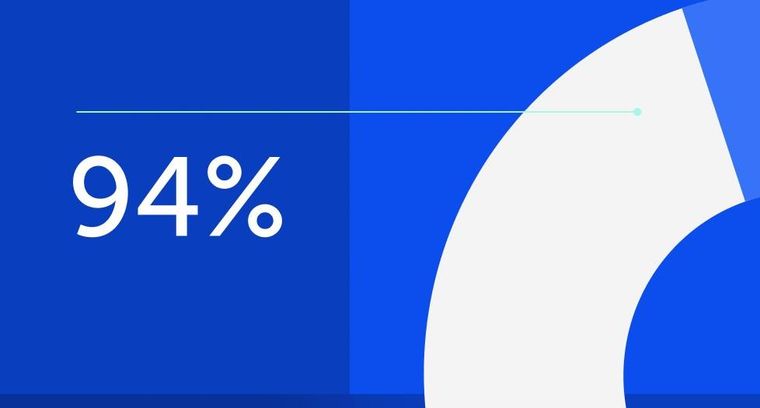
94% of researchers rate our articles as excellent or good
Learn more about the work of our research integrity team to safeguard the quality of each article we publish.
Find out more
ORIGINAL RESEARCH article
Front. Environ. Sci., 13 June 2022
Sec. Water and Wastewater Management
Volume 10 - 2022 | https://doi.org/10.3389/fenvs.2022.915465
This article is part of the Research TopicWastewater Treatment: Removal of Recalcitrant CompoundsView all 7 articles
Typical organophosphorus pesticides, such as glyphosate, trichlorfon, and ethephon, are widely used nowadays, and the treatment of their production wastewater is difficult to achieve by conventional water treatment methods. A Cu/Fe bimetallic system has been proposed as a viable technology to treat toxic and refractory pollutants. The performance of the system with different pH, Cu/Fe molar ratios, Cu/Fe dosages, and initial glyphosate concentrations was analyzed to investigate the operational factors affecting glyphosate removal by the Cu/Fe bimetallic system. Acidic pH (2.0), 250 g/L Cu/Fe dosage, 0.25% Cu/Fe, and 60 mg/L initial glyphosate concentration were the appropriate conditions for glyphosate removal, in which the efficiency of the system was in accordance with the pseudo–second-order kinetic model. Under this condition, the removal efficiencies of total phosphorus and total organic carbon reached 99 and 65%, respectively. The Fe/Cu bimetal surface was characterized by scanning electron microscopy, energy-dispersive X-ray spectroscopy, X-ray diffraction, and Fourier transform infrared spectroscopy. The optimal conditions for glyphosate decomposition were applied to the treatment of trichlorfon and ethephon. The removal efficiency of the system was poor, but its toxicity to luminescent bacteria Q67 was remarkably reduced.
Organophosphorus pesticides are widely used in agricultural production (Fu et al., 2022) due to low toxicity and high efficiency. According to the roles, they are categorized into herbicides, insecticides, plant growth regulators, and others. Commonly used glyphosate, trichlorfon and ethephon, are broad-spectrum and non-selective typical organophosphorus pesticides (Tian et al., 2016; Dubois et al., 2018), which are widely used in agriculture, forestry, orchards, and gardens. For example, glyphosate can effectively control 76 of the world’s 78 harmful weeds (Wang et al., 2006; Hansen and Roslev, 2016). However, due to the excessive use of organophosphorus pesticides in the environment, some harmful effects have been reported for plants, animals and agricultural production. Besides, they can be transferred into water environment through runoff resulting their frequent detection in the aqueous environment (Ajiboye et al., 2022). The concentration of glyphosate in natural water bodies were reported to range from 0.01 to 0.7 mg/L, and it may reach 1.7–5.2 mg/L in worst-case scenarios such as direct application or accidental spills (Peruzzo et al., 2008; Annett et al., 2014). The applied concentration of trichlorfon in ponds to eradicate ectoparasites range between 0.1 and 1.0 mg/L (Ma et al., 2018). Ethephon concentrations in the aqueous environment are rarely reported, but the residual level in plant origin samples reached as high as 4.5 mg/kg (Gormez et al., 2021).
Methods for treating wastewater from organophosphorus pesticide production include physicochemical treatment, chemical oxidation and biological treatment. For example, porous materials such as activated carbon (Mailler et al., 2016) and clay-biochar composite (Rallet et al., 2022) are used in the adsorption treatment of glyphosate. Besides, glyphosate and its degradation products, including aminomethylphosphonic acid, were reported to be adsorbed to mineral surface through coordination of both the carboxyl and phosphonic group (Khoury et al., 2010). Degradation of glyphosate is driven by primarily oxidation using minerals (Paudel et al., 2015), mineral-activated carbon composite (Cui et al., 2012), UV–photo-oxidation (Jaisi et al., 2016) or electrochemical degradation (Aquino Neto and de Andrade, 2009). The degradation pathways include the C–N bond cleavage pathway with aminomethylphosphonic acid as the main product, and the C–P bond cleavage pathway with the formation of sarcosine and glycine accompanied by phosphoric acid formation (Grandcoin et al., 2017; la Cecilia and Maggi, 2018).
