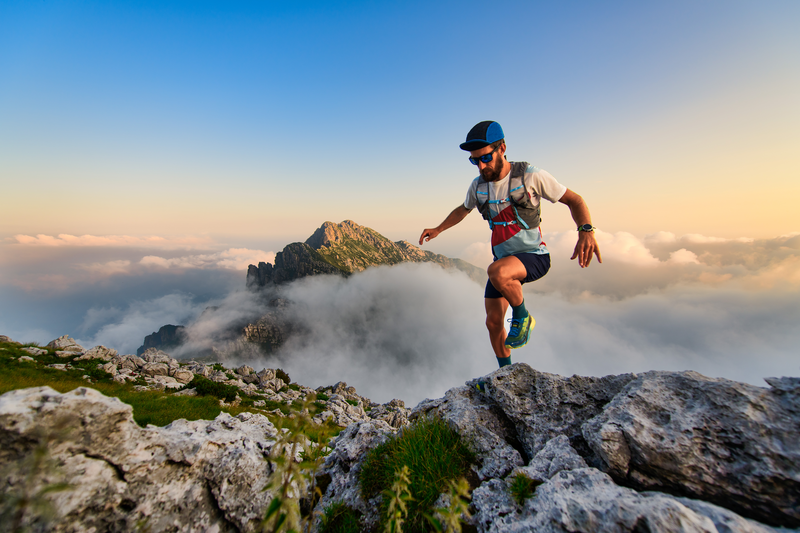
95% of researchers rate our articles as excellent or good
Learn more about the work of our research integrity team to safeguard the quality of each article we publish.
Find out more
ORIGINAL RESEARCH article
Front. Environ. Sci. , 31 August 2022
Sec. Toxicology, Pollution and the Environment
Volume 10 - 2022 | https://doi.org/10.3389/fenvs.2022.913293
This article is part of the Research Topic Biogeochemistry of Metals in Contaminated Environments View all 9 articles
China is the largest antimony resource globally. The mining and smelting of antimony will inevitably have a significant impact on the surrounding and downstream farmlands of the mining area, Therefore, it is necessary to understand the pollution characteristics of antimony and evaluate its potential environmental risks. In this paper, three typical antimony mining areas were used as research districts to analyze the contents of heavy metals in soils of the mining areas and watersheds. The single-factor pollution index method, Nemerow comprehensive pollution index method, geo-accumulation index method, and potential ecological risk index method were used to comprehensively evaluate the pollution status and ecological risk of heavy metals in soils around the mining areas. The soil around the Lengshuijiang tin mine was polluted by As, Cd, Zn, and Sb, it was a heavily polluted soil and at a high ecological risk level. The arable soil around the Nandan tea mountain antimony mine was polluted by As, Cd, Cu, Pb, Sb, and Zn, and it was a heavily polluted soil with very high ecological risk level. The soil around the Xunyang antimony mine was mainly polluted by Sb and it was a moderately polluted soil with moderate ecological risk level. The results revealed that the overall soil heavy metal levels in the Lengshuijiang mining area and the Nandan mining area were in a high-risk state, most likely due to the mining and smelting of metal ores and the weathering of mineralised rocks. showing a high-potential ecological risk in these mining areas. We expect to provide a scientific basis for the safe utilization of farmland and pollution control around the antimony mining areas and watersheds in China.
With the continuous and rapid growth of the demand for mineral resources due to continued economic and social development in China, the development and smelting intensity of mineral resources has also increased, with the accompanying environmental and safety problems becoming ever more prominent. Mining and smelting produce a large amount of wastewater, waste residue, and waste gas; then, these waste products enter the areas surrounding the mine and the farmland in the river watershed through atmospheric deposition, surface runoff, etc., increasing the heavy metal pollution of farmland over time. The heavy metals with levels exceeding government standards not only directly harm the quality and yield of crops around the mining area and watersheds but also endanger human health through the food chain, thereby affecting human safety and survival (Gebel, 1997; Esmaeili et al., 2014; Fu et al., 2016; Herath et al., 2017; Li et al., 2018). The concentration of heavy metals in the soil of smelting area, nearby mining area, mining area and tailing area shows obvious regional distribution characteristics due to different pollution intensities caused by smelting, mining, transportation and stacking (Tang et al., 2019). Antimony and its compounds are considered harmful to human health and even carcinogenic (Gebel, 1997; Jiang et al., 2010). Antimony exposure pathways include inhalation, ingestion and dermal contact, resulting in acute toxic effects on the skin, eyes, lungs, intestines, stomach, liver, kidney, and heart (Chai et al., 2016; Mubarak et al., 2015). Therefore, strengthening the research on heavy metals in farmland soil around the mining area and watersheds is of great practical significance to ensure the safety of the population and properties in the region and to support the ecological health of the environment in the region.
