- Jiangsu Key Laboratory for Biodiversity and Biotechnology, School of Biological Sciences, Nanjing Normal University, Nanjing, China
In aquatic ecosystems, cladocerans, an important part of zooplankton, are often exposed to new pollutant nanoparticles and poor quality food simultaneously. To evaluate the combined effects of poor quality food and nanoparticles on the development and early reproductive performance of cladocerans, we exposed Daphnia magna, a representative cladoceran, to different concentrations of ZnO nanoparticles under the food conditions containing different proportions of non-toxic Microcystis, recorded some key indicators of the early life history, and analyzed the possible differential dose effects. The results showed that non-toxic Microcystis and ZnO nanoparticles significantly delayed the times to maturation and reproduction and also significantly reduced the survival time, the body length at maturation, the number of offspring in the first brood, and the total offspring per female of D. magna. When the food contained non-toxic Microcystis, D. magna did not reach sexual maturity at 0.25 mg L−1 ZnO nanoparticles and did not develop eggs and reproduce offspring at 0.20 mg L−1 ZnO nanoparticles, especially non-toxic Microcystis and ZnO nanoparticles had a significant or nearly significant synergistic effect on the time to maturation, time to first brood, and the total offspring per female of D. magna, whereas for other life history indicators, non-toxic Microcystis and ZnO nanoparticles showed an additive effect, based on comparing the slopes of linear fitting of the relevant indicators with nanoparticle concentration under different food quality conditions. Such findings are helpful to realistically evaluate the comprehensive stress effect on zooplankton under the complex conditions of poor food quality and coexistence of pollutants.
Introduction
Zooplankton, the primary consumers in aquatic ecosystems, plays a key role in transferring the energy fixed by the primary producer algae to the higher trophic levels (Miner et al., 2012). Under natural conditions, in addition to the fluctuation of algal abundance, there are also changes in algal composition in the aquatic environment (Roshith et al., 2018), which will change the food quality of zooplankton, especially in eutrophic freshwaters, cyanobacteria blooms often break out, in which Microcystis is the dominant group (Humbert et al., 2013; Sun et al., 2013). Both toxic Microcystis and non-toxic Microcystis strains can be found in the same waters (Wilson et al., 2005), and their composition changes with seasonal succession (Rinta-Kanto et al., 2009). Toxic Microcystis not only lacks key nutritional composition but also produces microcystin, which brings fatal harm to zooplankton (Yang et al., 2011; Lyu et al., 2016a; Huang et al., 2020). Compared with green alga Chlorella, non-toxic Microcystis lacks essential fatty acids, sterols, and other nutrients necessary for zooplankton (Von Elert and Wolffrom, 2001; Martin-Creuzburg et al., 2008); thus, it is also a low-quality food for zooplankton. As a type of non-selective filter-feeding zooplankton, cladocerans inevitably ingest non-toxic Microcystis, which may lead to malnutrition of cladocerans living in eutrophic waters, resulting in a series of negative effects (Lürling, 2003; Huang et al., 2020). Lyu et al. (2016b) demonstrated that long-term feeding of non-toxic Microcystis aeruginosa will hinder the development of cladocerans and reduce its reproductive output.
Metal oxide nanoparticles (NPs) are commonly used in industry and daily necessities, and the discharge of untreated sewage containing NPs leads to the increasing concentration of NPs in natural aquatic ecosystems, which brings increasingly serious harm to plankton. Many studies have shown that metal oxide NPs have significant negative effects on zooplankton (Lekamge et al., 2018; Geppert et al., 2021; Qi et al., 2022), which usually can last for several generations (Martins et al., 2020). In general, non-toxic Microcystis and NPs can co-exist simultaneously in an aquatic environment. Some studies reported that NPs can inhibit the growth of Microcystis (Sankar et al., 2014), whereas more studies showed that NPs have toxic effects on cladocerans (Wang et al., 2019; Silva et al., 2021; Sun et al., 2022). Therefore, when cladocerans are exposed to Microcystis and NPs simultaneously, it may lead to complex interactions. The combined effect of toxic Microcystis and NPs on cladocerans has been reported (Wang et al., 2019), but when the aquatic ecosystem is dominated by non-toxic Microcystis, the combined stress of NPs and non-toxic Microcystis may have different consequences, especially in the sensitive period of the early life history stage of cladocerans.
Based on the aforementioned related research status, we put forward the following scientific hypothesis: except that different proportions of non-toxic Microcystis and different concentrations of NPs will produce different degrees of stress on cladocerans, the harm of non-toxic Microcystis and NPs may have synergistic effects or at least additive effects on cladocerans. Therefore, in order to test the aforementioned scientific hypothesis and accurately evaluate the possible changes in the tolerance of cladocerans to NPs when eating different quality foods, we placed a representative cladoceran Daphnia magna to different concentrations of ZnO NPs, one of the most commonly used metal oxide NPs in industry and daily necessities, under the food conditions containing different proportions of non-toxic Microcystis, recorded some key indicators of the early life history, and analyzed the possible differential dose effects.
