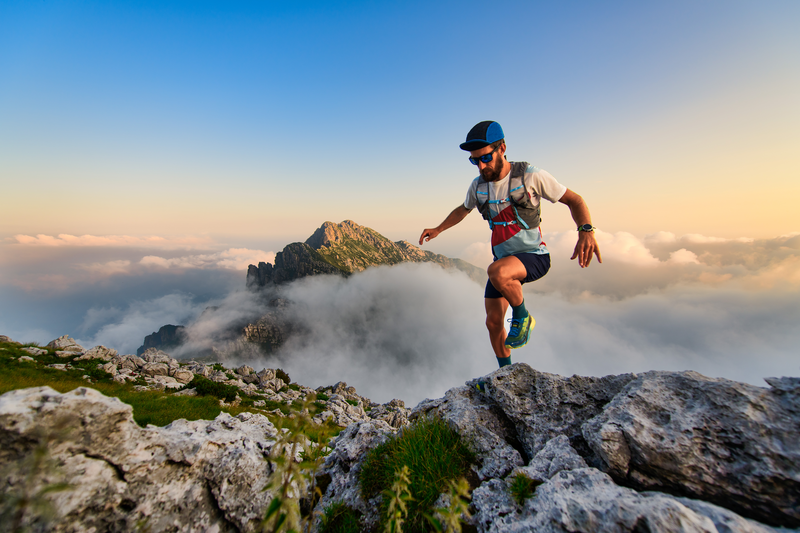
95% of researchers rate our articles as excellent or good
Learn more about the work of our research integrity team to safeguard the quality of each article we publish.
Find out more
ORIGINAL RESEARCH article
Front. Environ. Sci. , 08 June 2022
Sec. Toxicology, Pollution and the Environment
Volume 10 - 2022 | https://doi.org/10.3389/fenvs.2022.908819
This article is part of the Research Topic Biogeochemistry of Metals in Contaminated Environments View all 9 articles
Montmorillonite has been widely used in remediation of toxic metals. However, for the safety of the soil ecosystem, the impact of this technology on microorganisms is still unclear. Here, the influence of nano-montmorillonite on immobilization, accumulation of cadmium, and microbial activity in a soil–plant system was investigated. The results revealed that the nano-montmorillonite treatments reduced cadmium bioavailability and the uptake by oilseed rape. It was found that the addition of NMMT reduced the toxicity of Cd on soil microorganisms and improved enzyme activity. The nano-montmorillonite stimulated the relative abundance of Proteobacteria that was closely related to nutrient turnover in soil. In addition, the treatment with 0.5% addition of nano-montmorillonite significantly improved the microbial alpha diversity index and enhanced the relative abundance of Bacteroidetes and Planctomycetes. Thus, certain soil quality recovery occurred after the application of nano-montmorillonite. To successfully combine remediation and microbial effects, 0.5% nano-montmorillonite was recommended to be added to cadmium-contaminated soil to minimize the additional impact on the soil ecosystem.
With the rapid development of industry and agriculture, the prevalence of mining, smelting, wastewater irrigation, and chemical fertilizer abuse leads to cadmium (Cd) contamination in soil, which has become an increasingly serious environmental concern (Sharma and Archana, 2016; Sun Y. et al., 2016; Xu et al., 2017). In 2011, 46.7% of irrigated areas were heavily polluted by heavy metals, and the area of Cd-contaminated farmlands reached up to 1.3 × 103 hm2 in 25 regions of 11 provinces in China (Guo et al., 2006; Zhang and Pu, 2011). Moreover, Sharma and Archana (2016) and González-Alcaraz et al. (2018) reported that about 10.0% of all rice grown and sold in China failed to meet the government standards for Cd. Cadmium can damage plant growth, reduce crop yields, and potentially endanger human health throughout the food chain. Ingested Cd is linked to lung, endometrial, bladder, and breast cancer (Guo et al., 2006; Sun et al., 2013; Song et al., 2017a). Thus, the remediation of Cd-contaminated soils in China is crucial to ensure future food security and human health.
In situ immobilization is a cost-effective and potentially reliable method to stabilize heavy metals through physical packaging and chemical reaction (Zhang and Pu 2011; Bolan et al., 2014). Numerous amendments have been investigated to effectively immobilize Cd in soils, such as biochar, zeolite, and clay minerals (Usman et al., 2006; Khan et al., 2017; Zhu et al., 2017). Clay minerals are one of the most abundant substances on the earth, and the feasibility of clay minerals as an amendment to immobilize heavy metal-contaminated soil has been intensively studied (Qin et al., 2020). For example, Sun et al. (2016) showed that sepiolite was effective in immobilizing Cd and reducing Cd toxicity, thus improving the environmental quality of soils.
Montmorillonite (MMT), a typical 2:1 mineral material, has been explored as a promising remediation material (Lothenbach et al., 1999; Chen et al., 2015). With high cation exchange capacity (CEC) and specific surface area, it showed a promoting effect on bacterial growth by altering soil pH (Xing et al., 2020). Various modification methods have been carried out to enhance the adsorption ability of montmorillonite, due to the poor stability of the original montmorillonite (Yang et al., 2021). Montmorillonite-modified biochar composites were successfully studied for the addition of Zn (II) removal from an aqueous solution (Song et al., 2020). Also, nanoscale zero-valent iron supported onto montmorillonite was synthesized and efficiently removed SeVI and AsV from water systems (Suazo-Hernández et al., 2021). But most of these studies only explored the adsorption characteristics in the water phase.
