- Department of Zoology, Kingsway Campus, University of Johannesburg, Auckland Park, South Africa
Microplastics are defined as plastics smaller than 5 mm down to 0.05 mm. These plastics enter the environment and undergo certain physical changes, most notably density changes and a relative increase of surface size. Microplastics can then release or absorb toxicants from the surrounding environment. These plastics may then enter the food chain from producers to top predators. In this study, microplastics were investigated in four study sites in the upper Vaal River, South Africa. The goal of the study was to determine the levels of plastics in water, sediment and a top predator, the benthic fish Clarias gariepinus. In this study, a 10% KOH digestion of water and fish, and density separation of sediment with NaCl (1.2 g cm3) was used to extract microplastics for identification. Microplastics were detected in water (3, 300 particles m−³), fish (7.47 particles per fish) and sediment (46.7 particles kg−1). Microplastic intake was not attributed to the microplastic shape or size of the fish that ingested it. This highlights the need to understand how niche-specific microplastic concentrations are, which will not only aid in quantifying microplastics accurately in the environment but to better understand how they may influence various ecosystems.
1 Introduction
Plastic pollution is highlighted as an increasing threat to the natural environment and humans (Walker, 2021). Plastic pollution has been detected around the globe from the hydrosphere, atmosphere, lithosphere, and biosphere (Li et al., 2018). The extent of plastic pollution has led to a global movement of environmental research on plastic and, more specifically, microplastics (Walker, 2021). Microplastics are plastic particles smaller than 5 mm in size, and their presence in the environment is extensively researched (Arthur et al., 2008; Li et al., 2018; Alimi et al., 2021). Microplastics can consist of a wide variety of plastic polymers, shapes, and origins, from plastic beads (primary microplastics) or plastics that have degraded from plastic products such as clothing, containers, or products with inner plastic linings (secondary microplastics) (Hidalgo-Ruz et al., 2012; Lusher et al., 2017; Gatidou et al., 2018; Guo and Wang, 2019).
When microplastics enter the environment, they quickly enter the food chain, being mistaken for a food source or bioaccumulating up the food chain into larger predatory animals (de Stephanis et al., 2013; Gatidou et al., 2018; Li et al., 2018). A build-up of microplastics in the gut may lead to intestinal blockages, leading to starvation, reduced breeding, increased immobility, and the death of the organism (Gatidou et al., 2018). Toxicity studies of microplastics indicate its damaging effects on multiple phyla of aquatic animals by merely being present in the surrounding environment (Barboza et al., 2018; Syakti et al., 2019).
Microplastics can act as vectors to toxicants in the surrounding environment (Guo and Wang, 2019). Various toxicants such as metals and pesticides have been found on microplastics in the environment (Collicutt et al., 2019; Guo and Wang, 2019). These plastics may then be a pathway for toxicant uptake and bioaccumulation (Barboza et al., 2018). The adverse effects and general distribution of microplastics were highlighted by Walker (2021), where the author indicated that plastic and microplastics could be correlated to twelve United Nations Sustainable Development Goals (SDGs), which was initially only correlated to two or three of these goals.
Plastic and microplastic research in Africa remains limited compared to other continents, but recent efforts to highlight microplastic pollution in Africa has been made (Naidoo et al., 2015; Nel and Froneman, 2015; Biginagwa et al., 2016; Lourenco et al., 2017; Nel at al., 2017; Nel et al., 2018; Akindele et al., 2019; Dalu et al., 2019; Mayoma et al., 2019; Toumi et al., 2019; Weideman et al., 2019; Weideman et al., 2020; Dahms et al., 2020; Alimi et al., 2021; Dalu et al., 2021; Deme et al., 2022; Masiá et al., 2022). In South Africa, research has been conducted on microplastics in the Vaal River system, determining microplastic concentrations in water, sediment, and the ability for long-distance transfer of microplastics (Weideman et al., 2019; Weideman et al., 2020). However, the presence of microplastic in biota here remains limited. The Vaal River is part of the natural habitat for the fish species Clarias gariepinus, a benthic omnivorous fish and top predator (Groenewald, 1964). This species is adapted to feed as both a predator and filter feeder (Groenewald, 1964). This species can grow to 1.7 m and 59 kg and has a broad feeding strategy from feeding on diatoms, invertebrates, large fish, birds, and small mammals (Groenewald, 1964; Skelton, 2001). Clarias gariepinus is considered economically valuable and is used commercially in fish farming and is a source of protein for subsistence fishers (Ali and Jauncey, 2004; Vitule et al., 2006).
Thus, it is important to investigate the presence of microplastic in this species. The feeding strategy, social, and economic value, of C. gariepinus, highlights the importance of the research question of whether these animals in the Vaal River are ingesting microplastics. It was hypothesised in this study that 1) C. gariepinus is ingesting microplastics, 2) the microplastic shapes (fibres, angular, round, and other) found in the gut would be random with no predominant shape found, 3) larger individuals would ingest higher amounts of microplastics, 4) microplastic concentrations in sediment would correlate to microplastic ingestion in fish throughout the system.
2 Methods
2.1 Site Selection
The Vaal River plays an important role in South Africa for economic activities and the environment before it joins the greater Orange River system, which finally flows into the Atlantic Ocean (Wepener et al., 2011). In total four sites (Vaal Dam- VD; After Dam Wall- ADW; Loch Vaal Club- LVC; Vaal Rus- VR) were selected for this investigation on the upper Vaal River (Figure 1). Sites were investigated in pairs, to determine if the large constructions such as the Vaal Dam wall and the weir of the Vaal River Barrage could influence microplastic distributions, which could provide a more holistic view of the microplastic loads in the river. Site pairs were chosen based on an above and below section of river that passes by the large town Vanderbijlpark (recorded population of 95,840 in the South African census of 2011, predicted to be 246,754 in 2021 by population estimator Worldometer) to assess the influence of the large urban environment on microplastic load (Statistics South Africa, 2011; Worldometer, 2021). Sampling sites upstream and downstream of the two barriers (Vaal Dam wall and the Vaal River Barrage weir) were selected for this study. Sampling was conducted during the early spring season over 2 weeks from 24 August 2019 to 6 September 2019 (2 days per site). The low flow conditions would reduce the influence that external factors such as large storms and flooding could have had on microplastic loads during high flow conditions in the study region.
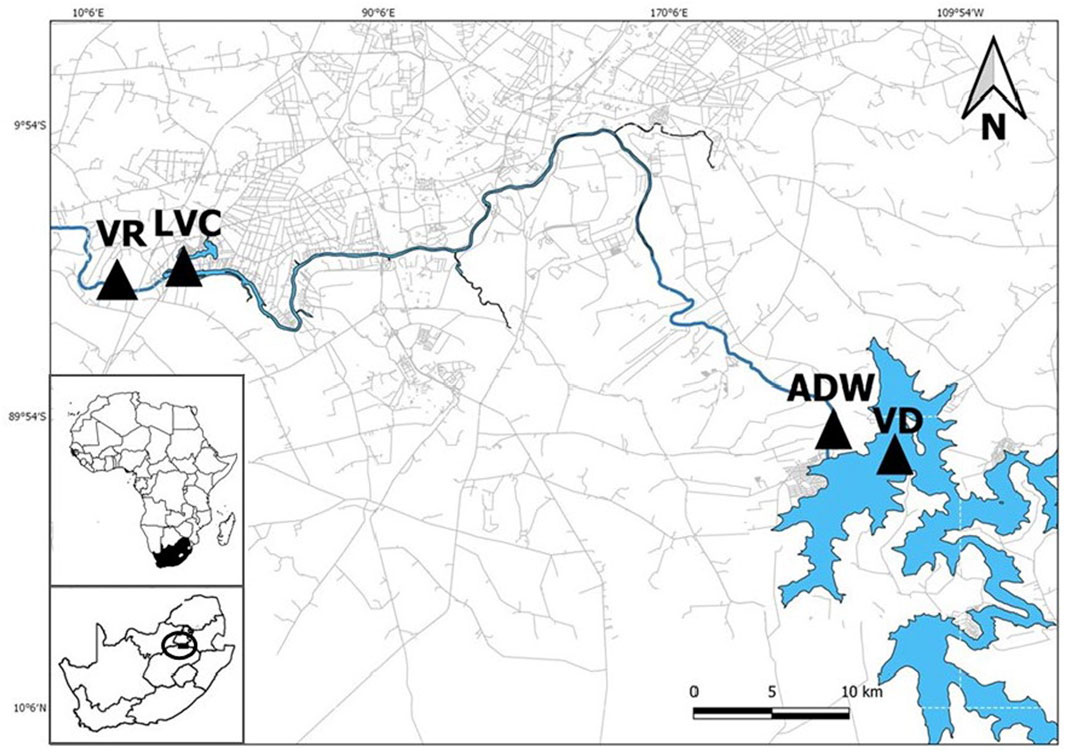
FIGURE 1. Map of sites investigated in the upper Vaal River (Blue-water, grey-roadways). From upstream to downstream: VD- Vaal Dam, ADW- After Dam Wall, LVC- Loch Vaal Club, and VR- Vaal Rus.