However, existing methods are limited due to the complex composition of organophosphorus pesticide production wastewater, which contains organophosphorus by-products and metal ions (Saleh et al., 2020). Biochemical treatment is ineffective because of the presence of toxic and non-biodegradable components in the wastewater (Badawy et al., 2006). Physicochemical treatment, such as adsorption method, requires an acidic environment, and existing adsorbents have no selectivity for pesticides. The treatment effect is likely affected by the competitive effect with metal ions and anions, such as PO43− and NO3− (Feng et al., 2020; Mostafa et al., 2021). The coagulation sedimentation method has high operating cost, produces a large amount of sludge for disposal, and is only used in the pretreatment process of organophosphorus pesticide wastewater. Advanced oxidation technology, such as Fenton method, has high degradation rate and simple operation, but its use is limited by acidic conditions, which increases the cost of water treatment. Moreover, Fenton method produces a large amount of iron-containing sludge (Ameta et al., 2018).
The Cu/Fe bimetallic system is constructed using copper as the inert electrode and plated on the surface of zero-value iron to form a galvanic battery (Xiong et al., 2017). This system is a cost-effective technology (Shahmahdi et al., 2020) with adaptability to a wider pH range (Lai et al., 2014). The Cu/Fe bimetallic system has been used to treat toxic and refractory pollutants (Xiong et al., 2015), including azo dye (Trujillo–Reyes et al., 2012), organochlorine compound (Ghauch and Tuqan, 2008; Chen et al., 2018), nitrobenzene and aromatic compounds (Lai et al., 2014), and nitrate (Shubair et al., 2018). The influencing factors of the system’s operation, including Fe0 dosage, initial pH, initial pollutant concentration, temperature, and the Cu/Fe molar ratio have been studied and reported by previous researches (Lai et al., 2016; Huong et al., 2021). The mechanism of removal by the Cu/Fe bimetallic system is complex and includes the direct reduction by Fe0; the indirect reduction by •H generated from H+ at the cathode surface (Xiong et al., 2017); and the adsorption, flocculation, and precipitation of iron hydroxides (Huong et al., 2021).
The feasibility of removing organophosphorus pesticides remains a challenge for Cu/Fe bimetallic system. In this work, a Cu/Fe bimetallic system with optimum operating parameters was established to realize efficient removal of glyphosate. Treatment performance and toxicity reduction were evaluated by treating trichlorfon and ethephon in the same system.
Glyphosate (analytical grade, J&K Scientific), ethephon (40%; Shanghai HuaYi Group HuaYuan Chemical Industry Co., Ltd.), and trichlorfon (30%, Shuyang Guoxiang Seedling and Gardening Farm) were separately dissolved and diluted into different concentrations to serve as the artificial pesticide wastewater. All the chemical reagents used were analytical grade or above. Ultrapure water (18.2 MΩ cm) was used for dissolution and dilution in the experiments.
Waste iron shavings are usually selected as zero-value iron materials owing to its good economic benefit (Shahmahdi et al., 2020). In this study, iron shavings were obtained from 20CrMoTi steel of a metal machinery plant. It was been pretreated to remove the oil and other impurities on the surface according to Li (Li et al., 2018). The modified iron shavings were added into CuSO4 solution according to desired Cu/Fe molar ratios. Then the iron shavings after pretreatment were added into CuSO4 solution according to the desired Cu/Fe molar ratios to form the Cu/Fe bimetallic. Then it was freeze-dried under vacuum to use.
The Cu/Fe bimetal was freeze-dried under vacuum. Scanning electron microscopy (SEM) images were obtained using a field-emission scanning electron microscope (Jeol JSM-6360LV, Japan) to visually observe the changes in surface morphology. Electron-dispersive X-ray spectroscopy (EDS) images made by an energy-dispersive spectrometer (EDAX, Falcon, United States) was used to analyze the main element composition of the surface of the iron fillers. X-ray diffraction (XRD) images made by a powder diffractometer (Bruker, D8 Advance, Germany) was used to analyze the changes in the crystallinity of the surface of the iron fillers. Fourier transform infrared spectrometry (FTIR; Thermo Electron, Nicolet 5700, United States) was used to analyze the bonding state of the groups when glyphosate or its degradation products were complexed with the metal.
Different dosages of Cu/Fe (62.5, 125, 187.5, and 250 g/L) bimetallic material with the desired Cu/Fe molar ratio (0.1, 0.25, and 0.5%) were added to the glyphosate solution (initial concentrations of 30, 60, 90, and 120 mg/L). The initial solution pH was adjusted to 2.0, 7.0, and 12.0 using H2SO4 (1.0 mol/L) and NaOH (1.0 mol/L). Experiments were conducted in a 500 ml conical flask at 120 rpm and 25°C for 240 min. Three replicates of samples were collected at 0, 15, 30, 60, 120, 180, and 240 min and filtered by 0.45 μm polyethersulfone syringe filter for analysis.
Based on the study of the operational factors, the pH, Cu/Fe dosage, and Cu/Fe molar ratio with the best treatment performance were selected to treat ethephon solution with a mass fraction of 2% and trichlorfon solution with a mass fraction of 3%. The shaking conditions and duration were the same as those for glyphosate treatment.