Recently, researchers have shown great interest in nanomaterials for the remediation of various pollutants in soil, due to their huge specific surface area (SSA), excellent chemical reactivity, and particular physical and chemical properties (Seifpanahi Shabani et al., 2017). Over the last decade, a wide range of natural or synthetic nanomaterials, such as carbon nanotubes, nanohydroxyapatite, and nanoscale zero-valent iron, have been investigated in soils contaminated by potentially toxic elements (Zhao et al., 2015; Sun R.-J. et al., 2016; Song et al., 2017b; Gil-Díaz et al., 2017). Applying nanomaterials to polluted soil can cause a significant change in the mobility and bioavailability of the heavy metal with the potential to improve ecosystem health (Huang et al., 2016). NMMT (nano-montmorillonite) also can cause metabolic recovery and enhancement of soil quality in paddy soils co-contaminated by cadmium and lead (Sun Y. et al., 2016). Nano-montmorillonite is a green natural material and is sometimes considered a “nanoclay” with particle shapes that include a sheet-like structure in which the two-dimensional length far exceeds its thickness (Chang et al., 2007). In our previous study, NMMT demonstrated highly efficient absorbance for the removal of cadmium ions from water solutions (Liu et al., 2018). Nevertheless, only the immobilization effect and mechanism of modified montmorillonite in heavy metal-contaminated soil were studied. The effect of applying nano-montmorillonite on microbial diversity remains elusive.
In this study, we hypothesized that NMMT could change soil property and bacterial communities and further facilitate stabilization of Cd in NMMT-applied soils. Consequently, the main objectives of the current research were: 1) to assess the immobilization ability of nano-montmorillonite for cadmium in the soil–plant system; 2) to reveal how nano-montmorillonite impacts soil microbial activity and community diversity; and 3) to recommend the optimal dosage of nano-montmorillonite for cadmium-contaminated soils.
Soil samples (0–20 cm) were collected from agriculturally cultivated fields in the suburbs of Baoding City, Hebei Province, China. The basic soil properties are shown in Supplementary Table S1. The natural nano-montmorillonite was purchased from Sanding Technology Co., Ltd. (Zhejiang Province, China). The NMMT had a surface area of 19.86 m2/g, as determined using the Brunauer–Emmett–Teller (BET; Autosorb-iQ-MP; Quantachrome Instruments Company) method. The material was characterized using X-ray powder diffraction (XRD; D8 ADVANCE; Bruker/Switzerland). The XRD diagram is described in Supplementary Figure S1. The results showed that the chemical formula is Na0.3(Al, Mg)2Si4O10(OH)2·6H2O, and the content of montmorillonite was more than 90%. The average particle size of NMMT is 76 nm. The Cd content of NMMT itself was determined to be 0.064 mg/kg.
The soil was ground to pass through a 20-mesh sieve and thoroughly mixed with the level of 5 mg/kg Cd (CdCl2·2.5H2O), and the soil was cycled between wet and dry for 6 weeks. Then, NMMT was blended into the artificially contaminated soils at a rate of 0% (control), 0.2%, 0.5%, and 1% (w/w, on an air-dry weight basis) and incubated for 8 weeks. The same base fertilizer rates (N/P/K = 1.5:1:1.5) in the form of CO(NH2)2, KCl, and KH2PO4 solution were appended to previously treated soils before seeding with Brassica campestris L. Also, 2 kg of prepared soil was loaded into each plant box (length × width × height = 200 × 170 × 110 cm). Also, 10 seeds of oilseed rape were sown into each pot. Soil moisture content was adjusted to 60% by adding distilled water daily. The pot experiment was conducted under greenhouse conditions with a temperature of 20–25°C during the day and 8–15°C at night. The pot was arranged in a randomized design within the greenhouse, and it was intermittently rotated to ensure consistent growth conditions. Each treatment was conducted in triplicate.
Plants were harvested after a 10-, 20-, 40- and 60-day growth. The plants were cleaned using deionized water, put in a drying oven at 105°C for 30 min, and then adjusted to 65°C to a constant weight. The dry weight of each plant was recorded. The plants were ground using an agate mortar and passed through a 100-mesh sieve for Cd analysis. Plant samples were digested with a solution of HNO3–HClO4 according to Yang et al. (2013). The total Cd content in the digests was determined using inductively coupled plasma mass spectrometry (ICP-MS; Thermo Fisher Scientific Co., Ltd; Shanghai, China).
The soil samples were collected from each pot at the time of plant harvest. Each sample was divided into two subsamples. One portion of fresh soil samples was immediately stored at −80°C for microbial measurements. Another 100-g soil sample was air-dried, ground, and passed through a sieve to determine the soil's physical–chemical properties and enzyme activities. The soil pH was measured at a soil: water ratio of 1:2.5 (m/v) by using a pH meter. The electrical conductivity (EC) was analyzed in an aqueous extract (soil: water ratio = 1:5 m/v) using a conductivity meter. The soil-available Cd was evaluated for diethylenetriaminepentaacetic acid (DTPA)-extractable heavy metals according to GB/T 23739-2009. Briefly, 5 g dried samples were extracted with 25 ml DTPA aqueous for 120 min at 180 rpm and then centrifuged at 4,000 r/min for 10 min. The concentration of available Cd was determined by ICP-MS.
Soil dehydrogenase, urease, and catalase were assayed according to the instructions in the kits (Comin Biotechnology Co., Ltd.; Suzhou, China) (Gnana et al., 2021; Gnana et al., 2021). Enzyme activities were expressed as 1 μg TF (triphenyl formazone) catalyzed g−1 dry soil at 37°C daily, 1 μg NH3–N released g−1 dry soil at 37°C daily, and 1 μmol H2O2-catalyzed g−1 dry soil daily for dehydrogenase, urease, and catalase, respectively. The soil samples were assigned to Shanghai Personal Biotechnology Co., Ltd. for the analysis of the soil microbial organisms' structure and diversity using the Illumina MiSeq platform (Huang et al., 2015). A composition and abundance distribution table of each sample was obtained at the variety classification levels using QIIME (Quantitative Insights into Microbial Ecology) software. Then, using R software, principal components analysis (PCA), nonmetric multidimensional scaling (NMDS), and redundancy analysis (RDA) were performed on the genera-level community composition structure. Also, two-dimensional images were used to describe the natural distribution characteristics of the samples.