As a country, China is the largest antimony resource globally, with rich ores, large reserves, and high production levels, ranking first in the world (Fan et al., 2004; Gu et al., 2012; Ding et al., 2021). Most of the antimony deposits in China are co-associated, small or medium in size, and are bounded by the Dabie, Qinling, and Kunlun mountain ranges, forming a dense spatial distribution pattern in the south and sparse distribution in the north. By the end of 2017, there were 214 antimony mining areas with proven reserves in China, mainly distributed in seven provinces, namely, Hunan, Guangxi, Tibet, Guizhou, Xinjiang, Yunnan, and Gansu, accounting for approximately 84% of the total reserves in China. According to statistics, there are more than 890 antimony deposits in China (more than 420 are small-scale or greater). The mining and smelting of antimony will inevitably have a significant impact on the surrounding and downstream farmlands of the mining area. In a study, soil samples from 26 different regions in four regions (smelting area, near ore road, mining area, and tailing area) of West Mine were analyzed. The contents of different heavy metals and similar metals (Sb, As, Pb, Cd) were different. In addition, geological accumulation index and potential ecological risk index are used to evaluate ecological risk, the results showed that the heavy metals Sb, As, Pb, and Cd in soil were seriously polluted and had a strong ecological risk, and Sb and Cd were the most important pollution factors (Tang et al., 2019). Some studies have explored the degree and spatial distribution of heavy metal pollution in agricultural soil affected by Sb ore. The data provided were compared with metal concentrations in soil at mining and smelting sites in China and other countries. The results show that the soil is seriously polluted by heavy metals, especially Sb and As. Heavy metal pollution has spread from mining and agricultural activities to the mining area, and Sb is mainly due to mining and smelting activities in the area (Wang et al., 2010a; Luo et al., 2021). Ecological risk levels for all heavy metals in all sampling areas are high (substantial), with Sb, Cd, and A considered to contribute significantly to the risk index (RI) (Long et al., 2017). However, for a long time, the relevant research has mainly focused on lead and zinc mining areas, while the impacts on antimony mining areas have seldomly been studied. In recent years, although some researchers have investigated and analysed soil pollution around antimony mining areas (Liu et al., 2009; He et al., 2012; Shi et al., 2015; Yang et al., 2015; Fei et al., 2017; Chu et al., 2021), nonetheless the related research mainly focuses on a single mining area or a single metallogenic belt and thus has a small research scope. Therefore, the existing research cannot effectively reflect the impact of antimony mining on surrounding areas. In this paper, we selected three typical antimony mining areas (Lengshuijiang tin mining area, Nandan tea mountain antimony mining area, and Xunyang antimony mining area) as the research districts according to the spatial distribution characteristics, metallogenic pattern, and mineral composition of the antimony deposits in China. Additionally, the heavy metal contents in the farmland soils around the mining areas and in the watersheds were analysed. Pollution index methods (single-factor index method, Nemerow comprehensive index method, geo-accumulation index method, and potential ecological risk index method) were used to analyse the pollution status of heavy metals in the farmland soils around the mining areas and in the watersheds, to assess potential ecological hazards. It aims to achieve the following objectives: 1) to understand the heavy metal pollution degree of the three regions according to the national standard measurement method of heavy metal content; 2) to evaluate the environmental quality and potential ecological risk of eight heavy metals in soil geochemical regionalization were by different indexes; 3) to discuss the relationship between different evaluation methods. This study as an example provides relevant information for risk assessment and the safe utilization of farmland and pollution control around the antimony mining areas and watersheds.
The Lengshuijiang tin mine is in Lengshuijiang City, Hunan Province. Lengshuijiang city is located in the middle reaches of zijiang River in central Hunan Province. It lies between E 111°18′57ʺ-- 111°36′40″ and N 27°30′49″-- 27°50′58″. The region has a subtropical humid monsoon climate with distinctive scenery and abundant rainfall in different seasons. The annual sunshine duration is about 1406.8 h, and the frost-free period is about 269 days (Huang et al., 2018). The mine of belongs to the central Hunan-northcentral Guangxi antimony metallogenic belt (Peng et al., 2003a; Wang et al., 2014), is a super-large-scale antimony mining area with the strongest metallogenic intensity in the south and is known as the “Antimony Capital of the World” (Peng et al., 2003a; Wang et al., 2010b). This mining area is divided into two mining regions, the North and the South. The North mining region is dominated by stibnite and cervantite, and the South mining region is dominated by stibnite (Guo et al., 2014). The Nandan tea mountain antimony mining area is in Chehe Town, Nandan County, Hechi City, Guangxi Province. Nandan County (107°01′to 107°55′E, 24°42′to 25°37′N), located in Guangxi, is famous for its production of non-ferrous metals. It has an altitude of 600–900 m above sea level and a typical central Asian mountain plateau climate. The average annual temperature is 16.9°C and the average annual rainfall is about 1,400 mm, most of which occurs in July and August. Nandan county is located in the “Danchi tin polymetallic belt” (Guo et al., 2018). Guangxi is the main mining area. The mine belongs to the Yunnan-Guizhou-Guanxi antimony metallogenic belt (Peng et al., 2003b; Wang et al., 2014) and is a large-scale antimony deposit in southern China, second only to the Lengshuijiang tin mine in terms of metallogenic density and metallogenic intensity. The mine is mainly dominated by stibnite, wolframite, and scheelite (Anderson, 2012; Wang et al., 2014). The Xunyang antimony deposit is in Xunyang County, Shaanxi Province. Xunyang County located between 32°29′–33°13′N and 108°58′–109°48′E, in the South of Shanxi Province, with a total area of 3,550 km2 , was selected as the research area. The climate of the study area is subtropical and humid. The average temperature in Xunyang County is 15.4°C and the average precipitation is 851 mm (Ao et al., 2017). This mine belongs to the Qinling-Central Asia mercury-antimony metallogenic belt (Wang et al., 2014) and is a large-scale antimony mining area in northern China with strong metallogenic intensity. It is divided into the Gongguan antimony mine and Qingtonggou antimony mine and is mainly dominated by cinnabar and stibnite (Anderson, 2012; Wang et al., 2014). The field investigation found that the farmlands around the three mining areas and the regional watersheds were dominated by dry land, primarily planted with corn and sweet potatoes.
According to the distribution of farmland around the mining area and watersheds, large-scale farmland (area ≥ 0.667 ha) was selected as the sampling area. There were nine sampling areas around the Lengshuijiang tin mining area and its watershed (Lianxi River and Zhuxi River watersheds) (Figure 1A), and nine sampling areas around the Nandan tea mountain antimony mining area and its watershed (Sanhe River and Diaojiang watersheds) (Figure 1B). A total of 16 sampling areas were set up around the Xunyang mining area and its watershed (Gongguan River and Shengjia River watersheds) (Figure 1C). Three soil samples were collected in each sampling area. Before collecting the soil samples, surface stones, litter, humus, etc. were removed. Then, the five-point sampling method was used for soil sample collection. The sampling depth was 0–20 cm, and 2 kg of sample was saved by the quartering method, put in a sealing, plastic bag, and taken back to the laboratory. The soil samples were sieved (0.15 mm) after being naturally air-dried and then stored for later use.
FIGURE 1. Study area and spatial distribution of sampling points [(A) Lengshuijiang mining area; (B) Nandan tea mountain mining area; (C) Xunyang mining area].