Materials and Methods
Maintenance of Test Organisms
D. magna organisms were maintained in COMBO medium and fed with green alga Chlorella pyrenoidosa (1.5 mgC L−1) at 21°C, 40 µmol photons m−2s−1 light intensity, and 14 h:10 h light: dark cycle. C. pyrenoidosa and non-toxic Microcystis aeruginosa (FACHB-469) were cultured separately in BG-11 medium under the same conditions as described previously and harvested at the exponential growth phase. These harvested algae were used as food of D. magna in our following experiments.
Experimental Design
The fresh stock solution of ZnO NPs (0.10 g L−1) was prepared by distributing the ZnO NPs in deionized water and then sonicated for 30 min at 40 kHz before the experiment (Wang et al., 2019; Sun et al., 2022). ZnO NPs aggregated flocculation in different shapes (including round, rectangular, and anomalous) with sizes of dozens of nanometers in diameter (for detailed physicochemical characterization, see Wang et al. (2019)). The specific testing solutions with different concentrations of ZnO NPs (0, 0.05, 0.10, 0.15, 0.20, 0.25, 0.30, and 0.40 mg L−1) were obtained by diluting the stock solution of ZnO NPs in COMBO medium. The test concentrations of ZnO NPs, respectively, represented the environmentally relevant concentrations in some specific aquatic environments at present and the possible increased levels without effective control in the future. Three food combinations with different nutritional qualities but containing the same carbon biomass (1.5 mg C L−1) were used in the experiment: 1) 100% C. pyrenoidosa (100%Ch), 2) a mixture of 85% C. pyrenoidosa+15% non-toxic M. aeruginosa (85%Ch+15%MF), and 3) a mixture of 70% C. pyrenoidosa+30% non-toxic M. aeruginosa (70%Ch+30%MF). There were a total of 24 treatments based on a fully factorial design of eight ZnO NP concentrations and three food types, and 10 replicates were set for each treatment. The neonates (<12 h) from the third broods of 20 maternal D. magna that grow simultaneously were randomly selected for the experiment. One D. magna neonate was randomly placed in a 50-ml beaker containing 50 ml COMBO medium as one replicate. The experiments were conducted under the same conditions as described previously and lasted for 16 days, which met our requirements for testing the early life history. The culture medium in each beaker was changed daily to maintain the relative stability of food abundance and ZnO NP levels.
Measurements of the Early Life History Traits
To analyze the early life history traits of D. magna under the aforementioned treatments, six key life history traits were recorded: survival time, time to maturation, time to first brood, body length at maturation, number of offspring in the first brood per female, and total offspring per female. Survival time was calculated according to the death of D. magna, which was determined as the time when the heart stopped beating under a dissecting microscope; time to maturation was determined when the first batch of eggs appeared in the brood pouch; time to first brood was determined when first brood offspring was released; body length at maturation was measured from the top of head to the base of the tail-spine under a stereo-microscope using Nikon software; the number of offspring in the first brood was counted and then removed; total offspring was the sum of neonates recorded daily.
Statistical Analysis
All data were presented in the form of mean values ± SE. Two-way ANOVA and Tukey test were used to assess the interaction of ZnO NPs concentrations and food types on D. magna life history traits, and tests statistics were deemed significant when p-values were less than 0.05. Since the life history traits of D. magna showed a linear change trend with the increase of ZnO NP concentrations, in order to accurately fit and obtain more meaningful parameters to compare the effects of food quality, all life history traits of D. magna changing with ZnO NPs concentrations were, respectively, fitted by a linear function, when the slope had a significant difference from zero (α = 0.05); this indicated that the ZnO NP concentration significantly affects this trait. When ZnO NP concentrations were 0.30 and 0.40 mg L−1, the reproductive indicator was 0 and not brought into the fitting. To determine which effect (synergistic, additive, or antagonistic effect) exists between food quality and ZnO NPs, the differences of slopes derived from the aforementioned linear functions under the three food types were compared with one-way ANOVA, followed by the Holm–Sidak method. All statistical analyses were performed using SigmaPlot 14.0.
Results
Survival Time
Two-way ANOVA showed that both non-toxic Microcystis and ZnO NPs significantly reduced the survival time of D. magna, but there was no significant interaction between them (Table 1; Figure 1). The survival time decreased linearly with the increase of ZnO NP concentrations, and the slopes of the linear functions under the three food types were similar (Figure1B), indicating that there was only an additive effect of non-toxic Microcystis on the harmful effects of ZnO NPs.
