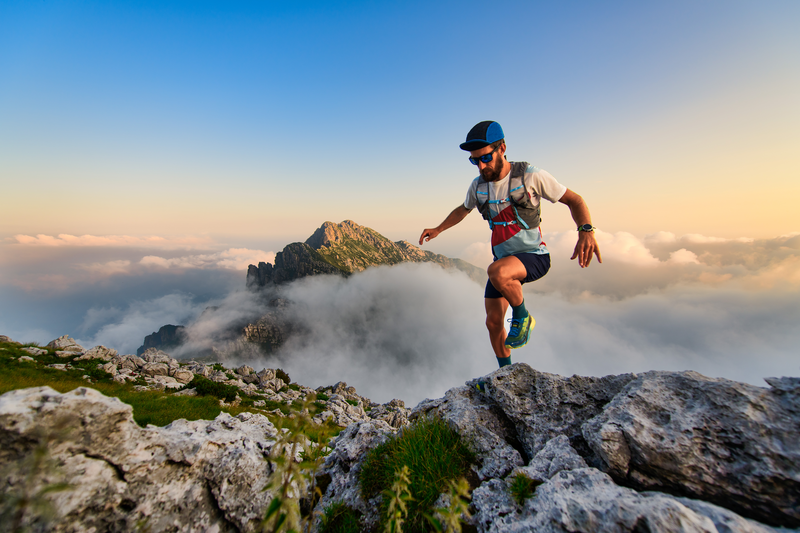
95% of researchers rate our articles as excellent or good
Learn more about the work of our research integrity team to safeguard the quality of each article we publish.
Find out more
ORIGINAL RESEARCH article
Front. Environ. Sci. , 30 June 2022
Sec. Water and Wastewater Management
Volume 10 - 2022 | https://doi.org/10.3389/fenvs.2022.926937
Landscape patterns significantly affect urban runoff pollution, and a reasonable arrangement of pervious patches in urban catchments is critical to control urban non-point-source pollution. Low impact development (LID) can be recognized as a pervious surface type, and many previous studies have analyzed the LID performance at a system scale. However, the influence of the LID spatial distribution on runoff pollutant transport at a catchment scale remains unclear. This study analyzed the influence of pervious/impervious patterns on runoff pollution within two urban catchments constructed with LID. It was found that the runoff pollution was influenced by the area ratio, circumference, shape, and pervious patch connectivity of pervious surfaces (i.e., LID). Catchments with high perviousness ratios are more efficient in reducing the runoff volume and suspended solids, and LID should focus on removing runoff pollutants at catchments with high imperviousness ratios. Six typical landscape metrics that represented the fragmentation, complexity and vergence of pervious surfaces were selected to investigate the influence of pervious/impervious patterns on runoff pollution. The results show that larger fragmentation, smaller complexity and smaller vergence of pervious patches result in larger runoff volumes and more pollutants. Therefore, it is recommended that large pervious areas should be retained and close to one another to enhance the retention capacity of LID, and the outline of pervious patches should be long and irregular to reduce pollution confluence. The results of this study are helpful in arranging LID structures for efficient runoff regulation and pollution control at an urban catchment scale.
Urban non-point source pollution has become a main reason for urban water pollution (Sansalone and Kim, 2008; Goonetilleke et al., 2014; Chang et al., 2022). Rapid urbanization extends impervious surfaces such as roofs and roads (i.e., sealed soil) in urban areas (Morabito et al., 2021). This leads to higher runoff volume and peak runoff, which increases the urban flood risk and water quality degradation (Deng, 2020). Source control is an efficient solution to reduce urban runoff and pollutants (Ma et al., 2019; Choi et al., 2020). Low impact development (LID) has been recognized as an effective source control technique to manage urban non-point source pollution at a micro-catchment scale (Jia et al., 2017). Therefore, reasonable arrangement of LID spatial distribution in urban catchments is critical to control urban non-point source pollution.
LID disconnects hydrological connections by dividing a conventional catchment into various sub-catchments. Previous studies have identified that hydrological responses and stormwater quality are scale dependent. Structure scale mainly focuses on comparing the input and outflow runoff quantity and quality to assess individual LID performance for optimized parameter design (Hunt et al., 2008; Chen et al., 2018; Bortolini and Zanin, 2019; Zaqout and Andradóttir, 2021; Zhang et al., 2021). Catchment scale normally aims at runoff control in micro urban catchments (<0.5 km2) (Hou et al., 2019; Randall et al., 2019; Yao et al., 2020). Barely focus on individual LID structure performance is inadequate to understand stormwater runoff regulation and pollution control within urban catchments. In order to investigate the role of LID spatial patterns on runoff pollution control, the LID performance should be assessed within urban catchments instead of an individual structure. This assessment requires analyzing runoff pollution during the entire pollutant transport process in LID constructed catchments.
