- 1Department of Microbiology, School of Sciences, P P Savani University, Surat, India
- 2Division of Applied & Environmental Microbiology, Industrial Wastewater Research Lab, Enviro Technology Limited, Ankleshwar, India
- 3Department of Biosciences, Veer Narmad South Gujarat University, Surat, India
Textile effluent generated during the dyeing process of textiles is a huge supplier to water toxicity all over the world. Textile dyes are the main toxic component found in the effluent sample which is difficult to treat. A bacterial consortium capable of decolourizing and degrading the textile dye acid orange is reported in this research article. The bacterial consortium was identified by 16 S rDNA sequence and phenotypic characteristics. It is composed of four strains i.e., Pseudomonas stutzeri (MW219251), Bacillus tequilensis (MW110471), Bacillus flexus (MW131645), Kocuria rosea (MW132411). The consortium was able to cause decolorization of azo dye acid orange (30 mg/L) in 23 h, when kept under static laboratory conditions. The optimal pH and temperature for color removal were pH 7.5 and 32°C, respectively. The decolorization of dye before and after was checked by UV-Visible absorption spectra. This gives evidence that decolorization was caused due to biodegradation. This was further assured by studying the reduction of biological oxygen demand (BOD5 (96%), chemical oxygen demand (COD) (79%), and TOC (total organic carbon) (54%) of the bacterial-treated water sample. The Fourier transform-infrared spectroscopy (FT-IR) spectroscopy and gas chromatography-mass spectroscopy (GC-MS) results confirmed the mineralization of the dye. The results indicate the effectiveness of the bacterial consortium SPB92 in the biodegradation of acid orange dye. This demonstrates that the consortium has immense potential and will serve as an important contributor to the treatment of textile wastewater.
Introduction
The treatment of polluted wastewater is a growing concern all over the world for decades. The ever-growing population and limited availability of fresh water in the ecosystem add up to the issue. The textile industry is known for generation of bulk amount of wastewater leading to immense water pollution (Gitis & Hankins, 2018). The effluent produced by the textiles has a huge amount of dye depositions in it. Dyes are a significant class of artificial organic compounds used regularly in the textile, leather, plastics, cosmetics, and food industries. They are the main constituents of common industrial pollutants (Bera & Tank, 2021b). Textile wastewater discharged from the industry is a composite assortment of many pollutants. This includes toxic compounds like organic chlorine, heavy metals, pigments, and dyes-based pesticides. These highly toxic pollutants should be removed before letting the effluents discharge into the environment. Dyes also prevent the penetration of light and oxygen transfer, which affects water bodies (Saratale et al., 2011). Nearly 90% of the textile dyes used during the coloring process are discharged directly into the active sludge samples in sewers. Many a time these unprocessed discharges are directly deposited in the rivers, resulting in severe water pollution. Roughly 100 L of water is utilized in the processing of just 1 kg textile fabric (Parisi et al., 2015). The use of bacteria and fungi to reduce the organic load is a newer approach in the tertiary wastewater treatment sector. Bacteria with their natural enzymatic activity are natural bioremediation and can serve great help in the degradation of dye molecules (Bera & Tank, 2021a). Biological treatment offers a cheaper and friendlier alternative to the treatment and reuse of wastewater in industrial processes (Karunya et al., 2014). The microorganism with degradation capabilities, when provided with optimal conditions improves the decomposition of organic substances. This allows the microorganism to cause faster metabolic activity and results in better efficiency. This also eliminates the use of chemicals and expensive physical treatment procedures (Sriram & Reetha, 2015). Appropriate environmental conditions like pH, aeration, and concentration of salt and temperature affect the potentiality of the microorganism (Khan et al., 2013). Synthetic dyes are easy to synthesize and are inexpensive, thus heavily used in textile industries. Acid orange (AO), a commercially used textile azo dye is degraded in the following study using the microbial culture (Mansour et al., 2007). It is an azo dye and is easily soluble in water. The toxicity is severe and it often affects the aquatic life as well as areas nearby the discharge sites (Singh et al., 2014). The study of physicochemical characterization and screening of original strains of bacteria isolated from the sites which are severely contaminated with dyes is the main idea of this research work. These bacteria are natural decomposers of dyes and help in the degradation and later mineralization of dyes and their intermediate metabolites (Patel et al., 2015). The decolorization method by microorganisms entails two ways, first by adsorption of the dye particles on its surface and second is by enzymatic actions produced during metabolic activities. The microorganisms capable of causing dye degradation usually have two main mechanisms. They either absorb the dyes on their surface or reduce them to intermediate metabolites by producing enzymes capable of degrading the dyes (Chen, 2006). The dyes have attractive bright colors, can easily dissolve in water thus are widely used for commercial purposes (Shah, 2014). The following study was focused on the isolation and characterization of an effective bacterial consortium, which has a notable capacity to degrade AO as the only carbon source. Different physicochemical parameters were optimized for effective dyeing decolorization (Nawaz & Ahsan, 2014). The degradation products of the dye were analysed by ultraviolet (UV-Vis) and FT-IR Spectroscopy. This was followed by a GC-MS study to know the metabolites and intermediates formed in the various stages of degradation. The mineralization was confirmed after the analysis. Going through the extremities of treating the water pollution caused by the textile industries and water scarcity issues in general, this research study will help in possible bioremediation.