2.2 Sample Collection
2.2.1 In-situ Water Quality Parameters
Water quality parameters were measured to determine if water quality could be related to increased microplastics in the environment. Water quality parameters were measured at the start and end of each sampling day to determine the water quality at each site on the day sampling occurred, and whether any significant changes occurred during the start and end of the sampling day (morning and afternoon). The in-situ parameters that were measured included pH, conductivity, and Total Dissolved Solids (TDS). The parameters were measured using a Eutech multi-probe water quality meter. Flow information for the Vaal Dam and Vaal River Barrage on the days of sampling was collected from the “daily flow information 2019” data sheet as reported by Randwater and the Department of Water and Sanitation online (Randwater, 2019).
2.2.2 Water Collection
To determine a better representation for microplastic distribution in the river, multiple bulk water (6 replicates per site) samples were collected. Over 2 days of sampling at each site, 100 L of water was collected three times on each day per site (morning, midday, and afternoon) to provide a more holistic representation of microplastics at each site. Water collection, therefore, followed similar procedures as described by Collicutt et al. (2019). After a thorough washing before each sample collection, a large 25 L container was placed at the water surface with the opening facing upstream in the river and filled. Caution was taken not to kick up or collect any sediment or materials that may have been trapped in the sediment. The water was then filtered through a series of stainless-steel sieves (4,000, 212, and 53 μm), with a 20 cm diameter. Larger material was removed, and any content or plastic caught on the sieves was rinsed a standardized number of three times into a 50 ml container. The containers were then stored in a fridge at 5°C until laboratory analysis occurred.
2.2.3 Clarias gariepinus Collection
After obtaining the required permitting (permit number: CPE2-113), C. gariepinus were caught using sets of gill nets (Connell et al., 2020). Nets with mesh sizes of 70–120 mm were used. Nets were laid in the river during the day, parallel to the riverbank, and checked in 2-h intervals to remove any bycatch safely and to collect any of the target species while minimizing the risk of the fish expelling their gut content. The target fish that were caught were weighed, measured, and sexed. The fish were then euthanised following the procedure as described by the South African National Standards: The care and use of animals for scientific purposes (SANS, 2008). After blunt force trauma to the head, a small incision was made behind the head on the dorsal side of the fish and the spinal cord was severed. An incision was then made from the anus and extended to the gill chamber. Great care was taken not to cut or break any of the gastrointestinal tracts during the dissection. The complete gastrointestinal tract was then gently removed and placed in a clear ziplock bag and immediately frozen at −20°C to prevent any of the gut content from spilling out of the anus or oesophagus. Due to the nature and time of the study, no standardization of the number of a species to use in microplastic research had been established, with some published work using between 3 and 20 individuals of a particular species, 2–10 individuals per site or between 40 and 160 fishes in total (Rochman et al., 2015; Silva-Cavalcanti et al., 2017; Baalkhuyur et al., 2018; Bessa et al., 2018; Kumar et al., 2018; Collicutt et al., 2019; Slootmaekers et al., 2019). Due to time constraints and the availability of the fish species, a total of 39 fish were collected for the study. A total of six fish were collected from VD, followed by nine fish from ADW, 15 from LVC, and nine fish from VR.
2.2.4 Sediment Collection
Bulk sediment samples were collected at all sites after the collection of water samples to prevent cross-contamination. Approximately 2 kg wet weight (ww) of the top (≈10 cm depth) sediment was collected at each site and placed in a freezer at −20°C for microplastic extraction and sediment particle size analysis (Nel and Froneman, 2015; Collicutt et al., 2019; Dahms et al., 2020). The sediment was then placed in clear ziplock bags, negative control samples of distilled water rinsed from the bags were later taken to indicate possible contamination as described in the contamination control section (Nel and Froneman, 2015; Collicutt et al., 2019; Dahms et al., 2020). Challenges were found at LVC with regard to the depth of the environment. In total, approximately 800 g ww of sediment could be collected there. Therefore, only one replicate was analysed for sediment at each site for standardization.
2.3 Laboratory Analysis
2.3.1 Microplastic Extraction From Water
Material collected from the water samples was placed in glass beakers and covered with aluminium foil to prevent air contamination. The volume of the water was measured, and the approximate weight of KOH was calculated to create a 10% KOH solution to remove any organic matter in the water (Joint Research Centre, 2014). After 24 h of digestion at room temperature (≈25°C), the remaining content were placed in a covered, clean, glass Petri dish for microscopic analysis (Joint Research Centre, 2014).
2.3.2 Microplastic Extraction From Clarias gariepinus
The whole frozen gastrointestinal tract of C. gariepinus was thawed at room temperature and weighed. The outer surfaces of the whole gut were then rinsed with distilled water to remove any external contamination and the complete gastrointestinal tract was placed in a glass beaker. The intestines were then placed in an oven and left to dry at 50°C to aid in the digestion process (Collicutt et al., 2019). A 10% KOH solution was then added to the intestinal tissue to digest the organic matter but not the plastics that may have been ingested by the animal (Joint Research Centre, 2014). The beakers were then covered with aluminium foil, placed in the oven and then left to digest for 5 days at 50°C in the 10% KOH solution, a process adapted by the method used by the authors Rochman et al. (2015). The digested solution was then placed into 50 ml Falcon tubes and centrifuged at 3,000 rpm for 10 min and repeated until all the digested material was analysed (Karami et al., 2016). The supernatant and pellet were then placed in glass Petri dishes after every round of centrifuging for microplastic identification (larger volumes required more rounds of centrifuging to work through the digested solution). Both the supernatant and pellets were investigated to ease counting and prevent the loss of any microplastics that may not have been separated during centrifugation.