The alteration of pervious surfaces to impervious surfaces is mainly responsible for urban flood and runoff pollution (Silva and Silva, 2020). The pervious/impervious ratio is a primary factor that affects the quantity and quality of surface runoff (Liao et al., 2021). Many researchers have investigated the threshold of the pervious/impervious ratio of urban catchments or watersheds for runoff pollution control (Leach and Coulibaly, 2020; Sohn et al., 2020; Wang et al., 2020). However, studies on the influences of pervious/impervious patterns on pollutant transport remain lacking. Since LID is also a type of pervious surface influencing pollutant transport, how to arrange LID structures within urban catchments for efficient runoff pollution control is unknown. Therefore, it is important to analyze the influence of pervious/impervious patterns on the runoff quantity and quality during different transport processes.
Given the knowledge gap and importance, the current study analyzed the influence of pervious (i.e., LID) and impervious patterns on runoff transport within two LID constructed catchments. The main objectives are as follows: 1) to investigate the influence of the pervious/impervious ratio on the quantity and quality of urban runoff; 2) to evaluate the influence of pervious/impervious patterns on runoff reduction and pollutant removal; 3) to recommend reasonable LID spatial arrangements for urban non-point-source pollution control. The results will be helpful in arranging LID structures for efficient runoff pollution control within urban catchments.
The study was undertaken in Longgang District in Shenzhen, Guangdong, China. Shenzhen is located in the subtropical marine climate zone with an average annual rainfall of 1933.3 mm. The rainy season in Shenzhen is from April to September, which accounts for 80% of the rainfall depth during the entire year. To investigate the influences of pervious/impervious patterns on pollution transport, the site selection considered two criteria: to select two catchments constructed with different LID intensities and different LID spatial distributions. The urban catchments Zhongxin and Shoudai with different pervious/impervious patterns were selected as the study catchments. Except for the pervious/impervious pattern, the two catchments are identical in other conditions such as land use, climate condition and population density. The two catchments were constructed with various LIDs, such as high-level particles, rain gardens, porous pavement and infiltration channels. Roof runoff pollution load is pre-reduced by high-level particles and rain barrels, while road runoff initially flows through LID and then flows into stormwater pipelines. These LID structures focus on the infiltration and retention of runoff pollution; therefore, they are assumed to be pervious areas in this study. The area of Zhongxin was 11,142 m2, and the impervious area accounted for 61.1%. The main impervious surface types in the catchment were road and roof surfaces, which accounted for 48.38% and 12.69% of the Zhongxin catchment, respectively. The area of Shoudai was 55,676 m2, and the impervious area accounted for 73.6%, including 39.05% road surfaces and 34.51% roof surfaces. Hence, Zhongxin was identified as the catchment of low imperviousness, while Shoudai was identified as the catchment of high imperviousness. Both catchments were constructed with separate sewer systems. According to the drainage, a parcel was selected within each catchment. The areas of parcels in Zhongxin and Shoudai were 3489.74 m2 and 5708.4 m2, respectively. Three study sites, including roof sites, road sites and sewer sites, were selected in each parcel for solid and runoff sample collection. The roof site was selected at the outlet of a downpipe from a building within the study parcel and used to collect roof runoff. The road site was selected at a catch basin, which was placed along a road to collect road runoff. The sewer site was the drainage outlet of the study parcel and used to collect sewer flow. It is noteworthy that the two paired study catchments were considered typical to investigate the role of various LID spatial patterns on urban runoff pollution control. The distribution of the study sites and catchments is shown in Figure 1.
Sample collection was undertaken at the study sites. Solids were collected on the roof and road surfaces within the study parcel both prior to and after each rainfall event. The sampling plot was a 1 m × 3 m square, and solids were collected using a vacuum cleaner. Runoffs, including roof runoff, road runoff and sewer runoff, were collected during rainfall events. Each runoff sample was collected every 5 min during the initial 15 min after runoff generation, every 10 min during the subsequent 30 min, every 20 min during the subsequent 60 min and every 30 min during the subsequent 90 min until there was no more runoff. The time and volume of every sample were recorded to calculate the runoff volume. The maximum volume of each runoff sample was 500 ml. If there were more than 500 ml samples, the excessive samples were discarded, and the time duration to collect 500 ml samples was recorded. Otherwise, the sample volume within the specific time interval was recorded. The sewer runoff volume was monitored using a Starflow 6526 flowmeter pre-installed at the sewer site. Runoff samples were stored in polyethylene bottles at 4°C until analysis. The rainfall depth was monitored using a HOBO RG3-M tilting rain gauge, and the rainfall characteristics are shown in Table 1. A total of 40 solids samples and 209 runoff samples were collected, which was able to provide robust data foundation for analysis.