The soil samples were digested using the national standard method (GB/T22105.2-2008), 0.2–1.0 g of soil samples that had been dried, ground and passed through 0.149 mm aperture sieve were weighed into 50 ml stopper colorimetric tube, moistened with a little water, and 10 ml (1 + 1) aqua regia (3.8) was added, shaken with fruit stopper and dissolved in boiling water bath for 2 h. Shake several times in the middle, remove and cool, dilute with water to scale, shake well and set aside. Absorb a certain amount of digestion test solution into a 50 ml colorimetric tube, add heavy metal corresponding reagent solution, shake well, take the supernatant to be tested. The contents of heavy metals (As, Cd, Cr, Cu, Ni, Pb, Sb, and Zn) in the digested solution were determined by inductively coupled plasma mass spectrometry (ICP-MS) (NexION™350X). The indexing of the instrument can reach ppm level and the accuracy is 10% and the analysis error is about 2%. National standard samples (GSS-5) were also analysed, and blank samples were used for quality control. The reagents used in the analysis were all guaranteed reagent grade.
The single-factor pollution index method (Hu et al., 2018). The calculation formula is as follows: If the value of the index is smaller, the better, the calculation formula is as follows:
In the formula,
The Nemerow comprehensive pollution index method (Zhang et al., 2010), Nemerow comprehensive pollution index method takes into account the average value and the highest value of single factor pollution index, which can be used to evaluate the comprehensive pollution level of heavy metals in each sample point. It is a kind of environmental quality index which is widely used at present. The calculation formula is as follows:
In the formula, PN is Nemerow pollution index; Pmax is the maximum value of soil heavy metal pollution index, and Pave is the average value of soil heavy metal pollution index. Classification standard: PN ≤ 0.7, safe; 0.7 < PN ≤ 1, alert level; 1 < PN ≤ 2, slight pollution; 2 < PN ≤ 3, moderate pollution; PN > 3, severe pollution.
The geo-accumulation index method (Fang et al., 2013), and the potential ecological risk index method (Lars, 1980) were used to evaluate the pollution status of the eight heavy metals (As, Cd, Cr, Cu, Ni, Pb, Sb, and Zn). The calculation formula is as follows:
In the formula, Igeo is the geological accumulation index; Cn is the measured value of soil elements; Bn is the background value of soil elements. This study uses the background value of heavy metal elements in the the sampling regions soil. Classification standard: Igeo < 0, no pollution; 0 < Igeo ≤ 1, mild-moderate pollution; 1 < Igeo ≤ 2, moderate pollution; 2 < Igeo ≤ 3, moderate-intensity pollution; 3 < Igeo ≤ 4, intensity pollution; 4 < Igeo ≤ 5, intensity-extreme intensity pollution; Igeo > 5, extreme intensity pollution.
The potential ecological hazard index method was proposed by Swedish scientists and is widely used in the study of soil environmental ecological risk. This method not only considers the effects of various pollutants in the environment, but also considers the ecological effects, environmental effects and toxicological characteristics of heavy metals (Yang et al., 2021). The calculation formula (Lu and Zhao, 2017) is as follows:
In the formula, RI is the comprehensive potential ecological hazard index; Ei is the potential ecological hazard coefficient of a single element,
The mean, standard deviation, and coefficient of variation were calculated using Excel 2007, and Origin 2018 was used to plot graphs.
In Table 1, the contents of the eight heavy metals (As, Cd, Cr, Cu, Ni, Pb, Sb, and Zn) in the farmland soil of the three mining areas varied significantly. Except for Cr and Ni in the Xunyang mining area, the highest content of other heavy metals were identified in the Nandan tea mountain mining area, then followed by Lengshuijiang and then Xunyang. The farmland soil of the Lengshuijiang mining area exhibited the highest content of Sb, then followed by Zn, As, Pb, Cu, Ni, and Cr, and the Cd content was the lowest. Compared with the soil environmental quality standard, the contents of Sb, As, Cd, and Zn in the soil in the Lengshuijiang mining area are on the high side, which are 53.54, 2.26, 14.07, and 1.77 times of the background value, respectively. In contrast to the Lengshuijiang mining area, the content of As in the farmland of the Nandan tea mountain mining area was the highest, followed by Zn, Sb, Pb, Cu, Cr, and Ni, and the Cd content was the lowest. The content of As, Cd, Cu, Pb, Sb, and Zn in the farmland of the Nandan tea mountain mining area exceeded the standards by factors of 101.91, 52.17, 1.90, 2.70, 77.48, and 8.93 times, respectively. The content of Sb in the farmland soil of the Xunyang mining area was the highest, followed by Zn, Cr, Ni, Cu, Pb, and As, and the content of Cd was the lowest. Compared with the soil environmental quality standard, only the content of Sb in the farmland soil of the Xunyang mining area exceeded the standard, at a value of 15.2 times higher than the standard. The content of these eight heavy metals varied significantly among the three antimony mining regions, and the degree of heavy metal pollution in the farmland soils of each mining areas also varied significantly.
TABLE 1. The concentration (mg kg−1)of eight heavy metals in farmland soils from the three typical antimony mining areas.
The coefficients of variation of the eight heavy metals in the farmland soils of the three mining areas were greatly different, with all exceeding 30% (except for Zn and Ni in the Xunyang mining area). The values of all heavy metals indicated a strong degree of variation, suggesting that the degree of heavy metal pollution in the farmland soils of these three mining areas has been greatly affected by mining-associated anthropogenic activities. The coefficients of variation of the eight heavy metals ranged from highest to lowest in the order of the Lengshuijiang mining area > Nandan tea mountain mining area > Xunyang mining area, which may be closely related to the length of the watershed.
The spatial distribution characteristics of the studied heavy metals in the farmland soils from the different mining areas are shown in Figure 2. The areas with the highest concentrations of the eight heavy metals and the farmland soils with the highest pollution levels of all three mining areas were located near the smelters and tailings and within the same 2-km radius at each site. The content of heavy metals downstream from the mining areas was significantly higher than that of the upstream regions, and the degree of pollution at each river confluence increased. In contrast, at farther distances into the watershed, the degree of pollution gradually decreased.
FIGURE 2. The distribution of heavy metal content in the soil of three typical antimony mining areas. [(A) Lengshuijiang mining area; (B) Nandan tea mountain mining area; (C) Xunyang mining area].
