- Laboratory of Soil Microbiology & Calorimetry, Corporación Colombiana de Investigación Agropecuaria Agrosavia, Centro de Investigación Tibaitatá Km 14 Vía Bogotá-Mosquera, Mosquera, Colombia
In cacao farms, the presence of cadmium (Cd) is a major issue for commercialization, particularly for countries such as Colombia. Cadmium-tolerant bacteria (CdtB) are an important functional group of microorganisms with a potential for bioremediation strategies. Cd immobilization activity by CdtB can be accurately measured by isothermal microcalorimetry (IMC). In this study, the metabolic capacity of an entire CdtB community in cacao farm soils from three cacao-producing districts of Colombia, with and without the addition of Cd was measured using IMC. The differences between the observed peaks in metabolic activity related to Cd immobilization were analysed to determine whether activation of CdtB populations occurred when Cd content was increased. The thermograms from Santander soil samples have a major metabolic activity of the CdtB community compared to peaks of maximal heat-flow in the samples from Antioquia and Arauca. IMC showed differences in Cd immobilization ratios of the soil samples of 0.11–0.30 mg kg−1 h−1 at 25°C over 12 days of thermal monitoring. Furthermore, the amplicons of cadA and smt genes from the soil samples allow elucidation of possible metabolic mechanisms used by CdtB soil populations. The gene amplification confirmed the existence of CdtB populations related to both bioweathering and biochelating metabolic capacities. Scanning electron microscope (SEM) images supported the existence of otavite biologically induced by CdtB naturally. A Pearson correlation analysis was made between kinetical growth parameters and thermodynamic data. Besides, a PCA was performed between CdtB cadA gene copies, soil pH and SOM indicating the effect of CdtB in Cd translocation. Thus, it is concluded that the combination of Cd immobilization ratios obtained using isothermal microcalorimetry, the molecular basis of metabolic immobilization, and SEM imagery could act as a useful toolkit to identify CdtB populations for bioremediation strategies in contaminated cacao farms. The research importance of this study is the use of combined tools for quantitative IMC measurements to identify and assess Cd metabolic capacities of CdtB populations in soil, in situ, as a new proxy for CdtB assessment in cacao-growing soils.
1 Introduction
Cadmium (Cd) is a non-essential element for almost all life systems, including humans, plants and some microorganisms (Himeno and Aoshima, 2019; Satarug, 2019). It is considered a heavy metal because is an element that have a high atomic weight and a density at least 5 times greater than that of water (Tchounwou et al., 2012) and due to its toxicity that imposes a human health risk particularly when it enters into the food chain. The European Union (EU) introduced a regulation in 2019 that indicates maximum levels of Cd in chocolates and cocoa-derivatives. This regulation can negatively affect the cacao exportations of producer countries, including Colombia, where some hotspots of high Cd content have been found in some cacao producing areas (Bravo et al., 2021). Therefore, several mechanisms of remediation are being studied to minimize Cd content throughout the cacao production chain. One understudied remediation strategy is bioremediation using Cd-tolerant bacteria (CdtB). This is an important strategy as the bioavailability and mobility of heavy metals in soils and sediments are controlled by their adsorption in soils through components such as microorganisms (Groenenberg and Lofts, 2014). Table 1 summarizes some studies using bacteria for soil bioremediation techniques.
CdtB are a non-phylogenetically related group of bacteria with the exceptional capacity of metabolize Cd and using it as source of energy (Bravo and Braissant, 2022). Their metabolic capacity may vary depending on the soil type, the soil Cd content as well as other soil characteristics (Bravo et al., 2018). The co-metabolism has been noted in this functional group, because the expression of Cd immobilization capacity is also related to P and K solubilization capacities. This has been highlighted in previous studies with CdtB isolated from cacao-growing farms in Colombia (Bravo et al., 2018). However, to date, the metabolic activity of these soil bacterial populations has not been assessed in situ in relation to the bioweathering of Cd into non-soluble forms such as secondary otavite.
The isothermal microcalorimetry method (IMC), is a sensitive and non-destructive approach to assess bacterial metabolic activity (Braissant et al., 2010) and could be useful to monitor the metabolic ratios of Cd immobilization in soil. This technique, in combination with methods to determine viability, vitality and metabolic rates of microorganisms could be an excellent proxy to assess overall Cd metabolism (Bravo et al., 2018; Braissant et al., 2020). Besides, the amplification of genes related to Cd immobilization is a first step to know if CdtB population are present in the bacterial community. The CadA and smt genes have been highlighted as an important resource to estimate the presence of CdtB (Bravo and Braissant, 2022).
In previous studies in Central and South America (Chavez et al., 2015; Gramlich et al., 2018; Engbersen et al., 2019; Ramtahal et al., 2019), including Colombia (Gil et al., 2022), physical and chemical soil parameters, such as soil pH, soil organic matter (SOM) and the Cd values in soils have been identified as having a major influence on the final content of Cd in cacao beans. However, the role in situ of CdtB in translocation rates of the metal from soil to crop hast not been studied.
This study aims to compare the IMC thermograms of CdtB in soils with 1) a molecular tool, the PCR amplification of genes CadA and smt, related to Cd immobilization of the soils used; 2) a visualization tool, the scanning electron microscope (SEM) imagery of the soil samples assessed, and 3) the use of reference CdtB strains to compare the Cd tolerance with each method. Moreover, the inclusion of soil parameters such as soil pH, SOM, average soil Cd values and average cacao bean Cd values, will allow an increase in our understanding on the role of CdtB with the Cd translocation from soils to the cacao beans, from which chocolate is made.
2 Materials and Methods
2.1 Study Area
The soil samples were collected from cacao-growing farms located in three cacao districts in Colombia. The districts were Antioquia, Arauca and Santander. The location districts were selected due to their high production and the economic importance of cacao in Colombia. Figure 1 shows the location of sampling sites. Each sample consisted of a composite sample made by mixing soil samples from 20 farms within each of the three districts. The soil type from Antioquia farms were classified as soils belong to the subgroup Oxic Dystrudepts of Inceptisols (Gil et al., 2022). The soil type of cacao farms sampled from Arauca were classified as Typic Endoaquepts of Inceptisols (Bravo and Benavides-Erazo, 2020). Moreover, the soil type observed in the 20 farms assessed in Santander district were classified as Entisols (Bravo et al., 2018). Two kg of composite samples were formed by collecting 0.4 kg of soil in a zip bag taken at 30 cm of soil depth and at 70 cm around five cacao trunks, distributed in zig-zag, in the centre of the farm. Quantification of Cd soil content and Cd in beans was conducted previously in these farms (Bravo et al., 2021). One set of soil samples was measured with ‘natural Cd content’ and the other set of the same samples was ‘spiked’ with 1 mg kg−1 of CdCl2. This is necessary in order to exhibit increased metabolic capacities of CdtB in soil samples, especially for IMC measurements. The samples were collected and transported and the assays were done at the Tibaitatá Research Centre of the Corporación Colombiana de Investigacion Agropecuaria–Agrosavia, in Mosquera, Colombia. The soil samples from each location were used for several experimental approaches detailed below.
