- 1State Key Laboratory of Plateau Ecology and Agriculture, College of Eco-Environmental Engineering, Qinghai University, Xining, China
- 2Key Laboratory of Restoration Ecology for Cold Regions Laboratory in Qinghai, Northwest Institute of Plateau Biology, Chinese Academy of Sciences, Xining, China
- 3Key Laboratory of Adaptation and Evolution of Plateau Biota, Northwest Institute of Plateau Biology, Chinese Academy of Sciences, Xining, China
- 4Qinghai Provincial Forage Seed Breeding Farm, Tongde, China
The forage yield and quality of artificial grasslands typically decline rapidly over time after planting. Moreover, the reason for this decline of artificial grassland remains unclear. Here, we evaluated the relationship between years since planting and annual net primary productivity (ANPP), seed yield, and reproductive allocation (ie., the ratio of seed yield to annual net primary productivity) for eight common gramineous species separately planted in monoculture artificial grasslands in the Qinghai-Tibetan Plateau. We utilized T50 values (the time when ANPP or seed yield drop to 50% of their maximum values) to quantify the decline rates of eight artificial grasslands respectively, and additionally evaluated the influence of evolutionary history and other plant attributes on T50 (ANPP) and T50 (seed yield). We found that both ANPP and seed yield decreased linearly over time following planting for all species. ANPP generally declined more rapidly than did seed yield. The reproductive allocation of Deschampsia cespitosa, Festuca kirilowii, Festuca kryloviana, and Festuca sinensis increased significantly over time after planting. We did not observe any association of evolutionary history, seed mass, plant height, reproductive allocation, ANPP (second year), seed yield (second year) with T50 (ANPP) and T50 (seed yield). In addition, clonal propagation mode may influence declines of artificial grassland, and evolutionary history may shape shifts in reproductive allocation with plant population aging. This study was the first to utilize T50 values for the study of artificial grassland declines. Our research framework thus has the potential to guide future quantitative studies of artificial grassland declines.
Introduction
Artificial grassland is a grassland cultivated by agricultural technical measures (Zhang and Liu 2004). Artificial grassland establishment is an important approach to restoring the productivity of degraded grasslands and alleviating the contradiction between grass and livestock in pastoral areas (Shang et al., 2008; Wu et al., 2010; Ma et al., 2017). Appropriately establishing artificial grasslands can facilitate social and economic sustainability in pastoral areas (Shang et al., 2008; Wu et al., 2010). However, the forage yield and quality of artificial grasslands typically decline rapidly after planting (about 3–5 years) (Zhou and Zhao, 1997; Krzic et al., 2003; Benjamin et al., 2004; Dong, 2010). As such, this decline of artificial grassland remains an important issue that warrants further research.
Plant attributes such as seed mass, plant height, seed yield, and annual net primary productivity (ANPP) can reflect plant competition strategies, nutrition acquisition strategies, and reproductive strategies (Grime, 1979; Westoby et al., 2002; Moles et al., 2009; Shipley, 2010; Ma et al., 2019; Zhang et al., 2019), all of which may in turn influence declines of plant population in monoculture artificial grasslands. For example, plant height can strongly influence the ability of a given plant to compete for light resources (Westoby et al., 2002; Moles et al., 2007), leading to high intraspecific competition within a given population of tall plants that can lead to rapid population decline. Higher ANPP and seed production can also consume more soil elements or drive the accumulation of soil pathogenic microorganisms (Ehrenfeld et al., 2005; Mariotte et al., 2018), thereby potentially driving rapid population decline.
In an evolutionary context, closely related species generally tend to share similar traits or niche preferences (Losos, 2008; Zhang et al., 2014, 2022). As such, the phylogenetic relatedness of species may influence differentiation in population decline rates for monoculture artificial grassland in this study.
Global climate change and anthropogenic disturbances have profoundly driven large-scale grassland degradation in the Tibetan Plateau over recent decades (Shang et al., 2008; Wu et al., 2010; Zhang et al., 2017). Deschampsia cespitosa, Festuca kirilowii, Festuca kryloviana, Festuca sinensis, Koeleria cristata, Poa crymophila, Poa pratensis, and Puccinellia tenuiflora are high-quality forage plants that are commonly utilized to establish artificial grassland in the Qinghai-Tibetan Plateau (Wang et al., 2015). Herein, we assessed the impact of time since planting on ANPP, seed yield, and reproductive allocation for these eight common monoculture artificial grasslands of alpine region in the Qinghai-Tibetan Plateau. Studying artificial grassland declines is complicated by the quantification of decline rates. In this study, we utilized the T50 values (the time when ANPP or seed yield dropped to 50%) to quantify the decline rates of monoculture artificial grasslands and further assessed the impact of evolutionary history and plant characteristics [seed mass, plant height, reproductive allocation, ANPP (second year) and seed yield (second year)] on T50 (ANPP) and T50 (seed yield). Through these efforts, we sought to provide a framework for the use of T50 values as a means of quantifying the decline rates of artificial grasslands so as to facilitate quantitative studies of artificial grassland declines. Lastly, we tested our hypothesis that plant attributes (e.g., seed mass, plant height, seed yield, and ANPP) and evolutionary history can influence declines of artificial grassland.