The spatial distribution characteristics of the As, Cr, Cu, Ni, Pb, and Sb in the farmland soil of the Lengshuijiang mining area were similar, and the areas with the highest pollution degree were distributed near the smelter and tailings. The areas with the highest Cd contamination levels were distributed near the smelter; The spatial distribution characteristics of Zn did not change significantly throughout the sampling area (Figure 2A). The spatial distribution characteristics of As, Cr, Cd, Cu, Pb, Sb, and Zn in the farmland soil of the Nandan mining area were similar; the areas with the highest degree of pollution were all near the tailings, and in the study area, the degree of pollution decreased concentrically with distance from the mining area (the centre). The areas with the highest levels of Ni were mainly in the upper reaches of the Diaojiang River (Figure 2B). The areas with the highest pollution levels of As, Cd, Cu, Ni, Pb, Sb, and Zn in the farmland soil of the Xunyang mining area were all in the gold-plating area of the Gongguan antimony mine, while the heavy metal content gradually decreased along the river; the highest degree of pollution of Cr was mainly at the confluence of the Gongguan River and the Shengjia River (Figure 2C).
From Table 2, the single-factor pollution indices of the eight heavy metals (As, Cd, Cr, Cu, Ni, Pb, Sb, and Zn) in the farmland soil of the three mining areas varied significantly among the areas. The value of single-factor pollution index of the heavy metals in the farmland soil in the Lengshuijiang mining area followed the order: Sb > Cd > As > Zn > Pb > Cu > Ni > Cr. The average value of the single-factor pollution indices (Pi) of Cr, Cu, and Ni were between 0 and 1, which belonged to the state of clean; the average values of Pi of Pb and Zn were between 1.03 and 1.77, respectively, which belonged to the state of light pollution; and the average values of Pi of As, Cd, and Sb were all higher than 3, indicating that the soil in this area was mainly polluted by a combination of these three heavy metals. The value of single-factor pollution index of heavy metals in farmland soil in the Nandan mining area followed the order of As > Sb > Cd > Zn > Pb > Cu > Ni > Cr. Except for the heavy metals Cr, Ni, and Cu, the average values of Pi of all other heavy metals were higher than 3, and the average values of Pi of As and Sb were as high as 118.09 and 79.03, respectively, indicating that the main factors of soil pollution in this area were As and Sb. The value of single-factor pollution index of heavy metals in farmland soil in the Nandan mining area and Xunyang mining area followed the order of Sb > Cd > As > Zn > Ni > Cu > Pb > Cr, the average value of Pi of Sb was as high as 12.93, and the average values of Pi of the other heavy metals were between 0 and 1, indicating that the soil of this mining area was mainly polluted by Sb.
TABLE 2. The single factor index and Nemerow index of heavy metals in the soil of three typical antimony mining areas.
Table 2 shows the Nemerow pollution index (PN) of all sampling sites. the Nemerow pollution index (PN) of Lengshuijiang mining area was higher than 3.0, indicating that the region was severe pollution; in addition, the PN near the mine (sampling site No. 4) was the highest. At this mine, the PN value first decreased and then increased along the Zhuxi River watershed (sampling site Nos 1–3), while along the Lianxi River (sampling site Nos 6–9), the PN value first increased and then decreased. The Nemerow pollution index (PN) of Nandan mining area shows that except for sampling site No. 4 in the Nandan mining area, the PN of the other sampling sites was higher than 3.0, indicating that the region also was severe pollution. However, along the Sanhe River watershed (sampling site Nos. 2, 1, 5, 8, and 9) and the Diaojiang River watershed (Nos. 3, 4, and 7), the soil PN in the arable land in this mining area gradually decreased. The Nemerow pollution index (PN) of Xunyang mining area showed that except for sampling site Nos. 3, 8, and 15 in the Xunyang mining area, the PN values of the other sampling sites were also higher than 3.0, indicating that there was severe pollution. Along the Shengjia River watershed (sampling sites Nos 9-10, 13–16) and Gongguan River watershed (sampling sites Nos 1–8), the PN values of the soil in the arable land decreased firstly and then increased, with the highest PN value reached at the confluence of the rivers (sampling site No. 13). The results of evaluation using the Nemerow pollution index method were severe pollution.
Table 3 demonstrated the geo-accumulation index of all sampling sites in three area. It can be seen that in the Lengshuijiang mining area, the average geo-accumulation index (Igeo) of Sb, Cd, and As were 4.83, 2.70, and 0.67, respectively, while the average Igeo values of the other heavy metals were all less than 0, indicating that the arable soil around the mine was mainly affected by Sb. In the Nandan mining area, except for the average Igeo values of Cr and Ni, the average Igeo values of the other heavy metals were all higher than 1.0, and the average Igeo values of Sb, As, and Cd reached 4.77, 4.63, and 4.56, respectively, indicating that this mining area was severely polluted by these heavy metals. In the Xunyang mining area, except for Sb with the average Igeo value of 2.74, the average Igeo values of the other heavy metals were less than 0, which indicated that the arable soil around the Xunyang mining area was mainly polluted by Sb, and the degree of pollution was moderate to high.
TABLE 3. The geoaccumulation index of soil heavy metals in three typical antimony mining areas tested.
From Table 4, the potential ecological risk levels in the soil of the Lengshuijiang mining area followed the order of Cd > Sb > As > Pb > Ni > Cu > Zn > Cr. Among them, Cd and Sb exhibited extremely high potential risks, and the value of comprehensive potential ecological risk (RI) reached up to 852.12, indicating that there was a high potential ecological risk of heavy metal pollution in the soil of the Lengshuijiang mining area. The potential ecological risks in the soil of the Nandan mining area followed the order of Cd > As > Sb > Pb > Cu > Zn > Ni > Cr, and heavy metal elements As, Cd, and Sb exhibited extremely high potential risks. In addition, RI value of Nandan mining area was 3240.52, indicating a very high potential ecological risk, and the soil of this mining area showed the highest potential ecological risk among the three mining areas. The potential ecological risks in the soil of the Xunyang mining area followed the order of Sb > Cd > As > Ni > Pb > Cu > Cr > Zn, and only Sb was found to have a high potential risk. The RI value of this mining area was 175.58, indicating a medium potential ecological risk.
TABLE 4. The potential risk index and compound ecological risk index of soil heavy metals in three typical antimony mining areas.