Means and standard deviation (SD) were calculated by Microsoft Office Excel 2019. The analysis of variance was performed by one-way ANOVA at a significance level of p < 0.05 using SPSS 26.0.
The soil physicochemical properties have changed by the addition of nano-montmorillonite. Amending soils with NMMT showed a slight increase in pH by 0.21, 0.17, and 0.08 units, compared with unamended soils on the 40th day (Figure 1A). The slightly increased soil pH was due to the alkali activation of montmorillonite. Similarly, Qin et al. (2020) observed that the pH after modified montmorillonite addition was higher than that of control. The acidic conditions increased the bioavailability and mobility of heavy metals, while in alkaline environments, the solubility of heavy metal ions decreased due to hydrolysis reaction and electrostatic adsorption. Additionally, the alkalinity of nano-montmorillonite may dissolve carbon dioxide in the soil pores, resulting in the precipitation of cadmium. The EC of all treatments was less than 0.5 ms/cm (Figure 1B). With the extension of plant culture, the EC remarkably reduced (p < 0.05), which can be the reason for the salts leaching and uptake by the plant. On the 40th day, the EC of the soil with NMMT applied at a rate of 0.5% was significantly lower than that of the non-amended sample. Soil salinity may affect the bioavailability and absorption of heavy metals in the soil because soil salinity and related biological stress may affect the ultimate demand for optimal plant growth and may have a synergistic/antagonistic effect with heavy metals (Chen et al., 2020). Raiesi and Sadeghi (2019) reported that the bioavailability of lead was significantly positively correlated with the increase in soil salinity. In the present research study, the electrical conductivity and cadmium also showed a positive correlation (Supplementary Figure S3). Therefore, the decrease in electrical conductivity inhibited the mobility of ions in the soil environment.
FIGURE 1. Effects of soil pH (A) and EC (B) under various NMMT treatments. Mean values denoted by the same letter in a column do not differ significantly according to the Duncan test (p < 0.05).
The changes of DTPA-extractable Cd were first increased and then decreased in all soil samples (Figure 2A) during the whole plant culture. With the addition of amendments to the soil, the metal will slowly and gradually transform into a more stable form. Therefore, the cultured time is one of the key factors for soil remediation. The results on the 20th day showed that NMMT “activate” Cd in soil, which promoted the conversion of other forms of cadmium into an effective state. When the soil sample was added with 0.2%, 0.5%, and 1% NMMT, the concentrations of extractable Cd significantly decreased by 0.40–7.10%, 9.28–22.66%, and 3.99–5.59% between 40th and 60th day, respectively, compared with the samples without NMMT treatments. The 0.5% NMMT treatment had the lowest available cadmium content (1.94 mg/kg) on the 60th day. It can be inferred that the active sites and functional groups on the nano-montmorillonite surface promote the stabilization of cadmium through complexation and cation exchange (Raiesi and Sadeghi, 2019; Peng et al., 2020). For cadmium dynamics of NMMT-treated soil, a large number of documents have demonstrated that ion exchange was the dominant mechanism of Cd sorption by bentonite and montmorillonite (Liu et al., 2018). In addition, the slight increase in soil pH after applying amendment may promote the adsorption of cadmium. A significant negative correlation between soil pH and the concentration of DTPA-Cd was also observed (Supplementary Figure S3), demonstrating that soil pH contributed to the cadmium bioavailability. Liu et al. (2018) showed the amendment treatments increased the soil pH to decrease the concentrations of DTPA-Cd in Cd-polluted soil. For one aspect, an increase in soil pH enhances the surface charge of soil clay minerals, soil organic matter content, and soil adsorption capacity for heavy metals (Zhou et al., 2014; Song et al., 2017a). For another aspect, when the pH increases, the increase in pH changes the passive adsorption of heavy metals into specific adsorption, which leads to a decrease in the concentration of soil-available heavy metals (Peng et al., 2020). Thus, nano-montmorillonite has a great effect on the enhancement of heavy metal immobilization in polluted soil. Based on the results that the soil pH increased and DTPA-Cd reduced by the application of nano-montmorillonite, we believe that future research should focus on the role of the nano-montmorillonite amendment in improving Cd-contaminated acidic soil.
FIGURE 2. Effects of NMMT application on Cd concentration in soil (A) and uptake in plants (B). The letters indicate significant differences between members of the same set (p < 0.05).
The effect of amendments on Cd concentration in plants is illustrated in Figure 2B. With an increase in applying NMMT in soils, Cd contents in plants decreased by 1.60–16.10%, 0.52–4.62%, and 1.96–4.43%, respectively, compared with that in non-amended soil, although no significant differences were detected during the mature period (p > 0.05). The enrichment of Cd in plants mainly depends on the available cadmium content in the soil. On one hand, more available heavy metal was stabilized by NMMT in the soil, and the corresponding risk of Cd migration to plants was reduced. On the other hand, soil is a complex ecosystem, and clay components in the soil also could fix Cd ions in the soil (Hamid et al., 2019; Houben et al., 2013). Because of the complicated processes among ions, clay components, roots, NMMT, and cadmium in soil, the addition of up to 1% NMMT did not further reduce Cd accumulation in Brassica campestris L.