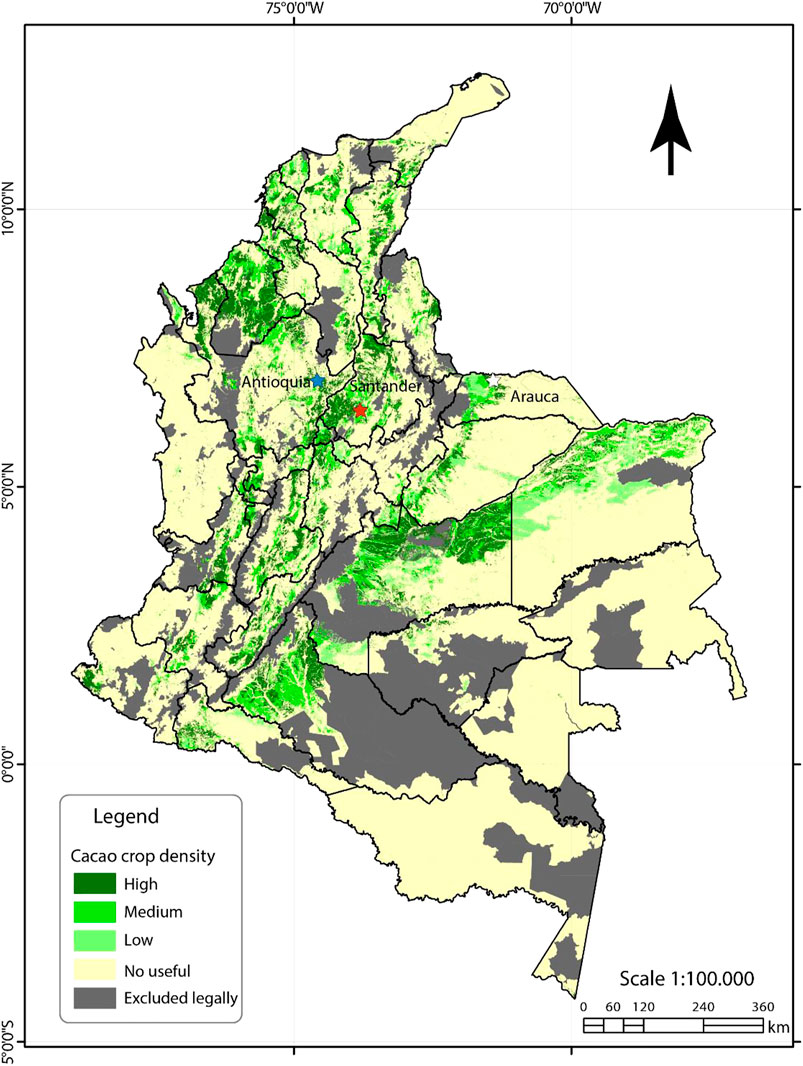
FIGURE 1. Locations of the assessed composite soil samples. Each composite soil sample was compiled based on the sampling of 20 cacao-growing farms at each location. Therefore, a representativeness was assured for each location, selected for its traditional land uses for cacao production and high yielding of fine flavour and aroma beans. In Antioquia district, the blue star included 20 samples from cacao-farms located in San Roque and Yolombó municipalities as part of the middle Magdalena mountain-system. The soil samples from the 20 assessed farms in Arauca are represented by a white star and are located in the municipality of Arauquita. In Santander, the 20 soil samples of farms assessed represented by a red star belongs to farms located in both San Vicente de Chucurí and El Carmen de Chucuri municipalities, as part of the Montaña Santandereana in the east cordilleras mountain-system. Adapted from UPRA’s map 2019.
2.2 IMC Measurement
For calorimetric assessment, the soil samples were sieved to avoid roots and particles bigger than 0.2 mm, therefore, a sieve with filter of 0.2 mm particle size was used in sterile conditions with a new sieve for each sample. Five grams of soil was added to glass ampoules of 20 mL. The samples were diluted in 10 mL of Mergeay medium (Bravo et al., 2018). The same amount of sample was amended with 10 mL of Mergeay medium containing 6 mg L−1 of CdCl2 (Sigma Aldrich, IL, United States). The amendment was included to excite both fast- and slow-growing CdtB populations. The samples were stirred with an electric stirring device adapted for mixing the soil samples with the media, which is constructed with a rotating stick and a stirring motor (TA Instruments, Delaware, United States; see http://www.tainstruments.com/wp-content/uploads/TAM-AIR-brochure.pdf, page 7). The stirring was at a constant of 80 rpm during all the thermal monitoring. This increases the heat-flow signal for target bacterial populations. The ampoules were inserted into the channels of a TAM Air 8 channels isothermal microcalorimeter (TA Instruments, Delaware, United States). The thermal monitoring was performed at 25°C for 12 days. This time is sufficient to perceive heat-flow from both, fast- and slow-growing CdtB populations.
2.3 Cadmium Immobilization
In parallel with the calorimetric experiment, a batch experiment was conducted to assess the Cd immobilization capacity of the CdtB populations from the soil samples. Five grams of the same soil samples were diluted in 500 mL of Mergeay medium amended with 6 mg L−1 of CdCl2. The samples were incubated at 25°C and shaken at 200 rpm for 12 days. Samples were taken at each 4 h. The strain Cupriavidus taiwanensis DSM17343 was used as positive control. The strain was grown in 500 mL of Mergeay liquid medium amended with the same concentration of Cd. Escherichia coli K12 DSM498 was used as a negative control which has been recognized as a non-tolerant Cd bacterium. To calculate the Cd immobilization ratios the pseudo-total Cd values were quantified in the subsamples and the controls. Cd was quantified by inductive coupled plasma with optical emission spectrometry (ICP-OES) in the supernatant. The digestion of samples and the conditions for ICP readings were carried out according to a previous methodology reported (Bravo et al., 2018). The residual Cd values were considered as the fraction of Cd that was not metabolized by CdtB in the soil sample or in the bacterial biomass, respectively.
2.4 CadA and smt Genes
To assess the presence of genes related to Cd immobilization, PCR amplifications of CadA and smt genes from soil samples were performed. The amplicons used were designed for CdtB populations. The primers used for CadA were Primer 1 forward: 5′AARACIGGIACIYTIACIAARGGIG3′, and Primer 2 reverse 5′GIGCRTCRTTIACICCRTCICCIA3’. The primers for smt were: primer 1, smt1, forward: 5′GAT CGA CGT TGC AGA GAC AG 3′ and primer 2, smt2, reverse: 5′GAT CGA GGG CGT TTT GAT AA 3'. These primers were designed from a consensus of available data (Naz et al., 2005; Aryal, 2020). The fragments obtained for CadA and smt genes were between 300 and 370 bp, respectively. The PCR was done using a master mix of, with a gold master mix solution (Qiagen, Germany), according to a previous study (Bravo et al., 2015). As a positive control the gene was amplified from DNA extracted from the strain C. taiwanensis reported previously as CdtB (Siripornadulsil and Siripornadulsil, 2013). Real-time quantitative PCR reactions were performed on an iCycler IQ5 real-time PCR System using the iQ SYBR Green supermix System (Bio-Rad, Hercules, CA, United States). The qPCR was performed in triplicate. The amplification of CadA gene in a final volume of work of 10 µL was carried out. Two µL of the diluted DNA from soil were mixed with 0.25 µL of the primers above-mentioned (final concentration of 1.25 µM). Five µL of SYBR green PCR kit (Qiagen, Germany) was included. The mix was diluted to 10 µL adding RNAase-free nanopure water. The qPCR program was a per previous work (Bravo et al., 2013). A standard curve and Ct values were obtained with dilutions of known copy numbers of the target gene from DNA from the positive control strain C. taiwanensis DSM17343.
