- 1College of Plant Science, Jilin University, Changchun, China
- 2Baicheng Academy of Agricultural Sciences, Baicheng, China
- 3Soil and Fertilizer Station of Jilin Province, Changchun, China
- 4Center for Resources, Environment and Food Security, China Agricultural University, Beijing, China
The limited available information on variations in yield gaps (differences between actual yields and the theoretically attainable yields) restricts the development of rational strategies to optimize yields and reduce environmental costs. Quantifying the yield potential and the variations in yield gaps will help identify factors that limit yields and will enable a narrowing of the current yield gap. Here, we applied an analytical framework to yield data to identify options for closing the yield gap at the county level. We used a database containing yields for 40 counties and data from 87 representative on-farm experiments in Jilin Province, China, from 2006 to 2008. The yield potential was simulated for each region-year using a Hybrid-Maize model (http://www.hybridmaize.unl.edu/) and weather data. We then conducted a systematic and spatial analysis of actual yields to identify yield gaps at the county level. The simulated average potential yield at 27 representative sites was 15.2 Mg ha−1 (range 8.1–17.6 Mg ha−1) in Jilin Province. The on-farm experiments suggested an attainable potential yield ranging from 8.7 to 16.7 Mg ha−1 across Jilin Province. During this period, the actual maize yield varied between 4.1 and 11.9 Mg ha−1, according to the county-level data. Farmers’ fields, therefore, achieved 52% of the model yield potential and 77% of the attainable potential yield. Widely different amounts of P fertilizer input among farmers contributed significantly to regional variations in YGE. Soil Olsen-P and rainfall were also major factors. The results indicate that there is great potential to substantially increase the maize yield in non-optimal P management regions, such as in the western Jilin Province. Hence, improvements in regional P management strategies, such as at the county level, need to be assessed separately to provide a basis for increasing the actual maize yield.
1 Introduction
Global agriculture is facing a great challenge to ensure food security with an urgent need to increase crop production by around 60% before 2050 (David Tilman et al., 2011). In 2014, China produced 20.7% of the global maize on 19.6% of global the agricultural land and consumed 21.5% of the global maize production (FAO, 2015). As a consequence of expected economic growth and changing diet, the demand for maize in China is anticipated to increase by 47% by 2030 compared to the present time (Cui et al., 2014). However, the rates of gain in maize yields have slowed markedly in the past 10–20 years, even though agricultural inputs such as phosphorus (P) have continued to increase (Chen et al., 2014). As a result, the net P surplus (P input minus output) was 23.6 kg ha−1 year−1 in the current maize system in China (Zhang et al., 2015). P surplus on land with already adequate P availability accelerates the rate of phosphate reserve depletion and increases the risk of water problems (Townsend and Porder, 2012). The consequences of these problems are meaningful on a global scale.
Northeast China is one of the major agricultural production areas in China. Maize production in this area accounts for approximately 53% of total production in China and for approximately 52% of the land devoted to this crop (2001–2015). A recent study reported the modeled potential yield of maize in this region varies from 8.3 t ha−1 (Lv et al., 2015) to 17.7 t ha−1 (Meng et al., 2013). Yield gaps for maize production (defined as the difference between yield potential and the yield actually achieved) are large and vary among different regions (Meng et al., 2013; Tao et al., 2015). In practice, most farmers and even extension staff still believe that high P fertilization rates and high grain yields are always directly related (Li et al., 2015). However, our previous studies showed that phosphorus fertilizer use efficiency (PUE) conflicts with increased yield under the current maize system in Northeast China (Zhang et al., 2015). This variation in yield gap and PUE underlines the importance of accurate estimates across meaningful land scales (Meng et al., 2013). Understanding the variation of yield gaps and their responses to P fertilization is necessary to devise improved broad-scale P management strategies and minimizing negative environmental impacts.
A previous study reported that potential yield and actual yield did not have good relevancy by region (Ciampitti and Vyn, 2014). Observations on maize yields in different regions indicate that different factors contribute to the sizes of their yield gaps and their responses to P management (Lv et al., 2015; Tao et al., 2015). However, there is still a considerable knowledge gap that makes it difficult for decision makers to develop appropriate P management strategies to increase maize production and P use efficiency.
In China, the county is the lowest administrative tier for executive policy implementation; most agricultural policies and recommended practices are conducted at a county scale. Therefore, understanding the variation of yield gaps and assessing the related impacts of P management strategies at the county level will aid policymakers to optimize future management strategies. The objectives of this study were (i) to estimate the model-based yield gap (YGM, model yield potential–actual farm yield) and the attainable yield gap (YGA, attainable maximum yield–actual farm yield) at the county level in Jilin Province; and (ii) to quantify yield gaps and their responses to P fertilization.
