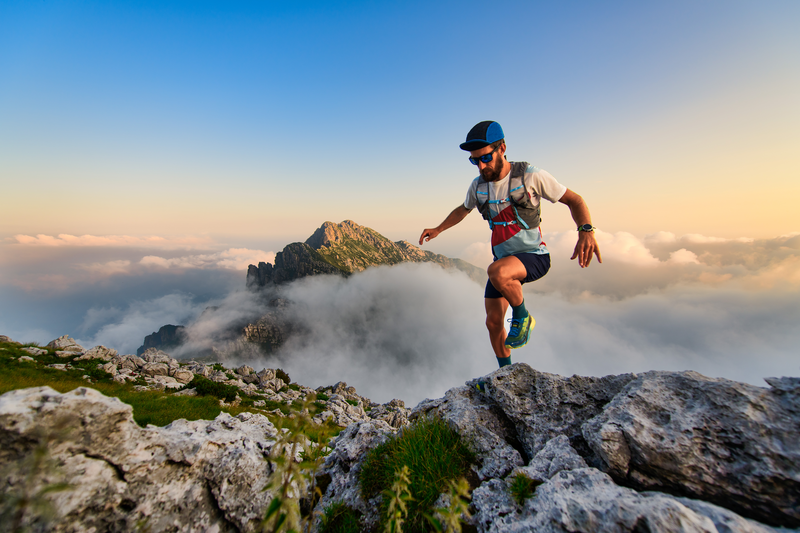
94% of researchers rate our articles as excellent or good
Learn more about the work of our research integrity team to safeguard the quality of each article we publish.
Find out more
MINI REVIEW article
Front. Environ. Sci. , 05 July 2022
Sec. Environmental Informatics and Remote Sensing
Volume 10 - 2022 | https://doi.org/10.3389/fenvs.2022.928064
This article is part of the Research Topic Rock Landslide Risk Assessment, Stability Analysis and Monitoring for The Development of Early Warning Systems and Reinforcement Measures View all 24 articles
Geotechnical anchoring technology is an important tool for disaster prevention and mitigation in slope engineering. Anchor bolts which are commonly used in slope engineering can be divided into prestressed anchors and non-prestressed anchors. Due to the superiority of anchor support technology, research on various aspects of anchor bolts, such as mechanical mechanism, anchorage effect, and the development of new-type anchor bolts, has been a significant research topic for scholars. This mini-review sums up the diverse past and current literature on anchor support technology of slope engineering in China. It focuses on the characteristics, applications, research methods, and practical cases of anchor bolts and briefly describes the history of slope anchor bolt development in China in the past 3 decades. Nowadays, the demand for engineering construction processes is increasing, and engineering geological conditions are becoming more complex, which promotes the development of anchor support technology. At the international level, achieving carbon neutrality is both an international trend and a general objective. Against the background of global commitment to carbon neutrality, the potential future perspectives for the developments of anchor support technology have been prospected in light of actual engineering needs.
Landslide is one of the most common geological disasters in the world, which causes great harm to the life and property of people. Geotechnical anchoring technology is an important tool for disaster prevention and mitigation. As a significant branch of geotechnical engineering, geotechnical anchoring techniques are widely applied to engineering construction due to their economy and efficiency (Ding et al., 2022). Anchor bolts play a crucial role in geotechnical anchoring (Shan et al., 2021), which could restrain the displacement of rock and soil mass (Nie et al., 2014b) so as to prevent disasters, such as landslides (Xu et al., 2014) and rock avalanches (Fan et al., 2017).
Anchor bolts have been exploited for over 100 years to reinforce rock and soil mass, which dated back to 1913, where they were generally utilized in the American mining industry until the 1940s (Grasselli, 2005). Due to the superiority of anchor support technology, China has been using anchor bolts in coal systems since the 1950s (Kang, 2016). With the development of China’s hydraulic engineering, power engineering, and urban construction, the application of anchor support technology in China entered a boom period in the 1980s (Cheng, 2001).
The reinforcement effects of anchor bolts are apparent as they can transfer the tension caused by rock deformation to the stable rock layer. The dangerous rock mass is combined with the stable rock mass through bolts (Li et al., 2016; Li Y. et al., 2017). Compared with the original rock, the uniaxial compressive strength and elastic modulus of the bolted rock improve to some extent (Yang et al., 2020). The bolting area can limit the extension and propagation of tensile cracks and slow down the failure process (Zong et al., 2014).
In this mini-review, we sum up the diverse past and current literature about anchor support technology of slope engineering in China. The characteristics, applications, and practical cases of various types of anchor bolts are the focus of our attention. Some research methods and the development history of anchor support technology are briefly described, and finally, the potential future perspectives for developments in the field are highlighted.
In slope engineering, anchors bolts are divided into two categories: the prestressed anchors and the non-prestressed anchors; the former can also be called prestressed anchor cables if the reinforcement body consists of steel strands and wires, while the latter are often called full grouted bolts (Cheng et al., 2015). The prestressed anchor implements the pre-stress in advance is a sort of active supporting technology, while the non-prestressed anchor is activated by the deformation of the surrounding rock; this is a sort of passive supporting technology (Guo et al., 2019).
Prestressed anchors have excellent characteristics of lightweight and high strength, which could decrease the amount of material for reinforcing structures so that the weight of the supporting structure would be controlled (Deng et al., 2021). In order to ensure the optimal position and length of the prestressed anchors, previous scholars have carried out remarkable studies. Hryciw (1991) proposed a theoretical research to confirm the optimum orientation of anchors by surface load. Yang et al. (2015) obtained a better reinforcement arrangement of prestressed anchors which relied on slope stress and displacement fields. An et al. (2020) presented a new three-dimensional optimization equation for the anchorage direction angle. Although the prestressed anchors can efficiently reinforce slopes, they still have many limitations. Elices et al. (2012) found that the common prestressed anchors with damage fail to warn against brittle failures. In addition to brittle failures caused by loads greatly exceeding the strength, stress corrosion cracking in complex geotechnical environments is another major cause of the deterioration (Karalis et al., 2012; Mak et al., 2019). Wang Y. et al. (2019) showed that the anchor head is relatively fragile through the collection and analysis of field samples. Sun et al. (2021)demonstrated that shear failure occurs with the extension and evolution of cracks by means of laboratory simulation tests. As a main defect of the prestressed anchors, it is necessary to study the mechanism of prestressing loss (Gao et al., 2021). Zhang et al. (2009) showed that the prestressing loss is divided into three parts according to the construction process: tensioning, locking, and time-dependent and specified the calculation formulas. Chen et al. (2013) further divided the prestressing loss into two parts based on a soft rock slope measurement analysis: instantaneous loss and time loss. Liu et al. (2021) revealed that the accelerated creep of the rock mass will contribute to tension failure or debonding failure of the bolt. Prestressed anchors are suitable for most projects, and they are most suitable for the excavation of high-steep slopes (Yang et al., 2020).