2.5 SEM Measurement
To visualize the CdtB active in soil samples, a SEM imagery was performed. The samples were taken when maximal peaks of heat-flow were observed during IMC experiments. The SEM was carried out using a protocol previously reported for bacteria visualization (Páez-Vélez et al., 2019). An aluminium support was used for fixing the samples with an exposure time of 50s. A quantification of the metal and other related ions were done in spectra performed with energy dispersive X-ray spectroscopy (EDS) coupled to the SEM, using the equipment JEOL JSM-6490LV (JEOL, Tokyo, Japan). The scanning electron microscope was equipped with an Oxford INCA PentaFetX3 EDS detector. The range of visualization was screened through the slide of 2 cm2 and the images were taken in a range between 0.2–7 µm. Magnification scales were included on each micrography.
2.6 Soil Parameters and Cd Determinations
Soil pH was measured in each sample. The soil pH was assessed with a pH meter (Toledo, Spain) according to a previous method (Gil et al., 2022). The soil organic matter was quantified by atomic absortion spectrometry (Agilent Technologies, CA, United States). The method to estimate the percent of SOM was also previously reported (Bravo et al., 2021). Cd content in leaves and in cacao-beans (fermented and dried) was quantified with ICP-OES according to a methodology established previously (Rodríguez Giraldo et al., 2022), at the laboratory of chemistry of Agrosavia, C.I. Tibaitatá, in Mosquera, Colombia.
2.7 Data Analysis
The IMC data was used to integrate the heat-flow into heat in Joules. Once integrated, the data were fitted with the Gompertz equation of bacterial growth (Zwietering et al., 1991; Braissant et al., 2013). This equation was used to estimate the growth rate (µ), the maximal growth rate (µmax), the adaptation phase lambda (λ), the time to reach the maximum peak of heat-flow (TTP) and the maximum heat produced (Qmax). With the Cd immobilization test, it was possible to estimate the Cd immobilization ratio (Cdimm) (Bravo et al., 2018) in the soil samples assessed.
For all the experimental setups, three replicates were considered for each treatment and the data are shown as mean values. A Pearson correlation was performed between the kinetical growth parameters and the thermodynamic parameters. A PCA corelation analysis was performed to see if the gene amplification of CadA is related to soil pH and SOM, and to elucidate if the CdtB populations have an influence on Cd values found in soils and cacao beans.
3 Results and Discussion
3.1 How IMC Improve Our Knowledge on Functional Activity in Soils
Three composite soil samples were used to study the metabolic activity of Cd immobilization due to CdtB metabolism. The thermograms from Figure 2 show the differences between the metabolic fluxes of Cd, particularly when the CdtB populations are excited due to spiking with Cd in a soluble form, with CdCl2. In natural Cd conditions even if high Cd values have been found in some hotspots in Colombia (i.e., in some farms from Santander or Arauca), much more activity is found in soil samples spiked with exogenous Cd soluble solution than in natural Cd in soils. Interestingly, when Cd was amended in all samples a second peak of heat-flows appears after 96 h, showing the metabolic flux of slow-growing CdtB. In samples where no Cd was added, only the first peak occurring at 24–48 h is attributed to the metabolic rates related to Cd use by fast-growing CdtB.
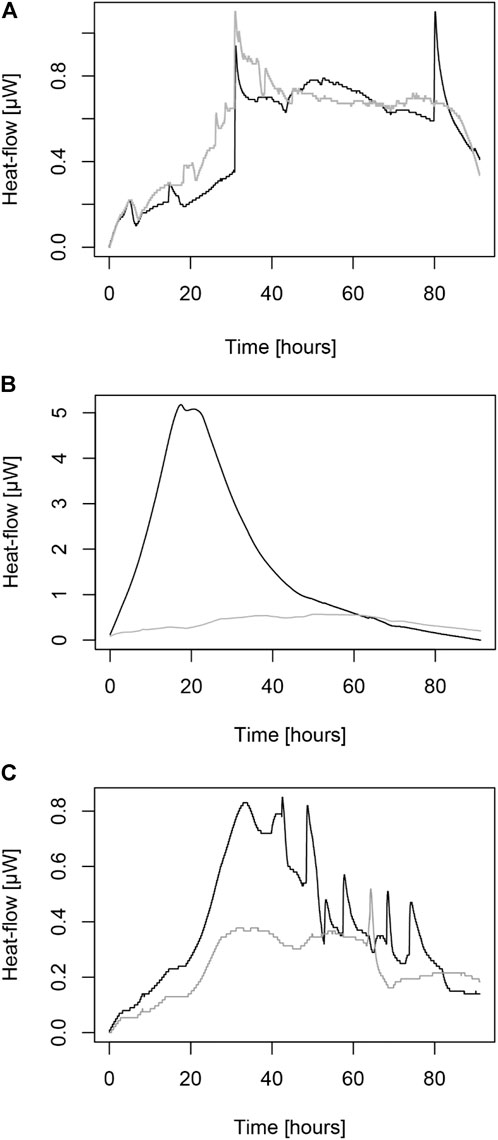
FIGURE 2. Thermograms of IMC showing the heat-flow of Cd metabolic activity in soils. (A) the heat-flow of soils from Antioquia. (B) the heat-flow of soils from Arauca. (C) the heat-flow of soils from Santander. Grey lines correspond to samples with natural Cd content. Black lines correspond to the metabolic activity of samples amended with 1 mg kg−1 of Cd.
Table 2 shows both the kinetical growth and the thermodynamic parameters obtained by IMC after fitting the Gompertz equation (Zwietering et al., 1991) into the edited data. Interestingly, both sets of parameters show the metabolic capacity of CdtB populations in situ, with a Cdimm from 0.16 to 0.30 mg kg−1h−1. Figure 3 shows a high correlation between the heat-flow and the maximum heat released by the soil samples both for natural Cd content and amended with 1 mg kg−1 Cd. The coefficient of determination is higher in natural conditions than those amended with Cd. However, it is highlighted that in both cases, the coefficient of determination is high enough for field samples measured by IMC. This has been also observed in previous metabolic measurements in a metabolic pathway related to C sequestration (Bravo et al., 2011; Bravo et al., 2015). Likewise, Figure 4 shows a correlation between the maximum growth rate (µmax) and the adaptation phase (λ) of CdtB exposed to natural Cd content or the amendment with Cd. This correlation shows two conditions. One condition, when CdtB are exposed to high adaptation phases and low growth. The other condition, when CdtB expresses a fast adaptation phase and a major maximum growth rate. The second condition might be of use for selecting viable cultures of CdtB to propose for bioremediation processes of Cd in cacao soils. The use of IMC in soil samples with natural Cd content, or in amendment with soluble source of Cd is an important tool to select specific CdtB populations, because fluctuations in energy availability can alter microbial activity related to Cd (Hart and Gorman-Lewis 2021), and thus, the capacity to adapt it to enriched soils where a bioremediation process needs to be settable.

TABLE 2. The kinetical growth and the thermodynamic parameters found in the assessed composite soil samples from Antioquia, Santander and Arauca. The parameters were calculated fitting the Gompertz equation with the edited data obtained from the heat-flow over time (thermograms), by IMC.