2 Materials and methods
2.1 Study site
The study region in Jilin Province (41°−46°N, 121°−130°E) was divided into three areas based on their ecological function: eastern humid mountainous area (EHMA), central semi-humid plain area (CSPA), and western semi-arid plain area (WSPA) (2013) (Figure 1). The most important crop in this region is spring maize, which is sown at the end of April and matures in early October. This region of Jilin Province has a warm-temperate and sub-humid climate, with cold winters and hot summers (Cui et al., 2013). Soil types include ablic luvisol, calcaric arenosol, calcaric fluvisol, haplic chernozem, haplic kastanozem, haplic luvisol, and luvic phaeozem (Gong et al., 1999; FAO, 2015). Long-term (1960–2012) average temperature in the study region during the growing season was 16.2–20.9°C, increasing from east to west (Figure 2A); average annual precipitation was 258.3–756.3 mm, increasing from northwest to southeast (Figure 2B). More than 70% of the annual precipitation (600–800 mm in EHMA, 450–500 mm in CSPA, and 250–350 mm in WSPA) falls during the May–September growing season of the rain-fed maize. Data were collected from farm land, and no specific permissions were required for these locations. The field studies did not involve endangered or protected species.
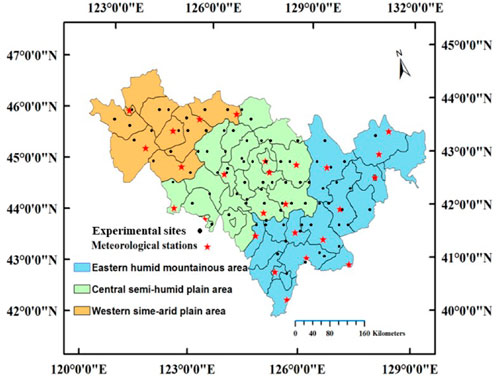
FIGURE 1. Locations of the eastern humid mountainous area (EHMA), central semi-humid plain area (CSPA), western semi-arid plain area (WSPA), and 27 meteorological stations in Jilin province.
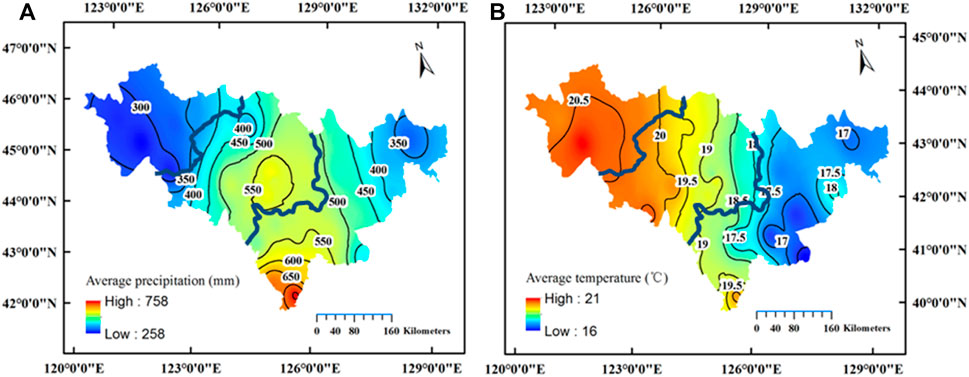
FIGURE 2. Spatial distribution of average precipitation (A) and temperature (B) during the growing season (May to September) from 1960 to 2012. Black lines are the boundaries of counties, and dark-blue lines are the boundaries of the eastern humid mountainous area (EHMA), central semi-humid plain area (CSPA), and western semi-arid plain area (WSPA).
2.2 Database description
The county-level modeled potential yield was simulated by Hybrid-Maize model, which has been validated and widely used in China, and has been shown to be reasonably accurate at estimating maize potential yield (Chen et al., 2011; Meng et al., 2013). In this study, water-limited yield potential (Yw) was estimated as 72% as the farmland grows maize under rain-fed conditions (2007–2009). The daily weather data, including maximum and minimum temperatures, relative humidity, precipitation, and wind speed used for the model were based on the 1960–2012 averages and were obtained from 27 meteorological stations in Jilin Province (Figure 1), operated by the National Meteorological Networks of China Meteorological Administration. The sowing and harvest dates, plant populations, and hybrid maturity dates for simulations at each site were similar to those for the actual crops grown by the local farmers. The spatial distribution of Yw was estimated by interpolation based on the simulated results from all 27 weather stations and a map using the ordinary Kriging method in ArcGIS (http://www.esri.com/software/arcgis). We used the Lambert-projected coordinate system with a spatial resolution of 200 m per pixel, and the latitude and longitude of each sampling point were provided by Google Earth (http://www.google.com/earth/).
The attainable potential yield (YA) was obtained from a total of 139 on-farm experiments, which were conducted from 2006 to 2008 on 40 farms in 40 counties of Jilin Province (41°−46°N, 121°−130°E); these experimental farms covered the humid and sub-humid areas, and included seven soil types (Figure 1). The experiments were conducted in farmers’ fields based on the recommendations of local agronomists as to the selection of cultivars, plant density, and fertilizer rates.
