70 mm for both the cathodes) and titanium wires. The BER with dual chemical cathodes (BERDC) possesses the same configuration as that of the BERCC, as shown in Figure 1B, which was applied as the control for the experiments. Both the chemical cathodic chambers were separated by the PEMs from the anodic chamber. The BERCC at the open circuit was used as the control to reflect the role of adsorption for Cr(VI) removal. The operation and analysis methods of this study are shown in the Supplementary Material.
3 Results and discussion
3.1 Performance of bioelectrochemical reactor with cooperative cathodes and bioelectrochemical reactor with dual chemical cathodes
3.1.1 Performance of hexavalent chromium reduction
As shown in Figure 2A, Cr(VI) was almost completely reduced within 55.5 and 57.5 h in the BERCC and BERDC, respectively, during the first Cr(VI) reduction cycle. After that, the Cr(VI) reduction rates of the BERDC gradually decreased, and it took 102.5 and 66.0 h for the BERDC to reduce Cr(VI) by 72.5% ± 2.8% and 52.1% ± 1.8%, respectively. The Cr(VI) reduction efficiency dropped to 39.9% ± 1.3% for the fifth cycle. Based on the abovementioned results, Cr(VI) could be entirely reduced for seven cycles in BERCC; however, it took much longer to reduce Cr(VI) in the BERDC than in the BERCC (Figure 2A). The variation in the Cr(VI) concentration in the BERCC at the open circuit revealed that adsorption was nonsignificant in the BERCC (Supplementary Figure S1). Similar results were also found by Li et al. (2018) that adsorption was nonsignificant in open-circuit microbial fuel cells. The reduction products (dark green precipitates) of Cr(VI) were found attached to the chemical cathode and sank to the bottom in the BERCC (Supplementary Figures S2A,B). Both the main constituent elements of the precipitates at the bottom of the cathodic chamber and those attached to the surface of the chemical cathode were Cr (Supplementary Figure S2; Supplementary Table S1). The main component of the precipitate in the cathodic chamber was chromium oxide (Supplementary Figure S3).
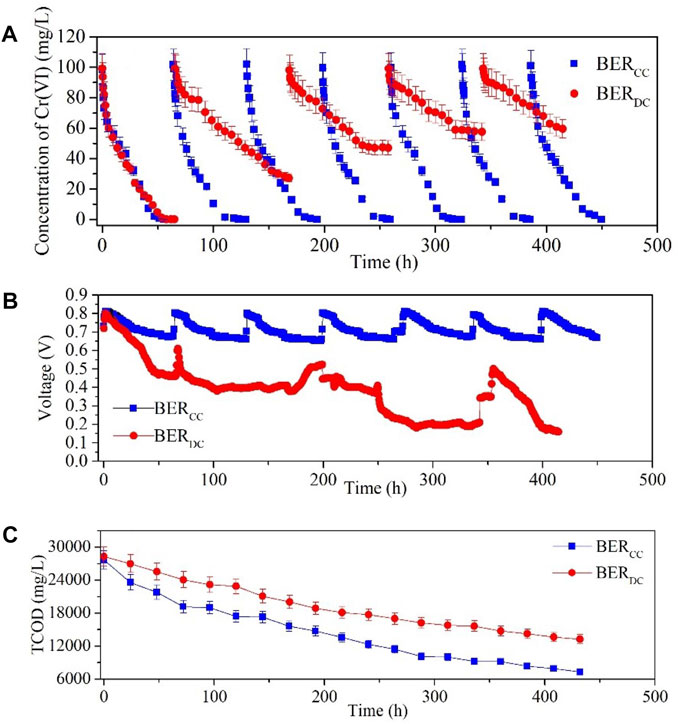
FIGURE 2. Variations in Cr(VI) concentration (A), voltage (B), and TCOD (C) during the operation of BERCC and BERDC.