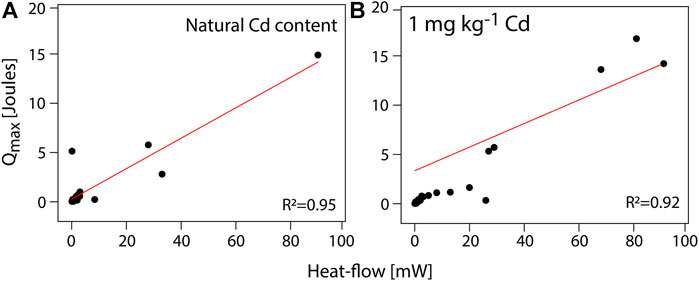
FIGURE 3. A Pearson correlation between heat-flow and the maximum heat obtained by IMC of composite soil samples exposed to (A) natural Cd content and (B) an amendment with 1 mg kg−1 CdCl2 (p ≤ 0.005).
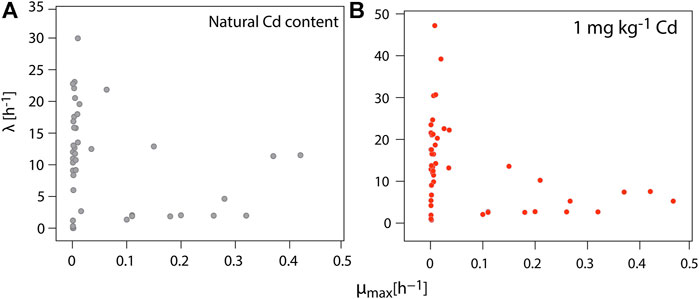
FIGURE 4. A Pearson correlation between the maximum growth rate and the adaptation phase of the CdtB populations into the composite soil samples, when exposed to (A) natural Cd content and (B) an amendment with 1 mg kg−1 of CdCl2. The kinetic growth parameters were calculated after fitting the edited data with the Gompertz equation (p ≤ 0.005).
This study highlights, the IMC method to characterize the metabolic activity of soil samples with natural content or spiked with Cd. With the heat-flow it is possible to fit a mathematical equation based on heat production. This feature is the main thermodynamic condition to access kinetical growth parameters of total microbial community, exhibiting the CdtB populations. It is worth mentioning, that spiked samples increase the growth rates, as well as the Qmax values. This is related to the increasing Cd immobilization capacity. It has been observed that CdtB have the property to increase in biomass (i.e., from 0.03 to 5.40 h−1 in Araucan sample) when increasing concentrations of Cd appears in the surroundings (i.e., from 0.11 to 0.21 mg kg−1 in Araucan sample). This has not been well documented before. Furthermore, it is pointed out that, the adaptation phase increase when soil samples were spiked with Cd. This could be related to the time the CdtB needs to make the metabolic switch from a minimum to a high toxic level of the metal in soil. This is also supported by changes observed with the TTP parameter where three major changes were observed when soil was spiked: 1) passing from fast to later peaks of metabolic activity; 2) increasing the signal of metabolic peaks and 3) yielding greater metabolic peaks that otherwise could not be possible to observe (see Figures 2A,C). Regarding the spiked soil samples, the first peak corresponds most likely to direct Cd consumption by faster growth rates of CdtB (i.e., Bacilli-like), due to availability of highly soluble forms of Cd, such as the most frequently bioavailable Cd2+ (Meter et al., 2019), which is the major Cd source added to the soil. The second peak could represent either the use of Cd by bacteria with slower growth rates (e.g., Streptomyces-like or actinomycetes-like) or the consumption of a less accessible pool of Cd, such as colloids of Fe/Mn oxides and phyllosilicates (Muehe et al., 2013; Bravo et al., 2018).
3.2 Cd Immobilization Capacity of CdtB in Soil
The parallel batch experiment to IMC shows that autochthonous populations of CdtB in composite soil samples from Antioquia exhibited major Cd immobilization capacities (0.30 mg kg−1) compared to those from Santander (0.25 mg kg−1) and Arauca (0.21 mg kg−1) after 12 days of incubation. It is important to mention that at this stage, the immobilization capacity was measured without any other mechanism to enhance the metabolic capacity. Therefore, a selection of microorganisms and the design of inoculums with high performance in formulated environments might increase the metabolic activity assessed in this study.
The soil samples were used to amplify two genes involved in Cd immobilization. Figure 5 shows the PCR amplification of genes CadA and smt. The bands correspond to the amplification of several populations with the gene present in soils. Even if the amplification does not mean that all the populations that have these genes are active, the brightness and width of the bands indicate a good concentration of the genes within the whole community of the composite soil samples. CadA gene is implied in cation pump exchange at periplasmic level, where internalization through the bioweathering pathway is the first step towards biological induction of CdCO3 or secondary otavite (Bravo and Braissant, 2022). Likewise, smt gene is involved in the regulation of ATPase dependant on reduction of Cd from soluble forms such as chlorates, or sulphates present in soil that CdtB transforms into non-soluble and geostable forms. The amplification of the smt and CadA genes is of note because they are related to metabolic pathways where the energy activation (ATPase systems) is needed, which could be expressed by the activation of the cad operon. The P-type ATPase efflux system causes transport of the metal by ATP hydrolysis, so the reaction is considered endothermic, due to the need for energy to hydrolyse ATP (Bravo and Braissant, 2022). This implies that the presence of these genes from CdtB populations in the assessed soil samples requires energy, which is confirmed by the endothermic reactions observed in Figure 2, and the last values of Figure 3, particularly when Cd is amended.
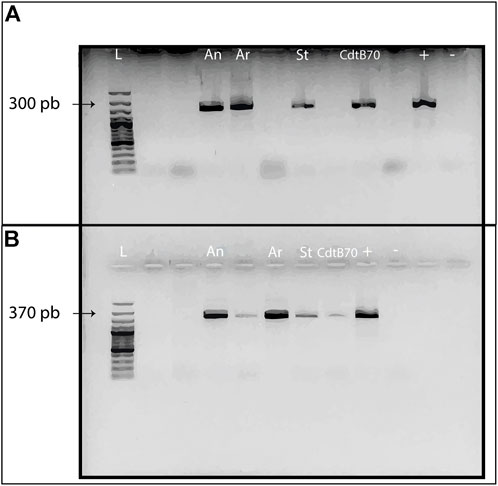
FIGURE 5. PCR amplifications of genes implied in Cd immobilization through the bioweathering pathway. (A). CadA amplicons from composite soil samples. (B). smt amplicons from the assessed soil samples. Conventions: L = Ladder; CdtB70 = CdtB strain from Santander, Colombia; + = Positive control strain Cupriavidus taiwanensis DSM17343; - = Negative control, ultra-nanopure sterile H2O with dimer-primers.