Our experimental design consisted of a single replication of three P treatments, no P as a control (P0), the recommended P rate (RPR), and 150% of the recommended P rate (150% RPR). The RPR was determined by an agronomist’s recommendation based on soil test results and a target yield (1.1 times the average yield of the past 5 years) (Zhang et al., 2010), which received 60–130 kg P2O5 ha−1 as triple superphosphate before planting. All plots received 75–220 kg N ha−1 (one-third was applied before sowing, and the remaining two-thirds, during sowing) and 60–120 kg K2O ha−1 before planting, based on soil N and K test results. The highest yield recorded from each experiment represents the YA for the farmer at the selected location.
A questionnaire survey on crop yield and nutrient inputs was conducted from 2006 to 2009 by the Soil and Fertilizer Station of Jilin Province (Li et al., 2011). We used data of maize yield and P input from 261 farmers located in the same villages as the experimental sites. Average farmers’ yields (YF) at the county level were calculated as average yields for 2006–2008 to increase accuracy and were mapped to the county level.
2.3 Yield gap calculation
YW and YA at the county level were obtained by converting grid map data into county map data using the command tools of “zonal statistics as table” according to the maize sowing area for 2006–2008. In combination with the county map, we used the YW and YA at the county level based on weighted averages according to the maize sowing area in 2006–2008. We estimated two different yield gaps using different measures of yield potential or attainable yields: (i) model-based yield gap (YGM), which was estimated as the difference between Yw and YF in each county; and (ii) the experiment-based yield gap (YGE), which was estimated as the difference between YA and YF in each county.
A mixed-model ANOVA was used to assess the variability of modeled yield potential, experimental yield potential, the average yields YGM and YGE among counties, [degrees of freedom (df) = 39], P input (df = 260), soil types (df = 6), and rainfall levels (df = 26) for all the experimental site-years.
3 Results
Modeled yield potential (Yw) was estimated by interpolation (Figure 3A) and by “zonal statistics as table” (Figure 3B). Average potential yield at the county level showed no significant differences among counties using these two methods of estimation. This means that it is reasonable to convert yield potential from a grid map into a county map. Yw varied across Jilin Province from 8.1 to 17.6 t ha−1, with a weighted average of 15.2 t ha−1 for the entire province based on the average maize sowing area during 2006–2008. Average potential yield increased with decreasing longitude for most of the Jilin Province except for the arid western part. In the EHMA, potential yield varied from 11.4 to 15.7 t ha−1, with a weak trend to decrease with increasing longitude. In the CSPA, potential yields ranged from 12.9 to 16.3 t ha−1, with a trend to increase with decreasing longitude.
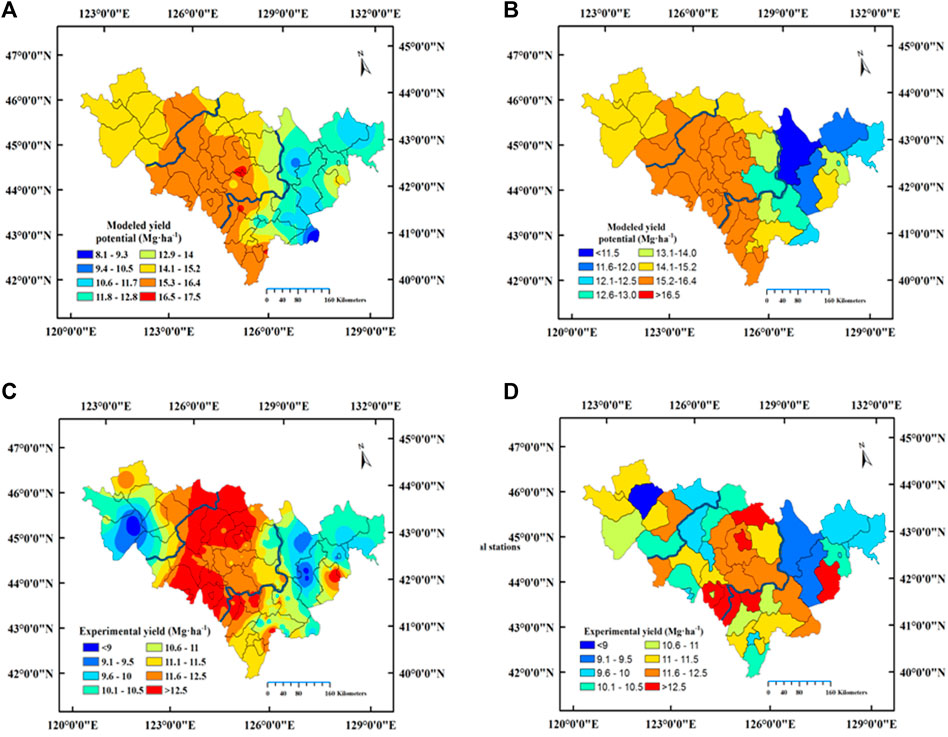
FIGURE 3. Spatial distribution of the modeled yield potential and attainable yield potential for Jilin province. (A) Modeled yield potential using the ordinary Kriging method in ArcGIS based on the simulated results of all 27 weather stations; (B) modeled yield potential at the county level using the tool command of “zonal statistics as table” according to the maize sowing area for 2006–2008. (C) Attainable yield potential was estimated by interpolation and a map using ArcGIS based on the simulated results of all 87 sites; (D) attainable yield potential at the county level using the tool command of “zonal statistics as table” according to the maize sowing area for 2006–2008. Black lines are the boundaries of counties, and dark-blue lines are the boundaries of the eastern humid mountainous area (EHMA), central semi-humid plain area (CSPA), and western semi-arid plain area (WSPA).