Materials and Methods
Chemicals
The textile azo dye Acid Orange 3 (CI No. 6373-74-6; molecular formula- C18H13N4NaO7S molecular weight- 452.4 g/mol) was brought from a local textile industry from Pandesara area, Surat, India. The dye was of industrial grades and is commonly used textile dye in the textile industry. The chemicals used for experimentations were from Sigma—Aldrich (Missouri, United States) and Hi-media (India).
Culture Medium Compositions
MSM containing the following components (g/L): Sodium hydrogen phosphate (12.0), Ammonium nitrate (0.50), Magnesium chloride hexahydrate (0.10), Calcium Nitrate Tetrahydrate (0.050), Potassium dihydrogen phosphate (2.0), Iron (II) chloride tetrahydrate (0.0075) 10 ml of the solution of trace elements per liter. Track solution of elements was set in accordance with the following compositions (mg/L): Zinc sulfate (10.0), Manganese (II) Chloride (3.0), Cobalt (II) chloride (1.0), Nickel (II) chloride (2.0), Sodium molybdate dihydrate (3.0), boric acid (30.0), Copper (II) Chloride (1.0). The flasks without the culture were also incubated as a control batch. The medium was finally adjusted to a pH of 7.0 ± 1.0. The MSM medium was added with agar (1.9% w/v) before using it for the analysis of isolation and preservation of the pure culture.
Isolation and Identification of Bacterial Strain
For isolating the bacterial strains, the MSM medium was provided with 100 mg/L of AO. About 10 ml of inoculum extracted from the activated sludge was added to the medium. The enriched substrate suspension was kept for incubation at 120 rpm 32°C. After 6 days, 10 ml aliquots were administered and transferred to 100 ml of freshly prepared MSM medium comprising 100 mg/L of the dye AO. This procedure was repeated thrice before obtaining the pure strains. The dilution boards were used to harvest the colonies. This was analyzed based on colony morphology and characteristics. For maintaining the purity of culture, the strains were transferred to freshly prepared Luria Broth (LB) agar plates several times. The tests were performed in triplets and the culture was kept for incubation at 37°C. The identification of unknown bacterial strains was performed by using the 16 S rDNA gene sequencing technique. The Gene Amp PCR System was employed to amplify the rDNA fragments. The system comprised of 0.25 U rTaq polymerase, 1 μL DNA template, 10 × PCR buffer, 1.5 mM MgCl, 2,500 mM KCl, and 0.1% (w/v) glutin. The PCR system also contained a PCR buffer, which was comprised of 1.5 mM MgCl2, 0.25 U rTaq polymerase, 200 mM each deoxynucleoside triphosphate, 0.5 mM forward primer 27F (5′- AGAGTTTAGATCCTGCTCAG-3′), 0.5 mM reverse primer 1592R (5′TACCTTGTTACGACTT3′), and 2.5 mM dNTP. The adjustment of final volume of the buffer was done by adding 50 ml of double-distilled water to it. The incubation of tubes was carried out at 95°C for 5 min followed by a heat cycle. The denaturation was carried out at 94°C for 1 min using the primer. The chain extension was carried at normal and prescribed temperature and time as per the standard protocols i.e., 72°C for 1 min 30 s, followed by 72°C for 30 min a total of 30 cycles. Sequencing on both strands of PCR amplified fragments was performed using the dideoxy chain termination in an automated sequencer using the program’s Basic Lookup Search Tool (BLAST) and 16 S rDNA homology searches against NCBI. The database was performed to find the 16 S rDNA databases for similar sequences. The protocol for the genome sequencing has been adapted from (Kushwaha et al., 2019) (Jadhav et al., 2021).
Physico-Chemical Parameters for Optimization
The effectiveness of bacterial consortium on acid orange was calculated at various pH (4–10) and temperature values (20–50°C). Acquired optimal pH 7.5 and temperature at 32°C was considered appropriate for studying the decolorizing activity. The other parameters included factors such as the initial concentration of dye (30–300 mg/L), concentration of salt (1–6%), and yeast extract concentration (0.1–2.0 g/L). Furthermore, the dye incubated with MSM medium was studied for the effectiveness of bacterial consortium under static conditions.