The solids were initially dried using a freeze dryer due to their high moisture. Then, the dried solids were divided into nine particle size fractions: <44 μm, 44–62 μm, 62–105 μm, 105–149 μm, 149–250 μm, 250–450 μm, 450–1,000 μm, 1,000–2000 µm and >1,000 µm using a Retsch AS200 jet sieving machine. The mass of each particle size class was weighed for the particle size distribution analysis. Runoff samples were analyzed for suspended solids (SS), particle size distribution (PSD), chemical oxygen demand (COD), total phosphorous and nitrogen (TP and TN), total dissolved phosphorous and nitrogen (TDP and TDN), particulate phosphorous and nitrogen (PP and PN), ammonia nitrogen (NH4+-N), and five heavy metals (Cd, Cr, Cu, Pb, and Zn). An aliquot of samples was filtered through 0.45-µm precombusted and preweighed glass fiber membranes (Whatman, GF/F, 47 mm). Particles on the membranes were dried and weighed, and the difference between the membranes was determined as the weight of SS. PSD was tested using a Malvern Mastersizer 2000 instrument. COD was tested using a HACH water quality analyzer. TP and TDP were analyzed according to ammonium molybdate spectrophotometric method GB 11893-89 (Prcmep, 1990). The samples were digested by K2S2O8, and phosphorous concentrations were determined using a UV-6100 spectrometer. PP was determined as the difference between TP and TDP. TN and TDN were analyzed according to the alkaline potassium persulfate digestion-UV spectrophotometric method GB 11894-89 (Prcmep, 1989). An aliquot of filtrate was digested by K2S2O8, and the nitrogen concentrations were determined using a UV-6100 spectrometer. PN was determined as the difference between TN and TDN. NH4+-N was analyzed according to Nessler’s reagent spectrophotometric method HJ 535-2009 (Prcmep, 2009). Nessler’s reagent was added to an aliquot of filtrate to digest the NH4+-N, and NH4+-N concentrations were determined using a visible spectrophotometer. Heavy metals in runoff samples were digested using nitric acid according to the microwave-assisted acid digestion method HJ 678-2013 (Prcmep, 2013). An aliquot (25 ml) of a well-mixed runoff sample was placed in a microwave digestion vessel, and 5 ml nitric acid and 1 ml hydrogen peroxide were added. The samples were digested at 180 °C for 15 min, removed from the microwave and cooled to room temperature. The digested samples were transferred to a 50-ml centrifuge tube. Vessels were washed with deionized water twice, and the deionized water was also transferred to the centrifuge tube. Then, the sample was diluted to 50 ml using deionized water. Finally, the digested samples were filtered through a 0.45-µm cellulose acetate membrane to remove any particles, and the concentrations of heavy metals in the filtrate were tested using PerkinElmer NexION 300X inductively coupled plasma mass spectrometry (ICP-MS) according to the US EPA method 200.8 (Usepa, 1994).
This study used landscape metrics to quantify the pervious/impervious pattern of the study catchments. The landscape metrics were calculated according to the following steps. Step 1: The catchment was divided into pervious surface areas, including grassland and bushes, and impervious surface areas, including roofs, roads and pavements. Step 2: Subcatchment delineation was conducted based on the location of catch basins and the microtopography using ArcMap and ArcSWAT according to the Thiessen polygon method and manually adjusted considering local buildings. Step 3: The subcatchments were divided into pervious and impervious surfaces, put into the same vector database and transferred to 1 m × 1 m raster images. Step 4: Images were imported into Fragstats 4.2, and relevant parameters were selected. Step 5: Landscape metrics were identified according to the patch class level.
A Pearson correlation analysis was performed to determine the relationships between runoff volume and pollutants load and landscape metrics. Pearson correlation analysis has been widely adopted by numerous studies to analyze the correlation between landscape patterns and water quality in watersheds (Shehab et al., 2021). The correlation coefficient was used to determine the degree of correlation: correlation coefficients of 0.8–1.0 represent strong correlations; 0.5–0.8 represent moderate correlations; 0.3–0.5 represent low correlations. Pearson correlation analysis was conducted using Rstudio 3.5.1. It is noteworthy that other statistical methods, such as Spearman correlation analysis, Principle Component Analysis and Kendall correlation analysis, are capable to analyze the relationship between landscape metrics and water quality and hydrological responses, and this study selected one of the suitable methods for correlation analysis.
The runoff volumes from the two catchments were compared. Since LID was constructed in both catchments, the surface runoff was treated through LID prior to discharge from the sewer. Hence, the surface runoff volume was reduced by LID. Figure 2 presents the runoff reduction in the two studied catchments. The overall reduction rate of runoff from the low-imperviousness catchment (mean 69.43%) was higher than that from the high-imperviousness catchment (mean 56.49%). This result suggests that relatively lower imperviousness is more efficient for runoff volume control.
FIGURE 2. Influence of pervious/impervious patterns and rainfall characteristics on the runoff reduction rate. [Note: Fw(%) is the percentage of runoff reduction from surface runoff to sewer runoff (%).].
With identical imperviousness ratios, the runoff reduction rate during various rainfall events was compared (Figure 2) to investigate the influence of rainfall characteristics on the volume control through LID. The results show that the runoff reduction rate during smaller rainfall depths was generally higher than higher rainfall depths. For example, although the rainfall events on May 23rd, Apr 18th, and Apr 30th had lower rainfall depths in the low-imperviousness catchment, the runoff reduction rates during the three rainfall events were higher. In contrast, the rainfall events on Apr 27th had the highest rainfall depth, while the runoff reduction rate during this rainfall event was the lowest in the low-imperviousness catchment. Similar to the low-imperviousness catchment, the runoff reduction rates decreased with increasing rainfall depths. These results suggest that LID is more efficient in reducing the runoff volume during smaller rainfall events. This can be attributed to the fact that soil saturation water capacity increased with during a rainfall event so that larger percentage of runoff volume can be retained by LID through smaller rainfall events than higher rainfall events.