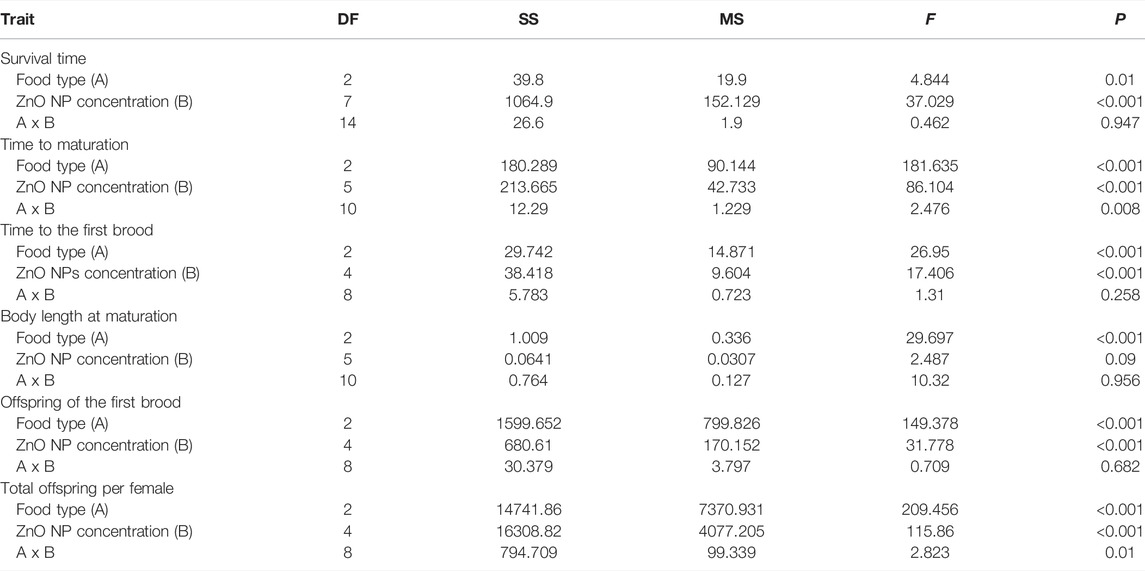
TABLE 1. Two-way ANOVA on survival, development, and reproduction subjected to various ZnO NP levels and food types.
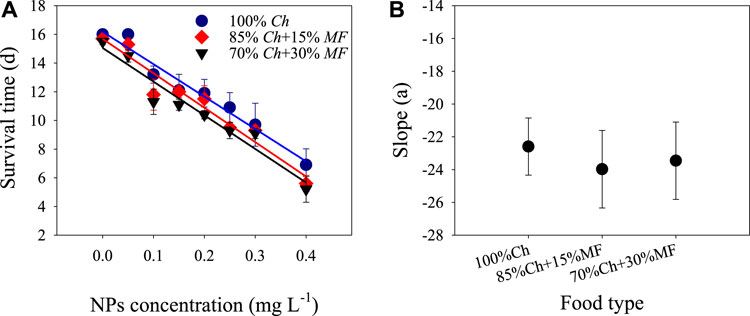
FIGURE 1. Survival time (A) and slope (B) of the fitted liner function of D. magna exposed to different ZnO NP levels under the three food types. Some error bars were too short and covered by the data symbol.
Development Traits
The non-toxic Microcystis and ZnO NPs significantly delayed the time to maturation and the time to the first brood of D. magna and reduced the body length at maturation, but two-way ANOVA showed that food types and ZnO NPs only had a significant interaction with the time to maturation of D. magna (Figure 2; Table 1). These developmental traits changed linearly with the increase of ZnO NP concentrations. The slope derived from the linear fitting on the time to maturation changing with ZnO NP concentrations under 30% non-toxic Microcystis food type was significantly higher than that under only Chlorella food (Figure 2B, p = 0.0163), indicating that the addition of 30% non-toxic Microcystis in the food significantly exacerbated the delay of ZnO NPs on the time to maturation of D. magna, thus causing a synergistic effect. Additionally, to a certain extent (tending to be significant), the addition of 30% non-toxic Microcystis aggravated the delay of ZnO NPs on the time to the first brood of D. magna based on the slope difference (p = 0.149). Both time to maturation and time to the first brood jointly showed that the addition of non-toxic Microcystis to food can significantly aggravate the harm of ZnO NPs to the development of D. magna. Additionally, linear fitting showed that there was no significant difference in the slopes of body length at maturation decreasing with the concentration of ZnO NPs under the three food types (Figure 2F), indicating that there was only an additive effect on body length at maturation between non-toxic Microcystis and ZnO NPs. More seriously, under food types containing non-toxic Microcystis, D. magna could not reach sexual maturity at 0.25 mg L−1 ZnO NPs and could not produce offspring at 0.20 mg L−1 ZnO NPs, meaning that D. magna organisms were subjected to serious comprehensive stress under these conditions.