Decolourization Assay
The experiments for studying the decolorization of Acid orange were carried out in a 250 ml Erlenmeyer flask holding 100 ml sterilized MSM broth. This was enhanced with yeast extract (0.1% w/v) and acid orange (30 mg/L). The reduction in dye was significant, in nearly 23 h almost 85% of the dye was completely decolorized. The yeast extract as a supplementary source showed a good effect on the decolourizing abilities of the bacterial consortium. Following this, 0.1% (w/v) yeast extract as co-substrate was added to the MSM media. The flask without the bacterial treatment was kept as the control for comparative studies. The flasks were then incubated at 30°C with both static and shaking (125 rpm) until the discoloration ends. The optical density for change in color was monitored at regular intervals. The 5 ml culture was used for the same. The supernatant was separated by centrifuging the culture at 9,000 rpm for 20 min. The UV-vis spectrophotometry was used to study the rate of absorbance at 560 nm.
Biodegradation of the Dye
To study the rate of degradation in the dye, the effluent sample was analyzed for the reduction in the level of physicochemical parameters like biological oxygen demand (BOD), chemical oxygen demand (COD), and total organic carbon (TOC). This is a clear indication of the degradation of the dye acid orange after bacterial treatment. The cell biomass was extracted by centrifuging both untreated and decolorized culture broth at 7,500 ×g for 15 min at 4 ± 0.20°C. the filtration of the cell biomass extract was carried out using 0.45 μm cellulose acetate filters (Sterlitech Corporation, United States). For estimating the BOD, a photometric method was employed. The COD was analyzed using the dichromate closed reflux titrimetric method (de Souza et al., 2010). The reduction in TOC was studied using a Sievers 5310C automated analyzer (GE Water & Process Technologies, United States).
Ultraviolet–Vis and Fourier Transform-Infrared Spectroscopic Analysis
The treated and untreated effluent samples were analyzed using the UV–Vis spectrophotometer. The wavelength range of the spectrophotometer was 300–700 nm (Pinheiro et al., 2004). This was followed by confirmatory FT-IR analysis carried in a range of 400–4,000 cm−1 using a spectrophotometer (Nicolet 6,700, Thermo Scientific, United States). This reveals the chemical nature of treated effluents. The pellets for FT-IR analysis was added with potassium bromide (KBr) before mixing it with the bacterial treated effluent samples.
Gas Chromatography-Mass Spectroscopy Analysis
Trimethylsilyl (TMS) was used to treat the extracted sample. The derivatized sample (2.0 µl aliquot) was injected into GC–MS (Trace GC Ultra Gas Chromatograph, Thermo Scientific, United States). This was equipped with a TriPlus autosampler coupled to TSQ Quantum XLS triple quadrupole mass spectrometer (Thermo Scientific, United States). For carrying out the separation process, the DB-5 MS capillary column (30-m length× 0.25 µm I.D. × 0.25 mm film thickness of 5% phenyl and 95% methylpolysiloxane) was used. The flow rate of helium was 1.1 ml min−1. The variation in the GC oven was between 65 and 230°C, and the time variation was at the rate of 6°C min−1. After nearly 20 min s the final temperature was recorded at 290°C (hold for 20 min) at a rate of 10°C min−1 high. The mass spectrum was operated using the positive electron ionization (+EI) mode at an electron energy of 70 eV with a solvent hold-up of 7 min it comprised of a full-scan mode from m/z, 45–800. The organic compounds were identified using MS library NIST v. 1.0.0.12.
Results & Discussion
Isolation and Identification of the Strain Degrading Acid Orange
The selection of isolates was done by observing the zone-forming abilities of the strains. The decolorization activity was also observed in the submerged culture conditions. After the preliminary examination, the potential isolates were sent for 16 S rRNA gene sequencing. The identification of strains is previously reported (Bera & Tank, 2020). Each strain was separately inoculated in nutrient broth and incubated at 32°C in static condition. The strains were mixed together to form a bacterial consortium. This was then used to study the bioremediation of Acid Orange dye. The assessment between the GenBank database of the National Center for Biotechnology Information (NCBI) and the experimentally isolated strains led to the identification of the strains.
According to the isolation source, the strains SPM-1, SPM-2, SPM-3, and SPM-4 belonged to P. stutzeri NCIM 5136 (100%), Bacillus tequilensis KCTC 13622 (99%), Bacillus flexus NBRC 15715 (100%) and Kocuria rosea DSM 20447 (99%), respectively. The phylogenetic tree construction using the neighbor-joining method was carried out for the strains and is depicted in Figure 1. All the four strains were submitted to the NCBI Gene Bank with respective accession No. MW219251, MW110471, MW132411, and MW131645.
