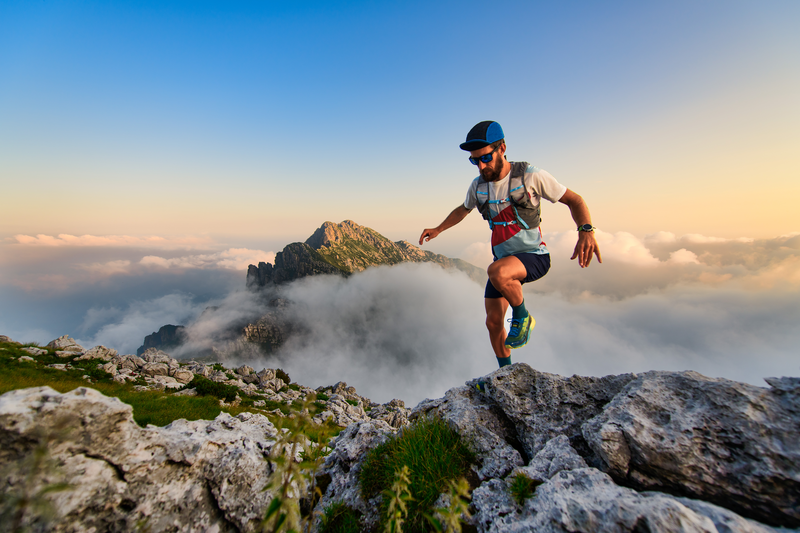
94% of researchers rate our articles as excellent or good
Learn more about the work of our research integrity team to safeguard the quality of each article we publish.
Find out more
ORIGINAL RESEARCH article
Front. Environ. Sci. , 05 August 2022
Sec. Toxicology, Pollution and the Environment
Volume 10 - 2022 | https://doi.org/10.3389/fenvs.2022.932161
This article is part of the Research Topic Connecting Biodiversity Protection, Nature Conservation Assessment, and Ecological Risk Assessment View all 6 articles
Freshwater ecosystems provide many benefits to people (ecosystem services), but their biodiversity and functioning is threatened by anthropogenic stressors, including chemical pollution. Environmental quality standards (EQSs) for chemicals, are designed to protect species, but their derivation takes no account of ecosystem processes or species interactions and hence their links to biodiversity, ecosystem functioning and ecosystem services are uncertain. Here we explore a novel approach for the derivation of chemical EQSs to protect ecosystem service providing units (i.e., assemblages of species with ecological traits that underpin an ecosystem service) and ultimately protect ecosystem service delivery in different freshwater bodies and river basins. This approach, which was illustrated for two Water Framework Directive priority chemicals (a pyrethroid insecticide and polybrominated diphenyl ethers), is the first application of an ecosystem services framework to derive EQS values. The four-step approach enabled the derivation of ecosystem service-specific and river basin-specific standards that can inform spatially-defined and targeted management of chemical impacts on the aquatic (freshwater) environment. The derivation of ecosystem service specific EQS values also helps in communicating and highlighting the incremental benefits of improving water quality. A Tier I assessment focusing on protecting ecosystem service providing units was successfully undertaken based on available ecotoxicological effects data for each chemical. However, Tier II and Tier III assessments require further scientific research and tool development to quantify chemical impacts on ecosystem services delivery based on service providing taxa and their functional traits.
Freshwater ecosystems provide multiple direct and indirect contributions to human well-being (i.e. ecosystem services) including the provision of water, the removal of pollutants, flood alleviation and the opportunity for recreational activities, including swimming, fishing and tourism (Aylward et al., 2005; Stosch et al., 2017). However, freshwater biodiversity, which underpins the delivery of many ecosystem services, is under threat (Albert et al., 2021). Globally, freshwater species are going extinct more rapidly than terrestrial or marine species, with freshwater vertebrate species declining by an average of 83% since 1970 (WWF, 2018) and a third of freshwater insects being threatened with extinction (Sánchez-Bayo & Wyckhuys, 2019). In Europe, 59% of freshwater molluscs and 40% of freshwater fish are threatened with extinction (IUCN, 2015). The main threats to freshwater ecosystems are climate change, exploitation of species, harmful algal blooms, invasive alien species including infectious disease organisms, habitat loss via changes in land and water use, and water pollution (IPBES, 2019; Reid et al., 2019); the latter posing one of the most immediate threats to freshwater biodiversity (Dudgeon, 2019). Assessing how these biodiversity threats may impact on ecosystem service delivery is key to managing freshwater ecosystems for the benefit of people and nature. Here we address this challenge by describing a novel approach to derive environmental quality standards (EQSs) for freshwater ecosystem services that could help inform river basin management decisions.
EQSs are used to protect aquatic biodiversity and ecosystems from the adverse impacts of chemicals. Currently EQSs derived for single substances, are based on data generated from single species toxicity tests and are typically driven by the most sensitive species, irrespective of its functional role (EC, 2018). The current approach takes no account of species interactions or ecosystem processes and hence the links between the EQS, biodiversity, ecosystem functioning and ecosystem services are uncertain. Applying a novel ecosystem-service based approach to derive EQS values has the potential to help to reduce this uncertainty.
The ecosystem services concept recognises the multiple benefits provided to society by ecosystems and therefore provides a comprehensive framework for assessing environmental protection, restoration and enhancement initiatives (UN Environment, 2017). It can be used to define and assess environmental quality (Paetzold et al., 2010), to develop specific environmental protection goals (Nienstedt et al., 2012), to establish regulatory acceptable environmental concentrations (Devos et al., 2019), to inform River Basin Management Planning (Blackstock et al., 2015), to evaluate the implications of different policy options and interventions (Holt et al., 2016), and to integrate environmental policies and produce an holistic approach to environmental management (Maltby et al., 2018). The major challenge is how the concept can be made operational and integrated into existing regulatory policies and processes (Faber et al., 2019; Maltby et al., 2021).