Total phosphorus (TP) was determined by alkaline potassium persulfate digestion and molybdenum antimony anti-spectrophotometry. Total iron and ferrous iron ions were determined by o-phenanthroline spectrophotometry. Total organic carbon (TOC) was determined by a TOC analyzer (Tsushima, TOC-L CPH CN200, Japan).
Glyphosate was mostly measured by high-performance liquid chromatography (HPLC) with a derivatization protocol (Pimenta et al., 2020), which used phosphate or borate buffer solution (Nedelkoska and Low, 2004; Kim et al., 2007). However, in this study, Cu/Fe bimetallic was used to treat glyphosate, and a large amount of dissolved iron ions would be generated during the experiment. Phosphate forms precipitation with iron ions, which will block the column if not pretreated. Therefore, ion chromatography (Zhu et al., 1999) was selected for glyphosate determination in this study using the following chromatography conditions: column, Dionex ICS-900; inhibition current, 84 mA; mobile phase, 28 mmol/L NaOH; flow rate, 1.0 ml/min.
Luminescent bacteria can serve as good indicators for toxic substrates and have been widely used in detecting pesticide residues (Jian et al., 2017). Luminescence inhibition assays were conducted using Vibrio qinghaiensis sp. Q67 as the luminescent bacteria to investigate whether the biodegradability of pesticide wastewater improved after treatment. Twelve concentration gradients were designed as geometric series with a dilution factor of 0.7 (Liu et al., 2009). The relative light units of various concentrations of pesticide and controls to Q67 were determined on a Veritas™ luminometer (Turner BioSystems, Inc., United States). The toxicity of a treatment to Q67 was expressed as an inhibition ratio of bioluminescence, which is calculated as follows:
where
The SEM image (Figure 1) shows that the surface of the Cu/Fe bimetal before the reaction was relatively smooth with smaller protruding particles, more uniform particle size, and fewer pores and cracks. Similar results were observed in other studies (Bransfield et al., 2007; Lai et al., 2014). The content of metallic iron was 83.2%, and the content of oxygen was very little. After the reaction, many protrusions were formed on the surface of the Cu/Fe bimetal, and the small particles aggregated into clumps of different sizes, making the surface rough and uneven. The content of metallic iron was 73.02%, and the content of oxygen rose up to 22.02%. Therefore, a large amount of iron oxides/hydroxides were produced during the reaction and covered the metal iron surface.
The XRD results of the Cu/Fe bimetallic surface after the reaction are shown in Figure 2. The crystal phases of oxides on the surface of the Cu/Fe bimetal include the crystal structures of magnetite, hematite, goethite, and leucoside. The formation of Fe3(PO4)2(OH)2 was detected, which proved that glyphosate degradation underwent the C–P bond cleavage pathway as shown in Equation 2, and the reaction was made go forwards due to the formation of precipitation of Fe(II, III) and PO43−(Priambodo et al., 2017).
Equation 2 Degradation of glyphosate by C–P bond cleavage
(2)
Figure 3 shows the infrared spectrum of the precipitate after the reaction of the Cu/Fe bimetallic system. The high-frequency region (∼3,300 cm−1) is the stretching vibration absorption band of –OH or H2O in the hydrate, and the wavenumber 1700–1,300 cm−1 is the carboxyl group (–COO–), amine group (–NH2), methylene group (–CH2), and carbon skeleton (–C–C–) vibration absorption band. The four absorption peaks at 1,200–950 cm−1 represent the stretching vibration of –P–O– in –PO2(OFe)–. The results of the spectrogram showed the presence of iron ion–organic complexes containing crystal water and iron (hydro)oxides in the precipitate. Glyphosate molecule contains negatively charged ligands (amine group, carboxyl group, and phosphonic acid group), leading to adsorption of glyphosate on mineral surfaces through surface complexation, and even coadsorption with Cu(II) at the mineral interface (Sheals et al., 2003; Yang et al., 2018). Iron hydroxides such as goethite and ferrihydrite can also adsorb glyphosate in acidic or neutral medium (Orcelli et al., 2018; Pereira et al., 2019), although the sorption amount of glyphosate decreased because the pH increased in the Cu/Fe bimetallic system.
According to Figure 4A, the glyphosate removal efficiency of the Cu/Fe bimetal system reached 90% after 4 h of reaction in acidic (pH 2.0) medium. Organophosphorus pesticide molecules were removed in the Cu/Fe bimetallic system as the result of the direct reduction of Fe0 and the indirect reduction of •H, and the treatment efficiency of both gradually weakened with increasing pH (Lai et al., 2014). Besides, acidic media enhanced the surface activation rate of Fe and make the plating process more efficient (Ghauch and Tuqan, 2008). Therefore, the glyphosate removal efficiency of the Cu/Fe bimetallic system decreased to 60% (pH 7.0) and 40% (pH 11.0) after 4 h with the increase in pH. The initial pH of the solution without pH adjustment was 3.5 and the medium remained acidic or weakly acidic throughout the reaction (Figure 6); therefore, adjusting the solution pH for the follow-up experiments was not necessary.
FIGURE 4. Glyphosate removal efficiency of the Cu/Fe bimetallic system with different (A) initial pH, (B) Cu/Fe molar ratios, (C) Cu/Fe dosages, and (D) initial glyphosate concentrations.