Solids are the main carrier of chemical pollutants in urban runoff. Hence, understanding the concentration and composition of suspended solids in runoff is critical for LID spatial optimization. Figure 3 compares suspended solid concentration, load and particle size distribution in runoff at catchments with different imperviousness ratios. The mean concentrations of suspended solids in road and roof runoff from the low-imperviousness catchment were 83.53 mg/L and 11.81 mg/L, and the concentrations of suspended solids in road and roof runoff from the high-imperviousness catchment were 117.62 mg/L and 19.45 mg/L (Figure 3A). Moreover, road and roof runoff from the low-imperviousness catchment contributed 95.17% and 4.83% solids respectively, and road and roof runoff from the high-imperviousness catchment contributed 83.07% and 16.93% solids respectively (Figure 3B). These results indicate that road surfaces are the main underlying surfaces in runoff treatment; therefore, LID should focus on runoff treatment from road surfaces at catchments where limited space is suitable for LID construction. In addition, the results show that suspended solids pollution in runoff from a high-imperviousness catchment was more serious than that from a low-imperviousness catchment. Therefore, catchments with higher imperviousness can generate more suspended solids in runoff. These outcomes suggest that LID should be enhanced to remove solids in runoff from road surfaces in high-imperviousness catchments.
FIGURE 3. (A) Concentration of suspended solids in road and roof runoff; (B) Mass percentage contributed by road and roof runoff; (C) Particle size distribution of suspended solids in road and roof runoff; (D) Load of suspended solids in surface and sewer runoff.
The particle size is an important factor that affects pollutant transport in urban runoff; therefore, the particle size distribution in runoff during different transport processes was analyzed. Figure 3C presents the particle size distribution in road and roof runoff from low- and high- imperviousness catchments. Results show that fine particles accounted for a higher mass percentage in the low-imperviousness catchment, while coarse particles in runoff were more abundant in the high-imperviousness catchment. This outcome suggests that LID should focus on removing fine solids in runoff from low-imperviousness catchments and coarse solids in runoff from high-imperviousness catchments.
Solids load in runoff discharged from the two catchments were also compared to investigate the LID performance with different pervious/impervious patterns. Figure 3D depicts the suspended solids load in surface runoff (i.e., before LID treatment) and sewer runoff (i.e., after LID treatment). In Figure 3D, the average reduction rate of suspended solids through LID at low- and high-imperviousness catchments was 76.04% and 59.85%, respectively, which indicates that suspended solid reduction at low-imperviousness catchment is relatively higher than that at high-imperviousness catchment. The reason is that high imperviousness leads to high intensity of roads and buildings, and space is very limited for LID construction. Although the catchment boundary of the LID structure is large, the catchment area is small. Consequently, a large amount of runoff directly flows into the drainage instead of being treated by LID. In other words, high imperviousness leads to decentralized perviousness (i.e., LID structures), which constrains the pollution treatment efficiency by LID. Hence, it is recommended that localized and large pervious areas are more efficient in removing suspended solids in runoff.
Chemical pollutant concentrations in road and roof runoff are compared between low- and high-imperviousness catchments. Figure 4B shows that the road and roof runoff contributed 98.09% and 1.91% TP, 79.33%, and 20.67% TN, 89.98%, and 10.02% COD in the low-imperviousness catchment and 95.04% and 4.96% TP, 56.80% and 43.20% TN, 74.49% and 25.51% COD in the high-imperviousness catchment, respectively. These results suggest that TP, TN, and COD are mainly contributed by road runoff. In addition, the concentrations of TP, TN, and COD in runoff from the high-imperviousness catchment were higher than those from the low-imperviousness catchment (Figure 4A). Therefore, it is recommended that the removal of TP, TN, and COD in runoff should focus on road runoff pollution, especially in catchments with high imperviousness.
In terms of heavy metals, the road and roof runoff contributed 81.74% and 18.26% Cd, 63.54% and 36.46% Cr, 76.44% and 23.56% Cu, 77.13% and 22.87% Pb, and 79.30% and 20.70% Zn in the low-imperviousness catchment. In contrast, the road and roof runoff contributed 49.30% and 50.70% Cd, 82.47% and 17.53% Cr, 49.59% and 50.41% Cu, 93.92% and 6.08% Pb, and 42.27% and 57.73% Zn in the high-imperviousness catchment (Figure 4B). Overall, road runoff was also a major contributor to heavy metals in the low-imperviousness catchment, but the contribution percentage to heavy metals by roof runoff was obviously higher than that of TP, TN, and COD. For high-imperviousness catchments, the contribution percentage to heavy metals by roof runoff was much higher, even greater than the road runoff. These results suggest that the contribution of roof runoff to heavy metals cannot be underestimated, and heavy metal pollution control should also consider roof runoff pollution reduction.