The adoption of an ecosystem services approach to deriving EQS values implies the calculation of use-based standards that are relevant to ecosystem services provided by specific waterbodies. Use-based standards are not a new approach. For example, prior to adoption of the EU Water Framework Directive (WFD; 2000/60/EC), statutory water quality objectives (WQOs), introduced under the United Kingdom Water Resources Act 1991, recognised a range of water uses, each of which required specific quality standards necessary to support those uses. These uses included amenity provision (including water contact activities), different types of fishery (salmonid, cyprinid, migratory, marine fish, shellfish), abstraction for different water uses (potable, industrial, agricultural) and ecosystem protection (general and specific). Because use categories apply to specific water bodies (i.e., river stretches) they provide a framework to consider both the benefits to the local community and the actions that must be taken to protect them (Everard, 1994).
The EU Biodiversity Strategy to 2020 articulated the vision that “By 2050, European Union biodiversity and the ecosystem services it provides—its natural capital—are protected, valued and appropriately restored for biodiversity’s intrinsic value and for their essential contribution to human wellbeing and economic prosperity, and so that catastrophic changes caused by the loss of biodiversity are avoided.” (EU, 2011). More recently, the European Green Deal has been developed to improve the well-being and health of citizens by reducing pollution and protecting biodiversity, amongst other things (EC, 2019). The WFD is the main legislative instrument for protecting European freshwater ecosystems and EQSs are a key tool for regulating chemical contamination within the WFD. While not explicitly using the term ecosystem services, the WFD addresses the need to safeguard the benefits that people derive from the sustainable use of water ecosystems (Bouwma et al., 2018). Consequently, RBMPs need to consider a number of potentially competing objectives (e.g. fisheries, water abstraction, nature conservation) and are therefore consistent with the ecosystem services approach (Everard, 2012; Vlachopoulou et al., 2014; Spray & Blackstock, 2016). The consideration of cultural and recreational ecosystem services in river basin management planning also serves to emphasise the direct link between environmental and human health and wellbeing (Ravenscroft & Church, 2011; Ziv et al., 2016).
Here an innovative four-step tiered process is described that would enable ecosystem services to be considered within the EQS derivation process and illustrate part of the approach (Tier I) using two exemplar chemicals - a pyrethroid insecticide and polybrominated diphenyl ethers (PBDE). We also discuss the potential regulatory implications for using ecosystem services-based EQSs in practice. The approach is developed for EQSs derived in the context of the WFD, but it could be applied to any environmental standard setting.
The key steps involved in using an ecosystem services approach to derive EQS values for individual hazardous chemicals are illustrated in Figure 1. The process begins with identifying freshwater ecosystem services that are potentially impacted by exposure to each chemical. The next step is to identify the ecological components that provide the ecosystem services potentially impacted by each chemical. These service providers are the focus of the hazard assessments used to derive ecosystem service-specific EQS values (EQSES), which in turn are used to derive river basin unit-specific EQS values (EQSRBU)—depending on the services provided in each river basin unit (or water body).
FIGURE 1. Key steps in using an ecosystem services approach to derive river basin-specific EQS values. The process begins with identifying freshwater ecosystem services that are potentially impacted by exposure to toxic chemicals. The next step is to identify the ecological components that provide potentially impacted ecosystem services. These service providers are the focus of the hazard assessments used to derive ecosystem service (ES)-specific EQS values, which in turn are used to derive river basin unit-specific EQS values.
Freshwaters have the potential to deliver a wide range of ecosystem services, but not all water uses will be impacted by chemical exposure (e.g. power generation, navigation). The first step is to identify all relevant ecosystem services, ensuring that all water uses identified in River Basin Management Plans (RBMPs) and other relevant management plans are captured. The list should then be screened to focus on ecosystem services that are potentially affected by chemical contamination. As not all ecosystem services arise from living structures and processes, it is important to distinguish between those ecosystem services where the impact of chemical contaminants on ecosystem service delivery is dependent on their effects on living organisms (i.e. biotic ecosystem services, potentially threatened by chemical toxicity) and those ecosystem services where this is not the case (i.e. abiotic ecosystem services or outputs such as mineral supply, hydro-electric energy generation and thermal energy storage) (c.f. Common International Classification for Ecosystem Services (CICES) v5.1, (Haines-Young & Potschin, 2018)). Table 1 illustrates this process for an exemplar set of ecosystem services (Spray & Blackstock, 2016).
TABLE 1. An illustrative screening of freshwater ecosystem services. Ecosystem services are classified as ‘Provisioning’, ‘Regulating and maintenance’ and ‘Cultural’. Ecosystem service class codes are from CICES v5.1 and are the basis for deciding whether ecosystem services are classified as biotic or abiotic. The list of ecosystem services is adapted from Spray and Blackstock (2016). NFM is natural flood management.
It should be noted that some ecosystem services may be provided by both biotic and abiotic processes. For example, the ecosystem services of ‘Recreation on/in water’ will be dependent on the presence of enough water to enable the recreational activity to take place (i.e., boating or swimming) and the fact that the water is not toxic to humans (i.e., potable water - abiotic ecosystem output). However, the recreational activity may also be enhanced by the presence of biotic components of the waterbody (i.e., charismatic species, aesthetic value) that could be negatively impacted by chemical contamination. There may also be multiple CICES classes contributing to the same ecosystem service. For example, the regulation of water quality by toxicant removal may be either by bioremediation (CICES Class 2.1.1.1) or by filtration/sequestration (CICES Class 2.1.1.2).
The components of biodiversity that provide biotic ecosystem services are referred to as service providing units. Although this term was originally applied to populations only (Luck et al., 2003), the concept was extended to include multiple levels of ecological organisation (Kremen, 2005). Here we apply the term ‘service providing unit’ to service providers that may be individuals, populations, species assemblages (functional groups, communities) or habitats (Luck et al., 2009). An ecosystem service may be delivered by multiple service providing units and individual service providing units may be involved in delivering multiple ecosystem services (Schmera et al., 2017). The role of individual species in providing ecosystem services is a function of their species-specific traits (Bello et al., 2010; Días et al., 2013; Balvanera et al., 2014) and therefore a trait-based approach to identifying service providing units should be adopted (Brown et al., 2021).