Methods
Dataset
The dataset used for the present study of ANPP and seed yields in artificial grasslands was previously described in detail by Wang et al. (2015) who compared ANPP and seed yields among species. Briefly, seeds of eight forage species used in the experiment were all provided by the technical extension station of Qinghai herbage breeding farm. For full details regarding tested species and corresponding attributes, see Supplementary Table S1. These eight forage species were planted in the experimental site (100°09 ′E, 35°09′ N; Supplementary Figure S1) of the technical extension station of Qinghai herbage breeding farm located in Tongde County, Qinghai Province on the eastern Qinghai-Tibetan Plateau. This site is located at an altitude of 3,280 m, has an average annual temperature of 0.2°C, and 429.8 mm of average annual precipitation. The experimental site has undergone artificial grassland planting for more than 20 years.
The experimental site was 400 m2 in area, with each plot being 3 m × 5 m (15 m2) in size. The distance between the two adjacent plots is 1 m. Each species was planted in three random replicate plots within the experimental site. In 2008, seeds were sown manually, with a row spacing of 30 cm and a seed depth of 2–3 cm. The sowing density of eight species was 0.39 g/m2 (D. caespitosa), 1.06 g/m2 (F. kirilovii), 0.49 g/m2 (F. kryloviana), 0.83 g/m2 (F. Sinersis), 0.36 g/m2 (P. crgmophila), 0.53 g/m2 (P. pratensis), 0.30 g/m2 (P. tenuiflora), and 0.47 g/m2 (K. cvistata), respectively. Weeds were removed once following seedling emergence during the initial planting year, and twice per year thereafter. ANPP and seed yield were monitored for all plots from 2009–2012. The aboveground biomass in a quadrant (1.0 m × 1.0 m) which was randomized between years in each plot was measured by harvesting individual plants within that quadrant at the soil surface at the flowering stage. These samples were then weighed to an accuracy of 0.01 g. Aboveground fresh biomass was taken as a proxy for ANPP in the present study. We additionally collected all seeds within another quadrant (1.0 m × 1.0 m) which was randomized between years in each plot at the stage of seed maturation. These samples were then weighed to an accuracy of 0.01 g, with weight serving as a proxy for seed yield. Reproductive allocation was calculated as the ratio of seed yield to ANPP. Data pertaining to seed mass for all eight species were obtained from Wang et al., 2015, and data pertaining to plant height were obtained from Wang et al., 2008, Sun et al., 2009 and Jing et al., 2017.
Phylogeny Construction
A hypothetical phylogenetic tree incorporating all studied species was constructed with the V. PhyloMaker R package (Jin and Qian, 2019), using the latest GBOTB tree including 74,533 species and all extant vascular plant families as a backbone for this analysis.
Statistical Analyses
ANPP, seed yield, and reproductive allocation were standardized prior to analysis by dividing them by their maximum values. Linear models were used to assess the relationship between ANPP, seed yield, reproductive allocation, and years since planting (i.e., population age). The T50 (ANPP) and T50 (seed yield) values were then estimated based on these linear models. T50 values are the time when ANPP or seed yield drop to 50% of their maximum values.
Phylogenetic signal in T50 (ANPP) and T50 (seed yield) was assessed using two commonly utilized metrics—Pagel’s λ (Pagel, 1999) and Blomberg’s K (Blomberg et al., 2003), both of which assess the degree to which a trait exhibits phylogenetic signal according to a Brownian motion model of evolution. Phylogenetic signal was estimated using the “phylosig” function in the phytools R package (v. 0.4e60) (Revell, 2012). A randomization test with 10,000 simulations was utilized to test for significant phylogenetic signal.
Lastly, the impacts of seed mass, plant height, reproductive allocation, ANPP (second year), and seed yield (second year) on T50 (ANPP) and T50 (seed yield) were assessed using linear models. All statistical testing was conducted using R (R Core Team, 2020).