Mining and smelting consistently lead to heavy metal pollution in the surrounding and downstream soil of the mining area, but the degree of pollution is closely related to the distance from the pollution source. This study found that the degree of heavy metal pollution in the surrounding farmlands and areas downstream of the three mining areas was not only closely related to the distance from the pollution source but was also affected by factors such as ore composition, climate, mining time, and smelting method. Studies have explored differences in metal and metalloid concentrations in samples from different sites due to spatial variability. The results show that the second factor (PC2) dominated by As, Cd, Cu, Pb, Sb, and Zn, which are related to smelting and mining activities. These metals and metalloid may have been discharged by smelters, suspended in mining tailings, and discharged during mineral processing (Zhong et al., 2016; Guo et al., 2018).
Due to the different main components of the ores in the three mining areas, the specific heavy metal elements that cause pollution in the soil around each mining area and downstream farmland and the degree of pollution varied. The Lengshuijiang mining area and the Nandan mining area belong to different antimony metallogenic belts (the Lengshuijiang mining area belongs to the central Hunan-northcentral Guangxi antimony metallogenic belt, and the Nandan tea mountain mining area belongs to the Yunnan-Guizhou-Guangxi antimony metallogenic belt; the farmlands in both mining areas are polluted by a combination of As, Cd, Sb, and Zn (Wang et al., 2014), even though their main components are all stibnite (Sb2S3) and stibnite contains 71.4% Sb and a small amount of As, Pb, Ag, Cu, Fe, etc. In addition to stibnite (Sb2S3), the main components of the ore in the Nandan mining area are scheelite (CaWO4) and wolframite (FeWO4 and MnWO4). Since Ca in tungsten ore can be replaced by Cu in the form of isomorphism, the soil around the Nandan mining area and downstream farmland was also polluted by Cu (Guo et al., 2014; Yang and Wu, 2014; Ye et al., 2019). The Xunyang mining area is different from the Lengshuijiang mining area and the Nandan mining area. The main components of the ore are cinnabar (HgS) and stibnite. Since Hg was not determined, the soil around the mining area and downstream farmland were mainly polluted by Sb.
The Lengshuijiang mining area, Nandan mining area, and Xunyang mining area all mainly adopt the pyrometallurgical process (Erusalimskii, 1971; Feng et al., 2003; He, 2007). The Lengshuijiang mining area has existed for 117 years, since the establishment of the official mining bureau in 1905, and has had a long-term impact on the heavy metal pollution of the soil in its vicinity. The Nandan mining area has a history of nearly 50 years since its establishment in 1973 and has profoundly impacted the heavy metal pollution of the soil around the mining area. The Xunyang mining area is 37 years old, dating to when the Xunyang mercury antimony mine in Shaanxi Province was established in 1985 for simple production. This mine has a far-reaching impact on the heavy metal pollution of the soil around it. Due to the antimony ore in smelting ore after combustion is easy to concentrate in the dust, through the atmospheric dust accumulation in the soil, so the longer the mining time, the greater the impact of heavy metal pollution on the soil around the mining area (Li et al., 2013; Liang et al., 2013; Sheng et al., 2022). However, because the ore composition of the Nandan mining area is more complex than that of the Lengshuijiang mining area, Pb and Cu pollution are also present, compared with the similar As, Cd, Sb, and Zn pollution at Lengshuijiang.
At the same time, there is a significant difference in rainfall between northern China and southern China. The North is dry with low rainfall, while the South is humid with high rainfall. Teutsch et al. (1999) found that differences in rainfall have an important influence on the geochemical behaviour of heavy metals in soil. Metal elements such as Cu, Pb, Zn, and As, are positively correlated with rainfall, such that the greater the rainfall, the greater the migration (Zhang et al., 2015; Xue et al., 2020). Yi et al. (2008) also proved that heavy metals are easily enriched in places with heavy precipitation.
Therefore, the Sb and As pollution in the Lengshuijiang mining area and the Sb, As, Cu, and Pb pollution in the Nandan mining area are probably caused by antimony smelting (Long et al., 2020). In slight variation from previous research results, the levels of Cu and Pb heavy metals in the Lengshuijiang mining area did not exceed the standards, which may be related to the fact that many plants are distributed around the mining area and there are obvious traces of phytoremediation. As a result, the pollution of the Lengshuijiang mining area was lower than that of the Nandan mining area. The Xunyang mining area was mainly polluted by Sb, and the other heavy metal pollutants were not of significant levels. First, the ore composition of the Xunyang mining area is different from the ore composition of the other two mining areas, Xunyang County is rich in mineral resources. Currently, it more than 39 kinds of mineral resources, including mercury, lead, zinc, gold, copper, tin, manganese, magnesium, limestone, and dolomite ores. Among them, mercury, Hg, Au, and Pb–Zn ore are the main deposits (Tang and Zhao, 2015). And second, the findings are related to the low rainfall and thus low heavy metal migration of this area.
In this study, according to the single-factor pollution index method and the Nemerow comprehensive pollution index method, the three mining areas were all severely polluted by heavy metals. Among them, Cd and Sb were the main contributors in the Lengshuijiang tin mine; Sb was the main contributor in the Xunyang antimony mine; and As, Cd, Sb, and Zn were the main contributors in the Nandan tea mountain antimony mine. The soil pollution in the three mining areas was the most severe near the smelter or near the tailings.
According to the evaluation using the potential ecological risk index method, the three mining areas all had a certain degree of potential ecological risk. The Lengshuijiang tin mine had a high potential ecological risk, while there was a moderate potential ecological risk in the Xunyang antimony mining area. In addition, there were certain similarities in the potential risk levels of these three mining areas; that is, the pollution level gradually decreased from upstream to downstream along the river watershed and increased to some extent at the river confluence. Heavy metals in the environment are often interrelated in complex ways. The high correlation between the parameters may mean that they come from similar sources of pollution (Shou et al., 2012). However, a study has been shown that the sources of soil pollution are complex among several heavy metals because some heavy metals are not related to each other. Therefore, it is difficult to protect soil from heavy metal pollution (Zhu et al., 2018). The above findings indicate that the mining of antimony ore will cause heavy metals to enter the soil; the closer the farmland to the mining area, the higher the heavy metal content, and the more serious the pollution (Yao et al., 2021; Hu et al., 2022).