For each biotic ecosystem service potentially affected by chemical contamination, Step 2 is to identify the key ecosystem function driving ecosystem service delivery and identify the ecological entities providing the key functions (i.e., service providing taxa) (Table 2). This process will be based on a combination of ecological knowledge, functional trait information and expert judgement. CICES v5.1 provides some information on the biophysical characteristics of ecosystems that underpin specific ecosystem services. Trait databases are available for freshwater invertebrates, algae, macrophytes and fish, and can be used to link the characteristics of species to ecological functions (e.g., Fishbase: http://www.fishbase.org; freshwaterecology. info: www.freshwaterecology.info).
TABLE 2. Illustrative ecosystem functions and service providers for biotic ecosystem services listed in Table 1.
Once service providing units have been identified, their susceptibility to chemical exposure can be assessed. A major challenge when implementing an ecosystem services approach to chemical risk assessment is how to extrapolate from what we measure—effects on a small number of species in standard laboratory toxicity tests—to effects on the large number of species underpinning ecosystem service delivery (Maltby et al., 2018). It should be noted, however, that this is also a challenge for the current EQS approach, which uses toxicity data for a limited number of species to protect all species that are potentially exposed to a chemical.
Under the WFD, maximum allowable concentration (MAC) EQSs and annual average (AA) EQSs, are derived for protecting aquatic organisms from acute and chronic effects, respectively. EQSs commonly relate to aqueous chemical concentrations (i.e. water EQS), but sediment or biota EQS values can also be derived. Biota EQS values have been set for some priority substances (including PBDEs) to protect against secondary poisoning of environmental taxa and/or to protect human consumers (EC, 2018).
EQSs are derived using either a deterministic approach, which takes the lowest credible toxicity datum and applies an assessment factor (AF) to extrapolate to a quality standard (QS), or a probabilistic approach, which uses species sensitivity distribution (SSD) modelling (EC, 2018). In SSD modelling, toxicity data for different species and/or trophic levels are quality-assessed (for reliability), species are then ranked according to toxicity threshold (e.g. no observed effect concentration) and a probabilistic model fitted to estimate the concentration which is hazardous to 5% of species (HC5). As for the deterministic approach an AF may also be applied to the HC5 to allow for the uncertainties in the available data, e.g. the fact that toxicity data are not available for all species and endpoints. Data from field studies and mesocosm studies may be used to derive QSs, but are more often used to inform the choice of the AF applied to laboratory data. A 2014 survey of Member States conducted on behalf of the Ministry of Environment and Food of Denmark, concluded that the majority of EQS values were derived using deterministic approaches (Vorkamp & Sanderson, 2016).
EQSES values that relate specifically to the direct impact of chemical contamination on human health via consumption of contaminated water or contaminated food can be derived using the deterministic approaches (based on toxicity data for mammalian test species/human surrogates) as per the current EQS derivation guidance (EC, 2018). However, EQSES for other biotic ecosystem services (often provided by multiple environmental species/taxa) could be derived using a tiered approach, beginning with an assessment of hazard to service providing taxa (Tier I) and ending with an assessment of hazard to ecosystem services delivery/provisioning (Tier III) (Figure 1).
A tiered effect assessment procedure is a central feature of some established chemical risk assessment schemes (e.g. plant protection products), with ecological realism and data complexity increasing through the tiers (EFSA PPR, 2013). The advantage of a tiered approach is that it provides the opportunity for a sequential refinement of the assessment process, depending on data availability, with each tier reducing the degree of uncertainty associated with the assessment. Reductions in uncertainty are reflected in lower AFs, potentially resulting in higher QS values (i.e. QS derived in lower tiers should always be more conservative than higher tiers). Where possible, separate EQS values should be derived for each ecosystem service of interest. Some EQSES may have a number of components, each with their own QS. For example, the EQSaquaculture is a function of the chemical’s toxicity to the service providing taxa (which are fed and reared on fish farms) plus an assessment of potential human health effects via consumption of contaminated food (i.e. bioaccumulation) whereas EQSfishing is a function of the chemical’s toxicity to the service providing taxa and their prey. In these cases, the EQSES is the lowest relevant QS value. The EQSbiodiversity protects general biodiversity and should therefore be based on all available toxicity data, which is comparable to the current objective for EQSs of protecting all aquatic life and the previous ‘general ecosystem’ WQO. In contrast, the EQSdesignated should focus on taxa that reflect the designated species or habitat (e.g., SSSI, Natura 2000) and is comparable to the previous ‘special ecosystem’ WQO (i.e., safeguard the special conservation interest for which the controlled water is identified (NRA, 1991)). Depending on the sensitivity of the designated taxa relative to non-designated taxa, the EQSdesignated may be lower than, the same as, or higher than the EQSbiodiversity (Table 3).
TABLE 3. Exemplar test species for biotic ecosystem services in Table 2 with corresponding EQS nomenclature. Exemplar ecosystem services include taxonomic groups not currently considered in EQS setting (e.g. regulation of water quality by microbes) and groups receiving limited coverage (e.g. regulation of water flow by rooted macrophytes). * algae used as surrogate primary producers if no macrophyte data are available.
Standard toxicity data for taxa representative of service providing units could be used in either deterministic or probabilistic approaches to derive EQSES values depending on the extent of the available dataset. Both these approaches apply the principle that protecting sensitive taxa within a service providing unit protects the ecosystem services they deliver. They also assume that the threshold value derived is sufficient to protect against indirect effects. Where there is evidence from single species studies that indirect effects on service providers may be more sensitive than direct effects, the key supporting taxa (e.g., food sources, habitat elements) should be included in the assessment of the threshold value. For example, where invertebrate fish prey are more sensitive than fish, the invertebrate toxicity data will be used to derive the threshold value. This is similar to the current approach used to derive QS values, but with the evaluation targeted on specific service providing taxa rather than all taxa.