3.1.2 Performance of electricity generation and sludge degradation
During seven Cr(VI) reduction cycles, the voltage of BERCC was consistently above 0.672 V, and the maximum voltage reached approximately 0.813 V at the beginning of each cycle in Figure 2B. The BERDC produced a high voltage only at the beginning stage of the first cycle, and the voltage gradually decreased as the Cr(VI) reduction proceeded. During the second reduction cycle of the BERDC, the voltage first increased slightly compared to that at the end of the first cycle, but it gradually declined as Cr(VI) reduction proceeded. The tendency of voltage for the fifth cycle was similar to that for the previous cycles. After 432 h of operation, the total chemical oxygen demand (TCOD) of the sludge decreased by 73.6% ± 4.4% from 27,692 ± 1,662 mg/L to 7,307 ± 438 mg/L in the anodic chamber of the BERCC and by 52.1% ± 3.1% from 27,692 ± 1,662 mg/L to 13,267 ± 796 mg/L in the anodic chamber of the BERDC. Of the electrons produced in organic matter degradation, a great amount was used for electricity generation, and this process could accelerate the organic matter degradation of the sludge in bioelectrochemical system (BES) (Xafenias et al., 2014).
That the BERCC had a higher performance of electricity generation was the main reason for the TCOD degradation of the BERDC being higher than that of the BERCC (ref. Section 3.1.1). The current generation decreased gradually during the 200 h of operation in the microbial fuel cell (MFC) with the cathode chamber treating chromium wastewater (Kim et al., 2017). A dual-chamber MES was tested on the wastewater from an electroplating industry; the voltage increased to its maximum value after 48 h and reached its peak at 72 h, after which the voltage started to drop with time (More and Gupta, 2021). A salt bridge was used as a proton-exchange membrane of the MES to treat Cr(VI)-containing water, and the wave phenomena of the current was also found for 456 h in this system (Sophia and Sai, 2016). These results have confirmed that the configuration of the dual chamber is the bottleneck that limits the application of the MES in the treatment of industrial wastewater with chromium.
The voltage of the BERDC decreased with the operation, and the reaction of extracellular electron transfer at the anode slowed. The decrease in the performance of the MES was attributed to the reduction of the catalytic activity of the electrode (Xafenias et al., 2014). Under the same conditions, although the electrons that reacted at the chemical cathode decreased with the decrease in Cr(VI) concentration in the BERCC, the electrons that reacted at the bio-cathode increased with it. Therefore, the anodic reaction could be kept at a high rate, and the voltage could be kept at a high level. A certain number of rod-shaped EAMs existed on the surface of the anode, and the biofilm could be found on the bio-cathode (Supplementary Figures S1C,D). These indicate that the EAMs maintained high activity with the cooperative cathodes of the chemical cathode and bio-cathode.
3.2 Quantification of the electron fluxes
The analysis of the electron flux could reveal the advantages of cooperative cathodes in the production and utilization of electrons within the BERCC. Electron fluxes were distributed among different electron sinks (viz., current generation, exoelectrogen growth, hydrogen gas production, methanogen growth, and methane production) based on the electron balances and estimated (exoelectrogen and methanogen) growth rates in the BER as reported in a previous study (Yu et al., 2018). Most of the electrons for current generation were transferred along the anode to the cathode and participated in the cathodic reaction with a small amount of loss.
During two Cr(VI) reduction cycles of the BERDC, the average degradation rate of TCOD was 18.42 ± 4.03 mg C/(L·h) [viz., mg Coulombs/(L·h)] (Supplementary Table S2), which contained 7.23 ± 1.94 mg C/(L·h) for methane production, 9.59 ± 0.43 mg C/(L·h) for biomass (exoelectrogen and methanogen) growth, portions for the average current production of 0.48 mA, and a Cr(VI) reduction rate of 1.07 ± 0.11 mg C/(L·h). Of the electrons produced in the anodic chamber at a unit time as shown in Figure 3, the proportions of electrons for methanogen growth, hydrogen production, methane production, and current generation (transferred along the anode) were 23.5% ± 2.7%, 6.4% ± 0.8%, 33.3% ± 3.5%, and 13.3% ± 1.7%, respectively. A total of 77.0% ± 6.9% of the electrons that were transferred along the anode moved to the chemical cathodes for Cr(VI) reduction. By contrast, the average degradation rate of TCOD was 8.21 ± 0.64 mg C/(L·h) higher in the BERCC than the BERDC, and the electrons for the average current were increased by 0.28 mA (Supplementary Table S2). These results are consistent with those mentioned in Sections 3.1.2.