Results
We observed a linear decrease in both ANPP and seed yield for the eight artificial grasslands with time since planting (Figure 1), such that years since planting were able to explain a large portion of the variation in ANPP (R2 > 65%; Supplementary Table S2) and seed yield (R2 > 75%; Supplementary Table S3) of artificial grasslands. The reproductive allocation of D. cespitosa, F. kirilowii, F. kryloviana, and F. sinensis increased gradually with time since planting, whereas no changes were observed over time for the other species (Figure 2; Supplementary Table S4).
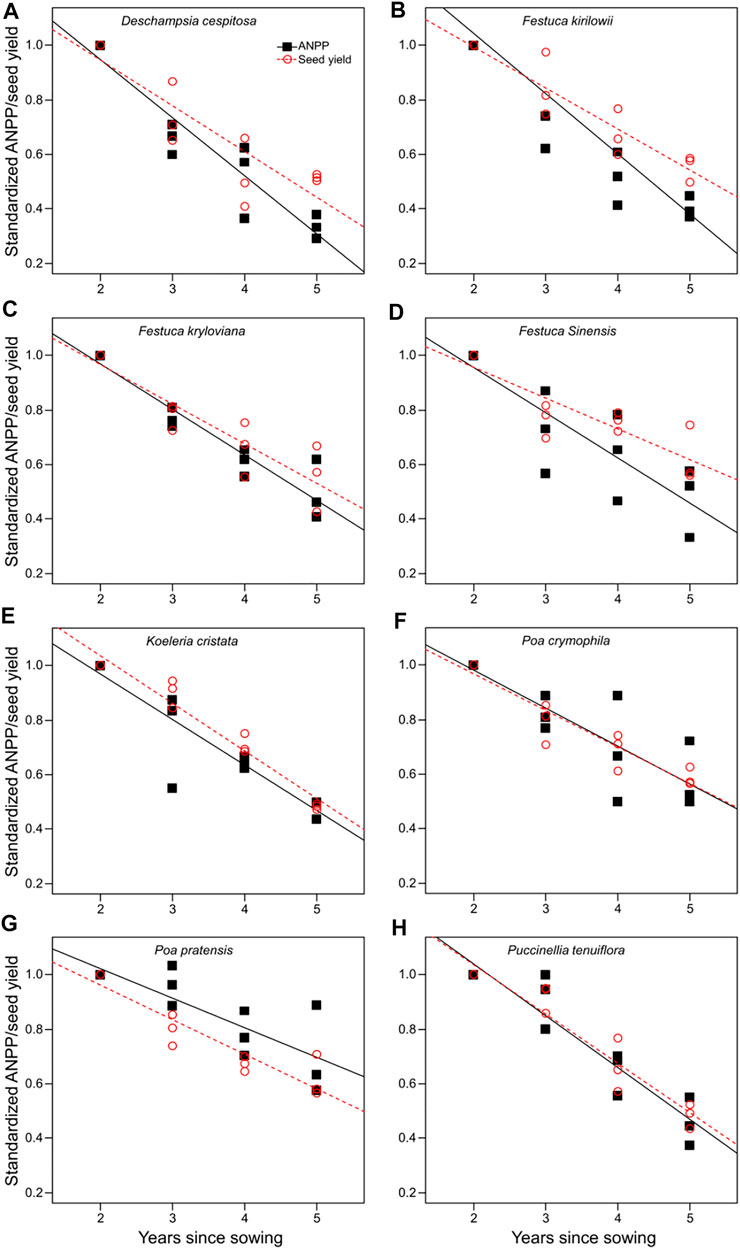
FIGURE 1. Relationship between ANPP, seed yield, and years since planting for each of eight species (A: Deschampsia cespitosa; B: Festuca kirilowii; C: Festuca kryloviana; D: Festuca sinensis; E: Koeleria cristata; F: Poa crymophila; G: Poa pratensis; H: Puccinellia tenuiflora). Data are presented in relation to the first year, treated here as 1. Significant relationships (p < 0.05) are denoted with solid (ANPP) or dashed (seed yield) lines. ANPP, seed yield, and reproductive allocation were standardized by dividing them by their maximum values.