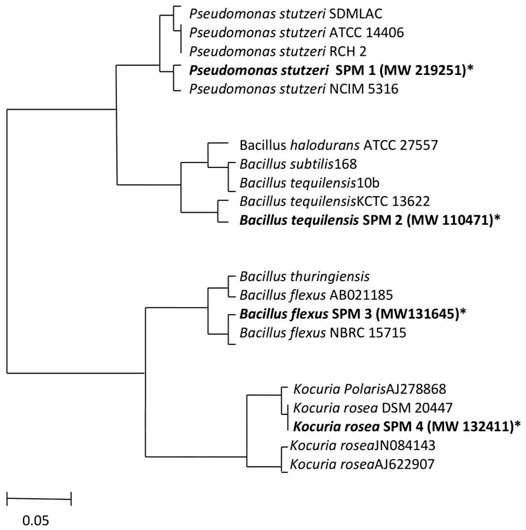
FIGURE 1. The evolutionary history was inferred using the Neighbor-Joining method. The evolutionary distances were computed by the Maximum Composite Likelihood method. The units of the number of bases substituted per site. The missing data and the positions that had gaps were removed from the database (Complete deletion option). For conducting the phylogenic analyses MEGA4 was used. A 5% divergence is presented in the scale bar.
Optimization of Physicochemical Parameters
The environmental parameters play a vital role in the bacterial detoxification process. The pH and temperature of the medium are important in the decolorization as well as degradation process. Mostly the optimum pH favorable for decolorization of azo dyes is reported to be in the range of 6.0–8.0. In the following study, the bacterial consortium was subjected to a varying range of pH and it was noticed that the optimal condition was at 7.5 pH and temperature 32 ± 0.2°C. The rate of decolorization was extremely low in the acidic range pH and equally ineffective in the highly basic range (Wang et al., 2014). The same goes for temperature also. Previously reported articles suggest that a temperature bit above the normal room temperature is usually optimum for the decolorization activity. Higher temperature tends to denature the bacterial enzyme resulting in the disruption of degradation activity. The bacterial consortium exhibited excellent decolourizing activity with nearly 86% decolorization activity within 23 h of incubation at 7.5 pH, 32 ± 0.2°C temperature, static condition, and in the presence of yeast extract. The consortium was also subjected to different dye concentrations and complete decolorization was observed at 30 mg/L concentration. There was nearly no decolorization activity at 300 mg/L as a higher concentration of dye tends to exhibit toxicity to the bacterial cells. The minor percentage decolorization and extended-time period to the high concentration of dye can be accredited to the occurrence of the four sulfonic acid groups on the orange acid that act as detergent acting inhibitory effect on the bacterial consortium (García-Martínez et al., 2015). The figure depicts all the physicochemical parameters studies in this article. Outlets from the textile industry generally contain sodium and potassium chloride salts, which are most commonly used for dyeing and discharging together with unused dyestuffs. For this reason, a simultaneous examination to understand the consequence of salt concentration (1–6% w/v) was performed to decolorize the AO with this novel bacterial consortium. The organism displayed a suitable decolorization up to a maximum concentration of 2% salt in MSM under optimum conditions after 23 h of incubation. It is previously been reported that the concentration of sodium higher than 3 g/L may result in the decline of bacterial metabolism. But, in our experimental study, the decolorization percentage was 72% and 46% at 3% and 4% salt concentration respectively. The minor activity was observed in the 6% salt concentration used in the medium. The loss of metabolic activity due to plasmolysis also adds up to accumulations of high-salt concentrations in the cell (Wu et al., 2012). Figure 2 shows the graphs for all the environmental factors that were considered during the research study.
Effect of Yeast Extract Concentration
The bacterial consortium was capable of reducing the AO (30 mg/L) effectively in the existence of yeast extract as co-substrate (Swain et al., 2021). Of all the other sources of nitrogen, only the yeast extract served as a better source of nitrogen for decolorizing orange acid in a shorter time. To know the effect of yeast extract on the bacterial consortium, different concentrations ranging from 0.1 to 2.0 g/L were evaluated. After the addition of 1 g/L of yeast extract in MSM, the total decolorization of AO was observed within 23 h. But a further increase in yeast extract concentration did not affect the decolorization activity. Thus, the usage of 1 g/L yeast was considered appropriate for the decolorization.
Decolorization Studies
The degraded products were analyzed to detect a possible discoloration mechanism of AO dye. UV-Vis absorbance (300–750 nm) of orange acids in MSM exhibited a single peak in the visible region at 486 nm (λmax). Figure 3 represents the UV-Vis spectroscopy analysis results. In the current investigation, the decolourizing potential of our bacterial consortium on textiles azo dye AO was examined. UV-Vis spectroscopic analysis was performed to confirm the staining of the dye. The figure below indicates the absorption spectrum of the AO previously and after its bacterial treatment within the visible range. It was found that the absorption maximum of the parental colorant (486 nm) was almost completely absent in the sample obtained after discoloration of the dye (Thung et al., 2018). During the experimentations, it was noted that the AO dye was adsorbed only at the beginning, and no adsorption was visibly observed after discoloration of the dye (Kumar Sonwani et al., 2021).