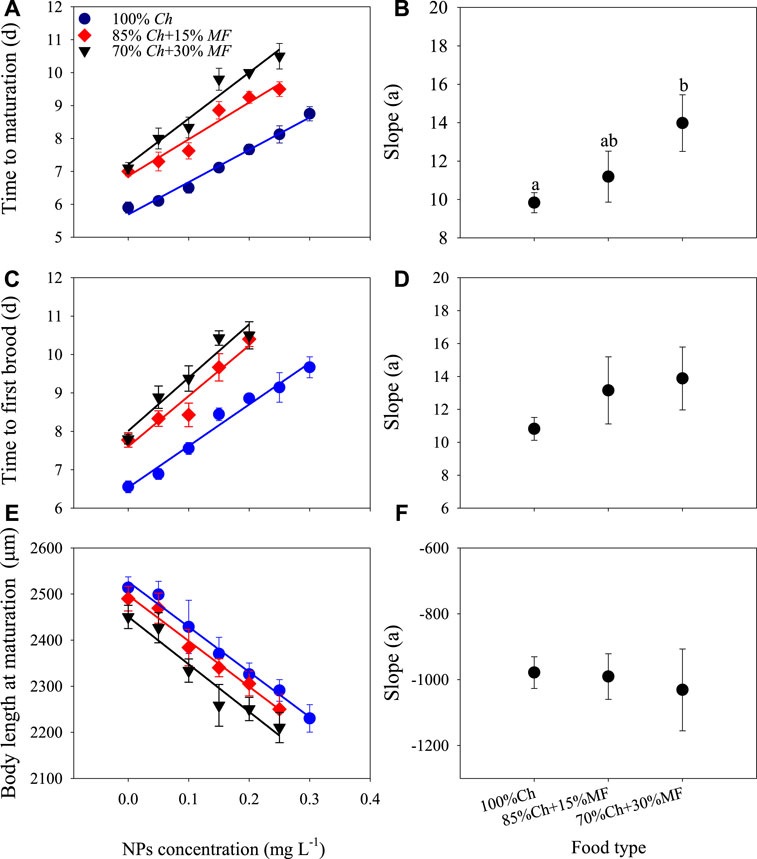
FIGURE 2. Development indicators and their slopes of D. magna under different conditions. Some error bars were too short and covered by the data symbol. Different lowercase letters in the figure represent significant differences.
Reproductive Performances
Two-way ANOVA showed that non-toxic Microcystis and ZnO NPs significantly reduced the reproductive indicators of D. magna (neonates of the first brood and the total offspring per female), and there was an interaction between the two factors with the total offspring per female (Table 1). When ZnO NP concentration was 0.40 mg L−1, D. magna could not develop eggs and produce offspring even at 100% Chlorella of completely good food, while in food containing non-toxic Microcystis, D. magna could not reproduce offspring even when ZnO NP concentration was 0.25 mg L−1 (Figures 3A, C). These reproductive performances decreased linearly with the increase of ZnO NP concentrations. The slopes of neonates of the first brood under the three food types had no significant difference (Figure 3B), indicating that there was only an additive effect on this indicator between non-toxic Microcystis and ZnO NPs. Moreover, the slope under the food type containing 15% non-toxic Microcystis was almost significantly lower than that under 100% Chlorella of completely good food (p = 0.079), indicating that there was a tendency toward synergistic effect and non-toxic Microcystis exacerbated the reduction of total offspring production of D. magna by ZnO NPs to a certain extent.
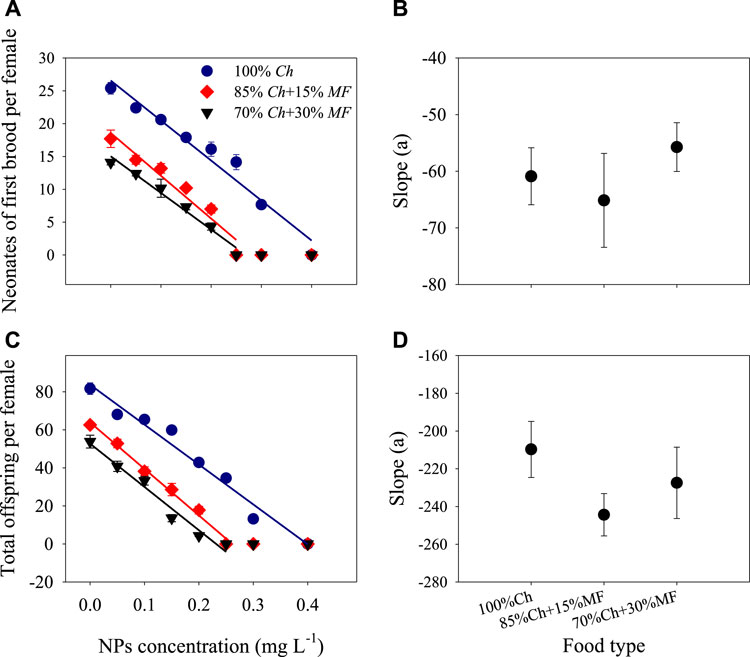
FIGURE 3. Reproductive performances and their slopes of Daphnia in different treatments. Some error bars were too short and covered by the data symbol.