Passive, fully grouted bolts can provide extra tensile and shear strength to the joints it intersects at the anchorage part when installed in a jointed rock mass, and it is a crucial support technology for the joint rock slope (Siad, 2001). The rock–bolt interface of fully grouted bolts is formed by bonding the grout materials (cement, resin, etc.), which generates the load-bearing capacity by its shear strength (Nie et al., 2014a). This shear strength is related to the frictional rather than bonding strength of the rock–bolt interface (Hyett et al., 1992). Furthermore, the relatively high axial load may motivate the formation of slippage at the rock bolt–grout interface, which results in the loss of supporting capability (Kaiser et al., 1992). Freeman (1978) explored the behavior of fully grouted bolts relatively early. The following scholars used laboratory tests, analytical methods, and numerical models in studying the mechanical property of fully grouted bolts (Hyett et al., 1996; Li and Stillborg, 1999; Blanco Martín et al., 2013; Liu and Li, 2020; Singh and Spearing, 2021). The increase in bolt length and cross-sectional area can boost the bearing capacity of the fully grouted bolts through the data of tests (Benmokrane et al., 1995; Kılıc et al., 2002). The strength of the rod material is one of the main factors in the bearing capacity of the fully grouted bolts (Li and Liu, 2019), and the bolting angle, properties of grouting materials, and rock mass quality also have an impact (Feng et al., 2017; Li and Liu, 2019). It is understood that ultima failure is caused by debonding of the rock–bolt interface if the bolt tensile strength is relatively strong (Li and Stillborg, 1999). Ho et al. (2019) confirmed that under high confining pressures, the damage is mainly due to shear failure, while under low confining pressures, the grout is destroyed by cracking from expansion. Luga and Periku (2021) also obtained similar results through multiple in situ fully grouted bolt pull-out tests.
It is indispensable to rationalize the anchoring scheme and maximize the supporting effect of anchor bolts. This is possible through the research of the optimal installation position and failure mechanism, mentioned above, which have their own unique contributions. Revealing the failure mechanism facilitates the innovation of new-type anchor bolts. Table 1 summarizes the features, advantages, disadvantages, applications, examples, and practical cases of some geotechnical anchors.
TABLE 1. Summary of the features, advantages, disadvantages, examples, and practical cases of some geotechnical anchors (Siad, 2001; Wu et al., 2005; Qin et al., 2007; Wang et al., 2008; Dong et al., 2010; Nie et al., 2014a; Cheng et al., 2015; Xu and Yin, 2016; Zhu et al., 2017; Wu S. G. et al., 2018; Wu X. et al., 2018; Tao et al., 2018; Mak et al., 2019; Tang et al., 2019; Gao et al., 2021).
Table 1 summarizes the features, advantages, disadvantages, examples, and practical cases of some geotechnical anchors (Siad, 2001; Wu et al., 2005; Qin et al., 2007; Wang et al., 2008; Dong et al., 2010; Nie et al., 2014a; Chen et al., 2014; Cheng et al., 2015; Xu and Yin, 2016; Zhu et al., 2017; Wu S. G. et al., 2018; Wu X. et al., 2018; Tao et al., 2018; Mak et al., 2019; Tang et al., 2019; Gao et al., 2021).
Before 2000s, anchor support technology was mostly exploited for the reinforcement and prevention of high-steep rock slopes of hydropower stations, open-pit mines, and highways (Zhang, 1995; Han et al., 1996; Li and Zhao, 1997). With the vigorous construction of the Three Gorges Project, the complex geological conditions in the Three Gorges area have given rise to numerous research needs and significant research on slope reinforcement, including excavation and reinforcement design, planar finite element calculations, stability analysis, field tests, and the establishment of the constitutive model for the high-steep rock slopes of the Three Gorges locks (Zhu and Zhang, 1996; Xu and Yang, 1997; Zhang and Zhu, 1997; Gao and Yang, 1998; Xu, 2000; Zhu and Cheng, 2000). Between 2000 and 2012, the research hotspots were the development of new anchor bolts and the study of the mechanical effects of anchorage. The development of load-dispersed anchors (cables), repeated grouting techniques, hollow-grouted anchors, and under-reamed anchors has occurred rapidly (Cheng, 2005). For the study of mechanical properties and the support effect of anchorage, numerical simulation and mechanical tests are mainly used (Zhang and Chen, 2003; Lin et al., 2005; Li et al., 2012). Inheriting previous developments, more complex problems were discovered during the project since 2012. When excavating slopes in areas of high in situ stress or extremely soft rock, ordinary anchors (cables) can no longer meet the needs of deformation in these areas, and the development of yield-absorbing anchors has become a significant research topic (Zhu et al., 2017).
In Table 2, we summarize the anchor bolts adopted by Chinese engineers in dealing with different slopes and the performances of practical engineering.
TABLE 2. Summary of the anchor bolts used as support for different kinds of slopes and their performance (Wang et al., 2008; Tang et al., 2012; Zhou et al., 2013; Nie et al., 2014a; Tao et al., 2015; Zhu et al., 2017; Bai et al., 2018; Xiang et al., 2022).
In terms of a performance study of anchor bolts, mechanical test, numerical simulation, and non-destructive test are three common research methods. Conventional mechanical tests generally include tensile test (Chang et al., 2017), pull-out test (Teymen and Kılıç, 2018; Xu et al., 2018), shear test (Li et al., 2019), shaking table test (Su et al., 2021), the drop hammer test, and the Split Hopkinson Pressure Bar test (Wu et al., 2019; Wang et al., 2022). Those tests include comparatively accurate mechanical properties such as tensile strength, elongation, and shear strength. However, the conventional mechanical tests are mostly laboratory tests, which are difficult to be applied in practical engineering situations and relatively expensive (Li et al., 2019).
The emergence of numerical simulation technology was welcomed by scholars due to its high precision, economy, and repeatability. By setting different working conditions, numerical simulation technology can calculate the parameters of anchor bolts in various working conditions (Chen and Li, 2022). Numerical analysis methods can be divided into continuous analysis methods and discontinuous analysis methods. Apparently, the finite element method (FEM) is the most preferred numerical method because of its applicability for solving mechanical problems (Soparat and Nanakorn, 2008). Advances in computer arithmetic have driven advances in discontinuous analysis methods (Yokota et al., 2019b). The discrete element method (DEM) can model jointed rock masses by using UDEC (Gao and Kang, 2016). The discontinuous deformation analysis (DDA) can efficiently simulate the crack formation and evolution (Yokota et al., 2019a).
It is difficult to obtain the installation defects and progressive damage to the installed anchor bolts. The installation defects and progressive damage are significant factors. Unlike the first two research methods, a non-destructive test (NDT) can carry out a quality inspection and assessment of the installed anchor bolts. For instance, in the aspect of bonding quality inspection of fully grouted bolts, vibration methods and wave propagation methods are two commonly used methods (Zima and Rucka, 2017; Bačić et al., 2020). Liu L. et al. (2022) proposed a new stress wave reflection NDT method using a two-sensor acquisition. Liu L. L. et al. (2022) presented an effective means of detecting internal defects based on an ultrasonic waveguide and an improved empirical mode decomposition method. Nevertheless, it is difficult to obtain the mechanical parameters of the installed anchors, such as the actual bearing capacity, which needs to be addressed.