An initial mapping of taxa used in toxicity test guidelines (i.e., OECD, ASTM and ISO) against ecosystem services has been undertaken (Maltby et al., 2018). In a deterministic approach, an AF would be applied to the lowest toxicity endpoint for each service providing taxa to derive the ecosystem service-specific EQS (Table 3). It is proposed that determination of the appropriate AF to apply should follow current EQS guidance (EC, 2018). As per the current EQS approach a MAC EQS would be derived based on acute toxicity data and an AA-EQS based on chronic toxicity data, if sufficient data available (EC, 2018). If only limited ‘base set’ standard toxicity data are available (e.g., Daphnia, algae, fish), the outcome of a deterministic assessment scheme may be similar to the current EQS derivation. However, the added value would be that this approach would identify which ecosystem services were potentially at risk and whether the risk was due to direct toxicity to service providing taxa or via trophic or other ecological interactions.
Under current WFD guidance, use of an SSD approach to derive an EQS is considered appropriate if the database contains preferably more than 15, but at least 10 NOECs/EC10s values, from different species covering at least eight taxonomic groups. An ecosystem services approach is more nuanced, with separate SSDs and subsequent EQS values being derived for each ecosystem service of interest. The question of appropriate taxonomic coverage would therefore need to be addressed within the context of the ecosystem service being assessed (i.e. test species should be relevant to the service providing taxa). For the majority of chemicals, the number of species for which there are toxicity data may often be greater for acute data than chronic data. It is therefore possible that AA-EQS for specific ecosystem services, may be based on acute SSDs, adjusted using appropriate acute-to-chronic ratios (May et al., 2016).
When using the ecosystem service framework to set specific protection goals, EFSA concluded that the relevant ecological component (entity) for most ecosystem services was population or functional group, rather than individual organisms, and in many cases the important attribute was biomass or abundance (Nienstedt et al., 2012). The abundance of populations or functional groups is a consequence of the effects of chemicals on the vital rates of individuals (growth, reproduction, survival), on species interactions (trophic interactions, competition), and for intermittent exposures, on recovery processes (e.g., dispersal behaviour, generation time). The approach outlined in Tier I addresses the direct effects of chemical exposure on individual vital rates and population responses of some service providing taxa (e.g., algae). However, for most service providing taxa, additional approaches will be required to extrapolate from effects on individual service providers to effects on service providing units (i.e., populations, functional groups, habitats). These include empirical and mechanistic modelling approaches that take direct and indirect effects into account such as multi-species and mesocosm studies, as well as population and food web models (Faber et al., 2019).
Multispecies empirical approaches can provide useful insight into the population-level effects of chemicals on primary producers and invertebrates, but are less suitable for assessing effects on fish populations, since for example in mesocsom studies fish can exert unrealistic predation pressure. Multispecies studies integrate direct and indirect effects and can provide information on impact and subsequent recovery. However, they are resource intensive and constrained in terms of study duration and spatial scale (Caquet et al., 2000). Modelling studies overcome these constraints. Over the last 2 decades there have been considerable advances in the development of population models to aid chemical risk assessment. More recently studies have considered how mechanistic modelling can be used to assess chemical risk within an ecosystem services framework. For example, population models have been used to investigate the effects of an endocrine disruptor on a recreational trout fishery (Forbes et al., 2019) and food-web models have been used to illustrate the impact of an insecticide on a lake fishery (Galic et al., 2019).
The effects of a chemical on service providing units is not equivalent to the effects on ecosystem service delivery. The final refinement is therefore to extrapolate effect distributions for service providing units to ecosystem services using ecological production functions that quantify the contribution of service providing units to ecosystem service delivery (NRC, 2005; Daily & Matson, 2008; Tallis & Polasky, 2009). A recent detailed literature review has identified production functions for nearly all ecosystem services but quantitative models linking standard toxicity test species to ecosystem service provision were extremely rare (Faber et al., 2021). A complementary approach to quantitative ecological production functions is to develop evidence-based logic-chains that qualitatively link toxicity test data to ecosystem service delivery (Hayes et al., 2018). Again, these are relatively rare, but are more feasible to construct in the short term than quantitative ecological production functions.
Under the WFD, a chemical-specific EQS is derived, which should be protective of a wide range of surface water ecosystems in Europe. A single substance-specific EQS value is derived, which is applied to all water bodies. Adopting an ecosystem services-based approach to setting EQS values assesses risk to specific ecosystem services and provides the opportunity for assessments to be tailored to the profile of ecosystem services identified in specific RBMPs.
A subset of EQSES values derived in Step 3 (Table 3) can be selected to address river basin unit-specific use categories identified as important in RBMPs. A river basin unit may be defined as a river basin district, catchment, water body or river reach and should be appropriate to the RBMP objectives. A river basin unit-specific EQS (EQSRBU) can be determined either by taking the lowest relevant EQSES or by undertaking an analysis that prioritises ecosystem services and considers the interventions needed to protect them. Variation in EQSRBU values will reflect variation in the ecosystem services to be protected, which in turn reflect variation in RBMP objectives.
The Tier I approach (Step 3 and Step 4) was applied to two exemplar chemicals: a pyrethroid insecticide and polybrominated diphenyl ethers (PBDEs). Maximum acceptable concentration standards (MAC-EQSES) and annual average standards (AA-EQSES) were calculated for ecosystem service bundles for three hypothetical river basin units (RBU1, RBU2 and RBU3) (Table 4). Eight ecosystem services were considered in total: aquaculture, drinking water provision, regulation of water quality, regulation of water flow, habitat provision, recreational fishing, scientific/educational value, existence value. MAC-EQSES values were based on acute data and AA-EQSES values were based on chronic data and the lowest relevant EQSES values were used to derive MAC-EQSRBU and AA-EQSRBU values for each of the three river basins. The EU EQS dossier for each chemical was used to source toxicity data and assessment factors. Human health EQS values for fish consumption and drinking water consumption were taken directly from the dossiers (see Supplementary Material).