During the plant cultivation, the ecology of oilseed rape growth was normal, and there were no obvious symptoms of heavy metal toxicity. The plant biomass after the 40th day of incubation was significantly (p < 0.05) higher than within the 20th day (Figure 3). Compared with the control, the plant biomass was enhanced by 3.24–21.43%, 3.03–12.96%, and 3.44–11.43% with NMMT application rates increasing, respectively (p > 0.05). The dry weight of 1% NMMT on 60th day was notably higher than that of other treatments (p < 0.05). Nevertheless, no statistically significant differences were observed among treatments for the other harvest times. High-Cd content in the soil, especially DTPA-Cd, was an important factor causing damage to plant roots, such as root cells. NMMT treatment can reduce Cd toxicity by changing a bioavailable fraction into a residual fraction and improving soil quality (Figure 2). NMMT, a green natural mineral, had another effect on plant growth by providing essential nutrients (Hamid et al., 2019). Therefore, the present results exhibited that the treatments of NMMT could inhibit the toxicity of cadmium to the oilseed rape and promote plant growth. The addition of up to 1% nano-montmorillonite did not further reduce cadmium accumulation in Brassica campestris L., which could be attributed easily to agglomerate material particles (Xu et al., 2019).
FIGURE 3. Effects of NMMT application on the dry weight. The letters indicate significant differences between members of the same set (p < 0.05).
Soil enzyme activities could act as a potential indicator to assess the remediation effect of the amendment on polluted soil and the improvement of soil quality (Yong et al., 2006; Sun et al., 2015). Catalase can decompose hydrogen peroxide into molecular oxygen and water, reducing the toxicity of hydrogen peroxide produced by soil and microorganisms in the metabolic process. The catalase activity of various treatments initially decreased and then remarkably increased with increasing cultivation time (Figure 4A). All nano-montmorillonite treatments tend to stably increase catalase activity, making its level significantly higher than that obtained with the unamended treatment. With an increase in the concentration of NMMT in soils, catalase activity increased accordingly, resulting in 0.39–2.71%, 2.11–4.85%, and 5.43–8.94% increment, respectively, compared with the control. The maximum catalase activity was achieved with the 1% addition of NMMT. Catalase activity and soil-available cadmium content have a significant negative correlation (Supplementary Figure S3). Hence, the application of nano-montmorillonite can effectively reduce the toxicity of cadmium ions in the soil environment and then improve soil catalase activity. The effective stabilization of heavy metals can benefit soil functions by reducing the unstable element pool of heavy metals. Therefore, soil enzyme activity can be used to evaluate the effectiveness of the soil remediation process (Raiesi and Sadeghi, 2019). In our study, the catalase activity can act as an indicator of improvements to the soil environment.
FIGURE 4. Effects of NMMT application on the activities of catalase (A), urease (B) and dehydrogenase (C). Letters above the bar diagram refer to the difference at a significance level of p < 0.05.
Soil urease activity can reflect the level and capacity of soil nitrogen supply and is often used as one of the indicators of soil fertility determination (Hamid et al., 2019). The urease activity of soil treatments all had the maximum value when sampling time on the 40th day (Figure 4B). After 60 days of plant cultivation, compared to control, the urease activity significantly increased by 4.67%, 15.40%, and 18.65% with increasing additions of NMMT (p < 0.05). Urease can catalyze the hydrolysis of urea to produce NH4− and CO32-. These products will increase the pH of the soil and combine with Cd2+ to generate CdCO3, thereby reducing the available content of cadmium in the soil (Sun et al., 2013). The application of NMMT inhibited the toxicity of heavy metal cadmium to a certain extent and increased biological activity.
Dehydrogenase was often considered one of the most sensitive indicators of the toxic effects of inorganic pollutants on soil microbes (Lebrun et al., 2012; Teulada and Xia, 1996). Dehydrogenase activity can be used for soil pollution warning systems and soil adaptive response mechanism evaluation. The soil dehydrogenase activity for NMMT treatments showed a small increase within the 40th day, compared to control (Figure 4C). The highest dehydrogenase activity (23.91 ug/d/g) was observed in the non-amendment soil, probably related to the higher total cadmium content in the soil relative to other treatments (Lebrun et al., 2012). During whole plant cultivation, the dehydrogenase activity of the soil treated with 0.2% NMMT gradually reduced, whereas the activity of other treatments decreased at first and then increased. The soil catalase and urease activities improved after applying the amendment, indicating that the enzyme activity may be affected by the effective cadmium concentration. In contrast, the inhibition of dehydrogenase activity in the nano-montmorillonite treatment soil indicated that it may not be suitable for evaluating soil restoration after cadmium immobilization.
This study evaluated the alpha diversity of the experimental soil samples by calculating the Chao1 index and Shannon index. Chao described the species richness of the samples, whereas the Shannon indices evaluated the species diversity of the community. The alpha diversity indices of the soil bacterial 16S rRNA gene are shown in Figure 5. The Chao1 index and Shannon index in the soil with the addition of nano-montmorillonite decreased by 3.02%–24.33% based on the control treatment, which may be due to the mild sterilization of the montmorillonite. The lower the Chao1 index of the amendment soil, the rarer species that may disappear. With the prolonged plant culture time, the Chao1 index of all soil samples (excluding the 0.5% NMMT treated) showed a decreasing trend. The addition of the NMMT restored the indices value, as well as 0.5% of the treatment on the 60th day, which was higher than the value on the tenth day. The change in the Shannon index between different treatments was not very obvious, and its changing trend was the same as the Chao1 index. The alpha diversity indices in the 0.5% addition of nano-montmorillonite were the largest on the 60th day, compared to other treatments. The extension of plant cultivation time reduces the bactericidal effect of the amendment. This may be due to the addition of nano-montmorillonite that improves some of the physical and chemical properties of the soil, including pH, cadmium available state, and enzyme activity. Sun et al. (2016) also reported that the microbial community richness was improved by the bentonite in cadmium-polluted soil. Thus, the diversity of soil microbial was maintained as well as the stability and functionality by nano-montmorillonite in Cd-contaminated soil.