The ideal anchor provides both high load capacity and a certain amount of yield energy absorption (Chunlin Li, 2010). The earliest concept of energy-absorbing anchors was introduced in South Africa in the late 20th century and was applied in South African coal mines (Li et al., 2014). The currently available energy-absorbing anchors mainly achieve the purpose of absorbing energy through the yielding structure (Wu X. et al., 2018), such as the Garford bolt (Varden, 2009), Roofex (Charette and Plouffe, 2007), the CRLD bolt (He et al., 2014), etc. However, there are relatively few innovative studies on rod material. The team of He successfully developed a new type of anchor steel rebar with a negative Poisson’s ratio effect (Gu et al., 2022). The new-type NPR anchor steel can balance the contradiction between high strength and high ductility of metal materials and realize the large deformation and energy-absorbing characteristics of anchor bolts from the material nature (Tao et al., 2022). According to the recent results of the drop hammer test and Split Hopkinson Pressure Bar Test, the elongation and energy-absorbing capacity of the constant resistance energy-absorbing material are superior to those of the common materials (Wang et al., 2022). The successful development of NPR anchor steel proves that the effect of ideal anchor bolts can also be achieved through the innovation of rod materials and that the combination of a reasonable yield-absorbing structure can maximize its yield-absorbing effect.
Metal anchors are often corroded by water seeping through cracks in the rock or by solutions containing chloride and sulfate ions (Gamboa and Atrens, 2003; Villalba and Atrens, 2009; Karalis et al., 2012; Kang et al., 2013). Investigations of anchor bolt failures have shown that the life of anchor bolts is principally controlled by corrosion, which influences the exposed free length (Jiang et al., 2014), while nonmetallic anchors based on fiber-reinforced polymer bars can overcome this drawback (Xu and Yin, 2016). In recent years, there have been many studies on the performance of glass fiber–reinforced polymer (GFRP) materials (Dutta and Hui, 2000; Yeung et al., 2007; Yu et al., 2007; Keller et al., 2008; Li C. et al., 2017). It is believed that GFRP materials and high-pressure grouting technology are sufficient to substitute steel reinforcement (Yeung et al., 2007). After glass fiber–reinforced polymer (GFRP), carbon fiber–reinforced polymer (CFRP), and aramid fiber composite–reinforced polymer (AFRP) are known, basalt fiber composite–reinforced polymer (BFRP) has arisen the interest of scholars (Lu and Xian, 2018; Wang X. et al., 2019; Bai et al., 2020). Compared to carbon fiber, basalt fiber is cheaper and simpler to manufacture (Larrinaga et al., 2014); compared to glass fiber, the mechanical performance is similar or even better (Fiore et al., 2015). Basalt fiber has excellent modulus, high strength and temperature resistance, non-toxic, natural, stable, easy to process, eco-friendly, and relatively cheap (Lopresto et al., 2011; Wei et al., 2011; Borhan, 2012). Compared with ordinary steel anchor bolts in laboratory shear test, the peak shear strength of the BFRP anchor rod is relatively low, while the residual shear strength and the ductility are relatively high, and the anchoring effect of the jointed rock body anchored by BFRP anchors is affected by the anchoring angle, and the shear strength is relatively higher when the anchoring angle is less than 60° (Zhang et al., 2022). The BFRP rebar was mostly exploited in reinforced concrete structures, and nowadays, it is increasingly applied in slope reinforcement engineering due to its excellent performance and progress of the process (Wu et al., 2021). Combined with the practical engineering needs, it is of extraordinary significance to design and manufacture new anchor bolts with mature properties, which is a research hot spot (Alraie et al., 2021).
Research on ecological slope protection technology has been a significant research topic, which can ensure the required strength and is more eco-friendly. Ecological slope protection and carbon reduction can be achieved by reducing the use of steel and integrating ecology and anchoring technologies. FRP material has great potential to replace steel and is environmentally friendly. FRP anchors have high tensile strength and strong corrosion resistance but insufficient shear strength (Guo et al., 2018). How to improve the shear strength of FRP anchors is a critical issue. The plant root system not only retains soil and water but also has been proven to be effective in strengthening slopes (Lin et al., 2010; Ma’ruf, 2012; Cao et al., 2018). Integrating anchoring technology with the ecological environment is another direction to achieving low-carbon anchoring. This could attain the balance between ecological performance and mechanical performance (Su et al., 2021). Su et al. (2018) proposed new style of ecological slope protection using “Anchor + Hinged Block”, which realized a better reinforcement and seismic performance. In addition, as a new-type material that has both biocompatibility and engineering function, it has not been studied; for instance, the feasibility of vegetation growing recycled concrete for grouting (Wang F. et al., 2019). In the context of global efforts to achieve carbon neutrality, these low-carbon and eco-friendly slope anchoring technologies will certainly become a vital part of slope support technology in the future.
In China, the anchor support technology for slope engineering is pretty mature. A reasonable anchoring scheme according to the actual slope conditions needs to be selected in order to maximize the support capacity of anchors and ensure engineering quality and safety.
1) In this mini-review, we introduce geotechnical anchors for slope engineering and their research methods, briefly describe the history of anchor development, and summarize the anchor bolts used to support different kinds of the slopes and their performances. This serves as a reference for engineers to quickly select applicable anchor bolts according to the actual engineering conditions. Meanwhile, we highlight the potential future perspectives for developments in the field.
2) In terms of improving the anchor support capacity, there are more improvements to the anchor structure but less development and use of new materials. FRP material has great potential to replace steel and is environmentally friendly; however, shear strength is insufficient. Finally, it is difficult to obtain the mechanical property parameters of the installed anchors by the existing research methods.
3) Nowadays, engineering geological conditions are becoming more complex and the ecological environment is more fragile, such as Sichuan—Tibet railway. The improvement of anchoring technology should be based on the actual engineering situation. In the context of global efforts to achieve carbon neutrality, scholars should strengthen the research on the integration of ecological slope protection and anchor support technology, which will contribute to the goal of carbon neutrality.
YD, HL, and LH were responsible for the work concept or design; HL were responsible for literature collection; YD and HL were responsible for drafting the manuscript; YD, HL, and SDC were responsible for making important revisions to the manuscript; YD and HL were responsible for approving the final version of the manuscript for publication.
The National Key Research and Development Program of China (2018YFE0101100), the National Natural Science Foundation of China (41702371), USTB-NTUT Joint Research Program (TW2019011), and State Key Laboratory for GeoMechanics and Deep Underground Engineering, China University of Mining and Technology, Beijing (SKLGDUEK2130).
The authors declare that the research was conducted in the absence of any commercial or financial relationships that could be construed as a potential conflict of interest.
All claims expressed in this article are solely those of the authors and do not necessarily represent those of their affiliated organizations, or those of the publisher, the editors, and the reviewers. Any product that may be evaluated in this article, or claim that may be made by its manufacturer, is not guaranteed or endorsed by the publisher.
This work was supported by the National Key Research and Development Project of China (2018YFE0101100), the National Natural Science Foundation of China (41702371), USTB-NTUT Joint Research Program (TW2019011), and State Key Laboratory for GeoMechanics and Deep Underground Engineering, China University of Mining and Technology, Beijing (SKLGDUEK2130).