Acute toxicity data were available for algae (1 species), invertebrates (16 species) and fish (11 species, including two salmonid and two cyprinid species). There were no toxicity data for macrophytes and algae were very insensitive to the chemical (i.e., toxicity threshold greater than solubility). Chronic toxicity data were available for algae (1 species), invertebrates (4 species) and fish (2 species, including a cyprinid). Again algae were insensitive with the chronic toxicity threshold being greater than the chemical’s solubility.
Toxicity data were used to derive QS values for: salmonids, cyprinids, fish, primary producers, invertebrates and ‘all taxa’ (Table 5). The lack of macrophyte data meant that algal data had to be used to derive QSprimary producers. There were sufficient acute data to use SSDs to derive MAC-QSinvertebrates, MAC-QSfish and MAC-QSall taxa. In the absence of chronic salmonid data, the lowest fish NOEC value was used to calculate AA-EQSsalmonid (Supplementary Material).
TABLE 5. Service provider quality standards (QS) and ecosystem services-specific EQS values for a pyrethroid insecticide. Maximum acceptable concentration (MAC) standards are based on acute data and annual average (AA) standards are based on chronic data.
MAC-EQSaquaculture was equivalent to the MAC-QSsalmonid whereas AA-EQSaquaculture was the lowest of QSsalmonid and QSfish consumption. For this chemical, AA-EQSaquaculture was driven by direct toxicity to fish. To account for both direct toxicity and indirect (trophic) effects, EQSfishing was the lowest of QSsalmonid/cyprinid and QSinvertebrate. Both the MAC-EQSfishing and AA-EQSfishing were driven by indirect effects on fish via toxicity to invertebrate prey. EQSdesignated is given as a range of values, but would be specified at the river basin unit level, depending in local designations.
Acute toxicity data were available for algae (1 species), invertebrates (1 species) and fish (2 species, including one salmonid). There were no toxicity data for macrophytes. Chronic toxicity data were available for algae (1 species), invertebrates (1 species) and fish (2 species, including a salmonid).
Toxicity data were used to derive QS values for: fish, primary producers, invertebrates and ‘all taxa’ (Table 6). The lack of macrophyte data meant that algal data had to be used to derive QSprimary producers. Limited data availability meant it was possible to calculate separate QS values for salmonids but not for cyprinids. The QSfish was based on the lowest fish toxicity value. Limited data also meant that the QSall taxa was based on the lowest QS values for the three taxonomic groups. In contrast to the pyrethroid insecticide example, for this chemical, human health effects of consuming contaminated fish drove AA-EQSaquaculture. The MAC-EQSfishing and AA-EQSfishing,salmonid was driven by indirect effects, but the AA-EQSfishing,cyprinid was driven by direct toxicity to fish.
TABLE 6. Service provider quality standards (QS) and ecosystem services-specific EQS values for PBDE. Maximum acceptable concentration (MAC) standards are based on acute data and annual average (AA) standards are based on chronic data.
EQS designated for a particular river basin unit (EQSRBU) is the lowest EQSES identified for that river basin unit. For the pyrethroid insecticide, the MAC-EQSRBU ranged from 0.56 ng/l to 10.4 ng/l and AA-EQSRBU ranged from 0.082 ng/l to 3 ng/l. The current statutory MAC-EQS for freshwater is 0.6 ng/l and the current AA-EQS is 0.08 ng/l. For PBDE, the MAC-EQSRBU ranged from 0.49 × 10–3 μg/l to 0.21 μg/l and the AA-EQSRBU ranged from 4.9 × 10–8 μg/l to 0.049 μg/l. The current statutory MAC-EQS for freshwater is 0.14 μg/l and the current AA-EQS is 4.9 × 10–8 μg/l (Table 7).
TABLE 7. MAC-EQSES and AA-EQSES values for a pyrethroid insecticide and a PBDE for three hypothetical river basin units (RBU 1, RBU 2, RBU 3). Values in bold denote the critical EQSES values, which are used to derive the overall MAC-EQSRBU and AA-EQSRBU for each river basin given in the bottom row of the table.
Because standards are set for individual ecosystem services, and ecosystem services profiles vary between river basin units, the ecosystem services determining the EQSRBU vary between river basin units and between chemicals. For example, as is currently the case, QS values for drinking water consumption only apply to those waterbodies where drinking water abstraction is relevant and QS values for secondary poisoning and the human health impacts of consuming contaminated fish are only calculated for chemicals that bioaccumulate. For RBU 1, recreational fishing was the ecosystem service at most risk from the pyrethroid insecticide but drinking water provision was most at risk from PBDE. Invertebrate taxa designated for nature conservation would also be at high risk from the pyrethroid insecticide. For RBU 2, fish aquaculture was the ecosystem service most at risk from both chemicals, but whereas the risk is driven by toxicity to fish for the pyrethroid insecticide, human health impacts of consuming contaminated fish drove the risk for PBDE. For RBU 3, recreational fishing and ‘biodiversity’ (existence value) were at greatest risk to both chemicals. Invertebrate designated taxa were at greatest risk from acute and chronic exposure to the pyrethroid insecticide. However, whereas invertebrate designated taxa were at greatest risk from acute exposure to PBDE, designated fish taxa were at greatest risk from chronic exposure to PBDE (Table 6).
In this scoping study, we proposed a four-step, tiered approach to the derivation of ecosystem services-based EQSs and explored the feasibility of the proposed Tier 1 assessment (pertaining to service providing taxa) for two exemplar chemicals and three hypothetical river basin units. We have demonstrated that Tier I assessment should be feasible for priority pollutants given current knowledge and tools, although data availability may limit the resolution of the assessment of some ecosystem services, especially those dependent on microbes and plants. The application of an ecosystem services approach to EQS setting, will require the development of an agreed list of freshwater ecosystem services that are relevant to river basin management planning and the mapping of national freshwater taxa to selected ecosystem services using trait-based approaches (i.e. service provider identification). Tier II and Tier III assessments require further scientific research and tool development. These include the development of empirical and mechanistic models to assess risk to service providing units and the development of evidence-based logic chains and quantitative ecological production functions for assessing risk to ecosystem service delivery.