FIGURE 5. The alpha diversity indices of the soil bacterial 16S rRNA gene. Letters a, b, c, and d represent 0%, 0.2%, 0.5%, and 1% NMMT added in Cd-contaminated soil, respectively.
The unweighted PCA and NMDS analysis not only reflected the diversity and abundance of microbial communities but also reflected the similarity and difference of bacteria under different treatments. The difference in the amount of NMMT added and the plant growth stage greatly affected the analysis of soil microbial diversity (Figure 6). The first and second principal components accounted for 38.86% and 22.12% of the total variation in the overall bacterial community structure, respectively. The bacterial communities in the soil of the control group and the 0.2% addition of the NMMT group were distributed on the left side of the figure, while the 0.5% and 1% application groups with more nano-montmorillonite were distributed on the right side of the figure. The NMDS analysis also showed similar differences in the community structure. Treatments of 1% NMMT addition were separated to the far on the right in the PCA analysis. Therefore, the observed phenomena indicated that nano-montmorillonite in soil samples may play a key role in cadmium contamination and therefore change the composition of bacterial communities. The NMMT addition would improve the soil microbial community in cadmium-polluted soil. In addition, soil samples were divided by plant culture time at a great distance, which indicated that time had a considerable influence on the microbial community composition. Thus, further investigations on nano-montmorillonite immobilization properties and the duration effect are necessary to explain the microbial population difference.
FIGURE 6. The principal component analysis (PCA) and nonmetric multidimensional scaling (NMDS) analysis of bacterial communities based on the European distance of 16S V4 region gene sequencing for each soil sample. Letters a, b, c, and d represent 0%, 0.2%, 0.5%, and 1% NMMT added in Cd-contaminated soil, respectively.
To finally confirm the specific variation of the bacterial community, a phylogenetic analysis was carried out to explore the dynamics of soil microbial communities. As shown in Figure 7, the dominant phyla of all samples were Proteobacteria, Acidobacteria, Planctomycetes, and Gemmatimonadetes, which account for 63.38–78.75% across the whole process of plant culture. The relative abundance of Proteobacteria increased in NMMT-treated soils, which suggested that nano-montmorillonite had a positive effect on promoting the growth of these bacteria. Similar results were found at the class level (Supplementary Figure S2). Usually, the structure of soil microbial communities was driven by environmental changes (Huang et al., 2015). On the one hand, nano-montmorillonite may indirectly promote the enrichment of bacteria by reducing the toxicity of cadmium. On the other hand, clay micro-aggregates were also conducive to the microbial interaction network to change the niche of microorganisms (Li et al., 2016; Fernández-Bayo et al., 2017; Jenkins et al., 2017). On the contrary, as the amount of NMMT increased, the relative abundances of Acidobacteria and Actinobacteria were decreased, while the control treatment had the highest abundance, which may be due to the increase in soil pH. It was shown that the existence of nano-montmorillonite damaged some sensitive bacteria phyla. Acidobacteria was inclined to acidic environments, which was consistent with the study of Wu et al. (2019). As reported, soil texture (clay content) and physicochemical properties (pH and organic matter) are key driving factors for the structure of soil bacterial communities, and clay content had a negative impact on most bacteria in the soil (Raiesi and Sadeghi, 2019; Liao et al., 2021). Montmorillonite is a typical clay, and its addition will both affect the pH of the soil and affect the texture of the soil. Therefore, the addition of NMMT may inhibit the activity of some microorganisms. Compared to other treatments, the 0.5% addition of NMMT treatments remarkably increased the abundance of Planctomycetes and Bacteroidetes, which may be contributed to the conversion of organic carbon bioavailability (Huang et al., 2015). With the extension of plant cultivation, the RA of the Proteobacteria for the soil treated with 0.5% NMMT decreased, which was opposite to other treatments. The changes in these bacteria may be related to the correlation between the bacteria and cadmium bioavailability (Gan et al., 2021). Qin et al. (2020) found that the addition of montmorillonite made the relationship between microorganisms and heavy metal availability more complicated. This may be because montmorillonite promotes the activity of key bacteria to a certain extent and activates the expression of some functional genes. This result may be the reason why nano-montmorillonite decreased the bioavailability of cadmium.
FIGURE 7. Relative abundance (RA) of bacterial community structures based on the phylum levels of soils with various NMMT treatment amounts. Letters a, b, c, and d were marked 0%, 0.2%, 0.5%, and 1% NMMT added in contaminated soil, respectively.
The present study demonstrated that the NMMT soil treatment can reduce the soil-available cadmium concentration. Nano-montmorillonite treatments stimulated catalase and urease activity, which were increased by 8.94% and 18.65%, respectively. Furthermore, our findings underscore the role of nano-montmorillonite in providing a more favorable ecological environment for microorganisms. Based on 16S rRNA analysis, microbial diversity was increased by the nano-montmorillonite of the soil and enhanced the RA of Bacteroidetes and Planctomycetes, compared to the control, respectively. An addition of 0.5% NMMT was suggested for soils containing 5 mg/kg Cd. However, to meet the long-term needs of agricultural farming and obtain a more practical application of the data, more extensive studies are required to confirm its reliability and long-term use.