According to the evaluation using the geo-accumulation index method, the Lengshuijiang tin mine had composite Cd and Sb pollution and was classified as severe pollution; Nandan tea mountain antimony mine had composite Sb, As, and Cd pollution and was classified as extremely severe pollution; and the Xunyang antimony mine had pollution from only Sb and was classified as moderately polluted. In addition, the average Igeo values of the three mining areas decreased gradually along the river watershed from upstream to downstream and increased at the confluence of the rivers. The research results of many scholars (Yao et al., 2021; Hu et al., 2022) showed that the potential ecological risk level of heavy metals in the riparian soil of the river watershed increased considerably from the upstream to the downstream areas, which varied from the results of this study. The reason may be that the sampling sites were centred on smelters and tailings. However, within the same radius, the degree of soil heavy metal pollution in the upstream and downstream farmlands was low, which was consistent with the results of previous studies.
In summary, the most serious soil pollution occurred near the smelter or near the tailings in the Lengshuijiang tin mine, the Nandan mining area, and Xunyang antimony mine, and the degree of pollution gradually decreased from upstream to downstream along the river watershed. Additionally, the degree of pollution increased to some extent at the river confluence point. At the same time, the heavy metal content and degree of pollution of the soil around the mining areas were related to the distance from each smelter; that is, taking the smelter and tailings as the centre points, the heavy metal content and the degree of pollution decreased concentrically from the centre into the surrounding areas.
The original contributions presented in the study are included in the article/supplementary material, further inquiries can be directed to the corresponding authors.
MZ: Conceptualization, methodology, software, investigation, formal analysis, data curation, writing-original draft. WP: Visualization, investigation. CJ and JW: Resources, supervision. WL: Writing-Review and editing. XW: Conceptualization, funding acquisition, resources, supervision, writing-review and editing. YQ: Software, investigation, formal analysis, data curation, writing-draft, revison.
The authors declare that the research was conducted in the absence of any commercial or financial relationships that could be construed as a potential conflict of interest.
All claims expressed in this article are solely those of the authors and do not necessarily represent those of their affiliated organizations, or those of the publisher, the editors and the reviewers. Any product that may be evaluated in this article, or claim that may be made by its manufacturer, is not guaranteed or endorsed by the publisher.
Anderson, C. G. (2012). The metallurgy of antimony. Geochemistry 72, 3–8. doi:10.1016/j.chemer.2012.04.001
Ao, M., Meng, B., Sapkota, A., Wu, Y., Qian, X., Qiu, G., et al. (2017). The influence of atmospheric Hg on Hg contaminations in rice and paddy soil in the Xunyang Hg mining district, China. Acta Geochim. 36, 181–189. doi:10.1007/s11631-017-0142-x
Chai, L., Mubarak, H., Yang, Z., Yong, W., Tang, C., Mirza, N., et al. (2016). Growth, photosynthesis, and defense mechanism of antimony (Sb)-contaminated Boehmeria nivea L. Environ. Sci. Pollut. R 23 (8), 7470–7481. doi:10.1007/s11356-015-5987-0
Chu, J., Hu, X., Kong, L., Wang, N., Zhang, S., He, M., et al. (2021). Dynamic flow and pollution of antimony from polyethylene terephthalate (PET) fibers in China. Sci. Total Environ. 771, 144643. doi:10.1016/j.scitotenv.2020.144643
Ding, J., Zhang, Y., Ma, Y., Wang, Y., Zhang, J., and Zhang, T. (2021). Metallogenic characteristics and resource potential of antimony in China. J. Geochem. Explor. 230, 106834. doi:10.1016/j.gexplo.2021.106834
Erusalimskii, E. I. (1971). The technology of antimony production and improving working conditions from 1966 to 1969. Sov. Zdr. Kirg. 6, 42–47.
Esmaeili, A., Moore, F., Keshavarzi, B., Jaafarzadeh, N., and Kermani, M. (2014). A geochemical survey of heavy metals in agricultural and background soils of the Isfahan industrial zone, Iran. Catena 121, 88–98. doi:10.1016/j.catena.2014.05.003
Fan, D. L., Zhang, T., and Ye, J. (2004). The Xikuangshan Sb deposit hosted by the Upper Devonian black shale series, Hunan, China. Ore Geol. Rev. 24 (1-2), 121–133. doi:10.1016/j.oregeorev.2003.08.005
Fang, C., Qu, Z., Wang, D., Wang, J., and Bi, L. (2013). Comparative study of geo-accumulation index and enrichment factor in source apportionment of atmospheric particulate matter. Adv. Mat. Res. 634-638, 72–75. Pts 1-5. doi:10.4028/www.scientific.net/AMR.634-638.72
Fei, J., Min, X., Wang, Z., Pang, Z., Liang, Y., and Ke, Y. (2017). Health and ecological risk assessment of heavy metals pollution in an antimony mining region: A case study from south China. Environ. Sci. Pollut. Res. 24 (35), 27573–27586. doi:10.1007/s11356-017-0310-x
Feng, X., Tang, S., Shang, L., Yan, H., Sommar, J., and Lindqvist, O. (2003). Total gaseous mercury in the atmosphere of Guiyang, PR China. Sci. Total Environ. 304 (1-3), 61–72. doi:10.1016/S0048-9697(02)00557-0
Fu, Z., Wu, F., Mo, C., Deng, Q., Meng, W., and Giesy, J. P. (2016). Comparison of arsenic and antimony biogeochemical behavior in water, soil and tailings from Xikuangshan, China. Sci. Total Environ. 539, 97–104. doi:10.1016/j.scitotenv.2015.08.146
Gebel, T. (1997). Arsenic and antimony: Comparative approach on mechanistic toxicology. Chem. Biol. Interact. 107 (3), 131–144. doi:10.1016/S0009-2797(97)00087-2