Discussion
Our study showed that both non-toxic Microcystis and ZnO NPs negatively affected the survival time, development, and reproductive performances of D. magna significantly, and there was a significant interaction between non-toxic Microcystis and ZnO NPs only in the time to maturation and the total offspring per female, indicating that there were differential comprehensive effects of poor food quality and ZnO nanoparticles on the different key traits of the early life history of D. magna. Based on whether there was a significant difference between the slopes of each trait under different food types, we concluded that poor food quality and ZnO NPs had significant synergistic effects on time to maturation or nearly significant synergistic effects on time to the first brood and the total offspring per female, whereas for survival, body length at maturation, and neonates of the first brood, there was only an additive effect between non-toxic Microcystis and ZnO NPs. Overall, the increase of the proportion of non-toxic Microcystis in mixed food significantly or to some extent exacerbated the harmful effects of ZnO NPs on the early life history traits of D. magna, which basically confirmed our scientific hypothesis.
As cladocerans are key ecological players in the aquatic ecosystem (Miner et al., 2012), poor quality food aggravating the ecotoxicological effects of pollutants on cladocerans may affect the higher trophic-level organisms through the food chain. In this study, we not only used two different nutritional quality algae but also tested different combinations of the algae. Although some studies have demonstrated the toxicity of ZnO NPs to D. magna (Adam et al., 2014; 2015), so far, our experiment was the first study about the comprehensive effects of non-toxic Microcystis and ZnO NPs on cladocerans, which provided a realistic evaluation about the comprehensive stress effect on zooplankton under the complex conditions of poor food quality and coexistence of pollutants.
In natural waters, cladocerans usually ingest green algae and Microcystis simultaneously (Hanazato and Yasuno, 1984). As non-toxic Microcystis lacks nutrients such as essential fatty acids and sterols (Von Elert and Wolffrom, 2001; Martin-Creuzburg et al., 2008), many studies have demonstrated that non-toxic Microcystis is a poor quality food for zooplankton (Hanazato and Yasuno, 1984; Smith, 1995). The nutritional deficiency of non-toxic Microcystis in mixed food in our experiment was compensated to a certain extent by the nutrition of a higher proportion of Chlorella, but it cannot be completely compensated, as reported in some previous studies that although cladocerans ingesting a good food with a small amount of non-toxic Microcystis can maintain their survival (Huang et al., 2020; Alva-Martínez et al., 2001), they also show a significant decline in development, reproduction, and growth (Hanazato and Yasuno, 1984; Huang et al., 2020), which was consistent with the results of our experiment using a certain proportion of non-toxic Microcystis.
D. magna can ingest all the suitable sizes of nanoparticles in the aquatic environment (Danabas et al., 2020) and exhibit delayed maturation and decreased offspring production (Park et al., 2021), which was further demonstrated in our experiment. The harmful effects of various concentrations of ZnO NPs on some indicators related to the development and reproduction of D. magna were exacerbated by the consumption of mixed food containing non-toxic Microcystis compared with high-quality food in our study. Most studies have shown that the sensitivity of zooplankton to environmental stress is affected by food quality (Heugens et al., 2006; Ieromina et al., 2014; Betini et al., 2020). Daphnia are non-selective filter-feeding zooplankton, so non-toxic Microcystis does not affect its total food intake, but the nutrients obtained are different. When fed with a high proportion of poor-quality Microcystis, D. magna cannot obtain enough energy or essential nutrition to resist the nanoparticles (Lyu et al., 2013), and thus the development and reproduction are inhibited synergistically (Elert et al., 2003; Martin-Creuzburg et al., 2008). It should be emphasized that we got special findings in our experiments, that is, some life history traits were affected by poor food quality and ZnO NPs synergistically or nearly synergistically, whereas other traits were only affected by the additive effect of the two factors, indicating there were sensitivity differences among different traits. The synergistic effect of non-toxic Microcystis and ZnO NPs basically acts on the traits of the reproductive period of D. magna, that is, the traits related to reproduction are more sensitive and vulnerable to the synergistic effects of low-quality food and pollutants, which may be due to the narrow tolerance range of organisms to external ecological factors during the reproductive period, just as the reproductive indicators of D. magna are more sensitive to the increase of salinity (Huang et al., 2022). Therefore, it is of great significance to select specific indicators when evaluating the comprehensive effects of pollutants.