The datasets presented in this study can be found in online repositories. The names of the repository/repositories and accession number(s) can be found at: NCBI with BioProject ID PRJNA822067 (https://www.ncbi.nlm.nih.gov/bioproject/?term=PRJNA822067).
WL: investigation, methods, and writing—review and editing. CZ: investigation, methods, writing—original draft preparation, and revision. YY: writing—review and editing. XS: writing—review and editing. HZ: writing—review and editing. SW: writing—review and editing.
This study is supported by the Natural Science Interdisciplinary Research Program of Hebei University (DXK201907).
The authors declare that the research was conducted in the absence of any commercial or financial relationships that could be construed as a potential conflict of interest.
All claims expressed in this article are solely those of the authors and do not necessarily represent those of their affiliated organizations, or those of the publisher, the editors, and the reviewers. Any product that may be evaluated in this article, or claim that may be made by its manufacturer, is not guaranteed or endorsed by the publisher.
The Supplementary Material for this article can be found online at: https://www.frontiersin.org/articles/10.3389/fenvs.2022.908819/full#supplementary-material
Bolan, N., Kunhikrishnan, A., Thangarajan, R., Kumpiene, J., Park, J., Makino, T., et al. (2014). Remediation of Heavy Metal(loid)s Contaminated Soils - to Mobilize or to Immobilize? J. Hazard. Mater. 266, 141–166. doi:10.1016/j.jhazmat.2013.12.018
Chang, T.-P., Shih, J.-Y., Yang, K.-M., and Hsiao, T.-C. (2007). Material Properties of Portland Cement Paste with Nano-Montmorillonite. J. Mat. Sci. 42, 7478–7487. doi:10.1007/s10853-006-1462-0
Chen, C., Liu, H., Chen, T., Chen, D., and Frost, R. L. (2015). An Insight into the Removal of Pb(II), Cu(II), Co(II), Cd(II), Zn(II), Ag(I), Hg(I), Cr(VI) by Na(I)-montmorillonite and Ca(II)-montmorillonite. Appl. Clay Sci. 118, 239–247. doi:10.1016/j.clay.2015.09.004
Chen, Y., Chen, F., Xie, M., Jiang, Q., Chen, W., and Ao, T. (2020). The Impact of Stabilizing Amendments on the Microbial Community and Metabolism in Cadmium-Contaminated Paddy Soils. Chem. Eng. J. 395, 125132. doi:10.1016/j.cej.2020.125132
Fernández-Bayo, J. D., Achmon, Y., Harrold, D. R., Claypool, J. T., Simmons, B. A., Singer, S. W., et al. (2017). Comparison of Soil Biosolarization with Mesophilic and Thermophilic Solid Digestates on Soil Microbial Quantity and Diversity. Appl. Soil Ecol. 119, 183–191. doi:10.1016/j.apsoil.2017.06.016
Gan, C.-d., Jia, Y.-b., and Yang, J.-y. (2021). Remediation of Fluoride Contaminated Soil with Nano-Hydroxyapatite Amendment: Response of Soil Fluoride Bioavailability and Microbial Communities. J. Hazard. Mater. 405, 124694. doi:10.1016/j.jhazmat.2020.124694
Gil-Díaz, M., Pinilla, P., Alonso, J., and Lobo, M. C. (2017). Viability of a Nanoremediation Process in Single or Multi-Metal(loid) Contaminated Soils. J. Hazard. Mater. 321, 812–819. doi:10.1016/j.jhazmat.2016.09.071
González-Alcaraz, M. N., Loureiro, S., and van Gestel, C. A. M. (2018). Toxicokinetics of Zn and Cd in the Earthworm Eisenia andrei Exposed to Metal-Contaminated Soils under Different Combinations of Air Temperature and Soil Moisture Content. Chemosphere 197, 26–32. doi:10.1016/j.chemosphere.2018.01.019
Guo, G., Zhou, Q., and Ma, L. Q. (2006). Availability and Assessment of Fixing Additives for the In Situ Remediation of Heavy Metal Contaminated Soils: A Review. Environ. Monit. Assess. 116, 513–528. doi:10.1007/s10661-006-7668-4
Hamid, Y., Tang, L., Yaseen, M., Hussain, B., Zehra, A., Aziz, M. Z., et al. (2019). Comparative Efficacy of Organic and Inorganic Amendments for Cadmium and Lead Immobilization in Contaminated Soil under Rice-Wheat Cropping System. Chemosphere 214, 259–268. doi:10.1016/j.chemosphere.2018.09.113
He, Y., Shen, D., Fang, C., He, R., and Zhu, Y. (2006). Effects of Metsulfuron-Methyl on the Microbial Population and Enzyme Activities in Wheat Rhizosphere Soil. J. Environ. Sci. Health, Part B Pesticides, Food Contam. Agric. Wastes 41, 269–284. doi:10.1080/03601230500357298
Huang, D., Xue, W., Zeng, G., Wan, J., Chen, G., Huang, C., et al. (2016). Immobilization of Cd in River Sediments by Sodium Alginate Modified Nanoscale Zero-Valent Iron: Impact on Enzyme Activities and Microbial Community Diversity. Water Res. 106, 15–25. doi:10.1016/j.watres.2016.09.050
Huang, X., Liu, L., Wen, T., Zhu, R., Zhang, J., and Cai, Z. (2015). Illumina MiSeq Investigations on the Changes of Microbial Community in the Fusarium Oxysporum f.Sp. Cubense Infected Soil during and after Reductive Soil Disinfestation. Microbiol. Res. 181, 33–42. doi:10.1016/j.micres.2015.08.004