Landscape metrics are widely adopted to quantify landscape characteristics within urban areas. Six landscape metrics were selected to represent pervious surface characteristics of the catchments: patch density (PD), largest patch index (LPI), mean shape index (MSI), landscape shape index (LSI), cohesion index (COHESION), and splitting index (SPLIT). The reason to select these landscape metrics is discussed in Section 4.1. PD is the number of pervious patches per unit area. LPI is the area percentage of the largest pervious patch within a catchment. PD and LPI represent the perviousness fragmentation. MSI is the complexity level of an individual pervious patch outline. LSI is the complexity level of the pervious patch shape. MSI and LSI represent the perviousness complexity. COHESION is the physical connection between pervious patches. SPLIT is the distribution of the pervious patch in a catchment. COHESION and SPLIT represent the perviousness vergence. The landscape metrics of the study catchments are shown in Supplementary Figures S1–S6.
The influence of landscape patterns on urban non-point-source pollution was analyzed through Pearson correlation analysis. Table 2 shows the results of the Pearson correction between runoff volume and pollution load and the selected landscape metrics (i.e., PD, LPI, MSI, LSI, COHESION, and SPLIT). Pollutants include dissolved pollutants (NH4+-N, TN, and COD) and particulate pollutants (TP and SS). Table 2 shows that PD was positively correlated with the runoff volume and dissolved pollutants, whereas LPI was negatively correlated with these parameters. This result suggests that increasing fragmentation (i.e., higher PD and lower LPI) of large pervious patches results in larger runoff volume and more dissolved pollutants. This is mainly because small and decentralized pervious patches have more difficulty retaining runoff than large and centralized pervious patches. Therefore, it is recommended that perviousness fragmentation should be reduced, and large pervious areas should be retained for efficient runoff regulation and pollution control.
Regarding complexity, LSI was positively correlated with the runoff volume and particulate pollutants, while MSI was negatively correlated with the runoff volume and particulate pollutants. The reason is that lower LSI corresponds to a more regular outline of the pervious surfaces. In this case, pervious patches show an aggregation distribution, which increases the runoff retention time through the pervious surfaces and reduces the runoff volume discharged from the catchment. In contrast, a larger MSI implies that the outline of the pervious patch is more irregular, which results in less runoff volume and particulate pollutants. The main reason is that there is greater runoff loss when runoff flows along the irregular patch outline; hence, less runoff is discharged from the catchment. Similarly, irregular outlines can strongly reduce the mobility of particles during runoff transport, and less particulate pollutant load is discharged. Therefore, it is recommended that the outline of pervious patches can be long and irregular to enlarge runoff transport pathways.
SPLIT was positively correlated with the runoff volume and dissolved pollutants, whereas COHENSION was negatively correlated with runoff volume and dissolved pollutants, which indicates that closer pervious patches correspond to lower runoff volume and less dissolved pollutants. The main reason is that when SPLIT is high and COHENSION is low, the physical connection of pervious patches is not sufficiently strong, and individual patches cannot cooperate with each other to retain runoff, which leads to higher runoff volume and pollutant concentration. In contrast, when SPLIT is low and COHENSION is high, pervious patches are close to one another, and adjacent patches can supplement to patches that are cannot retain sufficient runoff, which reduces the runoff volume and amount of pollutants. Therefore, it is recommended that pervious patches should be near one another to enhance the pollution retention capacity.
Since PD, LSI, and SPLIT at the high-imperviousness catchment (Shoudai) were higher, while LPI, MSI, and COHESION at Shoudai were lower, Shoudai had higher runoff volume and pollutant concentration. In other words, the high-imperviousness catchment reduced the runoff and pollutant load less. These results remain consistent with the results from Figures 2, 3. Therefore, lower PD and higher LPI, higher MSI, and lower LSI, and lower SPLIT and higher COHENSION (i.e., smaller fragmentation, larger complexity, and larger vergence) of perviousness can result in less runoff volume and pollutants in urban catchments. On one hand, large and centralized pervious surfaces can reduce runoff through strong infiltration and storage. On the other hand, circuitous and extended patch side lengths lead to weak mobility capacity of runoff and pollutants, which increases the pollutant retention time and reduces the runoff volume and pollutant load. In contrast, regular and decentralized pervious patches can attenuate runoff pollution control due to their weak capacity to retain runoff and pollutants. Hence, urban planning should consider increasing irregular and large pervious patches in catchments to enhance the runoff regulation and pollution control.
Landscape patterns have been widely recognized as an important parameter that affects the urban runoff pollution (Bin et al., 2018; Shehab et al., 2021). Many studies have investigated the influence of the perviousness/imperviousness ratio on the runoff volume and pollutant concentration from urban catchments and watersheds (Salerno et al., 2018; Hamilton et al., 2021; Liu et al., 2021). However, how landscape patterns impact pollutant transport processes remains unknown. This constrains the appropriate arrangement of LID, which can be considered a pervious surface type, within urban catchments to control runoff and pollutants during pollutant transport. Given this knowledge gap, this study analyzed the relationship between landscape metrics and runoff pollutants, and appropriate landscape metrics were selected to characterize urban runoff pollution in catchments. All landscape metrics were initially calculated through Fragstats, and an autocorrelation analysis was applied to these metrics using Pearson correlation analysis to reduce the repeatability of the metrics (Supplementary Figure S7). Subsequently, the relationship among the selected landscape metrics and runoff volume and pollutant load was also analyzed using Pearson analysis (Supplementary Figures S8, S9). The landscape metrics strongly related to the runoff volume and pollutant load were finally selected to characterize the runoff pollution. According to the results obtained from Pearson analysis, this study selected six landscape metrics that were classified into three groups: 1) PD and LPI represent the fragmentation of the impervious surfaces; 2) MSI and LSI represent the complexity of the impervious surfaces; 3) SPLIT and COHENSION represent the vergence of the impervious surfaces. These landscape metrics are independent of one another and strongly related to the runoff volume and pollutant load. Therefore, future studies can focus on these landscape metrics to analyze the influence of pervious/impervious patterns on runoff pollution at the catchment scale.