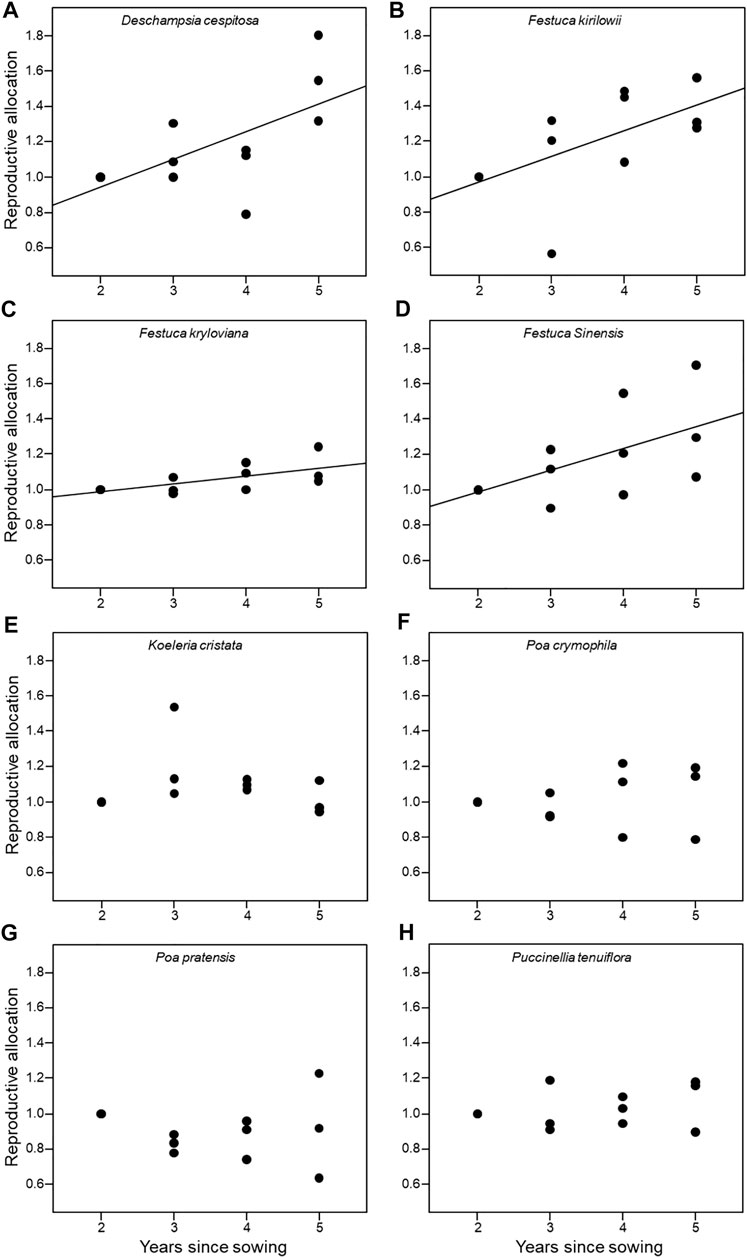
FIGURE 2. Relationships between reproductive allocation and years since planting for each of eight species (A: Deschampsia cespitosa; B: Festuca kirilowii; C: Festuca kryloviana; D: Festuca sinensis; E: Koeleria cristata; F: Poa crymophila; G: Poa pratensis; H: Puccinellia tenuiflora). Significant relationships (p < 0.05) are denoted with solid lines. Reproductive allocation was calculated as the ratio of seed yield to ANPP.
The T50 (ANPP) of D. cespitosa was the shortest of analyzed species (4.10 years), while that of P. pratensis was the longest (6.83 years) (Figure 3). The T50 (seed yield) of D. cespitosa was also the shortest of analyzed species (4.66 years), while that of F. sinensis was the longest (6.03 years) (Figure 3). As such, D. cespitosa grassland degraded most rapidly, with its ANPP and seed yield having been reduced by 50% at about 5 years after planting. With respect to ANPP, the decline rate of P. pratensis was the slowest, with ANPP having been reduced by half after 7 years. In terms of seed yield, F. sinensis exhibited the slowest decline rate, having been reduced by half after about 7 years. For all analyzed species other than P. pratensis, the ANPP decline rate was higher than that of seed yield (Figure 4).