Jenkins, J. R., Viger, M., Arnold, E. C., Harris, Z. M., Ventura, M., Miglietta, F., et al. (2017). Biochar Alters the Soil Microbiome and Soil Function: Results of Next-Generation Amplicon Sequencing across Europe. GCB Bioenergy 9, 591–612. doi:10.1111/gcbb.12371
Jeyasundar, P. G. S. A., Ali, A., Azeem, M., Li, Y., Guo, D., Sikdar, A., et al. (2021). Green Remediation of Toxic Metals Contaminated Mining Soil Using Bacterial Consortium and Brassica Juncea. Environ. Pollut. 277, 116789. doi:10.1016/j.envpol.2021.116789
Khan, M. A., Khan, S., Khan, A., and Alam, M. (2017). Soil Contamination with Cadmium, Consequences and Remediation Using Organic Amendments. Sci. Total Environ. 601-602, 1591–1605. doi:10.1016/j.scitotenv.2017.06.030
Lebrun, J. D., Trinsoutrot-Gattin, I., Vinceslas-Akpa, M., Bailleul, C., Brault, A., Mougin, C., et al. (2012). Assessing Impacts of Copper on Soil Enzyme Activities in Regard to Their Natural Spatiotemporal Variation under Long-Term Different Land Uses. Soil Biol. Biochem. 49, 150–156. doi:10.1016/j.soilbio.2012.02.027
Li, Z., Zu, C., Wang, C., Yang, J., Yu, H., and Wu, H. (2016). Different Responses of Rhizosphere and Non-rhizosphere Soil Microbial Communities to Consecutive Piper Nigrum L. Monoculture. Sci. Rep. 6, 1–8. doi:10.1038/srep35825
Liao, Q., He, L., Tu, G., Yang, Z., Yang, W., Tang, J., et al. (2021). Simultaneous Immobilization of Pb, Cd and as in Soil by Hybrid Iron-, Sulfate- and Phosphate-Based Bio-Nanocomposite: Effectiveness, Long-Term Stability and Bioavailablity/bioaccessibility Evaluation. Chemosphere 266, 128960. doi:10.1016/j.chemosphere.2020.128960
Liu, W., Zhao, C., Wang, S., Niu, L., Wang, Y., Liang, S., et al. (2018). Adsorption of Cadmium Ions from Aqueous Solutions Using Nano-Montmorillonite: Kinetics, Isotherm and Mechanism Evaluations. Res. Chem. Intermed. 44, 1441–1458. doi:10.1007/s11164-017-3178-y
Lothenbach, B., Furrer, G., Schärli, H., and Schulin, R. (1999). Immobilization of Zinc and Cadmium by Montmorillonite Compounds: Effects of Aging and Subsequent Acidification. Environ. Sci. Technol. 33, 2945–2952. doi:10.1021/es981317q
Peng, D., Qiao, S., Luo, Y., Ma, H., Zhang, L., Hou, S., et al. (2020). Performance of Microbial Induced Carbonate Precipitation for Immobilizing Cd in Water and Soil. J. Hazard. Mater. 400, 123116. doi:10.1016/j.jhazmat.2020.123116
Qin, C., Yuan, X., Xiong, T., Tan, Y. Z., and Wang, H. (2020). Physicochemical Properties, Metal Availability and Bacterial Community Structure in Heavy Metal-Polluted Soil Remediated by Montmorillonite-Based Amendments. Chemosphere 261, 128010. doi:10.1016/j.chemosphere.2020.128010
Raiesi, F., and Sadeghi, E. (2019). Interactive Effect of Salinity and Cadmium Toxicity on Soil Microbial Properties and Enzyme Activities. Ecotoxicol. Environ. Saf. 168, 221–229. doi:10.1016/j.ecoenv.2018.10.079
Seifpanahi Shabani, K., Doulati Ardejani, F., Badii, K., and Olya, M. E. (2017). Preparation and Characterization of Novel Nano-Mineral for the Removal of Several Heavy Metals from Aqueous Solution: Batch and Continuous Systems. Arabian J. Chem. 10, S3108–S3127. doi:10.1016/j.arabjc.2013.12.001
Sharma, R. K., and Archana, G. (2016). Cadmium Minimization in Food Crops by Cadmium Resistant Plant Growth Promoting Rhizobacteria. Appl. Soil Ecol. 107, 66–78. doi:10.1016/j.apsoil.2016.05.009
Song, B., Zeng, G., Gong, J., Liang, J., Xu, P., Liu, Z., et al. (2017a). Evaluation Methods for Assessing Effectiveness of In Situ Remediation of Soil and Sediment Contaminated with Organic Pollutants and Heavy Metals. Environ. Int. 105, 43–55. doi:10.1016/j.envint.2017.05.001
Song, B., Zeng, G., Gong, J., Zhang, P., Deng, J., Deng, C., et al. (2017b). Effect of Multi-Walled Carbon Nanotubes on Phytotoxicity of Sediments Contaminated by Phenanthrene and Cadmium. Chemosphere 172, 449–458. doi:10.1016/j.chemosphere.2017.01.032
Song, J., Zhang, S., Li, G., Du, Q., and Yang, F. (2020). Preparation of Montmorillonite Modified Biochar with Various Temperatures and Their Mechanism for Zn Ion Removal. J. Hazard. Mater. 391, 121692. doi:10.1016/j.jhazmat.2019.121692
Suazo-Hernández, J., Manquián-Cerda, K., de la Luz Mora, M., Molina-Roco, M., Angélica Rubio, M., Sarkar, B., et al. (2021). Efficient and Selective Removal of SeVI and AsV Mixed Contaminants from Aqueous Media by Montmorillonite-Nanoscale Zero Valent Iron Nanocomposite. J. Hazard. Mater. 403, 123639. doi:10.1016/j.jhazmat.2020.123639