3.3 Bioweathering of Cd by CdtB in Soils: From Soluble to Sequestered Forms
Using scanning electron microscopy with energy dispersive spectroscopy (SEM/EDS) it was possible to visualize the presence of CdtB in samples where CdCl2 was amended to excite CdtB populations over the whole bacterial community. The presence of Cd+ and CO− as precursor of CdCO3 were detected in EDS spectra. These are known to be biologically induced. Figure 6 shows the presence of CdtB in samples from each spiked composite soil sample. The figure highlights the presence of several morphologies and shapes of CdtB populations, which are indicated with an asterisk in the microphotographs and CdCO3, indicated with white arrows. It is assumed that the observed morphotype corresponds to the target populations. It is worth mentioning that the Cd content at the concentration added, negatively affects the growth of any microorganism not-tolerant to the metal (Bravo et al., 2018), therefore, the observed morphologies corresponds exclusively to CdtB. Furthermore, with the SEM/EDS system it was possible to examine micro-scale features of the soil samples with Cd. The EDS system make enough magnification possible to detect Cd chemical elemental composition. As pointed out in Figure 6, on the surface of the tested soil samples Cd was detected with carbonate aggregations in each sampled assessed with higher presence of C and O atoms at the samples. Thus, the inclusion of this technology to the IMC technique allows an increase in our existing capacity to provide evidence of Cd bioweathering of total CdtB community, using a complete testing package, including metabolic capacities in surface soil micro-particle analysis, such as in this case, in field cacao evaluations.
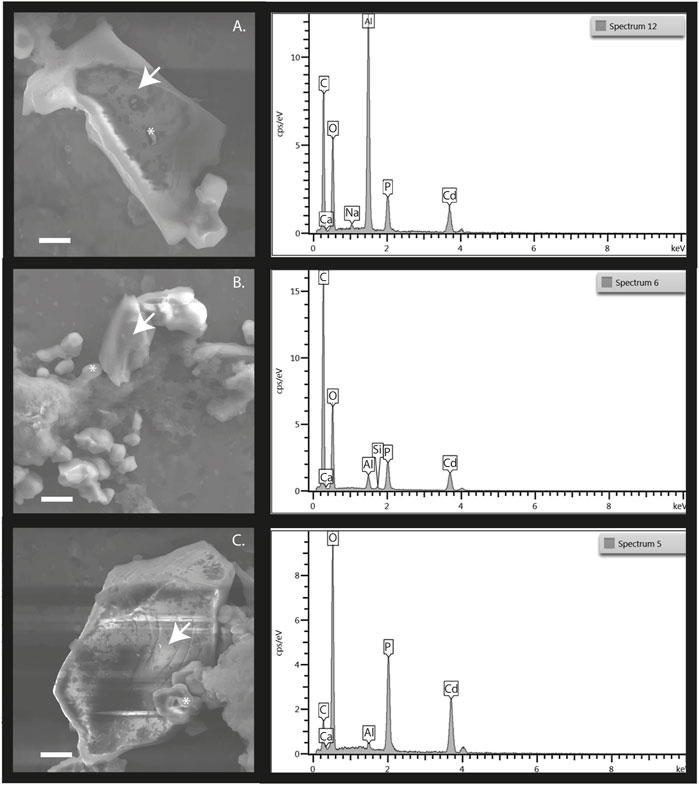
FIGURE 6. SEM-EDS of composite soil samples from (A). Antioquia, (B). Arauca and (C). Santander. The micrographs shown the presence of CdtB and the presence of cadmium carbonate or otavite (CdCO3). The white arrows point out the aggregates microbiologically induced. The presence of CdtB is highlighted by white asterisks. the EDS spectra show a greater presence of C, O ions, and the presence of Cd in all cases.
The PCA is shown in Figure 7. The PCA suggest that Cd in cacao beans is well explained by the factors assessed in this paper. The soil samples were clustered by location. The scale shows the Cd content in cacao beans as a reference variable. According to the scree plot, the percentage of explained variances was 61.1% on principal component 1 (see Supplementary Figure S1). Interestingly, Cd in soils is more related to soil CadA, soil pH, and SOM than Cd in cacao beans. This indicates that the presence of some genes could have more interaction in some soil Cd than others.
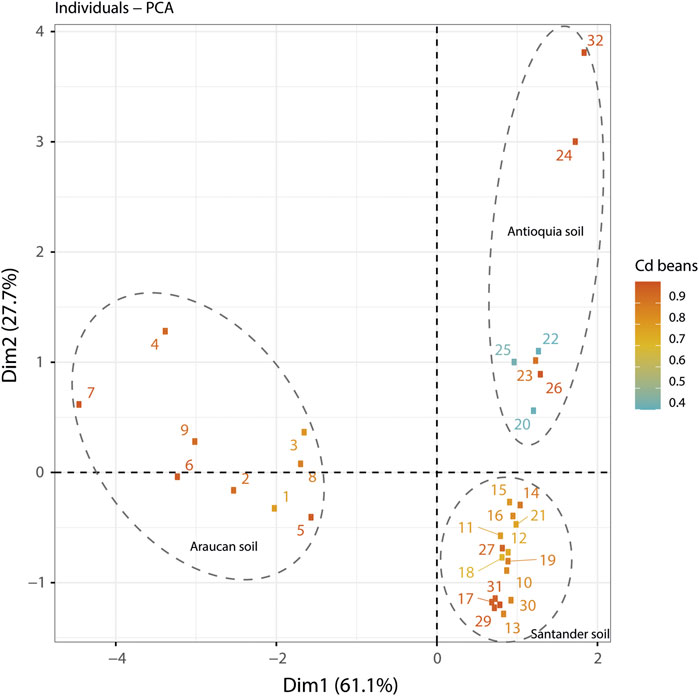
FIGURE 7. A PCA analysis of soil samples from Antioquia, Arauca and Santander. The scree plot suggested that 61.1% of the explained variances were observed in dimension 1. The origin of soil samples was clustered into each assessed location. The scale shown the Cd content in cacao beans as reference variable.
Another important variable to have into account is the type soil related with Cd immobilization capacity of CdtB. In this study three soil origin were confirmed. The soil type from Antioquia belong to the subgroup Oxic Dystrudepts of Inceptisols with some presence of Cd-like rocky types mainly in the forms of “Antioqueño Batholites” (Gil et al., 2022). The soil type of cacao farms from Arauca were Typic Endoaquepts of Inceptisols, with low levels of clay content (1.15%), SOM (less than 3%), and lower patchy parent material in alluvial sediments in farms nears to the rivers (Bravo and Benavides-Erazo, 2020). Moreover, the soil type observed in farms of Santander were Entisols made from the Umir formation, which is a lithostratigraphic unit, with high clay content around 85%, SOM of 5–20% and high heterogenous presence of Cd-rocky types mainly granitoids and some spots of shales, that have been detected in the central zone of Santander district (Bravo et al., 2018). Therefore, the relationship between the origin of soil with the chemical properties, may have an influence in the CdtB Cd immobilization capacity because the changes in soil type exert changes in potential capacity of phenotypic capacities of CdtB community due to the soil pH, clay content, the SOM percentage and the distribution of Cd, as mentioned previously. Nonetheless, Figure 7 shows that, even if pH is a well correlated variable to Cd in soil, there are other not directly related factors, such as the Cd content in cacao beans, which it has been also suggested by other authors (Argüello et al., 2019). This study supports the observation where more Cd in soils correlate with less in cacao beans, however, it also suggests that the presence of CdtB might have an influence on Cd geoaccumulation and Cd translocation factors, when the metabolic activity of microorganisms tolerant to the metal is included.