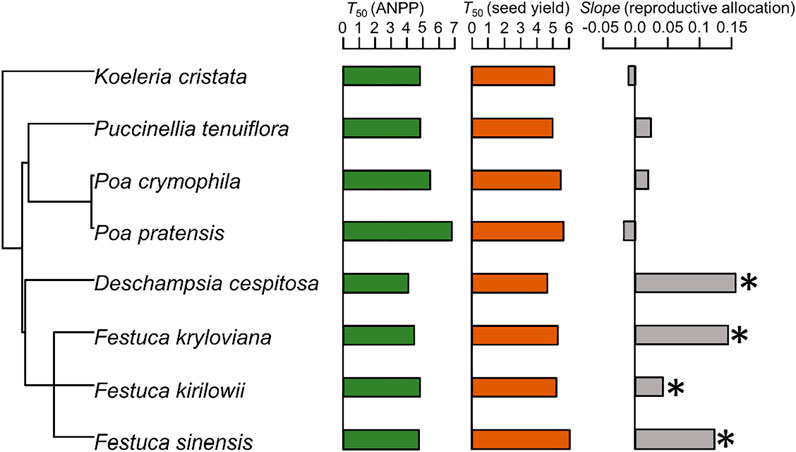
FIGURE 3. Visualization of the distribution of T50 (ANPP), T50 (seed yield) and slope (reproductive allocation) across phylogenetic tree. The slope was from linear models testing relationship between reproductive allocation and years after planting for each species. The asterisk indicates that the slope is significantly greater than 0.
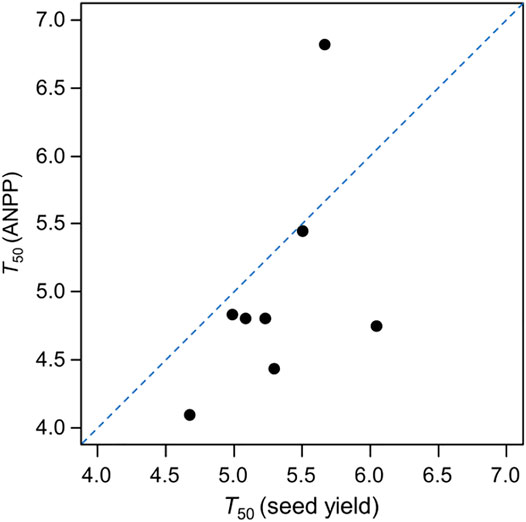
FIGURE 4. T50 (ANPP) and T50 (seed yield) for each species. The dashed line is the diagonal line with slope = 1.
We did not detect any significant phylogenetic signal for T50 (ANPP) or T50 (seed yield), nor did we observe any impact of seed mass, plant height, reproductive allocation, ANPP (second year), or seed yield (second year) on T50 (ANPP) (Supplementary Table S5) or T50 (seed yield) (Supplementary Table S6).
Discussion
Artificial grassland establishment is an important approach to restoring the productivity of degraded grasslands and alleviating the contradiction between grass and livestock in pastoral areas (Shang et al., 2008; Wu et al., 2010; Ma et al., 2017). However, artificial grassland declines remain an important problem that warrants further research. In this study, we found that ANPP and seed yield for eight artificial grasslands decreased significantly over time following planting, such that at 5–7 years after planting, the ANPP and seed yields of artificial grasslands decreased by 50%. We also found that the rate of ANPP decline was higher than the rate of seed yield decline for all analyzed species other than P. pratensis. It likely results from the life-history strategy that reproductive allocation is more important than growth allocation in ensuring population persistence (Weiner, 2004; Thomas, 2011).
P. pratensis exhibited the slowest rate of ANPP decline, with this rate being lower than that for corresponding seed yield. This may be attributable to the fact that P. pratensis was the only analyzed species that underwent rhizome propagation (Flora China Editing Group 2004). Relative to tillering propagation, rhizome propagation is more conducive to population colonization and regeneration in space (Wang, 1983; Zhang, 2018), and it additionally reduces intraspecific competition, thereby slowing population decline. P. pratensis may therefore allocate more biomass to rhizome propagation, such that its rate of seed production decline over time is greater than its corresponding decline in ANPP. In sum, the slow ANPP decline rate and good forage quality (Wang et al., 2015) of P. pratensis potentially make it become an excellent species for establishing artificial grassland in alpine areas. In addition, these results suggested that different clonal propagation modes may influence declines of artificial grassland.
Plant biomass allocation to different structures is a fundamental aspect of plant biology (Begon et al., 2006), with allocation patterns being reflective of evolutionary strategies associated with a range of selective pressures and limitations (Weiner, 2004). Interestingly, we found that reproductive allocation increased over time after planting for four species in analyzed artificial grasslands, indicating that these plants tended to allocate more of their biomass to seed production and seed regeneration over time. This is consistent with theoretical models suggesting that an optimal life-history strategy is one that entails a pattern of increasing reproductive allocation with age (Thomas, 2011). These data are also consistent with the results of certain empirical studies (Acosta et al., 1997; Lopez et al., 2001). We also found that the four species for which this result was observed were derived from a single phylogenetic branch, potentially affecting relationships between population age and reproductive allocation. Moreover, it suggested that evolutionary history may shape shifts in reproductive allocation with population aging.