An ecosystem services approach to setting EQS values provides greater transparency of what is, and what is not, being protected in specific river basin units and facilitates the development of standards that address the needs of individual RBMPs. Identification of targeted and likely most effective mitigation measures for chemicals could potentially be assisted by specifying EQSES values for each service and tracking progress in achievement following specific interventions. For example, for RBU 1, the order of ecosystem service protection as a result of interventions to reduce PBDE exposure would be: EQShabitat > EQSbiodiversity = EQSfishing, salmonid = EQSdesignated >> EQSdrinking water. This approach could help discern the effectiveness of RBMPs (EC, 2018) by indicating the incremental benefits of improving water quality for ecosystem services with low to high susceptibility to a given chemical.
The benefits of integrating an ecosystem services approach in to decision making within the context of the WFD have been articulated by several authors (Vlachopoulou et al., 2014; Blackstock et al., 2015) and are listed in the ‘EU guidance on integrating ecosystems and their services into decision-making’ (EC, 2019) as: emphasising the social and economic benefits of achieving good ecological status; enabling further integration of water policy with sector policies; justifying the costs of aquatic ecosystems restoration; motivating even broader public engagement; providing cost-effective solutions to mitigating flood risks and impacts. An ecosystem services framework can be used both for EQS setting and for the assessment of ecological condition (Brown et al., 2021) providing the potential for a more robust and transparent alignment between chemical and biological quality assessments. Using an ecosystem services approach both to set EQS values and to assess ecological condition would enable a more direct and nuanced evaluation of whether interventions to achieve EQS values deliver the desired ecological outcomes. Tracking EQSES achievement over time will help inform/corroborate cost-benefit analyses, which currently lack evidential data. In addition to monitoring the cost of mitigation measures versus EQSES achievement, cost-benefit analysis would be aided further by quantifying the value of improved and wider ranging ecosystem service delivery over time.
Adopting an ecosystem services approach offers the potential to use location-specific EQSRBU values to set discharge consents and to set EQSs for different ecosystem services, rather than just the single national EQS value as currently. However, realising this opportunity would require modification to the current processes used in permitting and in waterbody classification. Although permit setting is location specific, it is currently based on a single universal EQS value for each chemical.
For ecosystem services-based EQS setting, the EQS designated for a particular river basin unit (EQSRBU) will be the lowest EQSES identified in each specific case. For RBU 1, the minimum AA-EQSES for the pyrethroid insecticide is equal to EQSbiodiversity and EQSdesignated, whereas for PBDE it is equal to EQSdrinking water. This case example is entirely consistent with the current process for water quality classification and permitting of chemical discharges i.e. based on a minimum threshold exposure concentration to protect all aquatic life and including risks to human health via consumption of contaminated water for sites used for drinking water abstraction. For RBU 2, the minimum AA-EQSES is equal to EQSaquaculture for both exemplar chemicals. However, whereas direct toxicity to salmonid fish is the primary risk posed by the pyrethroid insecticides, the main risk posed by PBDE is to human health via consumption of contaminated fish. The AA-EQSRBU2 for PBDE is entirely consistent with the current approach of considering human health effects of bioaccumulative compounds when setting EQS value. The AA-EQSRBU2 for the pyrethroid insecticide is higher than the current AA-EQS, but the approach is consistent with the historic process for water quality classification and permitting of chemical discharges i.e. based on a use-specific threshold exposure concentration (NRA, 1991).
In conclusion, our novel ecosystem services approach to the setting of EQSs provides many potential benefits. These include the provision of a conceptual framework that directly links environmental quality standards and ecosystem condition to societal benefits and integrates across multiple stressors, scales, habitats and policies. Improved transparency in communicating the risks of chemical exposure to ecosystems and in identifying the societal benefits of costly programmes of measures. An indication of the incremental benefits of improving water quality to achieve EQSs designed to protect a range of prioritised ecosystem services with low to high susceptibility to a given chemical. The provision of the opportunity to compare measured chemical concentrations against specific EQSES values and therefore to indicate which ecosystem services are being protected, even if the minimum threshold EQSRBU is exceeded. The provision of a common approach for setting environmental standards and for assessing ecosystem condition retrospectively, thereby enabling validation of EQS setting and minimising the tendency for mismatches between chemical and biological quality. A Tier I assessment should be feasible for priority pollutants, for example, those listed under the WFD, given current knowledge and tools, although data availability may limit the resolution of the assessment of some ecosystem services. There is also a need to agree the key ecosystem services to be assessed and the relevant service providers. Tier II and Tier III assessments require further scientific research and tool development and are provided as an indication of what a future scheme may look like. A possible next step in exploring the feasibility of implementing an ecosystem services approach to EQS setting would be to undertake a proof-of-concept study in which Tier one ecosystem services-based EQS values, derived for a suite of chemicals with different toxic modes of action, are used to generate EQS values for case study river basin units.
The original contributions presented in the study are included in the article/supplementary material, further inquiries can be directed to the corresponding author.
LM and AB undertook the research and HW provided technical input in EQS setting and environmental regulations. All authors contributed to manuscript preparation.
This project was funded by the Environment Agency for England.
The authors declare that the research was conducted in the absence of any commercial or financial relationships that could be construed as a potential conflict of interest.
All claims expressed in this article are solely those of the authors and do not necessarily represent those of their affiliated organizations, or those of the publisher, the editors and the reviewers. Any product that may be evaluated in this article, or claim that may be made by its manufacturer, is not guaranteed or endorsed by the publisher.
The Supplementary Material for this article can be found online at: https://www.frontiersin.org/articles/10.3389/fenvs.2022.932161/full#supplementary-material
Albert, J. S., Destouni, G., Duke-Sylvester, S. M., Magurran, A. E., Oberdorff, T., Reis, R. E., et al. (2021). Scientists’ warning to humanity on the freshwater biodiversity crisis. Ambio 50, 85–94. doi:10.1007/s13280-020-01318-8
Aylward, B., Bandyopadhyay, J., Belausteguigotia, J.-C., Börkey, P., Cassar, A., Meadors, L., et al. (2005). “Freshwater ecosystems services,” in Human well-being: Policy responses, volume 3, Millennium Ecosystem Assessment. Editors K. Chopra, R. Leemans, P. Kumas, and H. Simons (Washington: Island Press), 215–258.