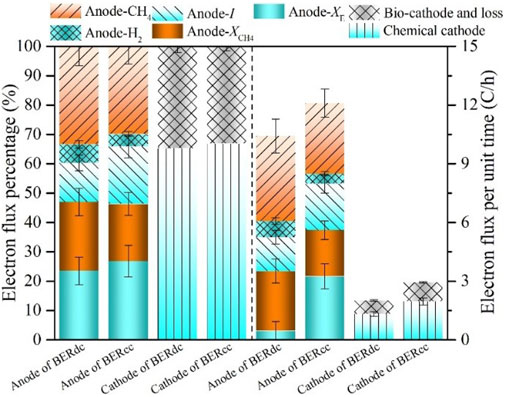
FIGURE 3. Distribution of electron fluxes among different electron sinks and the electron fluxes per unit time at the anode and cathode of BERDC and BERCC (Anode-CH4: estimated electrons used for methane production at the anode; Anode-H2: estimated electrons used for hydrogen production at the anode; Anode-I: electrons transferred from the anode to the cathode to generate current at the anode; Anode-XCH4: estimated electrons consumed for methanogen growth at the anode; Anode-XE: estimated electrons consumed for exoelectrogen growth at the anode; Bio-cathode and loss: estimated electrons transferred to the bio-cathode or loss in transfer; Chemical cathode: estimated electrons transferred to the chemical cathode).
For each cycle in the BERCC, the Cr(VI) concentration gradually decreased with the reduction of Cr(VI) by the chemical cathode, and the electrons for the chemical cathode were therefore reduced and the excess electrons transferred from the anode were used for bio-cathode. Cooperative cathodes supported the high activity of EAMs (Supplementary Figure S1) instead of gradual reduction, therefore Cr(VI) reduction could be continuously and efficiently maintained (Figure 2) at the chemical cathode for the next cycle. During two operation cycles, quantification of the electron fluxes revealed that 8.21 ± 4.03 mg C/(L·h) more electrons were produced from organic matter degradation in the BERCC than the BERDC. Of them, the electrons for Cr(VI) reduction increased by 56.1% ± 5.6%, and some electrons supported the current generation increase of 0.28 mA. The electrons for the chemical cathodic reaction and bio-cathodic reaction (and loss) were increased by 51.5% ± 4.6% and 41.1% ± 3.7%, respectively, in the BERCC as compared to the BERDC (Figure 3).
3.3 Distribution of microbial community
Microbial community analysis was used to further explore why more electrons were generated by sludge in the anodic chamber and utilized for electricity generation and Cr(VI) reduction within the BERCC than in the BERDC (Figure 4A). The proportions of Deltaproteobacteria, Clostridia, and Anaerolineae in the biofilms on the anodes of the BERCC and BERDC were, respectively, 9.5–17, 38–54, and 85–212 times those on the bio-cathode of the BERCC, which reflects the selective distribution of the EAMs on the anode (Figure 4B). Deltaproteobacteria were abundant in the anodic biofilm of the MES with high electricity generation (Bond et al., 2002; Lee et al., 2003), indicating that bacteria in relation to iron reduction and electricity generation might be present. Some bacteria belonging to Clostridia with bioelectrochemical activity and characteristics of Fe(III) reduction were isolated from the MFCs (Park et al., 2003). Geobacteraceae, which are well-known EAMs, can transfer electrons to the anode directly. Xanthomonas of the class Gammaproteobacteria is the EAM that is found in the MFCs under different operation conditions (Yu et al., 2015). The relative abundances of Comamonadaceae, Geobacteraceae, and Xanthomonadaceae were higher in the anodic biofilm of the BERCC than that of the BERDC, which implies that BERCC has a higher performance of electricity generation (Figure 4C). More effective cooperative cathodes than dual chemical cathodes have stimulated the growth of EAMs (Supplementary Table S3) and produced more electrons (ref. Section 3.2), which enhanced the performance of Cr(VI) reduction and electricity generation in the BERCC (ref. Section 3.1).