3.4 Cd: The Geoaccumulation Index (Igeo)
The geoaccumulation index (Igeo) was introduced by Müller in 1969 (Muller, 1969) and refers to the assessment of the degree of heavy metal (including Cd) enrichment due to 1) anthropogenic pollution, 2) the geochemical background values at the location and 3) the effect of natural diagenesis (Bravo and Braissant, 2022). Since the Igeo takes into account Cd measured in the rhizospheric zone, the sum of the three components explains the Cd content. The anthropogenic contribution can be calculated by the Cd input of fertilizers, manure or compost elements, mining, industry and petroleum extraction. In the soils collected from farms in Santander, the presence of coal mines might be an important form of Cd input. In soils collected from Arauca, petroleum refineries are nearby the cacao farms. The main anthropogenic factor influencing Cd input to the cacao system it seems to be due to P-like fertilizers with values greater than 60 mg kg−1 Cd (Bravo et al., 2022). However, it is likely that anthropogenic pollution is of minor importance.
Interestingly, there are strong differences between the geochemical background values from soil samples to each assessed district. Santander is known to have some hotspots of high Cd background in the soil (Bravo et al., 2021) with some spots of shales (Bravo et al., 2018), while soil samples from Antioquia (Gil et al., 2022) are rich in Batholite. This geological background is important due to the sedimentary process resulting in outcroppings that migrates from the subsoils (mainly by capillary fringe fractures) to the topsoil (mainly in the vadose zone).
On the contrary, soils from Arauca had lower values of Cd, supported by a lower geochemical background. However, the effect of natural diagenesis of Cd mobilized by the river and found in sediments, combined with phosphorites (Bravo et al., 2018), seems to be the key for faster release of available Cd at that location.
To this, it is important to add the translocation factor or TF of plants (Wiesler, 2012), especially in the case of the hyperaccumulator plant Theobroma cacao L. (Kirkham, 2006). The TF is the ratio of the Cd found in plant tissue and total Cd value found in soil (Roslan et al., 2016). The TF factor is particularly useful when it is compared to the pseudo-total Cd from soils with available Cd from plant tissue. Combining this with the Igeo, could improve the understanding on the origins of pseudototal Cd in soils, where the available fraction is recovered. In this, the role of CdtB is intriguing, because the bacterial metabolic pathways might induce a trade-off between solubilizing or weathering Cd in the cacao-soil system. Therefore, both the Igeo and the TF should take into account the role of CdtB in rhizosphere of cacao plants.
3.5 CdtB as Critical Factor in Cd Accumulation
This study suggests that the combination of Cdimm ratios obtained using isothermal microcalorimetry, the molecular basis of metabolic immobilization, and SEM imagery could be seen as a useful toolkit to select CdtB populations for bioremediation in contaminated cacao farms. The accumulation of Cd in cacao is cyclic, and therefore, depends on translocation fluxes from the underground to the topsoil, thus, the rhizosphere of cacao is an area of high Cd movement. It is worth mentioning, that not all the Cd found in subsoil, is retrieved in the cacao beans via root system. It has been estimated that approximately 60% of Cd in the cacao-growing soils is related to the acid-soluble fraction and bound to organic matter (Chavez et al., 2016), that might enter in some soil types, the roots as part of the so-called ‘available Cd’ and finally into the food chain. But how much of Cd could be regulated in the rhizosphere modifying the percent available into non-soluble forms of the heavy metal? The answer is in the metabolic activity of CdtB and its regulatory capacity of edaphic conditions in the surrounding soil.
In a recent review, it has been demonstrated that CdtB participate in at least seven known metabolic pathways that affect the Cd content in soil (Bravo and Braissant, 2022). The metabolic condition regardless of the availability of Cd, is excited by CdtB present in soil. How can we increase this metabolic capacity to kick-off bioremediation strategies in order to tackle Cd entry into the food chain? One key point in the bioremediation is the bioaugmentation of CdtB so that the biomass concentration is great enough to deal with high values of Cd, including the phytoavailable and the aqueous-phase fractions. But this is just the first step. It is necessary to go in deep into the metabolic mechanisms that rule CdtB via bioweathering, biochelation, or even by biosorption, or the metabolic pathway they are capable to exhibit. A compromise between the design of new culture media adapted to the CdtB metabolic capacities and increasing biomass production, could be a second stage in the strategy. However, at that point, there remain a set of questions that need to be addressed. For instance, what is the effect of the bioaugmentation of CdtB populations on the ecology of the cacao-soil system? or what is the effect of the bioaugmented microbial biomass applied in cacao-growing soils on the nutrition cycling of cacao plantation? Moreover, does the applicability of CdtB for bioremediation in other cacao growing areas around the world remain effective?
These questions require more researchers in bioremediation, to make the necessary probes that cover enough criteria to obtain a bioformulation that meets with the standards required to generate successful remediation. This focus has started in Colombia, however a joint-effort research project in cooperation between Colombia, Ecuador and Costa Rica allows inclusion of the bioremediation strategy as one of the proposed applications in field semi-controlled experiments. This approach could be also applied to other heavy metal pollution issues in cacao. For instance, the main issue in terms of heavy metals in Brazilian cacao plantations is not cadmium but lead. The presence of Pb-tolerant bacteria could be useful to explore using the chemometric approach here proposed. Interestingly, in all the above-mentioned stages, the IMC in combination with molecular tools and visualization of the probes, might yield high quality information to allow more detailed research in the bioremediation of complexes systems such as that found in a cacao plantation.
4 Conclusion
This study demonstrates the use of data from a combination of technical tools including isothermal microcalorimetry, to assess metabolic Cd immobilization capacity from total CdtB community in cacao-growing soils. This study highlighted the IMC method to characterize the metabolic activity of soil samples with natural Cd or spiked with it. This allows observation of differences between the metabolic fluxes of Cd, particularly when slow-growing CdtB populations (more likely of actinomycetes-like class) were excited with CdCl2 after 48 h of thermal monitoring, where a less accessible pool of Cd is found. The kinetic growth and thermodynamic parameters derived from IMC data are important criteria to recognize and select potential bioremediation populations with highly correlated factors such as heat-flow with maximum heat (Qmax) as well as the maximum growth rate and lambda.
The IMC combined with Cd immobilization test confirmed that the CdtB community in Antioquian soil samples have major Cd immobilization rates (0.30 mg kg−1) compared to the other sites sampled. The thermograms of IMC confirmed a major metabolic flux of Cd with the presence of major peaks of activity in both natural- and spiked-Cd soil samples. The presence of both, CadA and smt genes supported the metabolic mechanisms that CdtB uses to be a bioweathering pathway. IMC confirmed this pathway since both are endothermic reactions due to the regulation of ATPase dependant mechanisms that bind Cd into geostable forms. Likewise, the SEM/EDS imagery show the presence of both autochthonous CdtB populations and CdCO3 confirmed by the EDS spectroscopy. These results support the evidence of a bioweathering process occurring due to the metabolic activity observed by IMC.
The PCA performed suggested correlations between Cd in cacao beans with some key parameters in cacao-growing soils, such as CadA, pH and SOM. The soil type and origin influences the variation of these soil parameters into each set of soil samples from the three cacao-growing districts assessed in this study. The correlation between Cd in soils with Cd in beans was discussed in two main processes by which CdtB might have an influence: the Cd geoaccumulation index (Igeo) and the translocation factor TF in cacao plants.
In conclusion, the geomicrobiological aspects of the presence of total community of CdtB was possible to described using a chemometric approach. This study supports the idea that the role of CdtB is underestimated and is a key critical factor in Cd accumulation in cacao-growing farms. The combination of IMC with other molecular, statistical and theoretical tools could be seen as a useful toolkit for the chemometric approach for bioremediation of contaminated cacao farms. To expand the applicability of this approach, broad field-based experiments should be included in cacao plantations in other countries with the same issue.