Attainable rain-fed maize yield in Jilin Province, which is the average experimental yield potential, was 11.6 Mg ha−1 (range, 8.7–16.7 Mg ha−1) across all 87 experimental sites (Figures 3C,D). In the WSPA, the experimental yield ranged from 8.5 to 12.0 Mg ha−1. The lowest experimental yield was found in Tongyu Prefecture that suffers from the effects of soil salinization. The EHMA showed a large variation in experimental yields with a range from 8.7 to 15.6 t ha−1. The areas included Dunhua Prefecture and a narrow zone in the northeastern part that contained both plains and hilly areas where maize yield are low (less than 9.0 t ha−1). In the CSPA, traditionally, the main maize production area due to low temperature stress and high soil quality, the experimental yield ranged from 10.0 to 16.7 t ha−1.
The weighted average value of farmers’ yields in Jilin Province during 2006–2008 was 8.7 Mg ha−1 based on the harvest area (range, 4.1–11.9 Mg ha−1; Figure 4A). In 80% of the maize area, yields were above 7.5 Mg ha−1; in 43% of the area, mainly in the central part of Jilin Province, the yield exceeded 9.0 Mg ha−1. In 8% of the maize area, yields were between 4.1 and 6.5 Mg ha−1, and this area comprised mainly the eastern mountainous and semi-mountainous areas. Generally, the productivity of spring maize was much higher in the center than in the eastern and western parts of Jilin Province (Figure 4A). The yield gap between the average farmers’ yield and the modeled yield potential (YGM) at the county level was between 4.9 and 11.0 Mg ha−1 (Figure 4B). YGE totaled 3.6 Mg ha−1 (range, 2.3–10.8 Mg ha−1; Figure 4C).
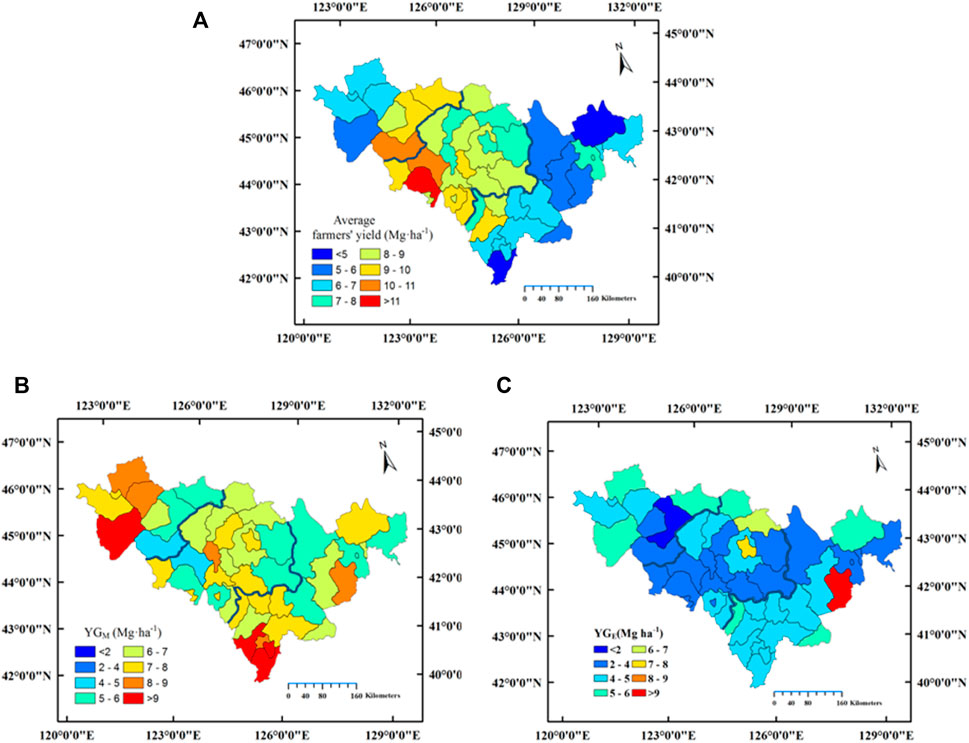
FIGURE 4. Spatial distribution of actual farms’ yield (A), YGM (B), and YGE (C) for Jilin province. YGM was estimated as the difference between the modeled yield potential and average farmers’ yield in each county; and YGE was estimated as the difference between the attainable yield potential and average farmers’ yield in each county. Black lines are the boundaries of counties, and dark-blue lines are the boundaries of eastern humid mountainous area (EHMA), central semi-humid plain area (CSPA), and western semi-arid plain area (WSPA).