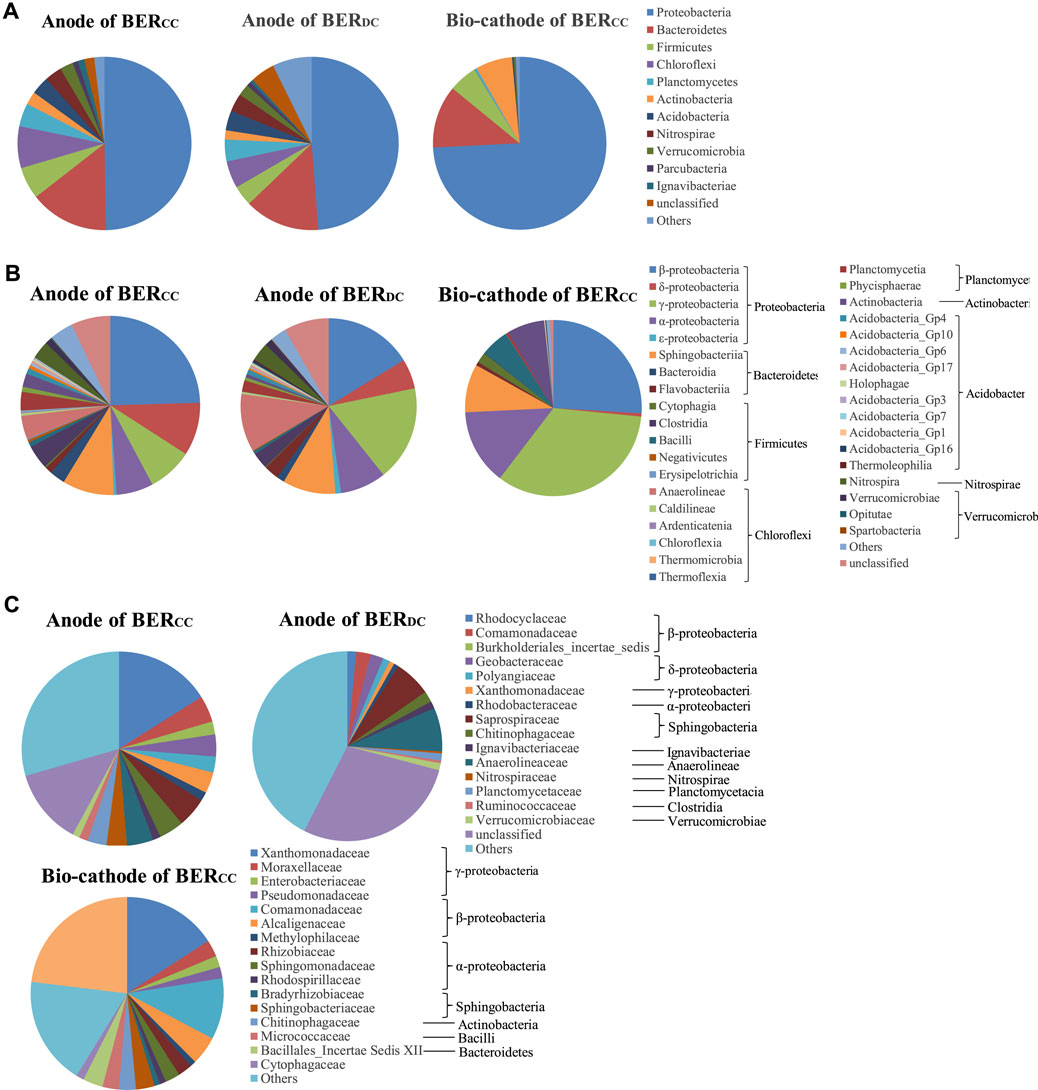
FIGURE 4. Relative abundances (%) of biofilm bacteria on the anode and the bio-cathode of BERCC and the anode of BERDC at the phylum (A), class (B), and genus levels (C).
4 Conclusion
A novel BER with cooperative cathodes of a chemical cathode and bio-cathode (BERCC) for continuous and effective Cr(VI) reduction and electricity generation was realized. Cr(VI) could be fully reduced, and the voltage was consistently above 0.672 V for at least seven cycles in the BERCC. The TCOD of sludge decreased by 73.6% ± 4.4% after at least seven cycles. The quantification of the electron fluxes have revealed that 8.21 ± 4.03 mg C/(L·h) more electrons were produced from sludge degradation and 47.9% ± 4.3% more electrons participated in cathodic reactions in the BERCC than in the BERDC. More EAMs were found in the anodic biofilm of the BERCC than of the BERDC, of which Geobacter was the most abundant. This study provides an alternative technology for the continuous and efficient remediation of Cr(VI)-contaminated wastewater and the treatment of excess sludge.
Data availability statement
The datasets presented in this study can be found in online repositories. The names of the repository/repositories and accession number(s) can be found below: https://www.ncbi.nlm.nih.gov/bioproject/, PRJNA836022.