Conclusion
We supported the wide body of literature that recognizes ZnO NPs as hazardous substances and extended the results under complex food conditions. Non-toxic Microcystis and ZnO NPs significantly delayed the time to maturation and the time to reproduction and also significantly reduced the survival time, the body length at maturation, the number of offspring in the first brood, and the total offspring per female of D. magna. Although the Microcystis used in this experiment was non-toxic, its low nutritional quality still had a significant or nearly significant synergistic effect with ZnO NPs on some key life history traits of D. magna, such as time to maturation, time to the first brood, and the total offspring per female of D. magna, whereas for other life history indicators, non-toxic Microcystis and ZnO NPs only showed an additive effect. Such findings are helpful to realistically evaluate the comprehensive stress effect on zooplankton under the complex conditions of poor food quality and coexistence of pollutants. However, the robustness of these findings requires evaluation of other clones and species of cladocerans from pristine and non-toxic Microcystis-impacted waters.
Data Availability Statement
The raw data supporting the conclusion of this article will be made available by the authors, without undue reservation.
Author Contributions
YS and ZY conceived and designed the experiment. YS, BY, YL, SQ, and KL performed the experiments and analyzed the data. YS and ZY wrote the manuscript. All authors gave their final approval of the submitted and published versions.
Funding
The study was supported by the National Natural Science Foundation of China (31730105) and the Priority Academic Program Development of Jiangsu Higher Education Institutions of China.
Conflict of Interest
The authors declare that the research was conducted in the absence of any commercial or financial relationships that could be construed as a potential conflict of interest.
Publisher’s Note
All claims expressed in this article are solely those of the authors and do not necessarily represent those of their affiliated organizations, or those of the publisher, the editors, and the reviewers. Any product that may be evaluated in this article, or claim that may be made by its manufacturer, is not guaranteed or endorsed by the publisher.
References
Adam, N., Schmitt, C., Galceran, J., Companys, E., Vakurov, A., Wallace, R., et al. (2014). The Chronic Toxicity of ZnO Nanoparticles and ZnCl2toDaphnia Magnaand the Use of Different Methods to Assess Nanoparticle Aggregation and Dissolution. Nanotoxicology 8, 1–9. doi:10.3109/17435390.2013.822594
Adam, N., Vergauwen, L., Blust, R., and Knapen, D. (2015). Gene Transcription Patterns and Energy Reserves in Daphnia Magna Show No Nanoparticle Specific Toxicity when Exposed to ZnO and CuO Nanoparticles. Environ. Res. 138, 82–92. doi:10.1016/j.envres.2015.02.014
Alva-Martínez, A. F., Sarma, S. S. S., and Nandini, S. (2001). Comparative Population Dynamics of Three Cladoceran Species (Cladocera) in Relation to Different Levels of Chlorella Vulgaris and Microcystis Aeruginosa. Crustaceana 74, 749–764. doi:10.2307/20105310
Betini, G. S., Wang, X., Avgar, T., Guzzo, M. M., and Fryxell, J. M. (2020). Food Availability Modulates Temperature‐dependent Effects on Growth, Reproduction, and Survival in Daphnia Magna. Ecol. Evol. 10, 756–762. doi:10.1002/ece3.5925
Danabas, D., Ates, M., Ertit Tastan, B., Cicek Cimen, I. C., Unal, I., Aksu, O., et al. (2020). Effects of Zn and ZnO Nanoparticles on Artemia salina and Daphnia Magna Organisms: Toxicity, Accumulation and Elimination. Sci. Total Environ. 711, 134869. doi:10.1016/j.scitotenv.2019.134869
Elert, E. V., Martin-Creuzburg, D., and Le Coz, J. R. (2003). Absence of Sterols Constrains Carbon Transfer between Cyanobacteria and a Freshwater Herbivore (Daphnia Galeata). Proc. R. Soc. Lond. B 270, 1209–1214. doi:10.1098/rspb.2003.2357