Recently, LID construction has increased in response to serious urban non-point-source pollution (Tang et al., 2021). LID focuses on runoff reduction and pollutant removal during small rainfall events through decentralized and microscale source control techniques (Liang et al., 2019; Bae and Lee, 2020). Reasonable arrangement of LID at the catchment scale demands full understanding of the pervious/impervious pattern within urban catchments. The outcomes of this study indicate that the LID performance is influenced by pervious/impervious landscape patterns such as the perviousness ratio, pervious surface area (i.e., fragmentation), pervious patch circumference and shape (i.e., complexity), and connectivity among pervious patches (i.e., vergence). The pervious surface area and connectivity among individual pervious patches determine the retention capacity of pervious surfaces. Larger areas and stronger connectivity of pervious patches can retain more runoff and pollutants. Therefore, regarding catchments with a high imperviousness ratio, the LID construction space is so limited that enlarging the pervious surface area and enhancing the connectivity should be considered. For example, small LID structures such as rain gardens and high-level parterres can connect to the existing green land to expand the pervious surface area and enhance the connectivity among individual pervious patches. In this context, overflow discharged from LID can be retained by green land.
The pervious patch circumference and shape determine pollution confluence. Longer circumferences and more complex shapes lead to less confluence of runoff and pollutants. Therefore, in terms of catchments with low imperviousness ratios, enhancing the complexity of pervious patches should also be considered during LID construction. For example, the complex outline and irregular shape of LIDs can be designed to extend the pollutant transport pathway to reduce the runoff volume and pollutant load. Large and irregular pervious patches should be near one another during urban planning to decrease perviousness fragmentation, increase perviousness complexity and increase perviousness vergence. In conclusion, taking imperviousness ratio and pervious/impervious landscape patterns into LID spatial arrangements provides a new idea for LID construction at the urban catchment scale.
This study investigated the influence of pervious/impervious patterns on urban runoff pollution at the catchment scale. Catchments with low imperviousness ratios are more efficiently in reducing the runoff volume and suspended solids than catchments with high imperviousness ratios. Moreover, the concentrations of runoff pollutants were higher in catchments with high imperviousness ratios than in catchments with low imperviousness ratios; therefore, LID should focus on removing runoff pollutants in catchments with high imperviousness ratios. Landscape pattern analysis selected six typical landscape metrics (PD, LPI, LSI, MSI, COHENSION, and SPLIT) to characterize the influence of pervious/impervious patterns on runoff pollution. Results found that larger fragmentation, smaller complexity and smaller vergence of pervious patches resulted in larger runoff volumes and more pollutants. Therefore, perviousness fragmentation should be reduced and large LID should be retained for efficient runoff regulation and pollution control. Besides, the outline of LID should be long and irregular to enlarge runoff transport pathways. Additionally, LID should be near to the existing green land to enhance the pervious patch connectivity and pollution retention capacity.
The original contributions presented in the study are included in the article/Supplementary Material, further inquiries can be directed to the corresponding author.
YM: Methodology, Data analysis, Writing- Original draft preparation HZ: Conceptualization, Methodology, Supervision, Writing- Reviewing and Editing.
This research was supported by the National Natural Science Foundation of China (No. 42077377) and the Major Science and Technology Program for Water Pollution Control and Treatment (No. 2015ZX07206-006-02).
The authors declare that the research was conducted in the absence of any commercial or financial relationships that could be construed as a potential conflict of interest.
All claims expressed in this article are solely those of the authors and do not necessarily represent those of their affiliated organizations, or those of the publisher, the editors and the reviewers. Any product that may be evaluated in this article, or claim that may be made by its manufacturer, is not guaranteed or endorsed by the publisher.