Alraie, A., Sahoo, D. R., and Matsagar, V. (2021). Development of Optimal Anchor for Basalt Fiber-Reinforced Polymer Rods. J. Compos. Constr. 25 (3), 04021011. doi:10.1061/(ASCE)CC.1943-5614.0001119
An, C. L., Liang, Y., Wang, L. Q., Deng, S., Sun, Z. H., Fan, B. Q., et al. (2020). Three-dimensional Optimization Design for the Direction Angle of Anchor Cable Reinforcement in Wedge Rock Slope. Rock Soil Mech. 41 (8), 2765–2772. doi:10.16285/j.rsm.2019.1710
Bačić, M., Kovačević, M. S., and Jurić Kaćunić, D. (2020). Non-Destructive Evaluation of Rock Bolt Grouting Quality by Analysis of its Natural Frequencies. Materials 13 (2), 282. doi:10.3390/ma13020282
Bai, X. Y., Jing, D. S., Wang, H. G., Yan, N., Wang, Y. H., and Zhang, M. Y. (2020). Research Status of Basalt Fiber-Reinforced Polymer Anchor in Slope Support Engineering. Sci. Technol. Eng. 20 (31), 12702–12710. doi:10.3969/j.issn.1671-1815.2020.31.004
Bai, X. Y., Zhang, M. Y., Kuang, Z., Wang, Y. H., Yan, N., and Zhu, L. (2018). Application of Fiber Grating Sensing Technology in Pull-Out Test on Glass Fiber Reinforced Polymer Anti-floating Anchor. Rock Soil Mech. 39 (10), 3891–3899. doi:10.16285/j.rsm.2017.2395
Benmokrane, B., Chennouf, A., and Mitri, H. S. (1995). Laboratory Evaluation of Cement-Based Grouts and Grouted Rock Anchors. Int. J. Rock Mech. Min. Sci. Geomechanics Abstr. 32 (7), 633–642. doi:10.1016/0148-9062(95)00021-8
Blanco Martín, L., Tijani, M., Hadj-Hassen, F., and Noiret, A. (2013). Assessment of the Bolt-Grout Interface Behaviour of Fully Grouted Rockbolts from Laboratory Experiments under Axial Loads. Int. J. Rock Mech. Min. Sci. 63, 50–61. doi:10.1016/j.ijrmms.2013.06.007
Borhan, T. M. (2012). Properties of Glass Concrete Reinforced with Short Basalt Fibre. Mater. Des. 42, 265–271. doi:10.1016/j.matdes.2012.05.062
Cao, W., Omran, B. A., Lei, Y., Zhao, X., Yang, X., Chen, Q., et al. (2018). Studying Early Stage Slope Protection Effects of Vegetation Communities for Xinnan Highway in China. Ecol. Eng. 110, 87–98. doi:10.1016/j.ecoleng.2017.08.033
Chang, X., Wang, G., Liang, Z., Yang, J., and Tang, C. (2017). Study on Grout Cracking and Interface Debonding of Rockbolt Grouted System. Constr. Build. Mater. 135, 665–673. doi:10.1016/j.conbuildmat.2017.01.031
Charette, F., and Plouffe, M. (2007). “Roofex® –Results of Laboratory Testing of a New Concept of Yieldable Tendon,” in Deep Mining 2007: Fourth International Seminar on Deep and High Stress Mining. Editor Y. Potvin (Perth: Australian Centre for Geomechanics).
Chen, J., and Li, D. (2022). Numerical Simulation of Fully Encapsulated Rock Bolts with a Tri-linear Constitutive Relation. Tunn. Undergr. Space Technol. 120, 104265. doi:10.1016/j.tust.2021.104265
Chen, T. L., Zhou, J., Wang, A. M., and Li, H. (2014). “Application of Self-Drilling Anchor on Coal Gangue Slope Treatment,” in Advances in Civil and Industrial Engineering IV (Haikou, China: Trans Tech Publications). doi:10.4028/www.scientific.net/amm.580-583.935
Chen, Y. J., Yin, J., and Hu, Y. F. (2013). Research on Prestress Quantitative Loss Law of Soft Rock Slope Anchor Cable. Chin. J. Rock Mech. Eng. 32 (8), 1685–1691. doi:10.3969/j.issn.1000-6915.2013.08.022
Cheng, L. K. (2001). Present Status and Development of Ground Anchorages. Chin. Civ. Eng. J. 03, 7–12+34. doi:10.3321/j.issn:1000-131X.2001.03.002
Cheng, L. K. (2005). Research and New Progress in Ground Anchorage. Chin. J. Rock Mech. Eng. 21, 5–13. doi:10.3321/j.issn:1000-6915.2005.21.001
Cheng, L. K., Zhang, P. W., and Wang, F. (2015). Several Mechanical Concepts for Anchored Structures in Rock and Soil. Chin. J. Rock Mech. Eng. 34 (04), 668–682. doi:10.13722/j.cnki.jrme.2015.04.003
Chunlin Li, C. (2010). A New Energy-Absorbing Bolt for Rock Support in High Stress Rock Masses. Int. J. Rock Mech. Min. Sci. 47 (3), 396–404. doi:10.1016/j.ijrmms.2010.01.005
Deng, D. P., Li, L., and Zhao, L. H. (2021). Stability Analysis of Slopes Reinforced with Anchor Cables and Optimal Design of Anchor Cable Parameters. Eur. J. Environ. Civ. Eng. 25 (13), 2425–2440. doi:10.1080/19648189.2019.1631216
Ding, W., Wang, Z., Huang, X., Chen, L., and Zheng, Y. (2022). Influence of Corrosion on Anchoring Bond Behavior of Jointed Rock Mass. KSCE J. Civ. Eng. 26 (4), 1914–1928. doi:10.1007/s12205-022-0324-x
Dong, J., Zhu, Y., Zhou, Y., and Ma, W. (2010). Dynamic Calculation Model and Seismic Response for Frame Supporting Structure with Prestressed Anchors. Sci. China Technol. Sci. 53 (7), 1957–1966. doi:10.1007/s11431-010-3241-z
Dutta, P. K., and Hui, D. (2000). Creep Rupture of a GFRP Composite at Elevated Temperatures. Comput. Struct. 76 (1), 153–161. doi:10.1016/S0045-7949(99)00176-5
Elices, M., Valiente, A., Caballero, L., Iordachescu, M., Fullea, J., Sánchez-Montero, J., et al. (2012). Failure Analysis of Prestressed Anchor Bars. Eng. Fail. Anal. 24, 57–66. doi:10.1016/j.engfailanal.2012.03.007
Fan, X., Xu, Q., Scaringi, G., Dai, L., Li, W., Dong, X., et al. (2017). Failure Mechanism and Kinematics of the Deadly June 24th 2017 Xinmo Landslide, Maoxian, Sichuan, China. Landslides 14 (6), 2129–2146. doi:10.1007/s10346-017-0907-7
Feng, X., Zhang, N., Li, G., and Guo, G. (2017). Pullout Test on Fully Grouted Bolt Sheathed by Different Length of Segmented Steel Tubes. Shock Vib. 2017, 1–16. doi:10.1155/2017/4304190
Fiore, V., Scalici, T., Di Bella, G., and Valenza, A. (2015). A Review on Basalt Fibre and its Composites. Compos. Part B Eng. 74, 74–94. doi:10.1016/j.compositesb.2014.12.034
Freeman, T. J. (1978). The Behaviour of Fully-Bonded Rock Bolts in the Kielder Experimental Tunnel. Tunn. Tunn. Int. 10 (5), 37–40.