Figure 4B shows that the removal efficiency rose when the Cu/Fe molar ratio increased from 0.1 to 0.25%. The reason for the improvement is that the amount of Cu attached to the surface of the iron shavings increased, forming more Cu–Fe galvanic corrosion cells on the material surface (Lai et al., 2014; Chen et al., 2018), and the reduction effect was enhanced. However, the treatment effect decreased when the Cu/Fe molar rate reached 0.5%. The contribution of Cu on the reduction benefits from facilitating the generation of surface-bond atomic hydrogen (Bransfield et al., 2006). However, when the produced Cu was excessive, the reaction sites on the Fe0 surface of the anode in the Cu–Fe galvanic cell are excessively occupied by Cu, which reduces the direct contact between the metal iron and the glyphosate and results in a decrease in mass transfer rate and poor treatment effect. A similar phenomenon of excess ratio of Cu/Fe was observed in a previous study (Chen et al., 2018). Therefore, the molar ratio of 0.25% was selected in the follow-up experiments for subsequent study.
Figure 4C shows that the amount of Cu/Fe bimetal has a positive effect on glyphosate removal. The removal efficiency was only about 50% after 4 h of reaction when the amount of Cu/Fe was 62.5 g/L but rose up to 88.2% when the amount of Cu/Fe increased to 250 g/L. Therefore, the glyphosate removal efficiency was easily enhanced by increasing the bimetal dose, which increases the available surface area for adsorption and reaction sites (Guan et al., 2015). However, when the dose was increased from 200 to 250 g/L, the enhancement of the removal performance was not obvious because of the limitation of glyphosate. Therefore, the dosage of 250 g/L was selected for the follow-up experiment.
The removal efficiency decreased slightly with the increase in initial glyphosate concentration as shown in Figure 4D. The glyphosate removal efficiencies of the Cu/Fe bimetallic system at all tested initial concentrations were between 75 and 85% after 4 h of reaction. A glyphosate solution with an initial concentration of 60 mg/L was selected for the follow-up experiments. The mass fraction of glyphosate was reported to be less than 2% (Dai et al., 2013; Shen et al., 2013) in production wastewater, which further decreases after recycling, so the initial concentration selected is close to the actual concentration.
The removal efficiencies of TP and TOC by the Cu/Fe bimetallic system after 3 h of reaction were 99 and 65%, respectively (Figure 5A). The Cu/Fe bimetallic system showed reaction equilibrium at 2 h. Almost all organics containing phosphonic acid groups were removed from the solution and the removal efficiency of TP was much higher than that of TOC, which indicates that glyphosate was not completely mineralized by the Cu/Fe bimetallic system.
FIGURE 5. (A) TOC and TP changes (B) Reaction kinetic curve fitting in Cu/Fe bimetallic system treating glyphosate.
Based on the previous discussion on the influence factors of the Cu/Fe bimetallic system for treating glyphosate, the optimum experimental conditions (Cu/Fe dosage, 250 g/L; initial glyphosate concentration, 60 mg/L, and Cu/Fe molar ratio, 0.25%) were selected to study the kinetics of the reaction. Reaction kinetic curve fitting was realized through the “non-linear curve fit” module in Origin 2022. The correlation coefficient (R2) of the second-order reaction kinetics was higher than that of the first-order reaction kinetics (Figure 5B). Therefore, the pseudo-second-order reaction model better describes the removal process of glyphosate in bimetallic systems. The reaction system was multiphase and involved various mechanism including redox reaction, precipitation, and surface adsorption.
The initial pH of the reaction system was 3.5 (Figure 6), which rose rapidly to 5.4 after 30 min of reaction and increase slowly from 5.4 to 6.3 in the next 90 min. The pH value was stable at about 6.3 after 4 h. Glyphosate degradation in acidic solution occurs through the direct reduction of metallic iron and electron reduction in the cathode region of the corrosion cell. The metal iron is oxidized and dissolved as a result of the reaction; therefore, the dissolved iron concentration in the solution gradually increases. This process is a reaction that consumes H+, and a secondary electrochemical corrosion of metallic iron occurred. Therefore, the pH of the solution rose slowly.
Another proton consumption process is the oxidation of Fe2+ into Fe3+, which has a strong ability to bind to hydroxide ions and precipitate when the pH is 2.7. Therefore, Fe(OH)3 was formed under the pH of the reaction system. This process results in a decrease in the concentration of dissolved iron in the solution. Moreover, the reaction process consumes OH− in the solution; therefore, the pH value of the solution will not continue to rise and finally stabilize in a weak alkaline environment.
The removal efficiencies of the TP and TOC of ethephon by the Cu/Fe bimetals was between 40 and 50% at the reaction time of 4 h, respectively (Figure 7), and the trend of reaction equilibrium at this time was not observed in the figure. This result indicates that a higher removal efficiency is expected for engineering applications. The treatment performance for trichlorfon was even lower with a TP removal efficiency of approximately 20%. The poor treatment effect of trichlorfon may be attributed to the difficulty of degrading trichlorfon as an ester-containing phosphorus (Bai et al., 2010; Tian et al., 2016), whereas glyphosate-containing phosphonic acid groups and its main intermediate product as phosphate are more easily adsorbed or precipitated by metals.
FIGURE 7. (A) TP and (B) TOC removal from two organophosphorus pesticides by the Cu/Fe bimetallic system.