The Supplementary Material for this article can be found online at: https://www.frontiersin.org/articles/10.3389/fenvs.2022.926937/full#supplementary-material
Bae, C., and Lee, D. K. (2020). Effects of Low-Impact Development Practices for Flood Events at the Catchment Scale in a Highly Developed Urban Area. Int. J. Disaster Risk Reduct. 44, 101412. doi:10.1016/j.ijdrr.2019.101412
Bin, L., Xu, K., Xu, X., Lian, J., and Ma, C. (2018). Development of a Landscape Indicator to Evaluate the Effect of Landscape Pattern on Surface Runoff in the Haihe River Basin. J. Hydrology 566, 546–557. doi:10.1016/j.jhydrol.2018.09.045
Bortolini, L., and Zanin, G. (2019). Reprint of : Hydrological Behaviour of Rain Gardens and Plant Suitability: A Study in the Veneto Plain (North-eastern Italy) Conditions. Urban For. Urban Green. 37, 74–86. doi:10.1016/j.ufug.2018.07.003
Chang, X., Jia, Z., Feng, J., Duan, T., and Li, Y.-X. (2022). Refining the Diagnostics of Non-point Source Metals Pollution to Urban Lakes Based on Interaction Normalized PMF Coupled with Bayesian Network. Environ. Pollut. 304, 119194. doi:10.1016/j.envpol.2022.119194
Chen, J., Yin, X., Wang, H., and Ding, Y. (2018). Evaluation of Durability and Functional Performance of Porous Polyurethane Mixture in Porous Pavement. J. Clean. Prod. 188, 12–19. doi:10.1016/j.jclepro.2018.03.297
Choi, J. Y., Jeong, H., Choi, K.-Y., Hong, G. H., Yang, D. B., Kim, K., et al. (2020). Source Identification and Implications of Heavy Metals in Urban Roads for the Coastal Pollution in a Beach Town, Busan, Korea. Mar. Pollut. Bull. 161, 111724. doi:10.1016/j.marpolbul.2020.111724
Deng, Y. (2020). Low-cost Adsorbents for Urban Stormwater Pollution Control. Front. Environ. Sci. Eng. 14 (5), 83. doi:10.1007/s11783-020-1262-9
Goonetilleke, A., Yigitcanlar, T., Ayoko, G. A., and Egodawatta, P. (2014). Sustainable Urban Water Environment: Climate, Pollution and Adaptation. Cheltenham UK & Northampton, MA, USA: Edward Elgar Publishing.
Hamilton, B., Coops, N. C., and Lokman, K. (2021). Time Series Monitoring of Impervious Surfaces and Runoff Impacts in Metro Vancouver. Sci. Total Environ. 760, 143873. doi:10.1016/j.scitotenv.2020.143873
Hou, J., Han, H., Qi, W., Guo, K., Li, Z., and Hinkelmann, R. (2019). Experimental Investigation for Impacts of Rain Storms and Terrain Slopes on Low Impact Development Effect in an Idealized Urban Catchment. J. Hydrology 579, 124176. doi:10.1016/j.jhydrol.2019.124176
Hunt, W. F., Smith, J. T., Jadlocki, S. J., Hathaway, J. M., and Eubanks, P. R. (2008). Pollutant Removal and Peak Flow Mitigation by a Bioretention Cell in Urban Charlotte, N.C. J. Environ. Eng. 134 (5), 403–408. doi:10.1061/(asce)0733-9372(2008)134:5(403)
Jia, H., Yu, S. L., and Qin, H. (2017). Low Impact Development and Sponge City Construction for Urban Stormwater Management. Front. Environ. Sci. Eng. 11 (4), 20. doi:10.1007/s11783-017-0989-4
Leach, J. M., and Coulibaly, P. (2020). Soil Moisture Assimilation in Urban Watersheds: A Method to Identify the Limiting Imperviousness Threshold Based on Watershed Characteristics. J. Hydrology 587, 124958. doi:10.1016/j.jhydrol.2020.124958
Liang, C.-Y., You, G. J.-Y., and Lee, H.-Y. (2019). Investigating the Effectiveness and Optimal Spatial Arrangement of Low-Impact Development Facilities. J. Hydrology 577, 124008. doi:10.1016/j.jhydrol.2019.124008
Liao, Y., Zhao, H., Jiang, Z., Li, J., and Li, X. (2021). Identifying the Risk of Urban Nonpoint Source Pollution Using an Index Model Based on Impervious-Pervious Spatial Pattern. J. Clean. Prod. 288, 125619. doi:10.1016/j.jclepro.2020.125619
Liu, J., Xu, J., Zhang, X., Liang, Z., and Rao, K. (2021). Nonlinearity and Threshold Effects of Landscape Pattern on Water Quality in a Rapidly Urbanized Headwater Watershed in China. Ecol. Indic. 124, 107389. doi:10.1016/j.ecolind.2021.107389
Ma, Y., He, W., Zhao, H., Zhao, J., Wu, X., Wu, W., et al. (2019). Influence of Low Impact Development Practices on Urban Diffuse Pollutant Transport Process at Catchment Scale. J. Clean. Prod. 213, 357–364. doi:10.1016/j.jclepro.2018.12.198
Morabito, M., Crisci, A., Guerri, G., Messeri, A., Congedo, L., and Munafò, M. (2021). Surface Urban Heat Islands in Italian Metropolitan Cities: Tree Cover and Impervious Surface Influences. Sci. Total Environ. 751, 142334. doi:10.1016/j.scitotenv.2020.142334
Prcmep (2009). Water Quality-Determination of Ammonia Nitrogen-Nessler's Reagent Spectrophotometry. Beijing: Ministry of Environmental Protection of the People's Republic of China.
Prcmep (1989). Water Quality-Determination of Total Nitrogen-Alkaline Potassium Persulfate Digestion-UV Spectrophotometric Method. Beijing: Ministry of Environmental Protection of the People's Republic of China.