Dye Degradation Studies
To study the degradation of dyes the bacterial treated effluent sample was analyzed for the reduction in the rate of BOD, COD, and TOC levels (García-Martínez et al., 2015). It was observed that a drastic reduction in all three parameters provides clear evidence for dye degradation. About 96% reduction in BOD5 level was observed in the treated sample. The COD level was decreased to 79% and TOC was at 54%. This was observed under the optimal environmental condition at 23 h of incubation. The rate of increase of cell number during the decolorization process was noted from 2.2 to 5.37 × 108 cfu/ml.
Fourier Transform-Infrared
FT-IR analysis revealed the metabolites produced during the degradation process of AO dye. Specific peaks were noted in the control dye sample due to the presence of azo bonds. The Figure 4A,B exhibits a typical FTIR spectrum of the control AO dye which have peaks at 2,369.9 and 1,522.4 cm−1 which is an indicative of stretching of alkanes and amines. The peak at 1,567.8 cm−1 attributed to the N-H stretching of N-phenylaniline while the appearance of the peak at 1,457.8 cm−1 is attributed to the S=O stretching of the sulfonic compounds. A sharp peak at 3,328.5 cm−1 in the bacterial treated effluent sample showed the azo groups–N=N–stretching. The samples which were not treated with the effluent sample showed several peaks at 507.5, 712.8, and 986.5 cm−1 represents the stretching of sulfate and nitroso groups. A visible peak was also observed at 1,141.3 and 684.8 cm−1, this can be because of absorption caused by the bending of aliphatic C–H and asymmetric stretching of COO− in the spectral. The absence of organic compounds as pollutants in the effluent sample treated with bacterial consortium gives clear validation of the breaking down of the dye molecules into consequent metabolites.
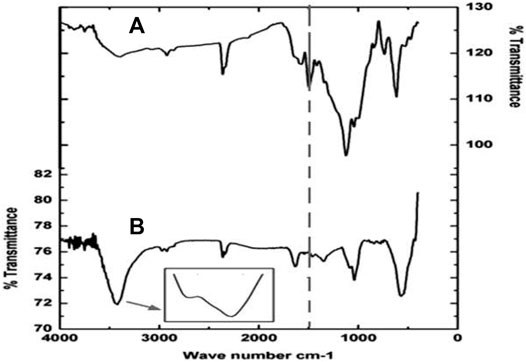
FIGURE 4. (A) FTIR Analysis of Control (Effluent containing the dye) and (B) FTIR Analysis of Bacterial treated effluent (23 h).
Gas Chromatography-Mass Spectroscopy Interpretation
The GC-MS analysis proposed the preliminary reductive cleavage of azo bond results in the formation of 3 intermediate compounds; i) 4- aminobenzene-1-sulfonic acid (MW 173.19, m/z- 298.94), ii) 1- aminonaphthalen-2-ol (MW- 159.188, m/z- 314.00) and iii) 2- aminonapthalen-1-ol (MW- 158.8, m/z- 282.95). The presence of several peaks indicated the presence of contaminants in the control sample. These were removed in the bacterial consortium treated effluent sample containing AO. The intermediates were further reduced to another three metabolites i) 4-methyl-sulfanyl-naphthalene 1,2-dione (MW- 86.13, m/z- 146.90), ii) 2-aminonapthalene 1,4- dione (MW-95.52, m/z- 132.85), and iii) 2- methoxynaphthalene (MW- 28.17, m/z- 72.94). Following the final metabolites formed during the GC-MS analysis, we concluded the dye is getting mineralized. Figure 5 represents the GC- MS results.