Gamboa, E., and Atrens, A. (2003). Environmental Influence on the Stress Corrosion Cracking of Rock Bolts. Eng. Fail. Anal. 10 (5), 521–558. doi:10.1016/S1350-6307(03)00036-0
Gao, F. Q., and Kang, H. P. (2016). Effects of Pre-existing Discontinuities on the Residual Strength of Rock Mass - Insight from a Discrete Element Method Simulation. J. Struct. Geol. 85, 40–50. doi:10.1016/j.jsg.2016.02.010
Gao, F., and Yang, Q. G. (1998). Field Test of Rock Anchoring Technique for TGP. Yangtze River 1, 17–18+21+47. doi:10.16232/j.cnki.1001-4179.1998.01.006
Gao, X., Tian, W., Li, J., Qi, H., and Zhang, Z. (2021). Research on Prediction Model of Prestress Loss of Anchor Cable in Soil-Rock Dual-Structure Slope. Adv. Mater. Sci. Eng. 2021, 1–10. doi:10.1155/2021/4986245
Grasselli, G. (2005). 3D Behaviour of Bolted Rock Joints: Experimental and Numerical Study. Int. J. Rock Mech. Min. Sci. 42 (1), 13–24. doi:10.1016/j.ijrmms.2004.06.003
Gu, J. B., Wang, J. Y., and Lu, W. (2022). An Experimental Assessment of Ultra High Performance Concrete Beam Reinforced with Negative Poisson's Ratio (NPR) Steel Rebar. Constr. Build. Mater. 327, 127042. doi:10.1016/j.conbuildmat.2022.127042
Guo, X., Riad, A., Chennareddy, R., and Reda Taha, M. M. (2018). Seismic Resistance of GFRP Bolted Joints with Carbon Nanotubes. J. Eng. Mech. 144 (11), 04018106. doi:10.1061/(ASCE)EM.1943-7889.0001528
Guo, X. X., Wang, B., Ma, Z. W., and Wang, Z. Y. (2019). Testing Mechanical Properties of Rock Bolt under Different Supports Using Fiber Bragg Grating Technology. Sensors 19 (19), 4098. doi:10.3390/s19194098
Han, H. Z., Wen, J. Q., and Wang, X. N. (1996). Collapse Mechanism and Protective Measures of Expansive Rock Slope along Nankun Line. Chin. J. Rock Mech. Eng. 2, 88–93.
He, M., Gong, W., Wang, J., Qi, P., Tao, Z., Du, S., et al. (2014). Development of a Novel Energy-Absorbing Bolt with Extraordinarily Large Elongation and Constant Resistance. Int. J. Rock Mech. Min. Sci. 67, 29–42. doi:10.1016/j.ijrmms.2014.01.007
Ho, D. A., Bost, M., and Rajot, J. P. (2019). Numerical Study of the Bolt-Grout Interface for Fully Grouted Rockbolt under Different Confining Conditions. Int. J. Rock Mech. Min. Sci. 119, 168–179. doi:10.1016/j.ijrmms.2019.04.017
Hryciw, R. D. (1991). Anchor Design for Slope Stabilization by Surface Loading. J. Geotechnical Eng. 117117 (8), 12608–21274. doi:10.1061/(ASCE)0733-941010.1061/(asce)0733-9410(1991)117:8(1260)
Hyett, A. J., Bawden, W. F., and Reichert, R. D. (1992). The Effect of Rock Mass Confinement on the Bond Strength of Fully Grouted Cable Bolts. Int. J. Rock Mech. Min. Sci. Geomechanics Abstr. 29 (5), 503–524. doi:10.1016/0148-9062(92)92634-O
Hyett, A. J., Moosavi, M., and Bawden, W. F. (1996). Load Distribution along Fully Grouted Bolts, with Emphasis on Cable Bolt Reinforcement. Int. J. Numer. Anal. Methods Geomech. 20 (7), 517–544. doi:10.1002/(sici)1096-9853(199607)20:7<517::aid-nag833>3.0.co;2-l
Jiang, S. H., Li, D. Q., Zhang, L. M., and Zhou, C. B. (2014). Time-dependent System Reliability of Anchored Rock Slopes Considering Rock Bolt Corrosion Effect. Eng. Geol. 175, 1–8. doi:10.1016/j.enggeo.2014.03.011
Kaiser, P. K., Yazici, S., and Nosé, J. (1992). Effect of Stress Change on the Bond Strength of Fully Grouted Cables. Int. J. Rock Mech. Min. Sci. Geomechanics Abstr. 29 (3), 293–306. doi:10.1016/0148-9062(92)93662-4
Kang, H. P. (2016). Sixty Years Development and Prospects of Rock Bolting Technology for Underground Coal Mine Roadway in China. J. China Univ. Min. Technol. 45 (06), 1071–1081. doi:10.13247/j.cnki.jcumt.000583
Kang, H., Wu, Y., Gao, F., Lin, J., and Jiang, P. (2013). Fracture Characteristics in Rock Bolts in Underground Coal Mine Roadways. Int. J. Rock Mech. Min. Sci. 62, 105–112. doi:10.1016/j.ijrmms.2013.04.006
Karalis, D. G., Melanitis, N. E., and Pantelis, D. I. (2012). Failure Analysis of a Rock Anchor Made of Stainless Steel in Marine Environment. Eng. Fail. Anal. 19, 123–130. doi:10.1016/j.engfailanal.2011.09.011
Keller, T., Haas, C., and Vallée, T. (2008). Structural Concept, Design, and Experimental Verification of a Glass Fiber-Reinforced Polymer Sandwich Roof Structure. J. Compos. Constr. 12 (3), 454–468. doi:10.1061/(ASCE)1090-026810.1061/(asce)1090-0268(2008)12:4(454)
Kılıc, A., Yasar, E., and Celik, A. G. (2002). Effect of Grout Properties on the Pull-Out Load Capacity of Fully Grouted Rock Bolt. Tunn. Undergr. Space Technol. 17 (4), 355–362. doi:10.1016/S0886-7798(02)00038-X
Larrinaga, P., Chastre, C., Biscaia, H. C., and San-José, J. T. (2014). Experimental and Numerical Modeling of Basalt Textile Reinforced Mortar Behavior under Uniaxial Tensile Stress. Mater. Des. 55, 66–74. doi:10.1016/j.matdes.2013.09.050
Li, C. C., Stjern, G., and Myrvang, A. (2014). A Review on the Performance of Conventional and Energy-Absorbing Rockbolts. J. Rock Mech. Geotechnical Eng. 6 (4), 315–327. doi:10.1016/j.jrmge.2013.12.008
Li, C., Gao, D., Wang, Y., and Tang, J. (2017a). Effect of High Temperature on the Bond Performance between Basalt Fibre Reinforced Polymer (BFRP) Bars and Concrete. Constr. Build. Mater. 141, 44–51. doi:10.1016/j.conbuildmat.2017.02.125
Li, C., and Stillborg, B. (1999). Analytical Models for Rock Bolts. Int. J. Rock Mech. Min. Sci. 36 (8), 1013–1029. doi:10.1016/S1365-1609(99)00064-7
Li, L., Hagan, P. C., Saydam, S., Hebblewhite, B., and Zhang, C. (2019). A Laboratory Study of Shear Behaviour of Rockbolts under Dynamic Loading Based on the Drop Test Using a Double Shear System. Rock Mech. Rock Eng. 52 (9), 3413–3429. doi:10.1007/s00603-019-01776-x
Li, X., He, S., and Wu, Y. (2012). Limit Analysis of the Stability of Slopes Reinforced with Anchors. Int. J. Numer. Anal. Meth. Geomech. 36 (17), 1898–1908. doi:10.1002/nag.1093
Li, Y., Li, C., Zhang, L., Zhu, W., Li, S., and Liu, J. (2017b). An Experimental Investigation on Mechanical Property and Anchorage Effect of Bolted Jointed Rock Mass. Geosci. J. 21 (2), 253–265. doi:10.1007/s12303-016-0043-8
Li, Y., and Liu, C. (2019). Experimental Study on the Shear Behavior of Fully Grouted Bolts. Constr. Build. Mater. 223, 1123–1134. doi:10.1016/j.conbuildmat.2019.06.207
Li, Y. T., and Zhao, H. L. (1997). Reinforcement Design of High Rock Slope at Xiaolangdi Project Inlet. Yellow River 2, 52–56.