2.3.3 Microplastic Extraction From Sediment
Sediment was dried for 3 days at 50°C to determine the dry weight of the sediment. A single subsample of 500 g from each site was then used to determine microplastic contamination through density separation as described by Nel and Froneman (2015), Coppock et al. (2017) and GESAMP (2019). Microplastic density separation remains one of the primary methods in the separation of microplastics from sediment, however, some shortfalls may occur (Hidalgo-Ruz et al., 2012; Coppock et al., 2017). Density separation can have high recovery rates (99%) for large microplastics (1–5 mm), although smaller microplastics may have recovery rates of 40%–72% (Coppock et al., 2017). The density of the solution used may similarly influence the recovery rate of certain forms of highly dense plastics (Coppock et al., 2017). In this study, a hypersaline NaCl solution (density of 1.2 g cm −3) was used as a standardized method for comparison to other studies that have investigated freshwater microplastics in South African environments, however, denser microplastics such as PVC, PET, alkyd, and PVOH may have exceeded the density of the solution (Nel and Froneman 2015; Coppock et al., 2017; Nel et al., 2018; Dahms et al., 2020; Choong et al., 2021). The dried sediment was placed in a glass beaker and filled with the hypersaline solution and filled to approximately 500 ml. The sediment was stirred vigorously for 2 min and a further 18 h on an orbital shaker to agitate and dislodge any plastics that may have been trapped between the sediment particles (Nel et al., 2018; GESAMP, 2019). The sediment was left to stand for 6 h, allowing the sediment to settle and for microplastics to remain suspended (Coppock et al., 2017; GESAMP, 2019). The hypersaline solution was then washed through a series of stainless-steel sieves (4,000 and 53 μm). The NaCl density separation step was followed four times to increase the recovery of any microplastics that may not have been dislodged (Nel et al., 2018).
2.3.4 Sediment Characteristics
A subsample (100 g) of the oven-dried sediment (2.3.3) was then used to determine the sediment grain profile. The sediment grain profile was determined by shaking the 100 g of dried sediment through a series of stainless-steel sieves (4,000, 2,000, 500, 212, and 53 µm) on a mechanical sediment shaker. After 10 min the constituents were measured to determine the percentages of each grain size in the specific dimensions (ASTM, 2000; Cyrus et al., 2000; United States Environmental Protection Agency (USEPA), 2001).
2.3.5 Microplastic Identification
Microplastics were identified in a clean, rinsed glass Petri dish using a Carl Zeiss Stemi DV4 dissection microscope. Microplastics (5–0.05 mm) were identified and classified using the colour and shape of the plastics (Marine and Environmental Research Institute (MERI), 2015; Rochman et al., 2015; Windsor et al., 2019). The identification of microplastics followed a step-by-step guide of elimination, to determine conservative estimates for microplastics, as described by Hidalgo-Ruz et al. (2012) and the Marine and Environmental Research Institute’s guide to microplastic identification [Marine and Environmental Research Institute (MERI), 2015]. For an item to be classified as a plastic it had to pass all of the following checkpoints: no organic or cell structure present (fibres and filaments had to undergo further identification through a light microscope), fibres and filaments had to be evenly thick, items that broke apart by gently pressing it with a needle were not counted, if the object had a glass-like texture when pressed with a dissection needle it was excluded, a minimum of one in three items or vastly different items had to pass the “hot needle test” in which a needle is heated up and is gently moved past the item. If the item curled or contracted, it was accepted as plastic. Only if an item had passed all of the previously named checkpoints, was it counted as a microplastic (Hidalgo-Ruz et al., 2012; Joint Research Centre, 2014; Lusher et al., 2017). There was an increase in scrutiny when identifying items of smaller sizes (<0.5 mm), as the accuracy of visual identification decreases as the particle size decreases. A recent study by De Frond et al. (2022), highlighted that microscopy is an accurate method to determine microplastic counts down to 0.02 mm in size. Laboratories tested in the study by De Frond et al. (2022), had recovery rates of 92 ± 12% for microplastics larger than 0.02 mm in size.
2.4 Contamination Control
Contamination control followed similar steps as described by Marine and Environmental Research Institute (MERI) (2015), Coppock et al. (2017), and Lusher et al. (2017). Glassware, containers, and all equipment used were washed with a soap and acid bath system as described by Giesy and Wiener (1977) and was constantly washed before, during and after use with distilled water. Workbenches, microscopes, and scales were washed before use and any containers used had been covered with aluminium foil to prevent airborne contamination. Movement around the workspaces was limited at all times. A black lab coat and purple nitrile gloves were worn during the analyses for the ease of identifying contamination from clothing. To determine the extent of contamination, negative control samples of distilled water followed the step-by-step process of the samples from field extraction, drying, digestion, shaking, and counting, to determine any possible contamination in each of the tested matrices. If microplastics were detected in the controls, a similar value was removed over the whole group (Güven et al., 2017). Water, fish, and sediment had unique controls for the various methods each followed. Fish being the most likely to be contaminated during the dissection process were washed before the drying and digestion process.
2.5 Statistical Analysis
Statistical analysis was performed through the use of IBM SPSS version 26. Microplastic counts were log-transformed to allow the data to be more interpretable due to the extremely high and low counts detected between the matrices. The Shapiro Wilk test was conducted to test if the data was normally distributed and a Levene’s test of homogeneity of variance was conducted to determine if data met the assumptions for further statistical analysis. Pearson’s correlations were used to determine correlations between the various microplastic counts. To better understand microplastic loads in fish, the levels in this study were reported as particles per gram of fish ww, particles per gram of gut ww and particles per individual fish. A Spearman’s rank correlation test was conducted between the various reporting units showing significant correlations (p < 0.01) between particles g−1 of fish ww, particles g−1 gut and particles per fish. Microplastic loads were therefore reported as particles per fish to ease statistical analyses between the various matrices (water, biota, and sediment) and to relate to other studies which use similar reporting units. The Mann-Whitney U test was conducted to determine any significant differences between the shapes of microplastics ingested by C. gariepinus. A Spearman’s rank correlation was conducted to determine any correlations between total microplastics ingested by fish and the size of the animal. A map of the study site was created through QGIS version 3.10.
3 Results
3.1 In-situ Water Quality Parameters
At the sites representing the Vaal Dam, the average TDS ranged between 105.5 ppm (VD) and 106.5 ppm (ADW) while the sites representing the Vaal River Barrage downstream had higher TDS average readings of 425 ppm (LVC) and 545 ppm (VR). Conductivity showed a similar trend, (Figure 2), with average readings of 172 μs cm−1 (VD) and 172 μs cm−1 (ADW) upstream and higher average readings of 684 μs cm−1 (LVC) and 843 μs cm−1 (VR) downstream. Between all sites (Figure 2), pH ranged between a maximum of 9.44 (VD) and a minimum of 7.86 (LCV), through the system The discharge of water had been approximately 17.19 m3 s−1 from the Vaal Dam Wall and the Vaal River Barrage a flow rate of approximately 10.13 m3 s−1 on the days sampling took place as reported by Randwater (2019).
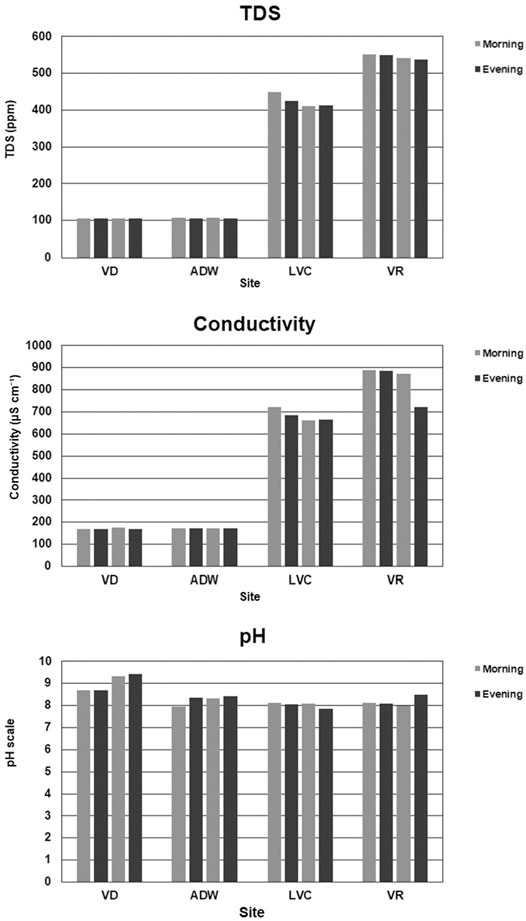
FIGURE 2. Bar graphs of water quality parameter Total Dissolved Solids- TDS (ppm), Conductivity (μs.cm−1) and pH in the morning and evening on the days sampled.