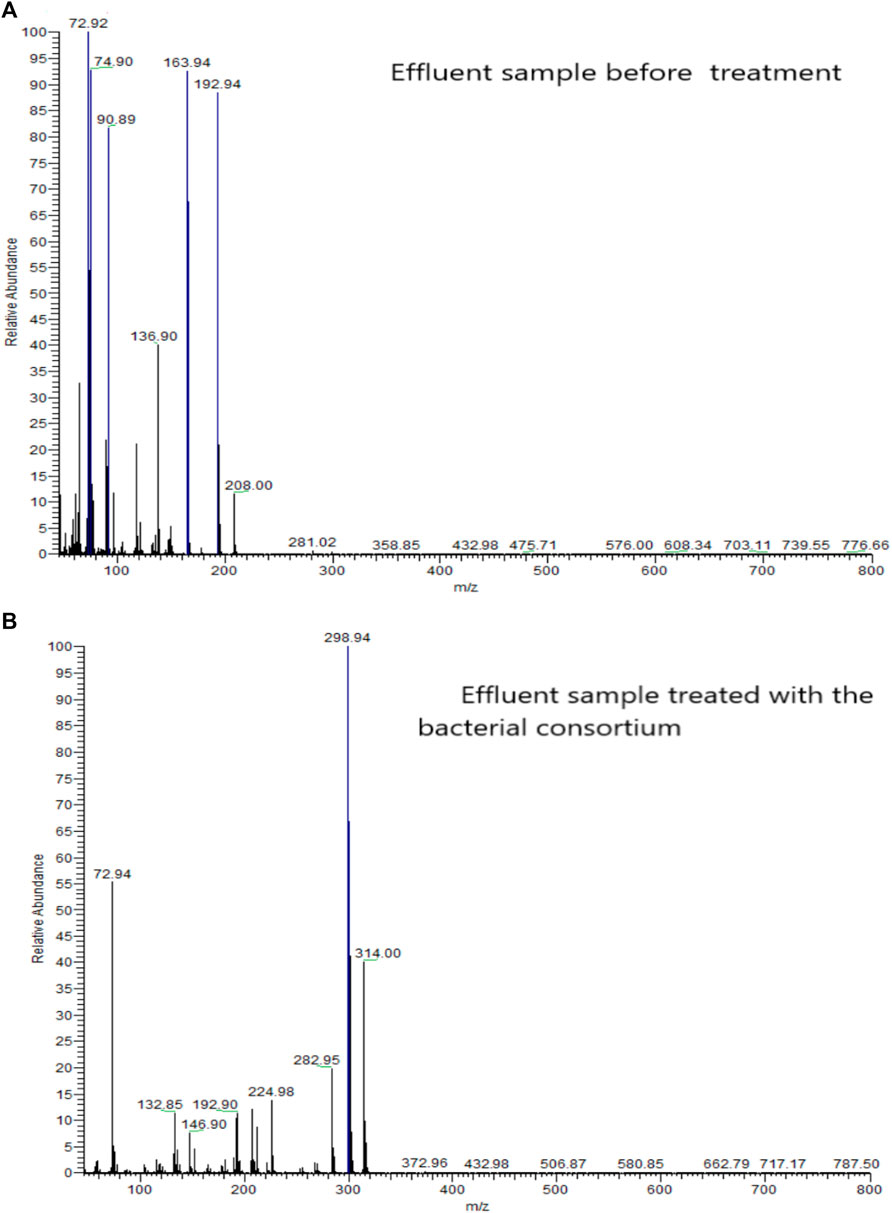
FIGURE 5. (A) GC-MS analysis of effluent before treatment and (B) GC-MS analysis of effluent after bacterial treatment.
Conclusion
This study has shown that the bacterial consortium is capable of degradation of Acid Orange as the only carbon source with minimal nutrition at static conditions. When provided with optimal environmental conditions along with yeast extract as supplementary substrate, the bacterial consortium SPB92 exhibited immense decolourizing as well as degradation ability. The shaking conditions displayed very minimal decolorization activity. This indicates the static state was essential, unlike most microbial species known for causing azo reduction. The principal reason behind the metabolic activities for azo dye degradation is the breakage of the azo bond by the enzyme azo reductase under aerobic conditions. However, there are reports of certain oxygen-sensitive aerobic azo reductases producing microorganisms (Devaraja, 2011). It discolors reactive, azo, diazo a triphenylmethane dye. These studies indicate that discoloration of the dye is mediated by live culture (Garrido-Baserba et al., 2015). The FT-IR results exhibited a comprehensive loss of the aromatic structure of the AO dye by the bacterial consortium. In addition, the bacterial consortium could decolorize five other structurally distinct azo dyes which are structurally similar to the AO dye. This indicates its usability in the arena of textile wastewater treatment. So, the bacterial consortium reported in this research article is a highly promising bacterial consortium. It shows noteworthy potential for wastewater treatment involving dye reduction.
Data Availability Statement
The original contributions presented in the study are included in the article/supplementary material, further inquiries can be directed to the corresponding author.
Author Contributions
All authors listed have made a substantial, direct, and intellectual contribution to the work and approved it for publication.
Conflict of Interest
Author MS is employed by Division of Applied and Environmental Microbiology, Industrial Wastewater Research Lab, Enviro Technology Limited.
The remaining authors declare that the research was conducted in the absence of any commercial or financial relationships that could be construed as a potential conflict of interest.