Li, Y., Zhou, H., Zhang, L., Zhu, W., Li, S., and Liu, J. (2016). Experimental and Numerical Investigations on Mechanical Property and Reinforcement Effect of Bolted Jointed Rock Mass. Constr. Build. Mater. 126, 843–856. doi:10.1016/j.conbuildmat.2016.09.100
Lin, D. G., Huang, B. S., and Lin, S. H. (2010). 3-D Numerical Investigations into the Shear Strength of the Soil-Root System of Makino Bamboo and its Effect on Slope Stability. Ecol. Eng. 36 (8), 992–1006. doi:10.1016/j.ecoleng.2010.04.005
Lin, H., Cao, P., and Zhen, Z. Y. (2005). Simulating Effects of Wholly Grouted Anchor Rods with FLAC3D. Rock Soil Mech. 26 (S2), 167–170. doi:10.16285/j.rsm.2005.s2.057
Liu, C., and Li, Y. (2020). Predicting the Shear Resistance Contribution of Passive Fully Grouted Bolts to Jointed Rock. Int. J. Geomech. 20 (2), 04019174. doi:10.1061/(ASCE)GM.1943-5622.0001581
Liu, L., Li, S., Jiang, X., and Tao, F. (2022a). A New Two-Sensor Non-destructive Testing Method of Grouted Rock Bolts. Constr. Build. Mater. 317, 125919. doi:10.1016/j.conbuildmat.2021.125919
Liu, L. L., Zhu, J., Zhang, S. H., and Sun, H. P. (2022b). Non-destructive Detection of Anchor Defects Using Ultrasonic Guided Wave and ICEEMDAN Method. Earth Sci. (Wuhan. China) 1, 17. doi:10.3799/dqkx.2022.102
Liu, Y., Zheng, P., and Wang, P. (2021). Multi-factors Influence of Anchorage Force on Surrounding Rock under Coupling Effect of Creep Rock Mass and Bolt/cable. Geomatics, Nat. Hazards Risk 12 (1), 328–346. doi:10.1080/19475705.2021.1872717
Lopresto, V., Leone, C., and De Iorio, I. (2011). Mechanical Characterisation of Basalt Fibre Reinforced Plastic. Compos. Part B Eng. 42 (4), 717–723. doi:10.1016/j.compositesb.2011.01.030
Lu, Z., and Xian, G. (2018). Resistance of Basalt Fibers to Elevated Temperatures and Water or Alkaline Solution Immersion. Polym. Compos. 39 (7), 2385–2393. doi:10.1002/pc.24220
Luga, E., and Periku, E. (2021). A Pioneer In-Situ Investigation on the Bearing Capacity and Failure Causes of Real Scale Fully Grouted Rockbolts. Constr. Build. Mater. 310, 124826. doi:10.1016/j.conbuildmat.2021.124826
Mak, M. W. T., Desnerck, P., and Lees, J. M. (2019). Corrosion-induced Cracking and Bond Strength in Reinforced Concrete. Constr. Build. Mater. 208, 228–241. doi:10.1016/j.conbuildmat.2019.02.151
Ma’ruf, M. F. (2012). Shear Strength of apus Bamboo Root Reinforced Soil. Ecol. Eng. 41, 84–86. doi:10.1016/j.ecoleng.2012.01.003
Nie, W., Zhao, Z. Y., Ning, Y. J., and Guo, W. (2014a). Numerical Studies on Rockbolts Mechanism Using 2D Discontinuous Deformation Analysis. Tunn. Undergr. Space Technol. 41, 223–233. doi:10.1016/j.tust.2014.01.001
Nie, W., Zhao, Z. Y., Ning, Y. J., and Sun, J. P. (2014b). Development of Rock Bolt Elements in Two-Dimensional Discontinuous Deformation Analysis. Rock Mech. Rock Eng. 47 (6), 2157–2170. doi:10.1007/s00603-013-0525-1
Qin, W. M., Ge, X. R., and Wang, H. (2007). Experimental Study on Sand Consolidated Anchorage Prestressed Bolt and its Applications. J. Rock Mech. Eng. 26 (4), 769–774. doi:10.1109/CISE.2010.5676771
Shan, R., Zhang, S., Xiao, S., and Liang, J. (2021). Research on Anchor Cable and C-Shaped Tube Support Method in Deep Layers Roadway, Experimental Study, and Numerical Simulation. Shock Vib. 2021, 1–13. doi:10.1155/2021/7537979
Siad, L. (2001). Stability Analysis of Jointed Rock Slopes Reinforced by Passive, Fully Grouted Bolts. Comput. Geotechnics 28 (5), 325–347. doi:10.1016/S0266-352X(01)00004-0
Singh, P., and Spearing, A. J. S. (2021). An Improved Analytical Model for the Elastic and Plastic Strain-Hardening Shear Behaviour of Fully Grouted Rockbolts. Rock Mech. Rock Eng. 54 (8), 3909–3925. doi:10.1007/s00603-021-02439-6
Soparat, P., and Nanakorn, P. (2008). Analysis of Anchor Bolt Pullout in Concrete by the Element-free Galerkin Method. Eng. Struct. 30 (12), 3574–3586. doi:10.1016/j.engstruct.2008.06.004
Su, H., Hang, Y., Song, Y., Mao, K., Wu, D., and Qiu, X. (2018). Seismic Response of Anchor + Hinged Block Ecological Slope by Shaking Table Tests. Adv. Mater. Sci. Eng. 2018, 1–13. doi:10.1155/2018/7684831
Su, H., Wu, D., Lu, Y., Peng, X., Wang, X., Chen, W., et al. (2021). Experimental and Numerical Study on Stability Performance of New Ecological Slope Protection Using Bolt-Hinge Anchored Block. Ecol. Eng. 172, 106409. doi:10.1016/j.ecoleng.2021.106409
Sun, G., Hu, J., Wang, H., and Li, P. (2021). Experimental Study on the Deformation and Failure Characteristics of Anchor under Graded Loading and Corrosion. Adv. Mater. Sci. Eng. 2021, 1–11. doi:10.1155/2021/8701180
Tang, H., Wasowski, J., and Juang, C. H. (2019). Geohazards in the Three Gorges Reservoir Area, China - Lessons Learned from Decades of Research. Eng. Geol. 261, 105267. doi:10.1016/j.enggeo.2019.105267
Tang, X. X., Luo, X. Y., Sun, Q., and Kuang, Y. C. (2012). “Test Research on Mechanical Property of GFRP Bolt under Freeze-Thaw Cycle Conditions,” in 2012 International Conference on Materials Science and Nanotechnology, November 16, 2012 - November 18, 2012 (Kem: Trans Tech Publications Ltd), 689–694. doi:10.4028/www.scientific.net/kem.531-532.689