A key aspect of this study was our investigation of reasons for artificial grassland declines. Our study suggested that clonal propagation mode may influence artificial grassland declines. However, we did not find influences of evolutionary history and other species attributes on artificial grassland declines. Future studies focused on larger numbers of species and more sampling sites will be necessary to fully explore these relationships in order to validate and expand upon our findings. In addition, abiotic attributes, such as soil nutrient elements and the presence of pathogenic microorganisms within the soil, may affect the declines of artificial grasslands (Dong, 2010), and thus warrant further research. In sum, factors driving artificial grassland declines are complex, and future research will be essential in order to fully elucidate them. Quantitative studies of artificial grassland declines are rare. We utilized T50 values (i.e., the time when ANPP or seed yield drop to 50% of their maximum values) to quantify the decline rates of artificial grassland. This study was also the first to utilize T50 values for the study of artificial grassland declines. Our research framework thus has the potential to guide future quantitative studies of artificial grassland declines.
Conclusion
In conclusion, we found that artificial grasslands underwent gradual declines over time, with ANPP declining faster on average relative to seed yield, thus resulting in reproductive allometry over time after planting. Moreover, evolutionary history may shape reproductive allometry with population aging. Although our study suggested that clonal propagation modes may influence declines of artificial grassland, the factors governing artificial grassland declines are complex and warrant further study. Importantly, the present study provides a framework for the use of T50 values to quantify artificial grassland decline rates, thus facilitating future quantitative analyses of artificial grassland declines.
Data Availability Statement
The original contributions presented in the study are included in the article/Supplementary Material, further inquiries can be directed to the corresponding authors.
Author Contributions
CZ and ZM conceived the study; XW, YR, LW, and DL collected the data; CZ developed the analyses with input from ZM and HZ; CZ, ZM, CL, YR, and LW wrote the manuscript and all authors contributed to revisions. All authors approved this final manuscript.
Funding
The Project of Qinghai Science and Technology Department (2020-ZJ-733), and the Open Project of State Key Laboratory of Plateau Ecology and Agriculture, Qinghai University (2017-ZZ-22). CZ was supported by the “1000 Talent” program of Qinghai Province.
Conflict of Interest
The authors declare that the research was conducted in the absence of any commercial or financial relationships that could be construed as a potential conflict of interest.
Publisher’s Note
All claims expressed in this article are solely those of the authors and do not necessarily represent those of their affiliated organizations, or those of the publisher, the editors and the reviewers. Any product that may be evaluated in this article, or claim that may be made by its manufacturer, is not guaranteed or endorsed by the publisher.
Supplementary Material
The Supplementary Material for this article can be found online at: https://www.frontiersin.org/articles/10.3389/fenvs.2022.907404/full#supplementary-material
References
Acosta, F. J., Delgado, J. A., López, F., and Serrano, J. M. (1997). Functional Features and Ontogenetic Changes in Reproductive Allocation and Partitioning Strategies of Plant Modules. Plant Ecol. 132, 71–76. doi:10.1023/A:1009799102985
Begon, M., Harper, J. L., and Townsend, C. R. (2006). Ecology: Individuals, Populations and Communities. 4th edn. Oxford: Blackwell.
Blomberg, S. P., Garland, T., Ives, A. R., and Crespi, B. (2003). Testing for Phylogenetic Signal in Comparative Data: Behavioral Traits Are More Labile. Evolution 57, 717–745. doi:10.1111/j.0014-3820.2003.tb00285.x
Dong, W. (2010). Effects of Rehabilitating Improvement Measures on Soil and Vegetation of Grain for Green Perennial Artificial Grassland in Guo Ma Ying Area. Dissertation: Lanzhou: Gansu Agricultural University.