Data Availability Statement
The datasets presented in this article are not readily available because the raw data supporting the conclusions of this article will be made available by the authors, once requested officially to Agrosavia intellectual property department. Requests to access the datasets should be directed to ZGJyYXZvQGFncm9zYXZpYS5jbw==.
Author Contributions
DB contributed to collecting the data, performing the experiments, editing and analysing the data, writing the manuscript and editing the final version.
Funding
This research was supported by the project ‘SGR Cacao Arauca - Contribuir mitigación niveles cadmio cacao’, through the Grant 1001450. The long name of the project is ‘Implementación de estrategias agroforestales y vinculación de avances en el manejo agronómico y poscosecha de nuevos clones, para mejorar la productividad y calidad del cacao en el departamento de Arauca’ with the SGR Grant BPIN 2018000100148. This project was also in part supported by the project: Multi-agency cocoa platform for Latin America and the Caribbean ‘Cocoa 2030-2050,’ with the grant ATN/RF-17235-RG. The opinions expressed in this publication are exclusively those of the authors and do not necessarily reflect the point of view of FONTAGRO, its Board of Directors, or the countries it represents.
Conflict of Interest
The author declares that the research was conducted in the absence of any commercial or financial relationships that could be construed as a potential conflict of interest.
Publisher’s Note
All claims expressed in this article are solely those of the authors and do not necessarily represent those of their affiliated organizations, or those of the publisher, the editors and the reviewers. Any product that may be evaluated in this article, or claim that may be made by its manufacturer, is not guaranteed or endorsed by the publisher.
Acknowledgments
I gratefully acknowledge the “Ministerio de Agricultura y Desarrollo Rural (MADR)” for their generous financial support of this study. I wish to thank to the Corporación Colombiana de Investigación Agropecuaria (AGROSAVIA) for technical support. Soil samples were collected according to the Colombian Resolution No. 1466 of 3rd December 2014, by which Agrosavia has a collecting permit of biological diversity sampling for non-commercial and scientific research purposes. I would also like to thank to Dr. Rachel Atkinson from Alliance Bioversity-CIAT, in Lima, Perú, for scientific discussions and proofreading this manuscript. To the reviewers of this manuscript for their generous comments and contributions for improvement.
Supplementary Material
The Supplementary Material for this article can be found online at: https://www.frontiersin.org/articles/10.3389/fenvs.2022.910234/full#supplementary-material
References
Argüello, D., Chavez, E., Lauryssen, F., Vanderschueren, R., Smolders, E., and Montalvo, D. (2019). Soil Properties and Agronomic Factors Affecting Cadmium Concentrations in Cacao Beans: A Nationwide Survey in Ecuador. Sci. Total Environ. 649, 120–127. doi:10.1016/j.scitotenv.2018.08.292
Aryal, M. (2020). A Comprehensive Study on the Bacterial Biosorption of Heavy Metals: Materials, Performances, Mechanisms, and Mathematical Modellings. Rev. Chem. Eng. 36, 1–40. doi:10.1515/revce-2019-0016
Braissant, O., Wirz, D., Göpfert, B., and Daniels, A. U. (2010). Use of Isothermal Microcalorimetry to Monitor Microbial Activities. FEMS Microbiol. Lett. 303, 1–8. doi:10.1111/j.1574-6968.2009.01819.x
Braissant, O., Bonkat, G., Wirz, D., and Bachmann, A. (2013). Microbial Growth and Isothermal Microcalorimetry: Growth Models and Their Application to Microcalorimetric Data. Thermochim. Acta 555, 64–71. doi:10.1016/j.tca.2012.12.005
Braissant, O., Astasov-Frauenhoffer, M., Waltimo, T., and Bonkat, G. (2020). A Review of Methods to Determine Viability, Vitality, and Metabolic Rates in Microbiology. Front. Microbiol. 11, 547458–547525. doi:10.3389/fmicb.2020.547458
Bravo, D., and Benavides-Erazo, J. (2020). The Use of a Two-Dimensional Electrical Resistivity Tomography (2D-ERT) as a Technique for Cadmium Determination in Cacao Crop Soils. Appl. Sci. 10, 1–16. doi:10.3390/app10124149
Bravo, D., and Braissant, O. (2022). Cadmium-tolerant Bacteria: Current Trends and Applications in Agriculture. Lett. Appl. Microbiol. 74, 311–333. doi:10.1111/lam.13594
Bravo, D., Braissant, O., Solokhina, A., Clerc, M., Daniels, A. U., Verrecchia, E., et al. (2011). Use of an Isothermal Microcalorimetry Assay to Characterize Microbial Oxalotrophic Activity. FEMS Microbiol. Ecol. 78, 266–274. doi:10.1111/j.1574-6941.2011.01158.x
Bravo, D., Martin, G., David, M. M., Cailleau, G., Verrecchia, E., and Junier, P. (2013). Identification of Active Oxalotrophic Bacteria by Bromodeoxyuridine DNA Labeling in a Microcosm Soil Experiments. FEMS Microbiol. Lett. 348 (2), 103–111.
Bravo, D., Braissant, O., Cailleau, G., Verrecchia, E., and Junier, P. (2015). Isolation and Characterization of Oxalotrophic Bacteria from Tropical Soils. Arch. Microbiol. 197, 65–77. doi:10.1007/s00203-014-1055-2
Bravo, D., Pardo-Díaz, S., Benavides-Erazo, J., Rengifo-Estrada, G., Braissant, O., and Leon-Moreno, C. (2018). Cadmium and Cadmium-tolerant Soil Bacteria in Cacao Crops from Northeastern Colombia. J. Appl. Microbiol. 124, 1175–1194. doi:10.1111/jam.13698
Bravo, D., Leon-Moreno, C., Martínez, C. A., Varón-Ramírez, V. M., Araujo-Carrillo, G. A., Vargas, R., et al. (2021). The First National Survey of Cadmium in Cacao Farm Soil in Colombia. Agronomy 11, 1–18. doi:10.3390/agronomy11040761
Bravo, D., Santander, M., Rodriguez, J., Escobar, S., Gideon, R., and Atkinson, R. (2022). ‘From Soil to Chocolate Bar’: Identifying Critical Steps in the Journey of Cadmium in a Colombian Cacao Plantation. Food Addit. Contam. Part A 39, 949–963. Accepted. doi:10.1080/19440049.2022.2040747
Chavez, E., He, Z. L., Stoffella, P. J., Mylavarapu, R. S., Li, Y. C., Moyano, B., et al. (2015). Concentration of Cadmium in Cacao Beans and its Relationship with Soil Cadmium in Southern Ecuador. Sci. Total Environ. 533, 205–214. doi:10.1016/j.scitotenv.2015.06.106
Chavez, E., He, Z. L., Stoffella, P. J., Mylavarapu, R. S., Li, Y. C., and Baligar, V. C. (2016). Chemical Speciation of Cadmium: An Approach to Evaluate Plant-Available Cadmium in Ecuadorian Soils under Cacao Production. Chemosphere 150, 57–62. doi:10.1016/j.chemosphere.2016.02.013
Diels, L., Dong, Q., Lelie, D., Baeyens, W., and Mergeay, M. (1995). The Czc Operon of Alcaligenes Eutrophus CH34: from Resistance Mechanism to the Removal of Heavy Metals. J. Indust. Microbiol. 14, 142–153. doi:10.1007/bf01569896
Engbersen, N., Gramlich, A., Lopez, M., Schwarz, G., Hattendorf, B., Gutierrez, O., et al. (2019). Cadmium Accumulation and Allocation in Different Cacao Cultivars. Sci. Total Environ. 678, 660–670. doi:10.1016/j.scitotenv.2019.05.001
Gil, J. P., López-Zuleta, S., Quiroga-Mateus, R. Y., Benavides-Erazo, J., Chaali, N., and Bravo, D. (2022). Cadmium Distribution in Soils, Soil Litter and Cacao Beans: a Case Study from Colombia. Int. J. Environ. Sci. Technol. 19, 2455–2476. doi:10.1007/s13762-021-03299-x
Gramlich, A., Tandy, S., Gauggel, C., López, M., Perla, D., Gonzalez, V., et al. (2018). Soil Cadmium Uptake by Cocoa in Honduras. Sci. Total Environ. 612, 370–378. doi:10.1016/j.scitotenv.2017.08.145
Groenenberg, J. E., and Lofts, S. (2014). The Use of Assemblage Models to Describe Trace Element Partitioning, Speciation, and Fate: A Review. Environ. Toxicol. Chem. 33, 2181–2196. doi:10.1002/etc.2642
Hart, C., and Gorman‐Lewis, D. (2021). Energetics of Acidianus Ambivalens Growth in Response to Oxygen Availability. Geobiology 19 (1), 48–62.