Balvanera, P., Siddique, I., Dee, L., Paquette, A., Isbell, F., Gonzalez, A., et al. (2014). Linking biodiversity and ecosystem services: Current uncertainties and the necessary next steps. Bioscience 64, 49–57. doi:10.1093/biosci/bit003
Bello, F., Lavorel, S., Díaz, S., Harrington, R., Cornelissen, J. H. C., Bardgett, R. D., et al. (2010). Towards an assessment of multiple ecosystem processes and services via functional traits. Biodivers. Conserv. 19 (10), 2873–2893. doi:10.1007/s10531-010-9850-9
Blackstock, K., Martin-Ortega, J., and Spray, C. (2015). “Implementation of the European Water Framework Directive: What does taking an ecosystem services-based approach add?,” in Water ecosystem services: A global perspective. Editors J. Martin-Ortega, R. C. Ferrier, I. J. Gordon, and S. Khan (Cambridge: University Press), 57–64.
Bouwma, I., Schleyer, C., Primmer, E., Winkler, K. J., Berry, P., Young, J., et al. (2018). Adoption of the ecosystem services concept in EU policies. Ecosyst. Serv. 29, 213–222. doi:10.1016/j.ecoser.2017.02.014
Brown, R., Marshall, S., Cooper, C., Whitehouse, P., Van den Brink, P., Faber, J., et al. (2021). Assessing the feasibility and value of employing an ecosystem services approach in chemical environmental risk assessment under the Water Framework Directive. Sci. Total Environ. 789, 147857. doi:10.1016/j.scitotenv.2021.147857
Caquet, T., Lagadic, L., and Sheffield, S. R. (2000). “Mesocosms in ecotoxicology (1): Outdoor aquatic systems,” in Reviews of environmental contamination and Toxicology. Editor G. Ware (New York, USA: Springer), 165.
Daily, G. C., and Matson, P. A. (2008). Ecosystem services: From theory to implementation. Proc. Natl. Acad. Sci. U. S. A. 105 (28), 9455–9456. doi:10.1073/pnas.0804960105
Devos, Y., Munns, W., Forbes, V., Maltby, L., Stenseke, M., Bussaard, L., et al. (2019). Applying ecosystem services for pre-market environmental risk assessments of regulated stressors. EFSA J. 17, e170705. doi:10.2903/j.efsa.2019.e170705
Días, A., Berg, M., De Bello, F., Van Oosten, A., Bila, K., Moretti, M., et al. (2013). An experimental framework to identify community functional components driving ecosystem processes and services delivery. J. Ecol. 101, 29–37. doi:10.1111/1365-2745.12024
Dudgeon, D. (2019). Multiple threats imperil freshwater biodiversity in the Anthropocene. Curr. Biol. 29, R960–R967. doi:10.1016/j.cub.2019.08.002
EC (2018). Technical guidance for deriving environmental quality standards. Guidance document No 27. Brussels: Publications Office, 2011.
EFSA PPR (2013). Guidance on tiered risk assessment for plant protection products for aquatic organisms in edge-of-field surface waters. EFSA J. 11 (7), 268. doi:10.2903/j.efsa.2013.3290
Everard, M. (1994). Water quality objectives as a management tool for sustainability. Freshw. Forum 4, 179–189.
Everard, M. (2012). Why does ‘good ecological status’ matter? Water Environ. J. 26, 165–174. doi:10.1111/j.1747-6593.2011.00273.x
Faber, J. H., Marshall, S., Brown, A. R., Holt, A., Van den Brink, P. J., Maltby, L., et al. (2021). Identifying ecological production functions for use in ecosystem services-based environmental risk assessment of chemicals. Sci. Total Environ. 791, 146409. doi:10.1016/j.scitotenv.2021.146409
Faber, J. H., Marshall, S., van den Brink, P. J., and Maltby, L. (2019). Priorities and opportunities in the application of the ecosystem services concept in risk assessment for chemicals in the environment. Sci. Total Environ. 651, 1067–1077. doi:10.1016/j.scitotenv.2018.09.209
Forbes, V. E., Railsback, S., Accolla, C., Birnir, B., Bruins, R. J. F., Ducrot, V., et al. (2019). Predicting impacts of chemicals from organisms to ecosystem service delivery: A case study of endocrine disruptor effects on trout. Sci. Total Environ. 649, 949–959. doi:10.1016/j.scitotenv.2018.08.344
Galic, N., Salice, C. J., Birnir, B., Bruins, R. J. F., Ducrot, V., Jager, H. I., et al. (2019). Predicting impacts of chemicals from organisms to ecosystem service delivery: A case study of insecticide impacts on a freshwater lake. Sci. Total Environ. 682, 426–436. doi:10.1016/j.scitotenv.2019.05.187
Haines-Young, R., and Potschin, M. (2018). Common international classification of ecosystem services (CICES) V5.1 and guidance on the application of the revised structure. http://www.cices.eu/.
Hayes, F., Spurgeon, D. J., Lofts, S., and Jones, L. (2018). Evidence-based logic chains demonstrate multiple impacts of trace metals on ecosystem services. J. Environ. Manag. 223, 150–164. doi:10.1016/j.jenvman.2018.05.053
Holt, A., Alix, A., Thompson, A., and Maltby, L. (2016). Food production, ecosystem services and biodiversity: We can’t have it all everywhere. Sci. Total Environ. 573, 1422–1429. doi:10.1016/j.scitotenv.2016.07.139
IPBES (2019). Summary for policymakers of the global assessment report on biodiversity and ecosystem services of the Intergovernmental Science-Policy Platform on Biodiversity and Ecosystem Services. Bonn Germany: IPBES Secretariat.