3.2 Water Microplastics
Microplastics were detected in all bulk water samples from each site. The highest concentration of microplastics was detected at ADW with an average of 12, 398 ± 7, 705 particles m−3, followed by LVC with an average of 478 ± 80.4 particles m−3, VR with an average of 237 ± 166 particles m−3 and the lowest readings at VD with an average of 85 ± 52.4 particles m−3 (Figure 3A). The Mann-Whitney U tests indicated that ADW had microplastic levels that were significantly higher (p < 0.01) compared to the other sites. Overall, angular shaped microplastics (92%) dominated water samples (Figure 4A), all of which were found at ADW. The second most abundant microplastic shape found were filaments (8%), followed by other shaped and round microplastics (each <1%). Microplastic colours (Figure 4B) found from the water had a similar pattern being dominated by green (92%) predominantly from ADW, followed by transparent/white, black, and other colours (all contributing approximately 2%), with red being the least abundant (<1%).
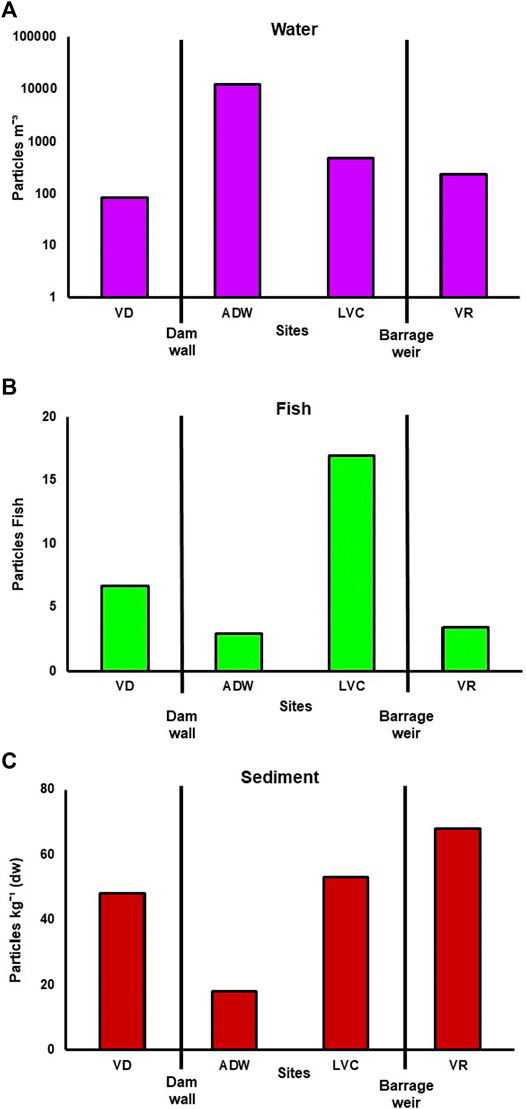
FIGURE 3. Bar graphs of the mean total number of microplastics found in water [(A)-logarithmic scale], fish (B) and sediment (C). dw—dry weight.
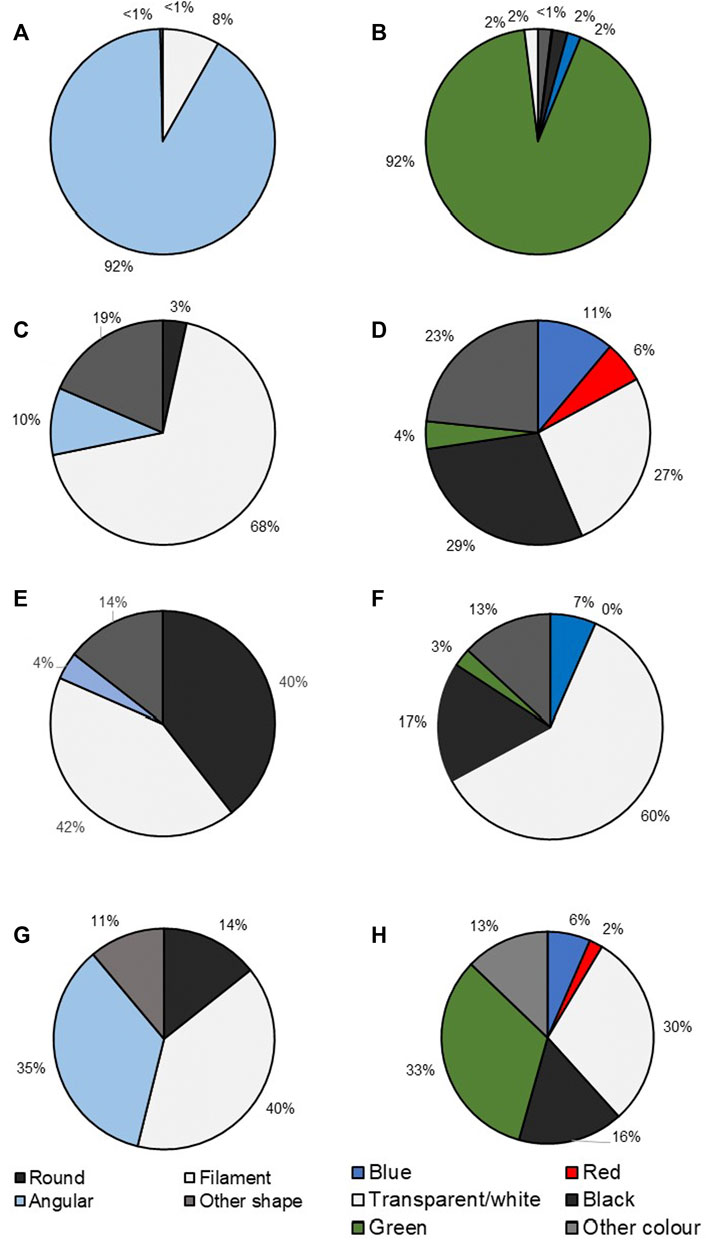
FIGURE 4. Pie charts illustrating the different forms and colours of microplastics collected in (A) (forms in water), (B) (colours in water), (C) (forms in Clarias gariepinus.), (D) (colours in Clarias gariepinus), (E) (forms in sediment), (F) (colour in sediment), (G) (all forms in total), and (H) (all colours in total) in the upper Vaal River.
3.3 Microplastic Ingestion by Clarias gariepinus
Microplastics were detected in C. gariepinus with 92.3% of all fish tested having at least one microplastic filament and a mean of 7.47 particles per fish. Fish collected in VD, LVC, and VR had a microplastic prevalence of 100% while ADW only had a 66% prevalence. In total, the abundance of microplastics in fish was highest at LVC (16.9 ± 8.9 particles per fish). This was followed by VD (6.67 ± 3.2 particles per fish), VR (3.4 ± 1.4 particles per fish), and ADW (2.88 ± 1.4 particles per fish). Although ADW had the lowest abundance of microplastics per fish, Figure 3B, was the only site where two pieces of large macroparticles were collected in the intestines of two separate fish. The most abundant microplastic shape found in fish were filaments (68%), followed by other shaped (19%), angular (10%) and round (3%) being the least abundant shape as seen in Figure 4C. The colours of the microplastics collected were dominated by black (29%), transparent/white (27%), and other colours (23%). This was followed by blue (11%), red (6%), and green (4%), the least abundant colour found (Figure 4D). A similarly random distribution seen for microplastics colours through the whole ecosystem (Figure 4H).