In our study area, averaged soil Olsen-P was 23.7 mg kg−1 and ranged from 8.3 to 48.9 mg kg−1. Mean soil Olsen-P values was 13.9 mg kg−1 for low soil P fertility (Olsen-P <20 mg kg−1) counties (n = 16), 23.4 mg kg−1 for medium soil P fertility (20 mg kg−1<Olsen-P <30 mg kg−1) counties (n = 18), and 31.9 mg kg−1for high soil P fertility (Olsen-P>30 mg kg−1) counties (n = 16). The areas of high soil P fertility and low soil P fertility were mainly located in EHMA and WSPA, respectively (Zhang et al., 2015). Under these conditions, YGE averaged 5.0, 4.1, and 4.2 Mg ha−1 (ranged from 4.5 to 10.7 Mg ha−1, 3.1–6.6 Mg ha−1 and 3.3–5.6 Mg ha−1) for the counties with low, medium, and high soil P fertility, respectively (Figure 5A). The maximum yield classification distributions of P0 and maximum YGE were both located in Jiutai and Helong, as was the minimum yield distribution, indicating a close relationship between the attainable yield gap and P0 yield. Based on established regression models, the yield gap increased with an increase in yield of the P0 treatment (YGE = 0.79 YP0 + 4.6; R2 = 0.80). Across all 261 farmers’ site-years, YGE with suitable P input (RPR±20%) averaged 3.8 Mg ha−1 and varied from 2.3 to 4.7 Mg ha−1 (Figure 5B). Comparing suitable P input to overuse of P (>120% RPR), adding more P did not close yield gaps, but reduced PFPP. A relative decrease in P (<80% RPR) led to a 16% yield gap expansion, confirming that the RPR±20% was within a reasonable range for farmers’ practice. Non-optimal P management significantly increases yield gap in the field with low soil P fertility.
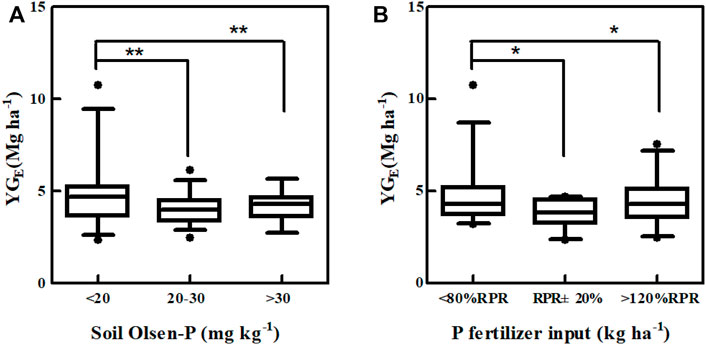
FIGURE 5. YGE with different soil Olsen-P ranges (A) and P fertilizer input (B) among farmer’s field (n = 261) in Jilin province.
The YGE averaged 4.4 Mg ha−1 and ranged from 2.3 Mg ha−1 to 10.7 Mg ha−1, varying significantly between counties (p < 0.01; Table 1). Little change occurred in mean YGE among soil types, which ranged from 3.8 Mg ha−1 to 5.6 Mg ha−1. In contrast, the mean YGE among different P input ranged from 2.3 Mg ha−1 to 10.7 Mg ha−1 (Table 1, p < 0.01), indicating that P input significantly affected variation in YGE. Precipitation and soil Olsen-P among different areas also led to significant variation in YGE (p < 0.05). The YGE increased with an increase in seasonal total rainfall, but decreased with an increase in soil Olsen-P. These data indicate that farmers’ P fertilizer practices and environmental conditions contribute significantly to regional variation in yield gap.