Tao, Z. G., Li, H. P., Sun, G. L., Yin, L. J., and Zhang, X. L. (2015). Development of Monitoring and Early Warning System for Landslides Based on Constant Resistance and Large Deformation Anchor Cable and its Application. Rock Soil Mech. 36 (10), 3032–3040. doi:10.16285/j.rsm.2015.10.038
Tao, Z. G., Ren, S. L., He, M. C., and Xia, M. (2022). Study on Static Tensile and Bolting Shear Mechanical Properties of Micro-NPR Bolt Steel in Underground Engineering. J. China Coal Soc. 1, 14. doi:10.13225/j.cnki.jccs.XR21.1703
Tao, Z., Zhu, C., Zheng, X., and He, M. (2018). Slope Stability Evaluation and Monitoring of Tonglushan Ancient Copper Mine Relics. Adv. Mech. Eng. 10 (8), 168781401879170. doi:10.1177/1687814018791707
Teymen, A., and Kılıç, A. (2018). Effect of Grout Strength on the Stress Distribution (Tensile) of Fully-Grouted Rockbolts. Tunn. Undergr. Space Technol. 77, 280–287. doi:10.1016/j.tust.2018.04.022
Varden, R. P. (2009). “Implementation of the Garford Dynamic Bolt at Kanowna Belle Mine,” in Controlling Seismic Hazard and Sustainable Development of Deep Mines: 7TH International Symposium On Rockburst and Seismicity in Mines (RASIM7) (Dalian, China: Rinton Press).
Villalba, E., and Atrens, A. (2009). Hydrogen Embrittlement and Rock Bolt Stress Corrosion Cracking. Eng. Fail. Anal. 16 (1), 164–175. doi:10.1016/j.engfailanal.2008.01.004
Wang, F., Sun, C., Ding, X., Kang, T., and Nie, X. (2019a). Experimental Study on the Vegetation Growing Recycled Concrete and Synergistic Effect with Plant Roots. Materials 12 (11), 1855. doi:10.3390/ma12111855
Wang, Q., Xu, S., He, M., Jiang, B., Wei, H., and Wang, Y. (2022). Dynamic Mechanical Characteristics and Application of Constant Resistance Energy-Absorbing Supporting Material. Int. J. Min. Sci. Technol. 32 (3), 447–458. doi:10.1016/j.ijmst.2022.03.005
Wang, S. W., Jiang, T., Liu, H. D., and Gao, D. Y. (2008). Study on Testing in Reinforced Slope with Hollow Grouted Bolt. Chin. J. Rock Mech. Eng. 27 (Suppl. 2), 3963–3967. doi:10.3321/j.issn:1000-6915.2008.z2.096
Wang, X., Zhao, X., and Wu, Z. (2019b). Fatigue Degradation and Life Prediction of Basalt Fiber-Reinforced Polymer Composites after Saltwater Corrosion. Mater. Des. 163, 107529. doi:10.1016/j.matdes.2018.12.001
Wang, Y., Sun, X., and Ren, A. (2019c). Investigations of Rock Anchor Corrosion and its Influence Factors by Exhumations in Four Typical Field Sites. Eng. Fail. Anal. 101, 357–382. doi:10.1016/j.engfailanal.2019.03.022
Wei, B., Cao, H., and Song, S. (2011). Degradation of Basalt Fibre and Glass Fibre/epoxy Resin Composites in Seawater. Corros. Sci. 53 (1), 426–431. doi:10.1016/j.corsci.2010.09.053
Wu, H., Wu, Z., Lei, H., and Lai, T. (2021). Application of BRFP New-type Anchor Cable Material in High Slopes against Earthquakes. Adv. Civ. Eng. 2021, 1–19. doi:10.1155/2021/6689718
Wu, Q., Li, X., Weng, L., Li, Q., Zhu, Y., and Luo, R. (2019). Experimental Investigation of the Dynamic Response of Prestressed Rockbolt by Using an SHPB-Based Rockbolt Test System. Tunn. Undergr. Space Technol. 93, 103088. doi:10.1016/j.tust.2019.103088
Wu, S. C., Zhang, Y. P., and Gao, Y. T. (2005). Analysis of Cutting Slope Reinforcement in Swelling Rock and Soil. J. Univ. Sci. Technol. Beijing 27 (2), 137–141+155. doi:10.13374/j.issn1001-053x.2005.02.035
Wu, S. G., Fu, H. M., and Zhang, Y. Y. (2018a). Study on Anchorage Mechanism and Application of Tension-Compression Dispersive Anchor Cable. Rock Soil Mech. 39 (6), 2155–2163. doi:10.16285/j.rsm.2016.1904
Wu, X., Jiang, Y., Guan, Z., and Wang, G. (2018b). Estimating the Support Effect of Energy-Absorbing Rock Bolts Based on the Mechanical Work Transfer Ability. Int. J. Rock Mech. Min. Sci. 103, 168–178. doi:10.1016/j.ijrmms.2018.01.041
Xiang, T., Li, Y. X., Gao, X. Y., and Shi, D. M. (2022). Multi-field Coupling Analysis of Frame-Anchor Supporting Slope Displacement in Seasonal Frozen Zone. J. China Coal Soc. 1, 11. doi:10.13225/j.cnki.jccs.2020.1681
Xu, C., Li, Z., Wang, S., Wang, S., Fu, L., and Tang, C. (2018). Pullout Performances of Grouted Rockbolt Systems with Bond Defects. Rock Mech. Rock Eng. 51 (3), 861–871. doi:10.1007/s00603-017-1373-1
Xu, C., Xu, X., Yao, X., and Dai, F. (2014). Three (Nearly) Complete Inventories of Landslides Triggered by the May 12, 2008 Wenchuan Mw 7.9 Earthquake of China and Their Spatial Distribution Statistical Analysis. Landslides 11 (3), 441–461. doi:10.1007/s10346-013-0404-6
Xu, D. S., and Yin, J. H. (2016). Analysis of Excavation Induced Stress Distributions of GFRP Anchors in a Soil Slope Using Distributed Fiber Optic Sensors. Eng. Geol. 213, 55–63. doi:10.1016/j.enggeo.2016.08.011
Xu, L. X., and Yang, Q. G. (1997). Study on High Excavated Rock Slope at TGP Shiplocks. Yangtze River 10, 29–31+55. doi:10.16232/j.cnki.1001-4179.1997.10.011
Xu, N. F. (2000). Excavation and Reinforcement Design for High Slope of TGP Permanent Shiplock. Chin. J. Rock Mech. Eng. 19 (S1), 1071–1076. doi:10.3321/j.issn:1000-6915.2000.z1.055