Author contributions
QZ and YZ participated in the conceptualization, supervision, and writing—reviewing and editing. HY participated in the conceptualization, methodology, software, and writing—original draft preparation. YC participated in the visualization and investigation. ZL participated in the data curation, methodology, and software.
Funding
The authors gracefully acknowledge funding from Project 51908100 and 51778176 supported by the National Nature Science Foundation of China, Projects 2020-HYLH-21 and 2021-BS-070 supported by the Liaoning Provincial Natural Science Foundation of China, Projects 3132021160 and 3132019334 supported by the Fundamental Research Funds for the Central Universities, and Project 2021TQ0052 funded by the China Postdoctoral Science Foundation.
Conflict of interest
The authors declare that the research was conducted in the absence of any commercial or financial relationships that could be construed as a potential conflict of interest.
Publisher’s note
All claims expressed in this article are solely those of the authors and do not necessarily represent those of their affiliated organizations, or those of the publisher, the editors, and the reviewers. Any product that may be evaluated in this article, or claim that may be made by its manufacturer, is not guaranteed or endorsed by the publisher.
Supplementary material
The Supplementary Material for this article can be found online at: https://www.frontiersin.org/articles/10.3389/fenvs.2022.932266/full#supplementary-material
References
An, Z. Y., Zhang, H. C., Wen, Q. X., Chen, Z. Q., and Du, M. A. (2014). Desalination combined with hexavalent chromium reduction in a microbial desalination cell. Desalination 354, 181–188. doi:10.1016/j.desal.2014.10.006
Bond, D. R., Holmes, D. E., Tender, L. M., and Lovely, D. R. (2002). Electrode-reducing microorganisms that harvest energy from marine sediments. Science 295, 483–485. doi:10.1126/science.1066771
Gupta, S., Yadav, A., and Verma, Nishith (2017). Simultaneous Cr(VI) reduction and bioelectricity generation using microbial fuel cell based on alumina-nickel nanoparticles-dispersed carbon nanofiber electrode. Chem. Eng. J. 307 (1), 729–738. doi:10.1016/j.cej.2016.08.130
He, Z., and Angenent, L. T. (2006). Application of bacterial biocathodes in microbial fuel cells. Electroanalysis 18, 2009–2015. doi:10.1002/elan.200603628
Huang, L., Song, S., Cai, Z., Zhou, P., and Puma, G. L. (2021). Efficient conversion of bicarbonate (HCO3−) to acetate and simultaneous heavy metal Cr(VI) removal in photo-assisted microbial electrosynthesis systems combining WO3/MoO3/g-C3N4 heterojunctions and Serratia marcescens electrotroph. Chem. Eng. J. 406, 126786. doi:10.1016/j.cej.2020.126786
Jiang, J. Q., Zhao, Q. L., Zhang, J. N., Zhang, G. D., and Lee, D. J. (2009). Electricity generation from bio-treatment of sewage sludge with microbial fuel cell. Bioresour. Technol. 100, 5808–5812. doi:10.1016/j.biortech.2009.06.076
Kim, C. M., Lee, C. R., Song, Y. E., Heo, J. H., Choi, S. M., Lim, D. H., et al. (2017). Hexavalent chromium as a cathodic electron acceptor in a bipolar membrane microbial fuel cell with the simultaneous treatment of electroplating wastewater. Chem. Eng. J. 328, 703–707. doi:10.1016/j.cej.2017.07.077
Lee, J., Phung, N. T., Chang, I. S., Kim, B. H., and Sung, H. C. (2003). Use of acetate for enrichment of electrochemically active microorganisms and their 16S rDNA analyses. FEMS Microbiol. Lett. 223, 185–191. doi:10.1016/s0378-1097(03)00356-2
Li, F., Min, D., Cheng, Z., Li, J., Wu, J., Tang, Q., et al. (2021). Systematically assessing genetic strategies for engineering electroactive bacterium to promote bioelectrochemical performances and pollutant removal. Sustain. Energy Technol. Assessments 47, 101506. doi:10.1016/j.seta.2021.101506