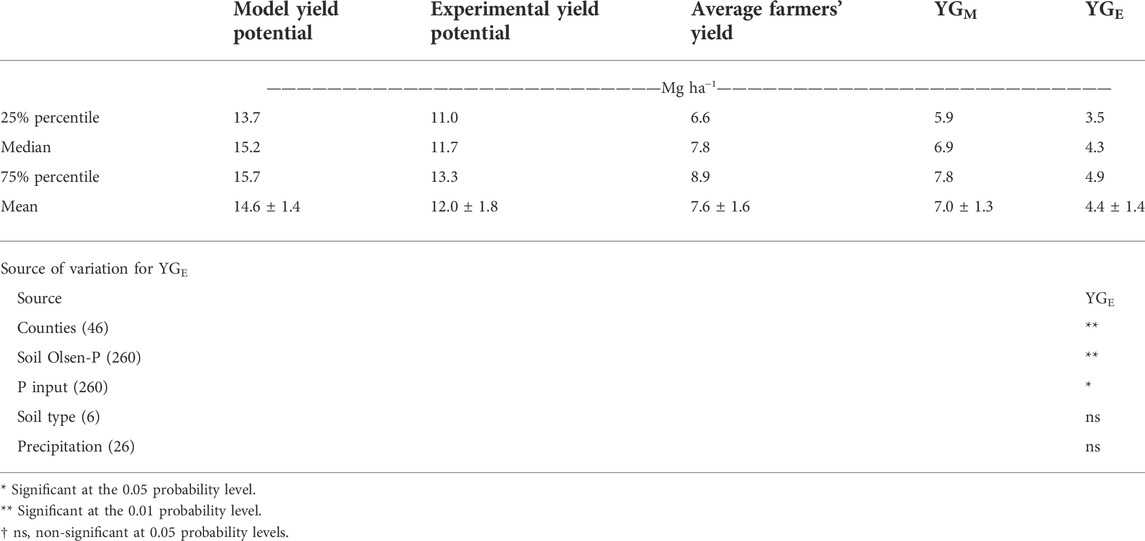
TABLE 1. Variability of the modeled yield potential, experimental yield potential, the average farmers’ yields, YGM, and YGE among counties and factors contributing to variation for YGE.
4 Discussion
Sustainably increasing global food output to feed the world’s growing population is a crucial challenge, and closing the yield gap is one of the strategies to achieve this goal (Zhang et al., 2015).
On-farm experiments provide data that demonstrate the variations in yield gap among different regions. In this study, the average model yield potential was 15.2 t ha−1 in Jilin Province, and this varied between different areas from 8.1 to 17.6 t ha−1. This yield potential is similar to those reported previously for this province (Lv et al., 2015; Liu et al., 2016). For Jilin Province as a whole, farmers achieved approximately 57% of the predicted yield potential; this rate is similar to that achieved in global maize production (Licker et al., 2010) but is considerably lower than that in the western United States where farmers achieve more than 80% of the yield potential (Grassini et al., 2011). The large YGM in Jilin Province indicates a great potential to substantially increase yield.
Evidence suggests that the large YGM for maize production in Northeast China is related to climatic factors (Liu et al., 2012; Tao et al., 2015). In this study, a positive correlation was found between the long-term average annual temperature and YGM. One of the ways to close the existing YGM is redesigning the crop system in different regions to improve light and heat resource use efficiency, for example, by increasing maize plant density in the central regions, adjusting the sowing dates, using long-duration varieties in the western regions, and using cold-resistant varieties in the eastern regions. A second possible approach will be to persuade farmers to adopt water-saving techniques such as residue mulching and no-tillage systems in Jilin Province, particularly in the arid western areas.
We found that farmers’ fields achieved 77% of the associated experimental yield potential, and YGE averaged 3.6 Mg ha−1. This yield gap is similar to those reported for other major maize-producing areas in Northeast China (Meng et al., 2013). Evidence shows that inefficient crop management practices constitute a principal factor for a large YGE in maize production (Chen et al., 2011; Li et al., 2015; Zhang et al., 2015). In the present study, farmers’ P fertilizer practices and environmental conditions contributed significantly to regional variations in the gap between actual farmers’ yields and attainable potential yields. Non-optimal P management by farmers is a major factor in YGE in the counties with low soil P fertility. For example, in Yongji Prefecture, a high YGE (exceeding 9.0 Mg ha−1) was mainly due to the low rate of P application (less than 60 kg ha−1). Yongji Prefecture is traditionally a rice-growing area and much less attention has been paid to the P fertilizer requirements for maize production. There is great potential to substantially increase maize yield by improvement of non-optimal P management in areas with low soil P fertility, such as in western Jilin Province.
The use of variable levels of P application among farmers is an important factor in the wide variation in YGE. In the short term, closing YGE seems to be a more effective approach to increase grain yield due to the similarities between experimental and farmers’ field conditions. In this study, the YGE increased with an increase in yield of the P0 treatment. However, in those areas with P0 yield over 8.9 Mg ha−1, the YGE increased, but the increased yield due to phosphorus fertilization decreased (data not shown). A potential explanation is that excessive P is no longer necessary to closing YGE though another yield-limiting factor may be present (e.g., hybrids, plant density, N supply, pest/disease management). In Northeast China, improving P management strategies other than applying more P fertilizer are crucial to closing yield gap and achieving high PUE, due to low soil temperature during spring that inhibits development of maize even in areas with high soil P fertility. Rhizosphere-based P managements, such as banding starter P fertilizer (Shen, et al., 2018) or increasing the colonization of roots by AM fungi (Hou, et al., 2021), provide effective approaches to improving PUE and closing the yield gap by exploiting the biological potential for efficient P acquisition by maize and achieve higher crop yields.