The dose–response relationship of several pesticides also fits the Weibull and Logit nonlinear functions (Scholze et al., 2001; Liu et al., 2009). The dose–response curves of ethephon to Q67 were also effectively described by the Weibull function using the “non-linear curve fit” module in Origin 2022 (Figure 8). After ethephon was treated with the Cu/Fe bimetal for 4 h, the inhibition of luminescence was weakened compared with that before the reaction, which indicates an increase in bioluminescence intensity and the decrease in solution toxicity. The concentration–luminescence inhibition relationship of trichlorfon was not consistently in accord with the Weibull model (the fitting curves of low and high concentrations with 0.24 mg/L as the boundary show different trends), but it still indicates that the inhibition rate was reduced after 4 h of treatment with the Cu/Fe bimetal.
The appropriate conditions of the Cu/Fe bimetallic system for treating glyphosate were as follows: acidic initial medium, 25% Cu/Fe ratio, 250 g/L Cu/Fu dosage, and 60 mg/L initial glyphosate concentration. The removal of glyphosate was in accordance with the pseudo–second-order kinetic model, and removal efficiency reached 99% because of the reduction and adsorption of the Cu/Fe bimetal. During treatment, the pH of the solution increased from 3.5 to 6.3 accompanied by the dissolution and oxidation of Fe ions. The toxicity of other organophosphorus pesticides, such as ethephon and trichlorfon, can be effectively reduced by the Cu/Fe bimetal, but the treatment efficiency needs to be improved (Bransfield et al., 2006).
The raw data supporting the conclusion of this article will be made available by the authors, without undue reservation.
XW: Data, Formal analysis, Writing—original draft, Writing—review and editing, Conceptualization. YW: Writing—review and editing. JX: Data, Investigation, Conceptualization. HW: Writing—review and editing, Conceptualization.
This work was supported by the National Natural Science Foundation of China (51778449).
The authors declare that the research was conducted in the absence of any commercial or financial relationships that could be construed as a potential conflict of interest.
All claims expressed in this article are solely those of the authors and do not necessarily represent those of their affiliated organizations, or those of the publisher, the editors and the reviewers. Any product that may be evaluated in this article, or claim that may be made by its manufacturer, is not guaranteed or endorsed by the publisher.
Ajiboye, T. O., Oladoye, P. O., Olanrewaju, C. A., and Akinsola, G. O. (2022). Organophosphorus Pesticides: Impacts, Detection and Removal Strategies. Environ. Nanotechnol. Monit. Manag. 17 (May), 100655. doi:10.1016/J.ENMM.2022.100655
Ameta, R., Chohadia, A. K., Jain, A., and Punjabi, P. B. (2018). “Fenton and Photo-Fenton Processes,” in Advanced Oxidation Processes for Wastewater Treatment. Editors S. C. Ameta, and R. Ameta (London, UK: Emerging Green Chemical Technology), 49–87.
Annett, R., Habibi, H. R., and Hontela, A. (2014). Impact of Glyphosate and Glyphosate-Based Herbicides on the Freshwater Environment. J. Appl. Toxicol. 34 (5), 458–479. doi:10.1002/jat.2997
Aquino Neto, S., and de Andrade, A. R. (2009). Electrochemical Degradation of Glyphosate Formulations at DSA Anodes in Chloride Medium: an AOX Formation Study. J. Appl. Electrochem 39 (10), 1863–1870. doi:10.1007/s10800-009-9890-6
Badawy, M. I., Ghaly, M. Y., and Gad-Allah, T. A. (2006). Advanced Oxidation Processes for the Removal of Organophosphorus Pesticides from Wastewater. Desalination 194 (1–3), 166–175. doi:10.1016/J.DESAL.2005.09.027
Bai, Y., Chen, J., Yang, Y., Guo, L., and Zhang, C. (2010). Degradation of Organophosphorus Pesticide Induced by Oxygen Plasma: Effects of Operating Parameters and Reaction Mechanisms. Chemosphere 81 (3), 408–414. doi:10.1016/J.CHEMOSPHERE.2010.06.071
Bransfield, S. J., Cwiertny, D. M., Roberts, A. L., and Fairbrother, D. H. (2006). Influence of Copper Loading and Surface Coverage on the Reactivity of Granular Iron toward 1,1,1-Trichloroethane. Environ. Sci. Technol. 40 (5), 1485–1490. doi:10.1021/es051300p