Li, M., Zhou, S. Q., Xu, Y. T., Liu, Z. J., Ma, F. Z., Zhi, L. L., et al. (2018). Simultaneous Cr(VI) reduction and bioelectricity generation in a dual chamber microbial fuel cell. Chem. Eng. J. 334, 1621–1629. doi:10.1016/j.cej.2017.11.144
Meng, F. Y., Zhao, Q. L., Zheng, Z. L., Wei, L., Wang, K., Jiang, J. Q., et al. (2019). Simultaneous sludge degradation, desalination and bioelectricity generation in two-phase microbial desalination cells. Chem. Eng. J. 36, 1180. doi:10.1016/j.cej.2018.12.063
More, A. G., and Gupta, S. K. (2021). Removal of chromium from electroplating industry wastewater using bioelectrochemical system: Kinetic study and statistical analysis. J. Hazard. Toxic. Radioact. Waste 25 (2), 04020069. doi:10.1061/(asce)hz.2153-5515.0000571
Park, H. S., Kim, B. H., Kim, H. S., Kim, H. J., Kim, G. T., Kim, M., et al. (2003). A novel ElectrochemicallyActive and Fe(III)-reducing bacterium phylogenetically related to Clostridium butyricum isolated from a microbial fuel cell. FEMS Microbiol. Lett. 223, 129. doi:10.1016/S0378-1097(03)00354-9
Rabaey, K., and Keller, J. (2008). Microbial fuel cell cathodes: From bottleneck to prime opportunity? Water Sci. Technol. 57, 655–659. doi:10.2166/wst.2008.103
Song, T. S., Jin, Y., Bao, J., Kang, D., and Xie, J. (2016). Graphene/biofilm composites for enhancement of hexavalent chromium reduction and electricity production in a biocathode microbial fuel cell. J. Hazard. Mat. 317, 73–80. doi:10.1016/j.jhazmat.2016.05.055
Sophia, A. C., and Sai, S. (2016). Modified microbial fuel cell for Cr(VI) reduction and simultaneous bio-electricity production. J. Environ. Chem. Eng. 4, 2402–2409. doi:10.1016/j.jece.2016.04.025
Xafenias, N., Zhang, Y., and Banks, C. J. (2014). Evaluating hexavalent chromium reduction and electricity production in microbial fuel cells with alkaline cathodes. Int. J. Environ. Sci. Technol. (Tehran). 12, 2435–2446. doi:10.1007/s13762-014-0651-7
Xie, S., Liang, P., Chen, Y., Xia, X., and Huang, X. (2011). Simultaneous carbon and nitrogen removal using an oxic/anoxic-biocathode microbial fuel cells coupled system. Bioresour. Technol. 102 (1), 348–354. doi:10.1016/j.biortech.2010.07.046
Yu, H., Zhao, Q. L., Dong, Q. S., Jiang, J. Q., Wang, K., and Zhang, Y. S. (2018). Electronic and metagenomic insights into the performance of bioelectrochemical reactor simultaneously treating sewage sludge and Cr(VI)-laden wastewater. Chem. Eng. J. 341, 495–504. doi:10.1016/j.cej.2018.01.159
Keywords: bioelectrochemical reactor, hexavalent chromium, cooperative cathodes, excess sludge, electron flux, electrochemically active microorganisms
Citation: Yu H, Cao Y, Zhao Q, Liang Z and Zhu Y (2022) Cooperative cathodes for enhanced hexavalent chromium reduction and electricity generation in bioelectrochemical reactor with simultaneous sludge degradation. Front. Environ. Sci. 10:932266. doi: 10.3389/fenvs.2022.932266
Received: 29 April 2022; Accepted: 04 July 2022;
Published: 13 September 2022.
Edited by:
Bor-Yann Chen, National Ilan University, TaiwanReviewed by:
Bin Bian, King Abdullah University of Science and Technology, Saudi ArabiaHossain M. Zabed, Jiangsu University, China
Copyright © 2022 Yu, Cao, Zhao, Liang and Zhu. This is an open-access article distributed under the terms of the Creative Commons Attribution License (CC BY). The use, distribution or reproduction in other forums is permitted, provided the original author(s) and the copyright owner(s) are credited and that the original publication in this journal is cited, in accordance with accepted academic practice. No use, distribution or reproduction is permitted which does not comply with these terms.
*Correspondence: Qingliang Zhao, cWx6aGFvQGhpdC5lZHUuY24=; Yimin Zhu, bnRwQGRsbXUuZWR1LmNu