3.4 Sediment Microplastics
Microplastics was detected in sediment from all sites, with an average of 46.7 particles kg−1. Vaal Rus (VR) contained the highest abundance of microplastics (68 particles kg−1), followed by LVC (53 particles kg−1), VD (48 particles kg−1), and ADW containing the lowest abundance (18 particles kg−1). Microplastic abundances showed an increase from below the dam wall downstream to VR, Figure 3C. Filaments (42%) were overall the most prevalent microplastic shape found in the sediment, closely followed by round microplastics (40%) with other shaped objects (14%), and angular (4%) being the least prevalent shapes found. The most prevalent colours found were transparent/white (60%), followed by black (17%), other colours (13%), blue (7%), and green (3%), with no red coloured plastics found (Figures 4E,F).
3.5 Sediment Grain Profiles
Sediment grain profiles were similar across the sites with the exception of ADW. Particle sizes at VD, LVC, and VR were predominantly smaller than 212 μm, however, ADW was dominated by particles larger than 212 μm, Figure 5. Following the classification guide of Cyrus et al. (2000), it was determined that over 90% of the sediment profile at VD consisted of medium to very fine sand, with LVC (>65%) and VR (>75%) consisting of similar sediment profiles. ADW consisted primarily of (>50%) coarse and very coarse sand with 19% of the sediment consisting of gravel.
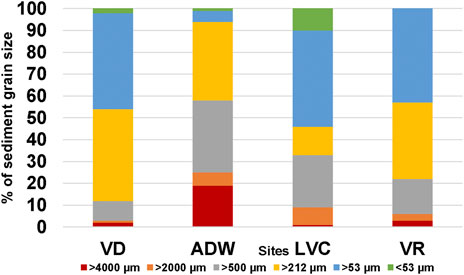
FIGURE 5. Bar graph indicating the percentage composition of the sediment grain profile at each site. Showing the larger sediment particles sampled at ADW.
3.6 Contamination Control
Control samples containing distilled water were prepared concurrently with all samples during preparation, extraction, and counting, to determine any possible contamination from the researcher. The total plastics found were subtracted over all samples to determine the influence of contamination over the whole dataset. The control for water contained six filaments (four black, one blue, and one transparent/white) found from the various samples taken. No contamination was detected in sediment and fish samples.
4 Discussion
Microplastics have been detected in multiple fish species and other organisms (Rochman et al., 2015). Once ingested these plastics could lead to a variety of negative health impacts. Microplastics polluted with toxicants such as metals or pesticides could be a vector, transporting toxicants into organisms (Guo and Wang, 2019). This could lead to increased bioaccumulation and reduce the physiological abilities of an organism to regulate ingested toxicants (Barboza et al., 2018; Stollberg et al., 2021). The microplastics detected in C. gariepinus of the Vaal River is the first indication that these organisms may be ingesting these pollutants in this system (Figure 3B). The microplastic levels in the fish (2.88–16.93 particles per fish with a mean of 7.47 particles per fish) were much higher than that found in the smaller benthic fish species Hoplosternum littorale (3.6 particles per fish) by Silva-Cavalcanti et al. (2017), juvenile Oncorhynchus tshawytscha (1.15 ± 1.41 particles per fish) by Collicutt et al. (2019) and in several fish species (0.2 ± 0.5 particles per fish) from a freshwater lake in Germany by Roch et al. (2019). Clarias gariepinus in comparison to several marine fish had on average more microplastics than these marine species reported by Rochman et al. (2015) (0 ± 2.5 particles per fish) with only the seven individuals of the family Carangidae (5.9 ± 5.1 particles per fish) and 17 fish of the species Decapterus macrosoma (2.5 ± 6.3 particles per fish) having similar levels of microplastics.
The prevalence of ingested microplastics in this study varied between sites with fish collected from ADW only having a 66% prevalence (Figure 3B). The site was located closely downstream of a dam wall which could have influenced the uptake of microplastics by C. gariepinus (Figure 1). The water here is more turbulent, and sediment grains are larger, creating an environment suited for microplastics to be transported downstream and not accumulated in sediment or biota (Dahms et al., 2020). Water samples indicated the highest concentration of microplastics here, however, they contained the lowest concentration in sediment found in this study (Figures 3A,C). Considering the species being a benthic fish it would have been in closer proximity to the sediment than the surface water where water samples were collected (Groenewald, 1964; Skelton, 2001). The study area ADW, located close to the dam wall, was receiving a constant input of water being released at 17.19 m3 s−1 from the Vaal Dam wall (Randwater, 2019). Studies have indicated how increased flow may lead to higher microplastic counts in water compared to sediment (Dahms et al., 2020). When the sediment grain profiles were analysed, it was found that ADW had a much larger sediment grain profile compared to the other sites (Figure 5). The authors Dahms et al. (2020), previously found relationships between how finer sediment may trap higher quantities of microplastics and larger grains may retain fewer. It could therefore be considered that the high concentration of microplastics collected in the water may not have had the opportunity to increase in density from biofilm growth, deposit in the sediment, and be ingested by C. gariepinus (Guo and Wang, 2019). The environmental factors that may contribute to the distribution of microplastics require further research.
Whether C. gariepinus may be ingesting microplastics directly or indirectly cannot be declared within this study. Researchers have found that some predatory organisms could be mistaking specific microplastic shapes or colours for a targeted food source (Ory et al., 2017). A Mann-Whitney U test indicated that no significant (p < 0.01) differences were detected between the ingested shapes found, although fibres were the most prevalent shape found in fish and the overall ecosystem (Figures 4C,G). This could indicate that C. gariepinus could be ingesting microplastics indirectly when feeding or it may have bioaccumulated up the food chain (Wang et al., 2019). A Spearman’s Rank correlation indicated no significant (p < 0.01) correlation between fish size and total microplastics ingested. The multiple feeding strategies of C. gariepinus and the large variety of food sources could lead to a variety of microplastic numbers and shapes ingested (Groenewald, 1964; Skelton, 2001). This could indicate that microplastics may be distributed widely within the ecosystem and more species must be investigated for the presence of ingested microplastics.
Exposure studies of the physiological responses of C. gariepinus to virgin microplastics have been conducted (Karami et al., 2016). The study found that Low-Density Polyethylene (LDPE) loaded with phenanthrene do lead to physiological stress even at low levels, which the authors stressed must be investigated further (Karami et al., 2016). Further research must be conducted to determine the mode of ingestion for microplastics in this fish species and whether the physiological response of C. gariepinus juveniles may be greater than those of adult fish.
The ingestion of microplastics by C. gariepinus could not be correlated to microplastic levels within the water or sediment samples collected at the sites, although, trends were seen between microplastic concentrations in sediment and fish (Figures 3B,C). Although not significant, an inverse Pearson’s r correlation of −0.883 was detected between sediment and water microplastic levels, which may again have been due to the increased flow at the sites ADW and VR (Randwater, 2019). Although biologically relevant due to the niche C. gariepinus inhabits, no statistical correlations or trends were detected between the species and the water or sediment microplastic counts (Figures 3B,C).
The water quality parameters indicated that a rapid decrease in water quality downstream after VD and ADW (Figure 2). The town of Vanderbijlpark is located between ADW and LVC, which may be a source of multiple environmental stressors including plastic pollution. Fish caught at LVC displayed the highest mean number of microplastics ingested (16.9 ± 8.9 particles per fish), but this was not identifiably related to the water quality itself. The mean number of plastics decreased downstream at VR (4 ± 1.4 particles per fish) (Figure 3A), while the water quality remained poor (Figure 2). The Vaal River has been under pressure from the release of sewage into the river that has been documented in South Africa (Hosken, 2018). Studies that investigated wastewater treatment plants have found that high quantities of microplastics may be released from these plants into rivers (Sun et al., 2019). This could possibly cause a drastic increase of microplastics into the river which may then be ingested by organisms as seen in this study. Further research must be conducted on the transport of microplastics within freshwater systems to determine how niche-specific organisms may be ingesting it (Huang et al., 2020).