Gu, X., Zhang, Y., Schulz, O., Vavtar, F., Liu, J., Zheng, M., et al. (2012). The Woxi W-Sb-Au deposit in Hunan, South China: An example of Late Proterozoic sedimentary exhalative (SEDEX) mineralization. J. Asian Earth Sci. 57, 54–75. doi:10.1016/j.jseaes.2012.06.006
Guo, G., Song, B., Xia, D., Yang, Z., and Wang, F. (2018). Metals and metalloids in PM10 in Nandan County, Guangxi, China, and the health risks posed. Environ. Geochem. Health 40 (5), 2071–2086. doi:10.1007/s10653-018-0083-2
Guo, X., Wang, K., He, M., Liu, Z., Yang, H., and Li, S. (2014). Antimony smelting process generating solid wastes and dust: Characterization and leaching behaviors. J. Environ. Sci. 26 (7), 1549–1556. doi:10.1016/j.jes.2014.05.022
He, M. (2007). Distribution and phytoavailability of antimony at an antimony mining and smelting area, Hunan, China. Environ. Geochem. Health 29 (3), 209–219. doi:10.1007/s10653-006-9066-9
He, M., Wang, X., Wu, F., and Fu, Z. (2012). Antimony pollution in China. Sci. Total Environ. 421-422, 41–50. doi:10.1016/j.scitotenv.2011.06.009
Herath, I., Vithanage, M., and Bundschuh, J. (2017). Antimony as a global dilemma: Geochemistry, mobility, fate and transport. Environ. Pollut. 223, 545–559. doi:10.1016/j.envpol.2017.01.057
Hu, R., Wang, Y., Zhang, X., Zhu, L., Luo, M., Liu, B., et al. (2022). Sources and factors controlling the distribution of heavy metals in coastal sediments of Haiyang, China. Mar. Pollut. Bull. 175, 113152. doi:10.1016/j.marpolbul.2021.113152
Hu, Z., Hu, L., Cheng, H., and Qi, D. (2018). Application of pollution index method based on dynamic combination weight to water quality evaluation. 2018 2nd Int. Workshop Renew. Energy Dev. 2018, 153. doi:10.1088/1755-1315/153/6/062008
Huang, C., Huang, P., Wang, X., and Zhou, Z. (2018). Assessment and optimization of green space for urban transformation in resources-based city - A case study of Lengshuijiang city, China, 30, 295–306. doi:10.1016/j.ufug.2017.12.016URBAN URBAN GREE
Jiang, X., Wen, S., and Xiang, G. (2010). Cloud point extraction combined with electrothermal atomic absorption spectrometry for the speciation of antimony(III) and antimony(V) in food packaging materials. J. Hazard Mater. 175 (1–3), 146–150. doi:10.1016/j.jhazmat.2009.09.141
Lars, H. (1980). An ecological risk index for aquatic pollution control.a sedimentological approach. Water Res. 14 (8), 975–1001. doi:10.1016/0043-1354(80)90143-8
Li, J., Zheng, B., He, Y., Zhou, Y., Chen, X., Ruan, S., et al. (2018). Antimony contamination, consequences and removal techniques: A review. Ecotoxicol. Environ. Saf. 156, 125–134. doi:10.1016/j.ecoenv.2018.03.024
Li, Z., Feng, X., Bi, X., Sun, G., Zhu, J., Qin, H., et al. (2013). Heavy metals in the ground surface dust and agricultural soil in artisanal and medium-scale zinc smelting area in northwest Guizhou province, China. E3S Web Conf. 1, 19004. doi:10.1051/e3sconf/20130119004
Liang, S., Li, X., Xu, H., Wang, X., and Gao, N. (2013). Spatial-based assessment of heavy metal contamination in agricultural soils surrounding a non-ferrous metal smelting zone. Bull. Environ. Contam. Toxicol. 91 (5), 526–532. doi:10.1007/s00128-013-1110-8
Liu, B., Wu, F., Deng, Q., Mo, C., Zhu, J., Zeng, L., et al. (2009). Pollution characteristics of antimony, arsenic and mercury in human hair at xikuangshan antimony mining area and guiyang city, China. Huanjing Kexue 30 (3), 907–912. (in Chinese).
Long, H., Zheng, Y., Peng, Y., Jin, G., Deng, W., Zhang, S., et al. (2020). Separation and recovery of arsenic and alkali products during the treatment of antimony smelting residues. Min. Eng. 153, 106379. doi:10.1016/j.mineng.2020.106379
Long, J., Tan, D., Deng, S., and Lei, M. (2017). Pollution and ecological risk assessment of antimony and other heavy metals in soils from the world's largest antimony mine area, China. Hum. Ecol. Risk Assess. An Int. J. 24 (3), 679–690. doi:10.1080/10807039.2017.1396531
Lu, J., and Zhao, X. (2017). Characteristics and ecological risk assessment of polluted soil by heavy metals in Shizishan, Tongling. Environ. Chem. 36 (9), 1958–1967. doi:10.7524/j.issn.0254-6108.2017010304
Luo, G., Han, Z., Xiong, J ., He, Y., Liao, J., and Wu, P. (2021). Heavy metal pollution and ecological risk assessment of tailings in the Qinglong Dachang antimony mine, China. Environ. Sci. Pollut. Res. 28 (25), 33491–33504. doi:10.1007/s11356-021-12987-7
Mubarak, H., Chai, L., Mirza, N ., Yang, Z., Pervez, A., Tariq, M., et al. (2015). Antimony (Sb) - pollution and removal techniques - critical assessment of technologies. Toxicol. Environ. Chem. 97 (10), 1296–1318. doi:10.1080/02772248.2015.1095549
Peng, J. T., Hu, R. Z., and Burnard, P. G. (2003a). Samarium-neodymium isotope systematics of hydrothermal calcites from the xikuangshan antimony deposit (hunan, China): The potential of calcite as a geochronometer. Chem. Geol. 200 (1-2), 129–136. doi:10.1016/S0009-2541(03)00187-6
Peng, J. T., Hu, R. Z., and Jiang, G. H. (2003b). Samarium-Neodymium isotope system of fluorites from the Qinglong antimony deposit, Guizhou Province: Constraints on the mineralizing age and ore-forming materials' sources. Acta Pet. Sin. 19 (4), 785–791. doi:10.1016/S0955-2219(02)00073-0
Sheng, L., Hao, C., Guan, S., and Huang, Z. (2022). Spatial distribution, geochemical behaviors and risk assessment of antimony in rivers around the antimony mine of Xikuangshan, Hunan Province, China. Water Sci. Technol. 85 (4), 1141–1154. doi:10.2166/wst.2022.030
Shi, T., Zhang, L., Cao, Y., and Wang, S. (2015). Antimony distribution and effect factors in the surface sediment of nansi lake, China. Fresen Environ. Bull. 24 (11C), 4157–4164.