Geppert, J. R., Buhani, P., Al-Shamery, K., Bininda-Emonds, O. R. P., and Ahlrichs, W. H. (2021). Lethal Effects and Ultrastructure of Cellular Uptake of Ingested Gold Nanoparticles in the Freshwater Rotifer Brachionus Calyciflorus (Monogononta: Brachionidae). Environ. Pollut. 289, 117897. doi:10.1016/j.envpol.2021.117897
Hanazato, T., and Yasuno, M. (1984). Growth, Reproduction and Assimilation of Moina Macrocopa Fed on Microcystis And/or. Chlorella. Jpn. J. Ecol. 34, 195–202. doi:10.18960/seitai.34.2_195
Heugens, E. H. W., Tokkie, L. T. B., Kraak, M. H. S., Hendriks, A. J., van Straalen, N. M., and Admiraal, W. (2006). Population Growth of Daphnia Magna under Multiple Stress Conditions: Joint Effects of Temperature, Food, and Cadmium. Environ. Toxicol. Chem. 25, 1399–1407. doi:10.1897/05-294R.1
Huang, J., Li, Y., Zhou, Q., Sun, Y., Zhang, L., Gu, L., et al. (2020). Non-toxic and Toxic Microcystis Aeruginosa Reduce the Tolerance of Daphnia pulex to Low Calcium in Different Degrees: Based on the Changes in the Key Life-History Traits. Chemosphere 248, 126101. doi:10.1016/j.chemosphere.2020.126101
Huang, J., Li, Y., Sun, Y., Zhang, L., Lyu, K., and Yang, Z. (2022). Size-specific Sensitivity of Cladocerans to Freshwater Salinization: Evidences from the Changes in Life History and Population Dynamics. Environ. Pollut. 296, 118770. doi:10.1016/j.envpol.2021.118770
Humbert, J.-F., Barbe, V., Latifi, A., Gugger, M., Calteau, A., Coursin, T., et al. (2013). A Tribute to Disorder in the Genome of the Bloom-Forming Freshwater Cyanobacterium Microcystis Aeruginosa. Plos One 8, e70747. doi:10.1371/journal.pone.0070747
Ieromina, O., Peijnenburg, W. J. G. M., de Snoo, G., Müller, J., Knepper, T. P., and Vijver, M. G. (2014). Impact of Imidacloprid onDaphnia Magnaunder Different Food Quality Regimes. Environ. Toxicol. Chem. 33, 621–631. doi:10.1002/etc.2472
Lekamge, S., Miranda, A. F., Abraham, A., Li, V., Shukla, R., Bansal, V., et al. (2018). The Toxicity of Silver Nanoparticles (AgNPs) to Three Freshwater Invertebrates with Different Life Strategies: Hydra Vulgaris, Daphnia Carinata, and Paratya Australiensis. Front. Environ. Sci. 6, 152. doi:10.3389/fenvs.2018.00152
Lürling, M. (2003). Daphnia Growth on Microcystin-Producing and Microcystin-free Microcystis Aeruginosa in Different Mixtures with the Green Alga Scenedesmus Obliquus. Limnol. Oceanogr. 48, 2214–2220. doi:10.2307/3597822
Lyu, K., Zhu, X., Wang, Q., Chen, Y., and Yang, Z. (2013). Copper/Zinc Superoxide Dismutase from the CladoceranDaphnia Magna: Molecular Cloning and Expression in Response to Different Acute Environmental Stressors. Environ. Sci. Technol. 47, 130710143609005–8893. doi:10.1021/es4015212
Lyu, K., Meng, Q., Zhu, X., Dai, D., Zhang, L., Huang, Y., et al. (2016a). Changes in iTRAQ-Based Proteomic Profiling of the Cladoceran Daphnia Magna Exposed to Microcystin-Producing and Microcystin-free Microcystis Aeruginosa. Environ. Sci. Technol. 50, 4798–4807. doi:10.1021/acs.est.6b001010.1021/acs.est.6b00101
Lyu, K., Guan, H., Wu, C., Wang, X., Wilson, A. E., and Yang, Z. (2016b). Maternal Consumption of Non-toxicMicrocystisbyDaphnia Magnainduces Tolerance to toxicMicrocystisin Offspring. Freshw. Biol. 61, 219–228. doi:10.1111/fwb.12695
Martin-Creuzburg, D., Von Elert, E., and Hoffmann, K. H. (2008). Nutritional Constraints at the Cyanobacteria-Daphnia Magna Interface: the Role of Sterols. Limnol. Oceanogr. 53, 456–468. doi:10.4319/lo.2008.53.2.0456
Martins, N., Pradhan, A., Pascoal, C., and Cássio, F. (2020). Effects of Metal Nanoparticles on Freshwater Rotifers May Persist across Generations. Aquat. Toxicol. 229, 105652. doi:10.1016/j.aquatox.2020.105652
Miner, B. E., De Meester, L., Pfrender, M. E., Lampert, W., and Hairston, N. G. (2012). Linking Genes to Communities and Ecosystems: Daphnia as an Ecogenomic Model. Proc. R. Soc. B 279, 1873–1882. doi:10.1098/rspb.2011.2404
Park, H. S., Behzadi Tayemeh, M., Yu, I. J., and Johari, S. A. (2021). Evaluation of Silver Nanowires (AgNWs) Toxicity on Reproductive Success of Daphnia Magna over Two Generations and Their Teratogenic Effect on Embryonic Development. J. Hazard. Mater. 412, 125339. doi:10.1016/j.jhazmat.2021.125339