5 Conclusion
This study found the presence of microplastics in C. gariepinus and how they have entered various parts of the ecosystem of the upper Vaal River. It also provides the first indication of microplastics in the fish species C. gariepinus, a top predatory fish and economically important species, in the upper Vaal River, South Africa. The microplastic levels found in the fish were higher than those recorded in similar studies of benthic freshwater fish, highlighting the need for further research on how the species may be influenced by microplastics. The results found in this study similarly highlights the difficulty to determine the correct environmentally relevant concentrations to use in toxicological studies with microplastics, as microplastics can accumulate and distribute in specific environmental conditions. More research must be completed on relating an organism to its environment and therefore the probability that it might be exposed to certain levels of microplastics. The hypotheses that: 1) microplastics will be detected in C. gariepinus was accepted, 2) the microplastic shapes (fibres, angular, round, other) found in the gut would be random with no predominant shape found is also accepted with no statistical evidence to show that C. gariepinus is mistaking specific microplastics as a food source, 3) The hypothesis that larger individuals would ingest larger quantities of microplastics than smaller individuals is not accepted, as no statistical evidence for this relationship was found, 4) the hypothesis that increased sediment microplastic levels would increase microplastics in fish could not be statistically indicated, however, a slight trend between study sites was detected. The authors stress that the transportation of microplastics in freshwater systems requires extensive research, to understand how it may be impacting freshwater aquatic biota.
Data Availability Statement
The datasets presented in this study can be found in online repositories. The names of the repository/repositories and accession number(s) can be found below: The data is in an MSc dissertation in the University of Johannesburg’s Institutional Repository. https://ujcontent.uj.ac.za/vital/access/manager/Repository?view=null&f0=sm_identifier%3A%22uj%3A43113%22&sort=sort_ss_title%2F. Raw data is also available on request from the corresponding author.
Ethics Statement
The animal study was reviewed and approved by University of Johannesburg, Faculty of Science Ethics Committee Ref # 2019-04-15/Greenfield_Dahms.
Author Contributions
HD, Conducted the experimental work, Wrote the first draft and lead author of the paper. GT Proof reading and editorial comments, Student co supervisor RG. Proof reading and editorial correction, Student supervisor, Submission of paper, PI on research project., Last author of the manuscript.
Funding
National Research Foundation Postgraduate Block Grant (grant number UID 128983).
Conflict of Interest
The authors declare that the research was conducted in the absence of any commercial or financial relationships that could be construed as a potential conflict of interest.
Publisher’s Note
All claims expressed in this article are solely those of the authors and do not necessarily represent those of their affiliated organizations, or those of the publisher, the editors and the reviewers. Any product that may be evaluated in this article, or claim that may be made by its manufacturer, is not guaranteed or endorsed by the publisher.
Acknowledgments
The authors would like to acknowledge the University of Johannesburg internal funding structures for funding this research and the National Research Foundation.
References
Akindele, E. O., Ehlers, S. M., and Koop, J. H. E. (2019). First Empirical Study of Freshwater Microplastics in West Africa Using Gastropods from Nigeria as Bioindicators. Limnologica 78, 125708. doi:10.1016/j.limno.2019.125708
Ali, M. Z., and Jauncey, K. (2004). Optimal Dietary Carbohydrate to Lipid Ratio in African Catfish Clarias gariepinus (Burchell 1822). Aquac. Int. 12, 169–180. doi:10.1023/B:AQUI.0000032065.28059.5b
Alimi, O. S., Fadare, O. O., and Okoffo, E. D. (2021). Microplastics in African Ecosystems: Current Knowledge, Abundance, Associated Contaminants, Techniques, and Research Needs. Sci. Total Environ. 755, 142422. doi:10.1016/j.scitotenv.2020.142422
Arthur, C., Baker, J., and Bamford, H. (2008). “International Research Workshop on the Occurrence, Effects, and Fate of Microplastic Marine Debris,” in Conference Proceedings of the International Research Workshop on the Occurrence, Effects, and Fate of Microplastic Marine Debris, Tacoma, WA. Sept. 9-11.
ASTM (2000). American Society for Testing and Materials Standards on Environmental Sampling. Standard Practice for Preparation of Sediment Samples for Chemical Analysis. Conshohocken, PA, USA: American Society for Testing and Materials, 163–165.
Baalkhuyur, F. M., Bin Dohaish, E.-J. A., Elhalwagy, M. E. A., Alikunhi, N. M., AlSuwailem, A. M., Røstad, A., et al. (2018). Microplastic in the Gastrointestinal Tract of Fishes along the Saudi Arabian Red Sea Coast. Mar. Pollut. Bull. 131, 407–415. doi:10.1016/j.marpolbul.2018.04.040
Barboza, L. G. A., Vieira, L. R., Branco, V., Carvalho, C., and Guilhermino, L. (2018). Microplastics Increase Mercury Bioconcentration in Gills and Bioaccumulation in the Liver, and Cause Oxidative Stress and Damage in Dicentrarchus labrax Juveniles. Sci. Rep. 8, 1–9. doi:10.1038/s41598-018-34125-z
Bessa, F., Barría, P., Neto, J. M., Frias, J. P. G. L., Otero, V., Sobral, P., et al. (2018). Occurrence of Microplastics in Commercial Fish from a Natural Estuarine Environment. Mar. Pollut. Bull. 128, 575–584. doi:10.1016/j.marpolbul.2018.01.044
Biginagwa, F. J., Mayoma, B. S., Shashoua, Y., Syberg, K., and Khan, F. R. (2016). First Evidence of Microplastics in the African Great Lakes: Recovery from Lake Victoria Nile Perch and Nile tilapia. J. Gt. Lakes. Res. 42, 146–149. doi:10.1016/j.jglr.2015.10.012
Choong, W. S., Hadibarata, T., and Ho Tang, D. K. (2021). Abundance and Distribution of Microplastics in the Water and Riverbank Sediment in Malaysia – A Review. Bioonterface Res. Appl. Chem. 11, 11700–11712. doi:10.33263/BRIAC114.1170011712
Collicutt, B., Juanes, F., and Dudas, S. E. (2019). Microplastics in Juvenile Chinook Salmon and Their Nearshore Environments on the East Coast of Vancouver Island. Environ. Pollut. 244, 135–142. doi:10.1016/j.envpol.2018.09.137
Connell, L. J., van Rensburg, G. J., Avenant-Oldewage, A., and Greenfield, R. (2020). Biomarker Responses in African Sharptooth Catfish, Clarias gariepinus (Burchell, 1822), as Indicators of Potential Metal and Organic Pollution along the Vaal River System, South Africa, Afr. J. Aquat. Sci., 45 (3), 1–12. doi:10.2989/16085914.2020.1735296
Coppock, R. L., Cole, M., Lindeque, P. K., Queirós, A. M., and Galloway, T. S. (2017). A Small-Scale, Portable Method for Extracting Microplastics from Marine Sediments. Environ. Pollut. 230, 829–837. doi:10.1016/j.envpol.2017.07.017
Cyrus, D. P., Wepener, V., Mackay, C. F., Cilliers, P. M., Weerst, S. P., and Viljoen, A. (2000). The Effects of Interbasin Transfer on the Hydrochemistry, Benthic Invertebrates and Ichythyofauna of the Mhlathuze Estuary and Lake Nsezi. WRC Report No. 722/1/00. Pretoria, South Africa: Water Research Commission.