Publisher’s Note
All claims expressed in this article are solely those of the authors and do not necessarily represent those of their affiliated organizations, or those of the publisher, the editors and the reviewers. Any product that may be evaluated in this article, or claim that may be made by its manufacturer, is not guaranteed or endorsed by the publisher.
Acknowledgments
The author is thankful to P P Savani University for proving us with necessary requirements during the research work.
References
Ben Mansour, H., Corroler, D., Barillier, D., Ghedira, K., Chekir, L., and Mosrati, R. (2007). Evaluation of Genotoxicity and Pro-oxidant Effect of the Azo Dyes: Acids Yellow 17, Violet 7 and Orange 52, and of Their Degradation Products by Pseudomonas Putida Mt-2. Food Chem. Toxicol. 45, 1670–1677. doi:10.1016/j.fct.2007.02.033
Bera, S. P., and Tank, S. K. (2021a). Bioremedial Approach of Pseudomonas Stutzeri SPM-1 for Textile Azo Dye Degradation. Archives Microbiol. 203 (5), 2669–2680. doi:10.1007/s00203-021-02258-x
Bera, S. P., and Tank, S. K. (2021b). Microbial Degradation of Procion Red by Pseudomonas Stutzeri. Sci. Rep. 11. doi:10.1038/s41598-021-82494-9
Bera, S. P., and Tank, S. K. (2020). Screening and Identification of Newly Isolated Pseudomonas Sp. For Biodegrading the Textile Azo Dye C I Procion Red H-3B. J. Appl. Microbiol. 130, 1949–1959. doi:10.1111/jam.14920
Chen, H. (2006). Recent Advances in Azo Dye Degrading Enzyme Research. Cpps 7, 101–111. doi:10.2174/138920306776359786
de Souza, S. M. d. A. G. U., Bonilla, K. A. S., and de Souza, A. A. U. (2010). Removal of COD and Color from Hydrolyzed Textile Azo Dye by Combined Ozonation and Biological Treatment. J. Hazard. Mater. 179, 35–42. doi:10.1016/j.jhazmat.2010.02.053
Devaraja, T. S. (2011). Indian Textile and Garment Industry-An Overview. New Delhi: Indian Council of Social Science Research.
García-Martínez, Y., Bengoa, C., Stüber, F., Fortuny, A., Font, J., and Fabregat, A. (2015). Biodegradation of Acid Orange 7 in an Anaerobic-Aerobic Sequential Treatment System. Chem. Eng. Process. - Process Intensif. 94, 99–104. doi:10.1016/j.cep.2014.12.011
Garrido-Baserba, M., Molinos-Senante, M., Abelleira-Pereira, J. M., Fdez-Güelfo, L. A., Poch, M., and Hernández-Sancho, F. (2015). Selecting Sewage Sludge Treatment Alternatives in Modern Wastewater Treatment Plants Using Environmental Decision Support Systems. J. Clean. Prod. 107, 410–419. doi:10.1016/j.jclepro.2014.11.021
Gitis, V., and Hankins, N. (2018). Water Treatment Chemicals: Trends and Challenges. J. Water Process Eng. 25, 34–38. doi:10.1016/j.jwpe.2018.06.003
Jadhav, K., Kushwaha, B., Jadhav, I., Shankar, P., Geethadevi, A., Kumar, G., et al. (2021). Genomic Analysis of a Novel Species Halomonas Shambharensis Isolated from Hypersaline Lake in Northwest India. Mol. Biol. Rep. 48 (2), 1045–1053. doi:10.1007/s11033-020-06131-w
Karunya, A., Rose, C., and Valli Nachiyar, C. (2014). Biodegradation of the Textile Dye Mordant Black 17 (Calcon) by Moraxella Osloensis Isolated from Textile Effluent-Contaminated Site. World J. Microbiol. Biotechnol. 30 (3), 915–924. doi:10.1007/s11274-013-1509-8
Khan, R., Bhawana, P., and Fulekar, M. H. (2013). Microbial Decolorization and Degradation of Synthetic Dyes: A Review. Rev. Environ. Sci. Biotechnol. 12 (1), 75–97. doi:10.1007/s11157-012-9287-6
Kumar Sonwani, R., Pandey, S., Kumar Yadav, S., Shekhar Giri, B., Katiyar, V., Sharan Singh, R., et al. (2021). Construction of Integrated System for the Treatment of Acid Orange 7 Dye from Wastewater: Optimization and Growth Kinetic Study. Bioresour. Technol. 337, 125478. doi:10.1016/j.biortech.2021.125478
Kushwaha, B., Jadhav, I., Verma, H. N., Geethadevi, A., Parashar, D., and Jadhav, K. (2019). Betaine Accumulation Suppresses the De-novo Synthesis of Ectoine at a Low Osmotic Concentration in Halomonas Sp SBS 10, a Bacterium with Broad Salinity Tolerance. Mol. Biol. Rep. 46 (5), 4779–4786. doi:10.1007/s11033-019-04924-2
Nawaz, M. S., and Ahsan, M. (2014). Comparison of Physico-Chemical, Advanced Oxidation and Biological Techniques for the Textile Wastewater Treatment. Alexandria Eng. J. 53, 717–722. doi:10.1016/j.aej.2014.06.007
Parisi, M. L., Fatarella, E., Spinelli, D., Pogni, R., and Basosi, R. (2015). Environmental Impact Assessment of an Eco-Efficient Production for Coloured Textiles. J. Clean. Prod. 108, 514–524. doi:10.1016/j.jclepro.2015.06.032
Patel, R., Tajddin, K., Patel, A., and Patel, B. (2015). Physico-Chemical Analysis of Textile Effluent. II(V), 33–37.