Yang, G., Zhong, Z., Zhang, Y., and Fu, X. (2015). Optimal Design of Anchor Cables for Slope Reinforcement Based on Stress and Displacement Fields. J. Rock Mech. Geotechnical Eng. 7 (4), 411–420. doi:10.1016/j.jrmge.2015.04.004
Yang, W. D., Luo, G. Y., Bo, C. J., Wang, L., Lü, X. X., Wang, Y. N., et al. (2020). Mechanical Properties and Reinforcement Effect of Jointed Rock Mass with Pre-stressed Bolt. J. Cent. South Univ. 27 (12), 3513–3530. doi:10.1007/s11771-020-4469-9
Yeung, A. T., Cheng, Y. M., Tham, L. G., Au, A. S., So, S. T., and Choi, Y. K. (2007). Field Evaluation of a Glass-Fiber Soil Reinforcement System. J. Perform. Constr. Facil. 21 (1), 26–34. doi:10.1061/(asce)0887-3828(2007)21:1(26)
Yokota, Y., Zhao, Z., Nie, W., Date, K., Iwano, K., and Okada, Y. (2019a). Experimental and Numerical Study on the Interface Behaviour between the Rock Bolt and Bond Material. Rock Mech. Rock Eng. 52 (3), 869–879. doi:10.1007/s00603-018-1629-4
Yokota, Y., Zhao, Z., Shang, J., Nie, W., Date, K., Iwano, K., et al. (2019b). Effect of Bolt Configuration on the Interface Behaviour between a Rock Bolt and Bond Material: A Comprehensive DDA Investigation. Comput. Geotechnics 105, 116–128. doi:10.1016/j.compgeo.2018.09.017
Yu, B., Till, V., and Thomas, K. (2007). Modeling of Thermo-Physical Properties for FRP Composites under Elevated and High Temperature. Compos. Sci. Technol. 67 (15), 3098–3109. doi:10.1016/j.compscitech.2007.04.019
Zhang, B. R. (1995). Study on Strengthening Technique for Toppling Rock Slope. Chin. Civ. Eng. J. 5, 70–75.
Zhang, J. L., Xu, W. Y., Xu, F., and Cai, D. W. (2009). Study on Prestress Loss Law of Anchor Cables in Deep Unloading Deformed Ripped Blocks. Chin. J. Rock Mech. Eng. 28 (Suppl. 2), 3965–3970. doi:10.3321/j.issn:1000-6915.2009.z2.098
Zhang, S. B., Wang, C. S., Wang, G., Wu, X. Z., Zheng, X., He, P., et al. (2022). Experimental Study on the Shear Behaviors of Bolted Rock Joints Reinforced with BFRP Bars. Chin. J. Rock Mech. Eng. 41 (04), 712–724. doi:10.13722/j.cnki.jrme.2021.0894
Zhang, S. P., and Chen, C. (2003). Study on Pull-Out Tests of Pressure Type Anchor in Rock. J. Chongqing Jianzhu Univ. 3, 22–25+90. doi:10.3969/j.issn.1674-4764.2003.03.005
Zhang, Y. J., and Zhu, W. S. (1997). Plane Finite Element Calculation of Anchorage Scheme for High Slope of Shiplock of TGP. Chin. J. Geotech. Eng. 1, 72–76.
Zhou, D. P., Liu, S. X., and Liu, H. (2013). Some Problems to Be Considered in Design for Compression Dispersion-type Anchor Cables. Chin. J. Rock Mech. Eng. 32 (08), 1513–1519. doi:10.3969/j.issn.1000-6915.2013.08.001
Zhu, A. L., Zhang, Y., Dai, M. L., and Xu, J. Q. (2017). Reinforcement Mechanism of Slopes with Yielding Anchor Cables Based on Numerical Simulation of FLAC3D. Chin. J. Geotech. Eng. 39 (4), 713–719. doi:10.11779/CJGE201704017
Zhu, W. S., and Cheng, F. (2000). Constitutive Model of Energy Dissipation and its Application to Stability Analysis of Ship-Lock Slope in Three Gorges Project. Chin. J. Rock Mech. Eng. 19 (3), 261–264. doi:10.3321/j.issn:1000-6915.2000.03.001
Zhu, W. S., and Zhang, Y. J. (1996). Primary Research on Stability Analysis and Reinforcement Schemes for Jointed Rock Mass of High Slope of Three Gorges Flight Lock. Chin. J. Rock Mech. Eng. 4, 2–8.
Zima, B., and Rucka, M. (2017). Non-destructive Inspection of Ground Anchors Using Guided Wave Propagation. Int. J. Rock Mech. Min. Sci. 94, 90–102. doi:10.1016/j.ijrmms.2017.03.005
Keywords: slope engineering, anchor bolt, energy-absorbing anchor, nonmetal bolt, carbon neutrality, ecological slope protection
Citation: Du Y, Li H, Chicas SD and Huo L (2022) Progress and Perspectives of Geotechnical Anchor Bolts on Slope Engineering in China. Front. Environ. Sci. 10:928064. doi: 10.3389/fenvs.2022.928064
Received: 25 April 2022; Accepted: 19 May 2022;
Published: 05 July 2022.
Edited by:
Yifei Sun, Hohai University, ChinaReviewed by:
Shao-Heng He, Zhejiang University, ChinaCopyright © 2022 Du, Li, Chicas and Huo. This is an open-access article distributed under the terms of the Creative Commons Attribution License (CC BY). The use, distribution or reproduction in other forums is permitted, provided the original author(s) and the copyright owner(s) are credited and that the original publication in this journal is cited, in accordance with accepted academic practice. No use, distribution or reproduction is permitted which does not comply with these terms.
*Correspondence: Heng Li, bGloZW5nXzBfMUAxNjMuY29t
Disclaimer: All claims expressed in this article are solely those of the authors and do not necessarily represent those of their affiliated organizations, or those of the publisher, the editors and the reviewers. Any product that may be evaluated in this article or claim that may be made by its manufacturer is not guaranteed or endorsed by the publisher.
Research integrity at Frontiers
Learn more about the work of our research integrity team to safeguard the quality of each article we publish.