Kremen, C. (2005). Managing ecosystem services: What do we need to know about their ecology? Ecol. Lett. 8, 468–479. doi:10.1111/j.1461-0248.2005.00751.x
Luck, G. W., Daily, G. C., and Ehrlich, P. R. (2003). Population diversity and ecosystem services. Trends Ecol. Evol. 18 (7), 331–336. doi:10.1016/s0169-5347(03)00100-9
Luck, G. W., Harrington, R., Harrison, P. A., Kremen, C., Berry, P. M., Bugter, R., et al. (2009). Quantifying the contribution of organisms to the provision of ecosystem services. BioScience 59 (3), 223–235. doi:10.1525/bio.2009.59.3.7
Maltby, L., Brown, A., Faber, J., Galic, N., Van den Brink, P., Warwick, O., et al. (2021). Assessing chemical risk within an ecosystem services framework: Implementation and added value. Sci. Total Environ. 791, 148631. doi:10.1016/j.scitotenv.2021.148631
Maltby, L., van den Brink, P. J., Faber, J. H., and Marshall, S. (2018). Advantages and challenges associated with implementing an ecosystem services approach to ecological risk assessment for chemicals. Sci. Total Environ. 621, 1342–1351. doi:10.1016/j.scitotenv.2017.10.094
May, M., Drost, W., Germer, S., Juffernholz, T., and Hahn, S. (2016). Evaluation of acute-to-chronic ratios of fish and Daphnia to predict acceptable no-effect levels. Environ. Sci. Eur. 28, 16. doi:10.1186/s12302-016-0084-7
Nienstedt, K. M., Brock, T. C. M., van Wensem, J., Montforts, M., Hart, A., Aagaard, A., et al. (2012). Development of a framework based on an ecosystem services approach for deriving specific protection goals for environmental risk assessment of pesticides. Sci. Total Environ. 415, 31–38. doi:10.1016/j.scitotenv.2011.05.057
NRC (2005). Valuing ecosystem services: Toward better environmental decision-making. Washington DC: The National Academies Press.
Paetzold, A., Warren, P. H., and Maltby, L. L. (2010). A framework for assessing ecological quality based on ecosystem services. Ecol. Complex. 7 (3), 273–281. doi:10.1016/j.ecocom.2009.11.003
Ravenscroft, N., and Church, A. (2011). The attitudes of recreational user representatives to pollution reduction and the implementation of the European Water Framework Directive. Land Use Policy 28, 167–174. doi:10.1016/j.landusepol.2010.05.009
Reid, A. J., Carlson, A. K., Creed, I. F., Erika, J., Eliason, E. J., Gell, P. A., et al. (2019). Emerging threats and persistent conservation challenges for freshwater biodiversity. Biol. Rev. 94, 849–873. doi:10.1111/brv.12480
Sánchez-Bayo, F., and Wyckhuys, K. A. G. (2019). Worldwide decline of the entomofauna: A review of its drivers. Biol. Conserv. 232, 8–27. doi:10.1016/j.biocon.2019.01.020
Schmera, D., Heino, J., Podani, J., Erős, T., and Dolédec, S. (2017). Functional diversity: A review of methodology and current knowledge in freshwater macroinvertebrate research. Hydrobiologia 787 (1), 27–44. doi:10.1007/s10750-016-2974-5
Spray, C., and Blackstock, K. (2016). Optimising water framework directive river basin management planning Using an ecosystem services approach. Centre of Expertise for Waters CREW.
Stosch, K., Quilliam, R., Bunnefeld, N., and Oliver, D. (2017). Managing multiple catchment demands for sustainable water use and ecosystem service provision. Water 9, 677. doi:10.3390/w9090677
Tallis, H., and Polasky, S. (2009). Mapping and valuing ecosystem services as an approach for conservation and natural-resource management. Ann. N. Y. Acad. Sci. 62, 265–283. doi:10.1111/j.1749-6632.2009.04152.x
UN Environment (2017) A framework for freshwater ecosystem management. Volume 2: Technical guide for classification and target-setting.
Vlachopoulou, M., Coughlin, D., Forrow, D., Kirk, S., Logan, P., Voulvoulis, N., et al. (2014). The potential of using the Ecosystem Approach in the implementation of the EU Water Framework Directive. Sci. Total Environ. 470-471, 684–694. doi:10.1016/j.scitotenv.2013.09.072
Vorkamp, K., and Sanderson, H. (2016) EQS variation study: European environmental quality standards (EQS) variability study. Analysis of the variability between national EQS values across Europe for selected water framework directive river basin-specific pollutants, Scientific Report from DCE, Available at: https://dce2.au.dk/pub/SR198.pdf, accessed on December 01, 2016
Keywords: biodiversity, ecological trait, service provider, chemical hazard, river basin, water quality
Citation: Maltby L, Brown R and Wilkinson H (2022) Applying ecosystem services principles to the derivation of freshwater environmental quality standards. Front. Environ. Sci. 10:932161. doi: 10.3389/fenvs.2022.932161
Received: 29 April 2022; Accepted: 29 June 2022;
Published: 05 August 2022.
Edited by:
Victor Wepener, North-West University, South AfricaReviewed by:
Richard Greenfield, University of Johannesburg, South AfricaCopyright © 2022 Maltby, Brown and Wilkinson. This is an open-access article distributed under the terms of the Creative Commons Attribution License (CC BY). The use, distribution or reproduction in other forums is permitted, provided the original author(s) and the copyright owner(s) are credited and that the original publication in this journal is cited, in accordance with accepted academic practice. No use, distribution or reproduction is permitted which does not comply with these terms.
*Correspondence: Lorraine Maltby, bC5tYWx0YnlAc2hlZmZpZWxkLmFjLnVr
Disclaimer: All claims expressed in this article are solely those of the authors and do not necessarily represent those of their affiliated organizations, or those of the publisher, the editors and the reviewers. Any product that may be evaluated in this article or claim that may be made by its manufacturer is not guaranteed or endorsed by the publisher.
From Frontiers
Learn more about the work of our research integrity team to safeguard the quality of each article we publish.