Dahms, H. T. J., van Rensburg, G. J., and Greenfield, R. (2020). The Microplastic Profile of an Urban African Stream. Sci. Total Environ. 731, 138893. doi:10.1016/j.scitotenv.2020.138893
Dalu, T., Malesa, B., and Cuthbert, R. N. (2019). Assessing Factors Driving the Distribution and Characteristics of Shoreline Macroplastics in a Subtropical Reservoir. Sci. Total Environ. 696, 133992. doi:10.1016/j.scitotenv.2019.133992
Dalu, T., Banda, T., Mutshekwa, T., Munyai, L. F., and Cuthbert, R. N. (2021). Effects of Urbanisation and a Wastewater Treatment Plant on Microplastic Densities along a Subtropical River System. Environ. Sci. Pollut. Res. 28, 36102–36111. doi:10.1007/s11356-021-13185-1
De Frond, H., Thornton Hampton, L., Kotar, S., Gesulga, K., Matuch, C., Lao, W., et al. (2022). Monitoring Microplastics in Drinking Water: An Interlaboratory Study to Inform Effective Methods for Quantifying and Characterizing Microplastics. Chemosphere 298, 134282. doi:10.1016/j.chemosphere.2022.134282
de Stephanis, R., Giménez, J., Carpinelli, E., Gutierrez-Exposito, C., and Cañadas, A. (2013). As Main Meal for Sperm Whales: Plastics Debris. Mar. Pollut. Bull. 69, 206–214. doi:10.1016/j.marpolbul.2013.01.033
Deme, G. G., Ewusi-Mensah, D., Olagbaju, O. A., Okeke, E. S., Okoye, C. O., Odii, E. C., et al. (2022). Macro Problems from Microplastics: Toward a Sustainable Policy Framework for Managing Microplastic Waste in Africa. Sci. Total Environ. 804, 150170. doi:10.1016/j.scitotenv.2021.150170
Gatidou, G., Arvaniti, O. S., and Stasinakis, A. S. (2019). Review on the Occurrence and Fate of Microplastics in Sewage Treatment Plants. J. Hazard. Mater. 367, 504–512. doi:10.1016/j.jhazmat.2018.12.081
GESAMP (2019). Guidelines for the Monitoring and Assessment of Plastic Litter and Microplastics in the Ocean. GESAMP No. 99. Editors P. J. Kershaw, A. Turra, and F. Galgani. GESAMP Joint Group of Experts on the Scientific Aspects of Marine Environmental Protection, (IMO/FAO/UNESCO-IOC/UNIDO/WMO/IAEA/UN/UNEP/UNDP/ISA). Rep. Stud, 130.
Giesy, J. P., and Wiener, J. G. (1977). Frequency Distributions of Trace Metal Concentrations in Five Freshwater Fishes. Trans. Am. Fish. Soc. 106, 393–403. doi:10.1577/1548-8659(1977)106<393:FDOTMC>2.0.CO10.1577/1548-8659(1977)106<393:fdotmc>2.0.co;2
Groenewald, A. (1964). Observations on the Food Habits of Clarias gariepinus Burchell, the South African Freshwater Barbel (Pisces: Clariidae) in Transvaal. Hydrobiologia 23, 287–291. doi:10.1007/BF00043737
Güven, O., Gökdağ, K., Jovanović, B., and Kıdeyş, A. E. (2017). Microplastic Litter Composition of the Turkish Territorial Waters of the Mediterranean Sea, and its Occurrence in the Gastrointestinal Tract of Fish. Environ. Pollut. 223, 286–294. doi:10.1016/j.envpol.2017.01.025
Guo, X., and Wang, J. (2019). The Chemical Behaviors of Microplastics in Marine Environment: A Review. Mar. Pollut. Bull. 142, 1–14. doi:10.1016/j.marpolbul.2019.03.019
Hidalgo-Ruz, V., Gutow, L., Thompson, R. C., and Thiel, M. (2012). Microplastics in the Marine Environment: A Review of the Methods Used for Identification and Quantification. Environ. Sci. Technol. 46, 3060–3075. doi:10.1021/es2031505
Hosken, G. (2018). Sunday Times. Troops Battle Vaal Sew. “Invasion” Aim Is to Avert Day Zero Gauteng, Fix Collapsed Sewerage. WWW Document. URL. Available at: https://www.timeslive.co.za/sunday-times/news/2018-12-02-troops---battle-vaal--sewage-invasion/ (Accessed 10 15, 20).
Huang, D., Tao, J., Cheng, M., Deng, R., Chen, S., Yin, L., et al. (2020). Microplastics and Nanoplastics in the Environment: Macroscopic Transport and Effects on Creatures. J. Hazard. Mater. 407, 124399, In Press, Journal Pre-proof. doi:10.1016/j.jhazmat.2020.124399
Joint Research Centre (2014). Guidance on Monitoring of Marine Litter in European Seas. Luxembourg City: Institute for Environment and Sustainability, Publication office of the European Union. doi:10.2788/99816
Karami, A., Romano, N., Galloway, T., and Hamzah, H. (2016). Virgin Microplastics Cause Toxicity and Modulate the Impacts of Phenanthrene on Biomarker Responses in African Catfish ( Clarias gariepinus ). Environ. Res. 151, 58–70. doi:10.1016/j.envres.2016.07.024
Kumar, V. E., Ravikumar, G., and Jeyasanta, K. I. (2018). Occurrence of Microplastics in Fishes from Two Landing Sites in Tuticorin, South East Coast of India. Mar. Pollut. Bull. 135, 889–894. doi:10.1016/j.marpolbul.2018.08.023
Li, J., Liu, H., and Paul Chen, J. (2018). Microplastics in Freshwater Systems: A Review on Occurrence, Environmental Effects, and Methods for Microplastics Detection. Water Res. 137, 362–374. doi:10.1016/j.watres.2017.12.056
Lourenço, P. M., Serra-Gonçalves, C., Ferreira, J. L., Catry, T., and Granadeiro, J. P. (2017). Plastic and Other Microfibers in Sediments, Macroinvertebrates and Shorebirds from Three Intertidal Wetlands of Southern Europe and West Africa. Environ. Pollut. 231, 123–133. doi:10.1016/j.envpol.2017.07.103
Lusher, A. L., Welden, N. A., Sobral, P., and Cole, M. (2017). Sampling, Isolating and Identifying Microplastics Ingested by Fish and Invertebrates. Anal. Methods 9, 1346–1360. doi:10.1039/c6ay02415g
Marine and Environmental Research Institute (MERI) (2015). Guide to Microplastic Identification. Florida, United States of America: University of Florida.
Masiá, P., Mateo, J. L., Arias, A., Bartolomé, M., Blanco, C., Erzini, K., et al. (2022). Potential Microplastics Impacts on African Fishing Resources. Sci. Total Environ. 806, 150671. doi:10.1016/j.scitotenv.2021.150671
Mayoma, B. S., Mjumira, I. S., Efudala, A., Syberg, K., and Khan, F. R. (2019). Collection of Anthropogenic Litter from the Shores of Lake Malawi: Characterization of Plastic Debris and the Implications of Public Involvement in the African Great Lakes. Toxics 7, 64. doi:10.3390/toxics7040064
Naidoo, T., Glassom, D., and Smit, A. J. (2015). Plastic Pollution in Five Urban Estuaries of KwaZulu-Natal, South Africa. Mar. Pollut. Bull. 101, 473–480. doi:10.1016/j.marpolbul.2015.09.044
Nel, H. A., and Froneman, P. W. (2015). A Quantitative Analysis of Microplastic Pollution along the South-Eastern Coastline of South Africa. Mar. Pollut. Bull. 101, 274–279. doi:10.1016/j.marpolbul.2015.09.043
Nel, H. A., Hean, J. W., Noundou, X. S., and Froneman, P. W. (2017). Do microplastic Loads Reflect the Population Demographics along the Southern African Coastline? Mar. Pollut. Bull. 115, 115–119. doi:10.1016/j.marpolbul.2016.11.056
Nel, H. A., Dalu, T., and Wasserman, R. J. (2018). Sinks and Sources: Assessing Microplastic Abundance in River Sediment and Deposit Feeders in an Austral Temperate Urban River System. Sci. Total Environ. 612, 950–956. doi:10.1016/j.scitotenv.2017.08.298
Ory, N. C., Sobral, P., Ferreira, J. L., and Thiel, M. (2017). Amberstripe Scad Decapterus Muroadsi (Carangidae) Fish Ingest Blue Microplastics Resembling Their Copepod Prey along the Coast of Rapa Nui (Easter Island) in the South Pacific Subtropical Gyre. Sci. Total Environ. 586, 430–437. doi:10.1016/j.scitotenv.2017.01.175
Randwater (2019). Vaal Barrage and Vaal Dam Daily Flow Information 2019. WWW. Document. Available at https://www.reservoir.co.za/flow.htm (Accessed 10 15, 20).