For the regional level, uniform P management strategies in hot spots would be a valuable first step to narrowing the variation in YGE. First, improvements in agro-technical service provision and agricultural machinery support could be offered to help farmers improve sowing quality, balance P concentrations, and to adopt proper plant-protection controls. Second, encouraging land transfer to merge small farms into larger land holdings should improve P use efficiency and yield.
The Chinese government has more than tripled its investment in agricultural research since 2000 to realize its goal of a 30–50% increase in crop yields. It has allocated 3 billion RMB annually since 2008 to a national network of organizations for developing modern agricultural technology (Zhang et al., 2013). Identifying the regional attribution benefits could help show the priorities for future adaptation practices aimed at resuming yield growth. This information may help policymakers identify areas of high potential for increased maize yield and enable the development of targeted innovative approaches.
5 Conclusion
In this study, we explored factors associated with yield potential and yield gaps in spring maize production. Our analyses provided insights into the causes of regional variation among counties in Northeast China and highlighted the constraints imposed by current P management practice and also the potential for closing the yield gap. Our results showed that farmers’ fields achieved 52% of the model yield potential and 77% of the attainable potential yield. Widely different amounts of P fertilizer input among farmers contributed significantly to regional variations in the YGE. Soil Olsen-P and rainfall were also major factors. Our results indicate that there is great potential to substantially increase maize yield in non-optimal P management regions, such as western Jilin Province. Hence, improvements in regional P management strategies, such as at the county level, need to be assessed separately to provide a basis for increasing actual maize yield. This study has demonstrated how to identify areas that would benefit most from improved cultivation practices and provides useful information for proper management strategies to close the yield gap.
Data availability statement
The original contributions presented in the study are included in the article/Supplementary Material; further inquiries can be directed to the corresponding author.
Author contributions
NC and JC designed the research and supervised the project. WS, YZ, MP, YS, WL, PL, ZL, and LS were key players for the field trials and collected data. MP and YS analyzed the data and verified the analytical methods. WS, YZ, NC, and ZC wrote the manuscript.
Funding
This study was funded by the National Key Research and Development Program of China (2017YFD0200202-2) and the Jilin Scientific and Technological Development Program (20190301022NY and 2020201124JC).
Conflict of interest
The authors declare that the research was conducted in the absence of any commercial or financial relationships that could be construed as a potential conflict of interest.
Publisher’s note
All claims expressed in this article are solely those of the authors and do not necessarily represent those of their affiliated organizations, or those of the publisher, the editors, and the reviewers. Any product that may be evaluated in this article, or claim that may be made by its manufacturer, is not guaranteed or endorsed by the publisher.
Reference
Chen, X. P., Cui, Z. L., Vitousek, P. M., Cassman, K. G., Matson, P. A., Bai, J. S., et al. (2011). Integrated soil-crop system management for food security. Proc. Natl. Acad. Sci. U. S. A. 108 (16), 6399–6404. doi:10.1073/pnas.1101419108
Chen, X., Cui, Z., Fan, M., Vitousek, P., Zhao, M., Ma, W., et al. (2014). Producing more grain with lower environmental costs. Nature 514 (7523), 486–489. doi:10.1038/nature13609
Ciampitti, I. A., and Vyn, T. J. (2014). Understanding global and historical nutrient use efficiencies for closing maize yield gaps. Agron. J. 106 (6), 2107–2117. doi:10.2134/agronj14.0025
Cui, Z. L., Dou, Z. X., Chen, X. P., Ju, X. T., and Zhang, F. S. (2014). Managing agricultural nutrients for food security in China: Past, present, and future. Agron. J. 106 (1), 191–198. doi:10.2134/agronj2013.0381
Cui, Z., Yue, S., Wang, G., Meng, Q., Wu, L., Yang, Z., et al. (2013). Closing the yield gap could reduce projected greenhouse gas emissions: a case study of maize production in China. Glob. Chang. Biol. 19 (8), 2467–2477. doi:10.1111/gcb.12213
David Tilman, C. B., Hillc, Jason, Befort, Belinda L., and Befort, B. L. (2011). Global food demand and the sustainable intensification of agriculture. Proc. Natl. Acad. Sci. U. S. A. 108, 20260–20264. doi:10.1073/pnas.1116437108
Gong, Z. T., Chen, Z. C., Luo, B. G., Zhang, G. L., and Zhao, W. J. (1999). Reference to chinese soil taxonomy. Soils 2, 57–63.
Grassini, P., Thorburn, J., Burr, C., and Cassman, K. G. (2011). High-yield irrigated maize in the western U.S. Corn belt: I. On-farm yield, yield potential, and impact of agronomic practices. Field Crops Res. 120 (1), 142–150. doi:10.1016/j.fcr.2010.09.012
Jilin Provincial Bureau of Statistics (2009). Jilin statistical year book (2009). China Statistics Press.