Ehrenfeld, J. G., Ravit, B., and Elgersma, K. (2005). Feedback in the Plant-Soil System. Annu. Rev. Environ. Resour. 30, 75–115. doi:10.1146/annurev.energy.30.050504.144212
Jin, Y., and Qian, H. (2019). V.PhyloMaker: an R Package that Can Generate Very Large Phylogenies for Vascular Plants. Ecography 42, 1353–1359. doi:10.1111/ecog.04434
Jing, M., Ma, Y., Li, S., and Wang, Y. (2017). Initial Assessment of Sixteen Perennial Grass Species in the Upper Datong River. Acta pratacult. Sin. 26, 76–68. (in Chinese with English abstract). doi:10.11686/cyxb2016388
Krzic, M., Newman, R. F., and Broersma, K. (2003). Plant Species Diversity and Soil Quality in Harvested and Grazed Boreal Aspen Stands of Northeastern British Columbia. For. Ecol. Manag. 182, 315–325. doi:10.1016/S0378-1127(03)00064-1
López, F., Fungairiño, S., de las Heras, P., Serrano, J., and Acosta, F. (2001). Age Changes in the Vegetative vs. Reproductive Allocation by Module Demographic Strategies in a Perennial Plant. Plant Ecol. 157, 13–21. doi:10.1023/A:1014597832475
Losos, J. B. (2008). Phylogenetic Niche Conservatism, Phylogenetic Signal and the Relationship between Phylogenetic Relatedness and Ecological Similarity Among Species. Ecol. Lett. 11, 995–1003. doi:10.1111/j.1461-0248.2008.01229.x
Ma, Z., Zhang, C., Zhou, H.-K., Yao, B.-Q., and Zhao, X.-Q. (2017). Role of Seed Bank in Establishment of Single and Mixed-Sowing Artificial Grasslands of Tibetan Plateau. Pol. J. Ecol. 65, 334–344. doi:10.3161/15052249PJE2017.65.4.003
Ma, Z., Willis, C. G., Zhang, C., Zhou, H., Zhao, X., Dong, S., et al. (2019). Direct and Indirect Effect of Seed Size on Seedling Survival along an Experimental Light Availability Gradient. Agric. Ecosyst. Environ. 281, 64–71. doi:10.1016/j.agee.2019.05.009
Mariotte, P., Mehrabi, Z., Bezemer, T. M., De Deyn, G. B., Kulmatiski, A., Drigo, B., et al. (2018). Plant-soil Feedback: Bridging Natural and Agricultural Sciences. Trends Ecol. Evol. 33, 129–142. doi:10.1016/j.tree.2017.11.005
Moles, A. T., Ackerly, D. D., Tweddle, J. C., Dickie, J. B., Smith, R., Leishman, M. R., et al. (2007). Global Patterns in Seed Size. Glob. Ecol. Biogeogr. 16, 109–116. doi:10.1111/j.1466-8238.2006.00259.x
Moles, A. T., Warton, D. I., Warman, L., Swenson, N. G., Laffan, S. W., Zanne, A. E., et al. (2009). Global Patterns in Plant Height. J. Ecol. 97, 923–932. doi:10.1111/j.1365-2745.2009.01526.x
Pagel, M. (1999). Inferring the Historical Patterns of Biological Evolution. Nature 401, 877–884. doi:10.1038/44766
R Core Team (2020). R: A Language and Environment for Statistical Computing. Vienna, Austria: R Foundation for Statistical Computing. URL: https://www.R-project.org/.
Revell, L. J. (2012). Phytools: an R Package for Phylogenetic Comparative Biology (And Other Things). Methods Ecol. Evol. 3, 217–223. doi:10.1111/j.2041-210X.2011.00169.x
Shang, Z. H., Ma, Y. S., Long, R. J., and Ding, L. M. (2008). Effect of Fencing, Artificial Seeding and Abandonment on Vegetation Composition and Dynamics of 'black Soil Land' in the Headwaters of the Yangtze and the Yellow Rivers of the Qinghai-Tibetan Plateau. Land Degrad. Dev. 19, 554–563. doi:10.1002/ldr.861
Shipley, B. (2010). From Plant Traits to Vegetation Structure: Chance and Selection in the Assembly of Ecological Communities. Cambridge, UK: Cambridge University Press.
Sun, M., Shi, Y., Dou, S., and Mo, Y. (2009). The Comparative Test of 4 Species Plant of Festucal L. Qinghai Pratacult. 3, 8–11. (in Chinese with English abstract). doi:10.3969/j.issn.1008-1445.2009.03.002
Thomas, S. C. (2011). “Age-related Changes in Tree Growth and Functional Biology: The Role of Reproduction,”. Size- and Age-Related Changes in Tree Structure and Function. Tree Physiology. Editors F. Meinzer, B. Lachenbruch, and T. Dawson (Dordrecht, Netherlands: Springer), Vol. 4. doi:10.1007/978-94-007-1242-3_2
Tracy, B. F., Renne, I. J., Gerrish, J., and Sanderson, M. A. (2004). Effects of Plant Diversity on Invasion of Weed Species in Experimental Pasture Communities. Basic Appl. Ecol. 5, 543–550. doi:10.1016/j.baae.2004.08.007
Wang, C., Guo, S., and Cheng, H. (2008). Research of Cultivation and Domestication of Festuca Kivilovii Steud. Pratacult. Anim. Husb. 6, 11–23. (in Chinese with English abstract). doi:10.3969/j.issn.1673-8403.2008.06.004
Wang, X., Zhou, H., and Lei, Z. (2015). Variety Comparative Test for the Productive Economic Traits of Eight Kind of Short Grasses in Qinghai Pastoral Area. Heilongjiang J. Anim. Sci. Veter. Medic. 10, 21–22. (in Chinese with English abstract). doi:10.13881/j.cnki.hljxmsy.2015.0190
Wang, Y. (1983). Characteristics of Rhizome Propagation and Branch Formation of Several Rhizomatous Plants in Songnen Grassland and Determination of Their Annual Production. Grassl. China 4, 21–25. (in Chinese with English abstract).