Qi, Q., Li, Q., Li, J., Mo, J., Tian, Y., and Guo, J. (2022). Transcriptomic Analysis and Transgenerational Effects of ZnO Nanoparticles on Daphnia Magna: Endocrine-Disrupting Potential and Energy Metabolism. Chemosphere 290, 133362. doi:10.1016/j.chemosphere.2021.133362
Rinta-Kanto, J. M., Konopko, E. A., Debruyn, J. M., Bourbonniere, R. A., Boyer, G. L., and Wilhelm, S. W. (2009). Lake Erie Microcystis: Relationship between Microcystin Production, Dynamics of Genotypes and Environmental Parameters in a Large Lake. Harmful Algae 8, 665–673. doi:10.1016/j.hal.2008.12.004
Roshith, C. M., Meena, D. K., Manna, R. K., Sahoo, A. K., Swain, H. S., Raman, R. K., et al. (2018). Phytoplankton Community Structure of the Gangetic (Hooghly-Matla) Estuary: Status and Ecological Implications in Relation to Eco-Climatic Variability. Flora 240, 133–143. doi:10.1016/j.flora.2018.01.001
Sankar, R., Prasath, B. B., Nandakumar, R., Santhanam, P., Shivashangari, K. S., and Ravikumar, V. (2014). Growth Inhibition of Bloom Forming Cyanobacterium Microcystis Aeruginosa by Green Route Fabricated Copper Oxide Nanoparticles. Environ. Sci. Pollut. Res. 21, 14232–14240. doi:10.1007/s11356-014-3362-1
Silva, M. L. N., Nogueira, D. J., Köerich, J. S., Vaz, V. P., Justino, N. M., Schmidt, J. R. A., et al. (2021). Multigenerational Toxic Effects on Daphnia Magna Induced by Silver Nanoparticles and Glyphosate Mixture. Environ. Toxicol. Chem. 40, 1123–1131. doi:10.1002/etc.4952
Smith, A. D., and Gilbert, J. J. (1995). Relative Susceptibilities of Rotifers and Cladocerans to Microcystis Aeruginosa. archiv_hydrobiologie 132, 309–336. doi:10.1127/archiv-hydrobiol/132/1995/309
Sun, H., Wang, W., Geng, L., Chen, Y., and Yang, Z. (2013). In Situ studies on Growth, Oxidative Stress Responses, and Gene Expression of Juvenile Bighead Carp (Hypophthalmichthys Nobilis) to Eutrophic Lake Water Dominated by Cyanobacterial Blooms. Chemosphere 93, 421–427. doi:10.1016/j.chemosphere.2013.05.022
Sun, Y., Liu, Q., Huang, J., Li, D., Huang, Y., Lyu, K., et al. (2022). Food Abundance Mediates the Harmful Effects of ZnO Nanoparticles on Development and Early Reproductive Performance of Daphnia Magna. Ecotoxicol. Environ. Saf. 236, 113475. doi:10.1016/j.ecoenv.2022.113475
Von Elert, E., and Wolffrom, T. (2001). Supplementation of Cyanobacterial Food with Polyunsaturated Fatty Acids Does Not Improve Growth of Daphnia. Limnol. Oceanogr. 46, 1552–1558. doi:10.4319/lo.2001.46.6.1552
Wang, Y., Qin, S., Li, Y., Wu, G., Sun, Y., Zhang, L., et al. (2019). Combined Effects of ZnO Nanoparticles and Toxic Microcystis on Life-History Traits of Daphnia Magna. Chemosphere 233, 482–492. doi:10.1016/j.chemosphere.2019.05.269
Wilson, A. E., Sarnelle, O., Neilan, B. A., Salmon, T. P., Gehringer, M. M., and Hay, M. E. (2005). Genetic Variation of the Bloom-Forming Cyanobacterium Microcystis Aeruginosa within and Among Lakes: Implications for Harmful Algal Blooms. Appl. Environ. Microbiol. 71, 6126–6133. doi:10.1128/AEM.71.10.6126-6133.2005
Keywords: Daphnia magna, development, life history, non-toxic Microcystis, reproduction, ZnO nanoparticles
Citation: Sun Y, Yu B, Lei Y, Qin S, Lyu K and Yang Z (2022) Differential Comprehensive Effects of Food Quality and ZnO Nanoparticles on the Key Traits of Early Life History of Daphnia magna. Front. Environ. Sci. 10:918570. doi: 10.3389/fenvs.2022.918570
Received: 12 April 2022; Accepted: 06 May 2022;
Published: 08 June 2022.
Edited by:
Chenglian Feng, Chinese Research Academy of Environmental Sciences, ChinaCopyright © 2022 Sun, Yu, Lei, Qin, Lyu and Yang. This is an open-access article distributed under the terms of the Creative Commons Attribution License (CC BY). The use, distribution or reproduction in other forums is permitted, provided the original author(s) and the copyright owner(s) are credited and that the original publication in this journal is cited, in accordance with accepted academic practice. No use, distribution or reproduction is permitted which does not comply with these terms.
*Correspondence: Zhou Yang, eWFuZ3pob3VAbmpudS5lZHUuY24=