Jilin Province Development and Reform Commission (2013). The major function-oriented zones planning of Jilin Province. The People's Government of Jilin Province, 20131106. Available at http://jldrc.jl.gov.cn/fzgz/201311/t20131106_5212662.html
Li, C. L., Wang, J. F., You, D., Li, D. Z., and Gao, Q. (2011). Investigation on the formula fertilization by soil testing of rural households in Jilin province. J. Anhui Agric. Sci. 39 (06), 3395–3396+3407. doi:10.13989/j.cnki.0517-6611.2011.06.127
Li, J., Xie, R. Z., Wang, K. R., Ming, B., Guo, Y. Q., Zhang, G. Q., et al. (2015). Variations in maize dry matter, harvest index, and grain yield with plant density. Agron. J. 107 (3), 829–834. doi:10.2134/agronj14.0522
Licker, R., Johnston, M., Foley, J. A., Barford, C., Kucharik, C. J., Monfreda, C., et al. (2010). Mind the gap: how do climate and agricultural management explain the ‘yield gap’ of croplands around the world? Glob. Ecol. Biogeogr. 19, 769–782. doi:10.1111/j.1466-8238.2010.00563.x
Liu, Z., Yang, X., Hubbard, K. G., and Lin, X. (2012). Maize potential yields and yield gaps in the changing climate of northeast China. Glob. Chang. Biol. 18 (11), 3441–3454. doi:10.1111/j.1365-2486.2012.02774.x
Liu, Z., Yang, X., Lin, X., Hubbard, K. G., Lv, S., and Wang, J. (2016). Maize yield gaps caused by non-controllable, agronomic, and socioeconomic factors in a changing climate of Northeast China. Sci. Total Environ. 541, 756–764. doi:10.1016/j.scitotenv.2015.08.145
Lv, S., Yang, X., Lin, X., Liu, Z., Zhao, J., Li, K., et al. (2015). Yield gap simulations using ten maize cultivars commonly planted in Northeast China during the past five decades. Agric. For. Meteorology 205, 1–10. doi:10.1016/j.agrformet.2015.02.008
Meng, Q., Hou, P., Wu, L., Chen, X., Cui, Z., and Zhang, F. (2013). Understanding production potentials and yield gaps in intensive maize production in China. Field Crops Res. 143, 91–97. doi:10.1016/j.fcr.2012.09.023
Tao, F., Zhang, S., Zhang, Z., and Rotter, R. P. (2015). Temporal and spatial changes of maize yield potentials and yield gaps in the past three decades in China. Agric. Ecosyst. Environ. 208, 12–20. doi:10.1016/j.agee.2015.04.020
The Ministry of Agriculture of the People's Republic of China, National bureau of statistics of China (2015). China agriculture yearbook (2015). Beijing, China: China Agriculture Press.
Townsend, A. R., and Porder, S. (2012). Agricultural legacies, food production and its environmental consequences. Proc. Natl. Acad. Sci. U. S. A. 109 (16), 5917–5918. doi:10.1073/pnas.1203766109
Weifeng Zhang, G. C., Li, Xiaolin, Zhang, Hongyan, Wang, Chong, Liu, Quanqing, Liu, Q., et al. (2016). Closing yield gaps in china by empowering smallholder farmers. Nature 537, 671–674. doi:10.1038/nature19368
Zhang, F., Chen, X., and Vitousek, P. (2013). An experiment for the world. Nature 497 (7447), 33–35. doi:10.1038/497033a
Zhang, F. S., Chen, X. P., and Chen, Q. (2010). Fertilization guide of major crop species in China. China Agricultural University Press.
Keywords: Northeast China, spring maize, yield gap, phosphorus fertilizer management, yield potential
Citation: Shi W, Zhang Y, Peng M, Shi Y, Li W, Liu P, Li Z, Song L, Cao N, Cui J and Cui Z (2022) Closing county-level yield gaps through better phosphorus fertilizer management in Northeast China. Front. Environ. Sci. 10:929802. doi: 10.3389/fenvs.2022.929802
Received: 27 April 2022; Accepted: 07 July 2022;
Published: 10 August 2022.
Edited by:
Haigang Li, Inner Mongolia Agricultural University, ChinaReviewed by:
Shaojun Qiu, Institute of Agricultural Resources and Regional Planning (CAAS), ChinaMetin Turan, Yeditepe University, Turkey
Yu Wang, Institute of Soil Science (CAS), China
Copyright © 2022 Shi, Zhang, Peng, Shi, Li, Liu, Li, Song, Cao, Cui and Cui. This is an open-access article distributed under the terms of the Creative Commons Attribution License (CC BY). The use, distribution or reproduction in other forums is permitted, provided the original author(s) and the copyright owner(s) are credited and that the original publication in this journal is cited, in accordance with accepted academic practice. No use, distribution or reproduction is permitted which does not comply with these terms.
*Correspondence: Ning Cao, Y2FvX25pbmdAamx1LmVkdS5jbg==
†These authors have contributed equally to this work