Himeno, S., and Aoshima, K. (2019). Cadmium Toxicity: New Aspects in Human Disease, Rice Contamination, and Cytotoxicity. Okayama, Japan: Springer.
Kirkham, M. B. (2006). Cadmium in Plants on Polluted Soils: Effects of Soil Factors, Hyperaccumulation, and Amendments. Geoderma 137, 19–32. doi:10.1016/j.geoderma.2006.08.024
Liu, Y., Wang, J., Li, P., Xie, Y., Xie, H., Xie, T., et al. (2020). Bioconversion of High-concentration Chelated Cd to nano-CdS Photocatalyst by Sulfate-reducing Bacteria. J. Chem. Technol. Biotechnol. 95, 3003–3011. doi:10.1002/jctb.6461
Long, J., Yu, M., Xu, H., Huang, S., Wang, Z., and Zhang, X.-X. (2021). Characterization of Cadmium Biosorption by Inactive Biomass of Two Cadmium-Tolerant Endophytic Bacteria Microbacterium Sp. D2-2 and Bacillus Sp. C9-3. Ecotoxicology 30, 1419–1428. doi:10.1007/s10646-021-02363-z
Meter, A., Atkinson, R., and Laliberte, B. (2019). Cadmium in Cacao from Latin America and the Caribbean. A Review of Research and Potential Mitigation Solutions. Lima, Peru: CIAT-Bioversity International.
Muehe, E. M., Obst, M., Hitchcock, A., Tyliszczak, T., Behrens, S., Schröder, C., et al. (2013). Fate of Cd during Microbial Fe(III) Mineral Reduction by a Novel and Cd-Tolerant Geobacter Species. Environ. Sci. Technol. 47, 14099–14109. doi:10.1021/es403365w
Naz, N., Young, H. K., Ahmed, N., and Gadd, G. M. (2005). Cadmium Accumulation and DNA Homology with Metal Resistance Genes in Sulfate-Reducing Bacteria. Appl. Environ. Microbiol. 71, 4610–4618. doi:10.1128/aem.71.8.4610-4618.2005
Páez-Vélez, C., Rivas, R. E., and Dussán, J. (2019). Enhanced Gold Biosorption of Lysinibacillus Sphaericus CBAM5 by Encapsulation of Bacteria in an Alginate Matrix. Metals 9, 1–10. doi:10.3390/met9080818
Qu, C., Chen, W., Fein, J. B., Cai, P., and Huang, Q. (2021). The Role of Interfacial Reactions in Controlling the Distribution of Cd within Goethite−humic Acid−bacteria Composites. J. Hazard Mater. 405, 1–9. doi:10.1016/j.jhazmat.2020.124081
Ramtahal, G., Umaharan, P., Hanuman, A., Davis, C., and Ali, L. (2019). The Effectiveness of Soil Amendments, Biochar and Lime, in Mitigating Cadmium Bioaccumulation in Theobroma Cacao L. Sci. Total Environ. 693, 1–11. doi:10.1016/j.scitotenv.2019.07.369
Rodríguez Giraldo, Y., Rodriguez Sánchez, E., Torres, L. G., Montenegro, A. C., and Pichimata, M. A. (2022). Development of Validation Methods to Determine Cadmium in Cocoa Almond from the Beans by ICP-MS and ICP-OES. Talanta Open 5, 1–6. doi:10.1016/j.talo.2021.100078
Roslan, N. A., Syed Ismail, S., and Praveena, S. M. (2016). The Transfer Factor of Cadmium in Fern Leaves and its Potential Health Risk. Asia Pac Environ. Occup. Health J. 2, 48–57.
Siripornadulsil, S., and Siripornadulsil, W. (2013). Cadmium-tolerant Bacteria Reduce the Uptake of Cadmium in Rice: Potential for Microbial Bioremediation. Ecotoxicol. Environ. Saf. 94, 94–103. doi:10.1016/j.ecoenv.2013.05.002
Siripornadulsil, S., Thanwisai, L., and Siripornadulsil, W. (2014). Changes in the Proteome of the Cadmium-Tolerant Bacteria Cupriavidus Taiwanensis KKU2500-3 in Response to Cadmium Toxicity. Can. J. Microbiol. 60, 121–131. doi:10.1139/cjm-2013-0713
Tchounwou, P. B., Yedjou, C. G., Patlolla, A. K., and Sutton, D. J. (2012). Heavy Metal Toxicity and the Environment. Exp. Suppl. 101, 133–164. doi:10.1007/978-3-7643-8340-4_6
Wiesler, F. (2012). “Chapter 9 - Nutrition and Quality,” in Marschner's Mineral Nutrition of Higher Plants. Editor P. Marschner. Third Edition (San Diego: Academic Press), 271–282. doi:10.1016/b978-0-12-384905-2.00009-1
Keywords: isothermal microcalorimetry (IMC), cadmium-tolerant bacteria (CdtB), cadmium, cacao (Theobroma cacao L.), metabolic activity, cocoa soils
Citation: Bravo D (2022) Bacterial Cadmium-Immobilization Activity Measured by Isothermal Microcalorimetry in Cacao-Growing Soils From Colombia. Front. Environ. Sci. 10:910234. doi: 10.3389/fenvs.2022.910234
Received: 01 April 2022; Accepted: 24 June 2022;
Published: 13 July 2022.
Edited by:
Juan Manuel Trujillo-González, University of the Llanos, ColombiaReviewed by:
Suzana Dimitrijevic-Brankovic, University of Belgrade, SerbiaMilan Momčilović, University of Belgrade, Serbia
Copyright © 2022 Bravo. This is an open-access article distributed under the terms of the Creative Commons Attribution License (CC BY). The use, distribution or reproduction in other forums is permitted, provided the original author(s) and the copyright owner(s) are credited and that the original publication in this journal is cited, in accordance with accepted academic practice. No use, distribution or reproduction is permitted which does not comply with these terms.
*Correspondence: Daniel Bravo, ZGJyYXZvQGFncm9zYXZpYS5jbw==