Bransfield, S. J., Cwiertny, D. M., Livi, K., and Fairbrother, D. H. (2007). Influence of Transition Metal Additives and Temperature on the Rate of Organohalide Reduction by Granular Iron: Implications for Reaction Mechanisms. Appl. Catal. B Environ. 76 (3–4), 348–356. doi:10.1016/J.APCATB.2007.06.003
Chen, S., Chu, W., Wei, H., Zhao, H., Xu, B., Gao, N., et al. (2018). Reductive Dechlorination of Haloacetamides in Drinking Water by Cu/Fe Bimetal. Sep. Purif. Technol. 203, 226–232. doi:10.1016/J.SEPPUR.2018.04.048
Cui, H., Li, Q., Qian, Y., Zhang, Q., and Zhai, J. (2012). Preparation and Adsorption Performance of MnO2/PAC Composite towards Aqueous Glyphosate. Environ. Technol. 33 (17), 2049–2056. doi:10.1080/09593330.2012.660641
Dai, Z. X., Li, F. H., Gong, Z., and Liao, Q. B. (2013). The Comprehensive Utilization of Waste Water Derived from the Glyphosate Production. Adv. Mater. Res. 781-784, 2051–2054. doi:10.4028/www.scientific.net/AMR.781-784.2051
Dubois, M., van den Broeck, L., and Inzé, D. (2018). The Pivotal Role of Ethylene in Plant Growth. Trends Plant Sci. 23 (4), 311–323. doi:10.1016/j.tplants.2018.01.003
Feng, D., Soric, A., and Boutin, O. (2020). Treatment Technologies and Degradation Pathways of Glyphosate: A Critical Review. Sci. Total Environ. 742, 140559. doi:10.1016/J.SCITOTENV.2020.140559
Fu, H., Tan, P., Wang, R., Li, S., Liu, H., Yang, Y., et al. (2022). Advances in Organophosphorus Pesticides Pollution: Current Status and Challenges in Ecotoxicological, Sustainable Agriculture, and Degradation Strategies. J. Hazard. Mater. 424, 127494. doi:10.1016/J.JHAZMAT.2021.127494
Ghauch, A., and Tuqan, A. (2008). Catalytic Degradation of Chlorothalonil in Water Using Bimetallic Iron-Based Systems. Chemosphere 73 (5), 751–759. doi:10.1016/J.CHEMOSPHERE.2008.06.035
Gormez, E., Golge, O., Dincay, O., and Kabak, B. (2021). A Potential Threat to Black Figs Intended for Export from Turkey: Ethephon. J. Food Compos. Analysis 102, 103989. doi:10.1016/J.JFCA.2021.103989
Grandcoin, A., Piel, S., and Baurès, E. (2017). AminoMethylPhosphonic Acid (AMPA) in Natural Waters: Its Sources, Behavior and Environmental Fate. Water Res. 117, 187–197. doi:10.1016/J.WATRES.2017.03.055
Guan, X., Sun, Y., Qin, H., Li, J., Lo, I. M. C., He, D., et al. (2015). The Limitations of Applying Zero-Valent Iron Technology in Contaminants Sequestration and the Corresponding Countermeasures: The Development in Zero-Valent Iron Technology in the Last Two Decades (1994-2014). Water Res. 75, 224–248. doi:10.1016/J.WATRES.2015.02.034
Hansen, L. R., and Roslev, P. (2016). Behavioral Responses of Juvenile Daphnia Magna after Exposure to Glyphosate and Glyphosate-Copper Complexes. Aquat. Toxicol. 179 (August), 36–43. doi:10.1016/j.aquatox.2016.08.010
Huong, D. T., Van Tu, N., Anh, D. T., Tien, N. A., Ngan, T. T. K., and Van Tan, L. (2021). Removal of Phenol from Aqueous Solution Using Internal Microelectrolysis with Fe-Cu: Optimization and Application on Real Coking Wastewater. Processes 9 (4), 720. doi:10.3390/pr9040720
Jaisi, D. P., Li, H., Wallace, A. F., Paudel, P., Sun, M., Balakrishna, A., et al. (2016). Mechanisms of Bond Cleavage during Manganese Oxide and UV Degradation of Glyphosate: Results from Phosphate Oxygen Isotopes and Molecular Simulations. J. Agric. Food Chem. 64 (45), 8474–8482. doi:10.1021/acs.jafc.6b02608
Jian, Q., Gong, L., Li, T., Wang, Y., Wu, Y., Chen, F., et al. (2017). Rapid Assessment of the Toxicity of Fungal Compounds Using Luminescent Vibrio Qinghaiensis Sp. Q67. Toxins 9, 335. doi:10.3390/toxins9100335
Khoury, G. A., Gehris, T. C., Tribe, L., Torres Sánchez, R. M., and dos Santos Afonso, M. (2010). Glyphosate Adsorption on Montmorillonite: An Experimental and Theoretical Study of Surface Complexes. Appl. Clay Sci. 50 (2), 167–175. doi:10.1016/J.CLAY.2010.07.018
Kim, M., Stripeikis, J., Iñón, F., and Tudino, M. (2007). A Simplified Approach to the Determination of N-Nitroso Glyphosate in Technical Glyphosate Using HPLC with Post-Derivatization and Colorimetric Detection. Talanta 72 (3), 1054–1058. doi:10.1016/j.talanta.2006.12.047
la Cecilia, D., and Maggi, F. (2018). Analysis of Glyphosate Degradation in a Soil Microcosm. Environ. Pollut. 233, 201–207. doi:10.1016/J.ENVPOL.2017.10.017