Pinheiro, H. M., Touraud, E., and Thomas, O. (2004). Aromatic Amines from Azo Dye Reduction: Status Review with Emphasis on Direct UV Spectrophotometric Detection in Textile Industry Wastewaters. Dyes Pigments 61, 121–139. doi:10.1016/j.dyepig.2003.10.009
Saratale, R. G., Saratale, G. D., Chang, J. S., and Govindwar, S. P. (2011). Bacterial Decolorization and Degradation of Azo Dyes: A Review. J. Taiwan Inst. Chem. Eng. 42 (1), 138–157. doi:10.1016/j.jtice.2010.06.006
Shah, M. P. (2014). Microbial Degradation and Decolorization of Acid Orange Dye by Anaerobic/Aerobic Sequential Process. Int. J. Environ. Bioremediation Biodegrad. 2 (3), 112–116. doi:10.12691/ijebb-2-3-310.12691/ajmr-2-5-5
Singh, R., Singh, R., and Singh, P. (2014). Bacterial Decolorization of Textile Azo Dye Acid Orange by staphylococcus Hominis RMLRT03. Toxicol. Int. 21 (2), 160. doi:10.4103/0971-6580.139797
Sriram, N., and Reetha, D. (2015). Isolation and Characterization of Dye Degrading Bacteria from Textile Dye Effluents. Central Eur. J. Exp. Biol.
Swain, G., Singh, S., Sonwani, R. K., Singh, R. S., Jaiswal, R. P., and Rai, B. N. (2021). Removal of Acid Orange 7 Dye in a Packed Bed Bioreactor: Process Optimization Using Response Surface Methodology and Kinetic Study. Bioresour. Technol. Rep. 13, 100620. doi:10.1016/j.biteb.2020.100620
Thung, W.-E., Ong, S.-A., Ho, L.-N., Wong, Y.-S., Ridwan, F., Lehl, H. K., et al. (2018). Biodegradation of Acid Orange 7 in a Combined Anaerobic-Aerobic Up-Flow Membrane-Less Microbial Fuel Cell: Mechanism of Biodegradation and Electron Transfer. Chem. Eng. J. 336, 397–405. doi:10.1016/j.cej.2017.12.028
Wang, X., Wang, L., Li, J., Qiu, J., Cai, C., and Zhang, H. (2014). Degradation of Acid Orange 7 by Persulfate Activated with Zero Valent Iron in the Presence of Ultrasonic Irradiation. Sep. Purif. Technol. 122, 41–46. doi:10.1016/j.seppur.2013.10.037
Keywords: dye degradation, wastewater treament, bioremediation, pollutants, bioaugmentaion
Citation: Bera SP, Shah MP and Godhaniya M (2022) Microbial Remediation of Textile Dye Acid Orange by a Novel Bacterial Consortium SPB92. Front. Environ. Sci. 10:930616. doi: 10.3389/fenvs.2022.930616
Received: 28 April 2022; Accepted: 26 May 2022;
Published: 24 June 2022.
Edited by:
Juliana Heloisa Pinê Américo-Pinheiro, Brazil University, BrazilReviewed by:
Deepak Parashar, Medical College of Wisconsin, United StatesHarishkumar Madhyastha, University of Miyazaki, Japan
Khac-Uan Do, Hanoi University of Science and Technology, Vietnam
Nazia Hossain, RMIT University, Australia
Copyright © 2022 Bera, Shah and Godhaniya. This is an open-access article distributed under the terms of the Creative Commons Attribution License (CC BY). The use, distribution or reproduction in other forums is permitted, provided the original author(s) and the copyright owner(s) are credited and that the original publication in this journal is cited, in accordance with accepted academic practice. No use, distribution or reproduction is permitted which does not comply with these terms.
*Correspondence: Sweta Parimita Bera, c3dldGFwYXJpbWl0YWJlcmFAZ21haWwuY29t