Shou, Z., Feng, C., Yang, Y., Niu, J., and Shen, Z. (2012). Risk assessment of sedimentary metals in the yangtze estuary: New evidence of the relationships between two typical index methods. J. Hazard. Mat. 241, 164–172. doi:10.1016/j.jhazmat.2012.09.023
Tang, B., and Zhao, X. (2015). Analysis of the influence of heavy metal pollution in the Pb–Zn mining area on environment ecology. Metall. Min. Ind. 8, 86–93.
Tang, Z., Deng, R., Zhang, J., Ren, B., and Hursthouse, A. (2019). Regional distribution characteristics and ecological risk assessment of heavy metal pollution of different land use in an antimony mining area - xikuangshan, China. Hum. Ecol. Risk Assess. An Int. J. 26 (7), 1779–1794. doi:10.1080/10807039.2019.1608423
Teutsch, N., Erel, Y., Halicz, L., and Chadwick, O. A. (1999). The influence of rainfall on metal concentration and behavior in the soil. Geochim. Cosmochim. Acta 63 (21), 3499–3511. doi:10.1016/S0016-7037(99)00152-0
Wang, X., He, M. C., Lu, X., and Xi, J. (2010b). Arsenic distributions in rivers around the world largest antimony mine of Xikuangshan in Hunan province of China. Arsenic Geosph. Hum. Dis. 97 (1), 50–51. doi:10.1016/j.microc.2010.05.011
Wang, X., He, M., Xie, J ., Xi, J., and Lu, X. (2010a). Heavy metal pollution of the world largest antimony mine-affected agricultural soils in Hunan province (China). J. Soils Sediments 10 (5), 827–837. doi:10.1007/s11368-010-0196-4
Wang, Y., Xu, J., Zhang, C., Wang, C., Chen, Z., and Huang, F. (2014). Summary of metallogenic regularities of antimony deposits in China. Dizhi Xuebao 88 (12), 2208–2215. (in Chinese).
Xue, H., Zhao, L., and Liu, X. (2020). Characteristics of heavy metal pollution in road runoff in the Nanjing urban area, East China. Water Sci. Technol. 81 (9), 1961–1971. doi:10.2166/wst.2020.249
Yang, H., He, M., and Wang, X. (2015). Concentration and speciation of antimony and arsenic in soil profiles around the world's largest antimony metallurgical area in China. Environ. Geochem. Health 37 (1), 21–33. doi:10.1007/s10653-014-9627-2
Yang, H., Xu, H ., and Zhong, X. (2021). Study on the hyperspectral retrieval and ecological risk assessment of soil Cr, Ni, Zn heavy metals in tailings area. Bull. Environ. Contam. Toxicol. 108 (4), 745–755. doi:10.1007/s00128-021-03383-5
Yang, J., and Wu, Y. (2014). A hydrometallurgical process for the separation and recovery of antimony. Hydrometallurgy 143, 68–74. doi:10.1016/j.hydromet.2014.01.002
Yao, W., Hu, C., Yang, X., and Shui, B. (2021). Spatial variations and potential risks of heavy metals in sediments of Yueqing Bay, China. Mar. Pollut. Bull. 173, 112983. doi:10.1016/j.marpolbul.2021.112983
Ye, L., Ouyang, Z., Chen, Y., Wang, H., Xiao, L., and Liu, S. (2019). Selective separation of antimony from a Sb-Fe mixed solution by hydrolysis and application in the hydrometallurgical process of antimony extraction. Sep. Purif. Technol. 228, 115753. doi:10.1016/j.seppur.2019.115753
Yi, Y., Wang, Z., Zhang, K., Yu, G., and Duan, X. (2008). Sediment pollution and its effect on fish through food chain in the Yangtze River. Int. J. Sediment Res. 23 (4), 338–347. doi:10.1016/S1001-6279(09)60005-6
Zhang, C., Zhang, C., Gao, Z., and Wu, Z. (2015). Pollution feature analysis on heavy metals in rainfall runoff of Qingdao residential area. Proc. 2015 Int. Forum Energy, Environ. Sci. Mater. 40, 1244–1249. doi:10.2991/ifeesm-15.2015.228
Zhang, J., Wang, J., Chen, D., and Li, D. (2010). Assess vegetable field soil heavy metal pollution in dagu river watershed. Conf. Environ. Pollut. Public Health 1-2, 740.
Zhong, X., Yu, Y., Lu, S., Yang, Z., Kang, K., Kuang, H., et al. (2016). Evaluation of heavy metal contamination in soils in mining-intensive areas of Nandan, Guangxi. J. Agro-Environment Sci. 35, 1694–1702.
Keywords: soil, heavy metals, pollution characteristics, ecological risk, antimony mining area
Citation: Qi Y, Wei X, Zhao M, Pan W, Jiang C, Wu J and Li W (2022) Heavy metal pollution characteristics and potential ecological risk assessment of soils around three typical antimony mining areas and watersheds in China. Front. Environ. Sci. 10:913293. doi: 10.3389/fenvs.2022.913293
Received: 05 April 2022; Accepted: 01 August 2022;
Published: 31 August 2022.
Edited by:
Joginder Singh, Lovely Professional University, IndiaReviewed by:
Vineet Kumar, National Environmental Engineering Research Institute (CSIR), IndiaCopyright © 2022 Qi, Wei, Zhao, Pan, Jiang, Wu and Li. This is an open-access article distributed under the terms of the Creative Commons Attribution License (CC BY). The use, distribution or reproduction in other forums is permitted, provided the original author(s) and the copyright owner(s) are credited and that the original publication in this journal is cited, in accordance with accepted academic practice. No use, distribution or reproduction is permitted which does not comply with these terms.
*Correspondence: Xiangdong Wei, eGlhbmdkb25nd0AxMjYuY29t; WaiChin Li, d2FpY2hpbkBlZHVoay5oaw==
Disclaimer: All claims expressed in this article are solely those of the authors and do not necessarily represent those of their affiliated organizations, or those of the publisher, the editors and the reviewers. Any product that may be evaluated in this article or claim that may be made by its manufacturer is not guaranteed or endorsed by the publisher.
Research integrity at Frontiers
Learn more about the work of our research integrity team to safeguard the quality of each article we publish.