Lai, B., Zhang, Y., Chen, Z., Yang, P., Zhou, Y., and Wang, J. (2014). Removal of P-Nitrophenol (PNP) in Aqueous Solution by the Micron-Scale Iron-Copper (Fe/Cu) Bimetallic Particles. Appl. Catal. B Environ. 144, 816–830. doi:10.1016/J.APCATB.2013.08.020
Lai, B., Yuan, D.-H., Chen, Z.-Y., Yuan, Y., and Yang, P. (2016). Influence of Temperature on the Removal of Extremely High Concentrationp-Nitrophenol Simulated Wastewater by Fe/Cu/H2O Systems. Clean. Soil Air Water 44 (4), 345–355. doi:10.1002/clen.201400321
Li, X., Chen, W., Ma, L., Wang, H., and Fan, J. (2018). Industrial Wastewater Advanced Treatment via Catalytic Ozonation with an Fe-Based Catalyst. Chemosphere 195, 336–343. doi:10.1016/J.CHEMOSPHERE.2017.12.080
Liu, S.-S., Song, X.-Q., Liu, H.-L., Zhang, Y.-H., and Zhang, J. (2009). Combined Photobacterium Toxicity of Herbicide Mixtures Containing One Insecticide. Chemosphere 75 (3), 381–388. doi:10.1016/J.CHEMOSPHERE.2008.12.026
Ma, Y., Li, B., Ke, Y., Zhang, Y., and Zhang, Y. (2018). Transcriptome Analysis of Rana Chensinensis Liver under Trichlorfon Stress. Ecotoxicol. Environ. Saf. 147, 487–493. doi:10.1016/J.ECOENV.2017.09.016
Mailler, R., Gasperi, J., Coquet, Y., Buleté, A., Vulliet, E., Deshayes, S., et al. (2016). Removal of a Wide Range of Emerging Pollutants from Wastewater Treatment Plant Discharges by Micro-grain Activated Carbon in Fluidized Bed as Tertiary Treatment at Large Pilot Scale. Sci. Total Environ. 542, 983–996. doi:10.1016/J.SCITOTENV.2015.10.153
Mostafa, M., Bin Jumah, M. N., Othman, S. I., Alruhaimi, R. S., Salama, Y. F., Allam, A. A., et al. (2021). Effective Removal of Different Species of Organophosphorus Pesticides (Acephate, Omthosate, and Methyl Parathion) Using Chitosan/Zeolite-A as Multifunctional Adsorbent. Environ. Technol. Innovation 24, 101875. doi:10.1016/J.ETI.2021.101875
Nedelkoska, T. v., and Low, G. K.-C. (2004). High-Performance Liquid Chromatographic Determination of Glyphosate in Water and Plant Material after Pre-column Derivatisation with 9-Fluorenylmethyl Chloroformate. Anal. Chim. Acta 511 (1), 145–153. doi:10.1016/j.aca.2004.01.027
Orcelli, T., di Mauro, E., Urbano, A., Valezi, D. F., da Costa, A. C. S., Zaia, C. T. B. V., et al. (2018). Study of Interaction between Glyphosate and Goethite Using Several Methodologies: An Environmental Perspective. Water Air Soil Pollut. 229 (5). doi:10.1007/s11270-018-3806-1
Paudel, P., Negusse, A., and Jaisi, D. P. (2015). Birnessite‐Catalyzed Degradation of Glyphosate: A Mechanistic Study Aided by Kinetics Batch Studies and NMR Spectroscopy. Soil Sci. Soc. Am. J. 79 (3), 815–825. doi:10.2136/sssaj2014.10.0394
Pereira, R. C., Anizelli, P. R., Eduardo, M., Daniel, F. V., Costa, A. C. S. D., Zaia, C. T. B. V., et al. (2019). The Effect of PH and Ionic Strength on the Adsorption of Glyphosate onto Ferrihydrite. Geochem Trans. 20 (1), 1–14. doi:10.1186/s12932-019-0063-1
Peruzzo, P. J., Porta, A. A., and Ronco, A. E. (2008). Levels of Glyphosate in Surface Waters, Sediments and Soils Associated with Direct Sowing Soybean Cultivation in North Pampasic Region of Argentina. Environ. Pollut. 156 (1), 61–66. doi:10.1016/j.envpol.2008.01.015
Pimenta, E., da Silva, F., Barbosa, É., Cacique, A., Cassimiro, L., de Pinho, G., et al. (2020). Quantification of Glyphosate and AMPA by HPLC-ICP-MS/MS and HPLC-DAD: A Comparative Study. J. Braz. Chem. Soc. 31 (2), 298–304. doi:10.21577/0103-5053.20190175
Priambodo, R., Shih, Y.-J., and Huang, Y. (2017). Phosphorus Recovery as Ferrous Phosphate (Vivianite) from Wastewater Produced in Manufacture of Thin Film Transistor-Liquid Crystal Displays (TFT-LCD) by a Fluidized Bed Crystallizer (FBC). RSC Adv. 7 (65), 40819–40828. doi:10.1039/c7ra06308c
Rallet, D., Paltahe, A., Tsamo, C., and Loura, B. (2022). Synthesis of Clay-Biochar Composite for Glyphosate Removal from Aqueous Solution. Heliyon 8 (3), e09112. doi:10.1016/J.HELIYON.2022.E09112
Saleh, I. A., Zouari, N., and Al-Ghouti, M. A. (2020). Removal of Pesticides from Water and Wastewater: Chemical, Physical and Biological Treatment Approaches. Environ. Technol. Innovation 19, 101026. doi:10.1016/J.ETI.2020.101026
Scholze, M., Boedeker, W., Faust, M., Backhaus, T., Altenburger, R., and Grimme, L. H. (2001). A General Best-Fit Method for Concentration-Response Curves and the Estimation of Low-Effect Concentrations. Environ. Toxicol. Chem. 20, 448–457. doi:10.1002/etc.5620200228