Weiner, J. (2004). Allocation, Plasticity and Allometry in Plants. Perspect. Plant Ecol. Evol. Syst. 6, 207–215. doi:10.1078/1433-8319-00083
Westoby, M., Falster, D. S., Moles, A. T., Vesk, P. A., and Wright, I. J. (2002). Plant Ecological Strategies: Some Leading Dimensions of Variation between Species. Annu. Rev. Ecol. Syst. 33, 125–159. doi:10.1146/annurev.ecolsys.33.010802.150452
Wu, G.-L., Liu, Z.-H., Zhang, L., Hu, T.-M., and Chen, J.-M. (2010). Effects of Artificial Grassland Establishment on Soil Nutrients and Carbon Properties in a Black-soil-type Degraded Grassland. Plant Soil 333, 469–479. doi:10.1007/s11104-010-0363-9
Zhang, C., Willis, C. G., Burghardt, L. T., Qi, W., Liu, K., Moura Souza‐Filho, P. R., et al. (2014). The Community‐level Effect of Light on Germination Timing in Relation to Seed Mass: a Source of Regeneration Niche Differentiation. New Phytol. 204, 496–506. doi:10.1111/nph.12955
Zhang, C., Willis, C. G., Klein, J. A., Ma, Z., Li, J., Zhou, H., et al. (2017). Recovery of Plant Species Diversity during Long-Term Experimental Warming of a Species-Rich Alpine Meadow Community on the Qinghai-Tibet Plateau. Biol. Conserv. 213, 218–224. doi:10.1016/j.biocon.2017.07.019
Zhang, C., Ma, Z., Zhou, H., and Zhao, X. (2019). Long-term Warming Results in Species-specific Shifts in Seed Mass in Alpine Communitiesfic Shifts in Seed Mass in Alpine Communities. PeerJ 7, e7416. doi:10.7717/peerj.7416
Zhang, C., Zhou, H., Du, G., and Ma, Z. (2022). Light Plasticity of Germination on the Eastern Tibetan Plateau: Phylogeny, Trait, and Environmental Correlates. J. Plant Physiology 272, 153670. doi:10.1016/j.jplph.2022.153670
Zhang, H. (2018). Response of Rhizome Expansibility and Accumulation of Nitrogen and Phosphorus of Two Ecotype Kentucky Bluegrass to Water and Nitrogen and Phosphorus Fertilization. Doctoral Thesis. Lanzhou, Gansu Agricultural University.
Zhang, Z., and Liu, T. (2004). Study on Types of Artificial Grassland in China. Grassl. China 26, 32–36. (in Chinese with English abstract). doi:10.3969/j.issn.1673-5021.2004.05.006
Keywords: artificial grassland declines, clonal propagation, plant height, reproductive allocation, seed mass
Citation: Li C, Ren Y, Wu L, Wang X, Liang D, Zhou H, Ma Z and Zhang C (2022) Effects of Population Age on the Annual Net Primary Productivity and Seed Yield of Artificial Grasslands on the Eastern Qinghai-Tibetan Plateau. Front. Environ. Sci. 10:907404. doi: 10.3389/fenvs.2022.907404
Received: 29 March 2022; Accepted: 20 April 2022;
Published: 05 May 2022.
Edited by:
Jianjun Cao, Northwest Normal University, ChinaCopyright © 2022 Li, Ren, Wu, Wang, Liang, Zhou, Ma and Zhang. This is an open-access article distributed under the terms of the Creative Commons Attribution License (CC BY). The use, distribution or reproduction in other forums is permitted, provided the original author(s) and the copyright owner(s) are credited and that the original publication in this journal is cited, in accordance with accepted academic practice. No use, distribution or reproduction is permitted which does not comply with these terms.
*Correspondence: Chunhui Zhang, emhhbmdjcWh1QDE2My5jb20=; Zhen Ma, bWF6aGVuQG53aXBiLmNhcy5jbg==
†These authors have contributed equally to this work