Prcmep (1990). Water Quality-Determination of Total Phosphorus-Ammonium Molybdate Spectrophotometric Method. Beijing: Ministry of Environmental Protection of the People's Republic of China.
Prcmep (2013). Water Quality-Digestion of Total Metals-Microwave Assisted Acid Digestion Method. Beijing: Ministry of Environmental Protection of the People's Republic of China.
Randall, M., Sun, F., Zhang, Y., and Jensen, M. B. (2019). Evaluating Sponge City Volume Capture Ratio at the Catchment Scale Using SWMM. J. Environ. Manag. 246, 745–757. doi:10.1016/j.jenvman.2019.05.134
Salerno, F., Gaetano, V., and Gianni, T. (2018). Urbanization and Climate Change Impacts on Surface Water Quality: Enhancing the Resilience by Reducing Impervious Surfaces. Water Res. 144, 491–502. doi:10.1016/j.watres.2018.07.058
Sansalone, J. J., and Kim, J.-Y. (2008). Transport of Particulate Matter Fractions in Urban Source Area Pavement Surface Runoff. J. Environ. Qual. 37 (5), 1883–1893. doi:10.2134/jeq2007.0495
Shehab, Z. N., Jamil, N. R., Aris, A. Z., and Shafie, N. S. (2021). Spatial Variation Impact of Landscape Patterns and Land Use on Water Quality across an Urbanized Watershed in Bentong, Malaysia. Ecol. Indic. 122, 107254. doi:10.1016/j.ecolind.2020.107254
Silva, C. D. M., and Silva, G. B. L. D. (2020). Cumulative Effect of the Disconnection of Impervious Areas within Residential Lots on Runoff Generation and Temporal Patterns in a Small Urban Area. J. Environ. Manag. 253, 109719. doi:10.1016/j.jenvman.2019.109719
Sohn, W., Kim, J.-H., Li, M.-H., Brown, R. D., and Jaber, F. H. (2020). How Does Increasing Impervious Surfaces Affect Urban Flooding in Response to Climate Variability? Ecol. Indic. 118, 106774. doi:10.1016/j.ecolind.2020.106774
Tang, S., Jiang, J., Zheng, Y., Hong, Y., Chung, E.-S., Shamseldin, A. Y., et al. (2021). Robustness Analysis of Storm Water Quality Modelling with LID Infrastructures from Natural Event-Based Field Monitoring. Sci. Total Environ. 753, 142007. doi:10.1016/j.scitotenv.2020.142007
Usepa (1994). “Metod 200.8,” in Trace Elements in Waters and Wastes by Inductively Coupled Plasma-Mass Spectrometry (Washington, DC: US Environmental Protection Agency).
Wang, Z., Zhang, S., Peng, Y., Wu, C., Lv, Y., Xiao, K., et al. (2020). Impact of Rapid Urbanization on the Threshold Effect in the Relationship between Impervious Surfaces and Water Quality in Shanghai, China. Environ. Pollut. 267, 115569. doi:10.1016/j.envpol.2020.115569
Yao, L., Wu, Z., Wang, Y., Sun, S., Wei, W., and Xu, Y. (2020). Does the Spatial Location of Green Roofs Affects Runoff Mitigation in Small Urbanized Catchments? J. Environ. Manag. 268, 110707. doi:10.1016/j.jenvman.2020.110707
Zaqout, T., and Andradóttir, H. Ó. (2021). Hydrologic Performance of Grass Swales in Cold Maritime Climates: Impacts of Frost, Rain-On-Snow and Snow Cover on Flow and Volume Reduction. J. Hydrology 597, 126159. doi:10.1016/j.jhydrol.2021.126159
Keywords: urban runoff pollution, pervious/impervious landscape pattern, low impact development, pollutant transport, catchment scale
Citation: Ma Y and Zhao H (2022) The Role of Spatial Patterns of Low Impact Development in Urban Runoff Pollution Control Within Parcel Based Catchments. Front. Environ. Sci. 10:926937. doi: 10.3389/fenvs.2022.926937
Received: 25 April 2022; Accepted: 10 June 2022;
Published: 30 June 2022.
Edited by:
Celso Santos, Federal University of Paraíba, BrazilReviewed by:
Jose Navarro Pedreño, Miguel Hernández University of Elche, SpainCopyright © 2022 Ma and Zhao. This is an open-access article distributed under the terms of the Creative Commons Attribution License (CC BY). The use, distribution or reproduction in other forums is permitted, provided the original author(s) and the copyright owner(s) are credited and that the original publication in this journal is cited, in accordance with accepted academic practice. No use, distribution or reproduction is permitted which does not comply with these terms.
*Correspondence: Hongtao Zhao, aHR6aGFvQHJjZWVzLmFjLmNu
Disclaimer: All claims expressed in this article are solely those of the authors and do not necessarily represent those of their affiliated organizations, or those of the publisher, the editors and the reviewers. Any product that may be evaluated in this article or claim that may be made by its manufacturer is not guaranteed or endorsed by the publisher.
Research integrity at Frontiers
Learn more about the work of our research integrity team to safeguard the quality of each article we publish.