Sun, R.-J., Chen, J.-H., Fan, T.-T., Zhou, D.-M., and Wang, Y.-J. (2016a). Effect of Nanoparticle Hydroxyapatite on the Immobilization of Cu and Zn in Polluted Soil. Environ. Sci. Pollut. Res. 25, 73–80. doi:10.1007/s11356-016-8063-5
Sun, Y., Li, Y., Xu, Y., Liang, X., and Wang, L. (2015). In Situ stabilization Remediation of Cadmium (Cd) and Lead (Pb) Co-contaminated Paddy Soil Using Bentonite. Appl. Clay Sci. 105-106, 200–206. doi:10.1016/j.clay.2014.12.031
Sun, Y., Sun, G., Xu, Y., Liu, W., Liang, X., and Wang, L. (2016b). Evaluation of the Effectiveness of Sepiolite, Bentonite, and Phosphate Amendments on the Stabilization Remediation of Cadmium-Contaminated Soils. J. Environ. Manag. 166, 204–210. doi:10.1016/j.jenvman.2015.10.017
Sun, Y., Sun, G., Xu, Y., Wang, L., Liang, X., and Lin, D. (2013). Assessment of Sepiolite for Immobilization of Cadmium-Contaminated Soils. Geoderma 193-194, 149–155. doi:10.1016/j.geoderma.2012.07.012
Usman, A. R. A., Kuzyakov, Y., Lorenz, K., and Stahr, K. (2006). Remediation of a Soil Contaminated with Heavy Metals by Immobilizing Compounds. Z. Pflanzenernähr. Bodenk. 169, 205–212. doi:10.1002/jpln.200421685
Wenhao, Y., Hong, H., Mei, R., and Wuzhong, N. (2013). Changes of Microbial Properties in (Near-) Rhizosphere Soils after Phytoextraction by Sedum Alfredii H: A Rhizobox Approach with an Artificial Cd-Contaminated Soil. Appl. Soil Ecol. 72, 14–21. doi:10.1016/j.apsoil.2013.05.006
Wu, C., Shi, L., Xue, S., Li, W., Jiang, X., Rajendran, M., et al. (2019). Effect of Sulfur-Iron Modified Biochar on the Available Cadmium and Bacterial Community Structure in Contaminated Soils. Sci. Total Environ. 647, 1158–1168. doi:10.1016/j.scitotenv.2018.08.087
Xing, Y., Luo, X., Liu, S., Wan, W., Huang, Q., and Chen, W. (2020). Synergistic Effect of Biofilm Growth and Cadmium Adsorption via Compositional Changes of Extracellular Matrix in Montmorillonite System. Bioresour. Technol. 315, 123742. doi:10.1016/j.biortech.2020.123742
Xu, C., Qi, J., Yang, W., Chen, Y., Yang, C., He, Y., et al. (2019). Immobilization of Heavy Metals in Vegetable-Growing Soils Using Nano Zero-Valent Iron Modified Attapulgite Clay. Sci. Total Environ. 686, 476–483. doi:10.1016/j.scitotenv.2019.05.330
Xu, Y., Liang, X., Xu, Y., Qin, X., Huang, Q., Wang, L., et al. (2017). Remediation of Heavy Metal-Polluted Agricultural Soils Using Clay Minerals: A Review. Pedosphere 27, 193–204. doi:10.1016/S1002-0160(17)60310-2
Yang, F., Chen, Y., Huang, Y., Cao, X., Zhao, L., Qiu, H., et al. (2021). New Insights into the Underlying Influence of Bentonite on Pb Immobilization by Undissolvable and Dissolvable Fractions of Biochar. Sci. Total Environ. 775, 145824. doi:10.1016/j.scitotenv.2021.145824
Zhang, M., and Pu, J. (2011). Mineral Materials as Feasible Amendments to Stabilize Heavy Metals in Polluted Urban Soils. J. Environ. Sci. 23, 607–615. doi:10.1016/S1001-0742(10)60455-X
Zhao, Y., Mao, G., Han, S., and Gao, L. (2015). Effect of Namomaterials on Heavy Metal Transport in Alkaline Soil. Soil Sediment Contam. Int. J. 24, 694–703. doi:10.1080/15320383.2015.1001057
Keywords: nano-montmorillonite, remediation, cadmium, enzyme activity, microbial diversity
Citation: Liu W, Zhao C, Yuan Y, Song X, Zhao H and Wang S (2022) Physicochemical Properties, Metal Availability, and Bacterial Community Structure in Cadmium-Contaminated Soil Immobilized by Nano-Montmorillonite. Front. Environ. Sci. 10:908819. doi: 10.3389/fenvs.2022.908819
Received: 31 March 2022; Accepted: 22 April 2022;
Published: 08 June 2022.
Edited by:
Chuan Wu, Central South University, ChinaReviewed by:
Bhaba Biswas, The University of Newcastle, AustraliaCopyright © 2022 Liu, Zhao, Yuan, Song, Zhao and Wang. This is an open-access article distributed under the terms of the Creative Commons Attribution License (CC BY). The use, distribution or reproduction in other forums is permitted, provided the original author(s) and the copyright owner(s) are credited and that the original publication in this journal is cited, in accordance with accepted academic practice. No use, distribution or reproduction is permitted which does not comply with these terms.
*Correspondence: Wei Liu, YXVobHc4MEAxMjYuY29t
Disclaimer: All claims expressed in this article are solely those of the authors and do not necessarily represent those of their affiliated organizations, or those of the publisher, the editors and the reviewers. Any product that may be evaluated in this article or claim that may be made by its manufacturer is not guaranteed or endorsed by the publisher.
Research integrity at Frontiers
Learn more about the work of our research integrity team to safeguard the quality of each article we publish.