Roch, S., Walter, T., Ittner, L. D., Friedrich, C., and Brinker, A. (2019). A Systematic Study of the Microplastic Burden in Freshwater Fishes of South-Western Germany - Are We Searching at the Right Scale? Sci. Total Environ. 689, 1001–1011. doi:10.1016/j.scitotenv.2019.06.404
Rochman, C. M., Tahir, A., Williams, S. L., Baxa, D. V., Lam, R., Miller, J. T., et al. (2015). Anthropogenic Debris in Seafood: Plastic Debris and Fibers from Textiles in Fish and Bivalves Sold for Human Consumption. Sci. Rep. 5, 14340. doi:10.1038/srep14340
SANS (2008). South African National Standard: The Care and Use of Animals for Scientific Purposes. Occupational Health and Safety Act. Pretoria, South Africa: South African Bureau of Standards (SABS) Standards Division.
Silva-Cavalcanti, J. S., Silva, J. D. B., França, E. J. d., Araújo, M. C. B. d., and Gusmão, F. (2017). Microplastics Ingestion by a Common Tropical Freshwater Fishing Resource. Environ. Pollut. 221, 218–226. doi:10.1016/j.envpol.2016.11.068
Skelton, P. (2001). A Complete Guide to the Freshwater Fishes of Southern Africa. Cape Town, South Africa: Struik Publishers.
Slootmaekers, B., Catarci Carteny, C., Belpaire, C., Saverwyns, S., Fremout, W., Blust, R., et al. (2019). Microplastic Contamination in Gudgeons (Gobio gobio) from Flemish Rivers (Belgium). Environ. Pollut. 244, 675–684. doi:10.1016/j.envpol.2018.09.136
Statistics South Africa (2011). Statistics South Africa. Statistics by Place. WWW Document. Available at: http://www.statssa.gov.za/?page_id=4286andid=11169 (Accessed 15-10-20).
Stollberg, N., Kröger, S. D., Reininghaus, M., Forberger, J., Witt, G., and Brenner, M. (2021). Uptake and Absorption of Fluoranthene from Spiked Microplastics into the Digestive Gland Tissues of Blue Mussels, Mytilus edulis L. Chemosphere 279, 130480. doi:10.1016/j.chemosphere.2021.130480
Sun, J., Dai, X., Wang, Q., van Loosdrecht, M. C. M., and Ni, B.-J. (2019). Microplastics in Wastewater Treatment Plants: Detection, Occurrence and Removal. Water Res. 152, 21–37. doi:10.1016/j.watres.2018.12.050
Syakti, A. D., Jaya, J. V., Rahman, A., Hidayati, N. V., Raza'i, T. S., Idris, F., et al. (2019). Bleaching and Necrosis of Staghorn Coral (Acropora Formosa) in Laboratory Assays: Immediate Impact of LDPE Microplastics. Chemosphere 228, 528–535. doi:10.1016/j.chemosphere.2019.04.156
Toumi, H., Abidli, S., and Bejaoui, M. (2019). Microplastics in Freshwater Environment: the First Evaluation in Sediments from Seven Water Streams Surrounding the Lagoon of Bizerte (Northern Tunisia). Environ. Sci. Pollut. Res. 26, 14673–14682. doi:10.1007/s11356-019-04695-0
United States Environmental Protection Agency (USEPA) (2001). Methods for Collection, Storage and Manipulation of Sediments for Chemical and Toxicological Analyses: Technical Manual. Washington DC, USA: United States Environmental Protection Agency, Office of Water, 194–208. EPA 823-B-01-002. Appendix G.
Vitule, J. R. S., Umbria, S. C., and Aranha, J. M. R. (2006). Introduction of the African Catfish Clarias gariepinus (Burchell, 1822) into Southern Brazil. Biol. Invasions. 8, 677–681. doi:10.1007/s10530-005-2535-8
Walker, T. R. (2021). (Micro)plastics and the UN Sustainable Development Goals. Curr. Opin. Green Sustain. Chem. 30, 100497. doi:10.1016/j.cogsc.2021.100497
Wang, W., Gao, H., Jin, S., Li, R., and Na, G. (2019). The Ecotoxicological Effects of Microplastics on Aquatic Food Web, from Primary Producer to Human: A Review. Ecotoxicol. Environ. Saf. 173, 110–117. doi:10.1016/j.ecoenv.2019.01.113
Weideman, E. A., Perold, V., and Ryan, P. G. (2019). Little Evidence that Dams in the Orange-Vaal River System Trap Floating Microplastics or Microfibres. Mar. Pollut. Bull. 149, 110664. doi:10.1016/j.marpolbul.2019.110664
Weideman, E. A., Perold, V., and Ryan, P. G. (2020). Limited Long-Distance Transport of Plastic Pollution by the Orange-Vaal River System, South Africa. Sci. Total Environ. 727, 138653. doi:10.1016/j.scitotenv.2020.138653
Wepener, V., van Dyk, C., Bervoets, L., O’Brien, G., Covaci, A., and Cloete, Y. (2011). An Assessment of the Influence of Multiple Stressors on the Vaal River, South Africa. Phys. Chem. Earth, Parts A/B/C 36, 949–962. doi:10.1016/j.pce.2011.07.075
Windsor, F. M., Tilley, R. M., Tyler, C. R., and Ormerod, S. J. (2019). Microplastic Ingestion by Riverine Macroinvertebrates. Sci. Total Environ. 646, 68–74. doi:10.1016/j.scitotenv.2018.07.271
Worldometer (2021). South Africa Population (Live). WWW Document. Available at: https://www.worldometers.info/world-population/south-africa-population/ (Accessed 03 16, 2021).
Keywords: freshwater, Africa, sediment, fish, ingestion, pollution
Citation: Dahms HTJ, Tweddle GP and Greenfield R (2022) Gastric Microplastics in Clarias gariepinus of the Upper Vaal River, South Africa. Front. Environ. Sci. 10:931073. doi: 10.3389/fenvs.2022.931073
Received: 29 April 2022; Accepted: 06 June 2022;
Published: 21 June 2022.
Edited by:
Nico M. Van Straalen, VU Amsterdam, NetherlandsReviewed by:
Omowunmi H. Fred-Ahmadu, Covenant University, NigeriaAna Virginia Filgueiras, Spanish Institute of Oceanography (IEO), Spain
Copyright © 2022 Dahms, Tweddle and Greenfield. This is an open-access article distributed under the terms of the Creative Commons Attribution License (CC BY). The use, distribution or reproduction in other forums is permitted, provided the original author(s) and the copyright owner(s) are credited and that the original publication in this journal is cited, in accordance with accepted academic practice. No use, distribution or reproduction is permitted which does not comply with these terms.
*Correspondence: Richard Greenfield, rgreenfield@uj.ac.za