Shahmahdi, N., Dehghanzadeh, R., Aslani, H., and Bakht Shokouhi, S. (2020). Performance Evaluation of Waste Iron Shavings (Fe0) for Catalytic Ozonation in Removal of Sulfamethoxazole from Municipal Wastewater Treatment Plant Effluent in a Batch Mode Pilot Plant. Chem. Eng. J. 383, 123093. doi:10.1016/J.CEJ.2019.123093
Sheals, J., Granström, M., Sjöberg, S., and Persson, P. (2003). Coadsorption of Cu(II) and Glyphosate at the Water-Goethite (α-FeOOH) Interface: Molecular Structures from FTIR and EXAFS Measurements. J. Colloid Interface Sci. 262 (1), 38–47. doi:10.1016/S0021-9797(03)00207-8
Shen, J., Huang, J., Liu, L., Ye, W., Lin, J., and van der Bruggen, B. (2013). The Use of BMED for Glyphosate Recovery from Glyphosate Neutralization Liquor in View of Zero Discharge. J. Hazard. Mater. 260, 660–667. doi:10.1016/J.JHAZMAT.2013.06.028
Shubair, T., Eljamal, O., Khalil, A. M. E., and Matsunaga, N. (2018). Multilayer System of Nanoscale Zero Valent Iron and Nano-Fe/Cu Particles for Nitrate Removal in Porous Media. Sep. Purif. Technol. 193, 242–254. doi:10.1016/J.SEPPUR.2017.10.069
Tian, J., Dong, Q., Yu, C., Zhao, R., Wang, J., and Chen, L. (2016). Biodegradation of the Organophosphate Trichlorfon and its Major Degradation Products by a Novel Aspergillus Sydowii PA F-2. J. Agric. Food Chem. 64 (21), 4280–4287. doi:10.1021/acs.jafc.6b00909
Trujillo-Reyes, J., Sánchez-Mendieta, V., José Solache-Ríos, M., and Colín-Cruz, A. (2012). Removal of Remazol Yellow from Aqueous Solution Using Fe-Cu and Fe-Ni Nanoscale Oxides and Their Carbonaceous Composites. Environ. Technol. 33 (5), 545–554. doi:10.1080/09593330.2011.584571
Wang, Y.-J., Zhou, D.-M., Sun, R.-J., Cang, L., and Hao, X.-Z. (2006). Cosorption of Zinc and Glyphosate on Two Soils with Different Characteristics. J. Hazard. Mater. 137 (1), 76–82. doi:10.1016/j.jhazmat.2006.02.032
Xiong, Z., Lai, B., Yang, P., Zhou, Y., Wang, J., and Fang, S. (2015). Comparative Study on the Reactivity of Fe/Cu Bimetallic Particles and Zero Valent Iron (ZVI) under Different Conditions of N2, Air or without Aeration. J. Hazard. Mater. 297, 261–268. doi:10.1016/J.JHAZMAT.2015.05.006
Xiong, Z., Yuan, D., Yang, P., and Lai, B. (2017). Cu 2+ Release and Transfer in Various Fe/Cu-Based Processes during Wastewater Treatment. J. Taiwan Inst. Chem. Eng. 80, 669–677. doi:10.1016/J.JTICE.2017.08.048
Yang, Y., Deng, Q., Yan, W., Jing, C., and Zhang, Y. (2018). Comparative Study of Glyphosate Removal on Goethite and Magnetite: Adsorption and Photo-Degradation. Chem. Eng. J. 352, 581–589. doi:10.1016/J.CEJ.2018.07.058
Keywords: organophosphorus pesticides, Cu/Fe bimetallic, glyphosate, reduction, operational factors, toxicity
Citation: Wu X, Wang Y, Xu J and Wang H (2022) Cu/Fe Bimetallic Treatment Performance on Organophosphorus Pesticides. Front. Environ. Sci. 10:915465. doi: 10.3389/fenvs.2022.915465
Received: 08 April 2022; Accepted: 19 May 2022;
Published: 13 June 2022.
Edited by:
Dunia E. Santiago, University of Las Palmas de Gran Canaria, SpainReviewed by:
Oscar Manuel Rodriguez Narvaez, Centro de Innovación Aplicada en Tecnologías Competitivas (CIATEC), MexicoCopyright © 2022 Wu, Wang, Xu and Wang. This is an open-access article distributed under the terms of the Creative Commons Attribution License (CC BY). The use, distribution or reproduction in other forums is permitted, provided the original author(s) and the copyright owner(s) are credited and that the original publication in this journal is cited, in accordance with accepted academic practice. No use, distribution or reproduction is permitted which does not comply with these terms.
*Correspondence: Hongwu Wang, d2FuZ2hvbmd3dUB0b25namkuZWR1LmNu
Disclaimer: All claims expressed in this article are solely those of the authors and do not necessarily represent those of their affiliated organizations, or those of the publisher, the editors and the reviewers. Any product that may be evaluated in this article or claim that may be made by its manufacturer is not guaranteed or endorsed by the publisher.
Research integrity at Frontiers
Learn more about the work of our research integrity team to safeguard the quality of each article we publish.