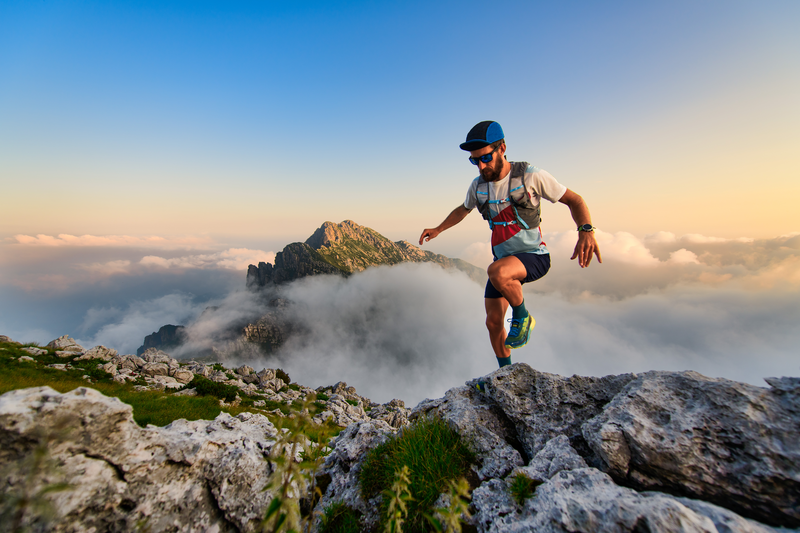
94% of researchers rate our articles as excellent or good
Learn more about the work of our research integrity team to safeguard the quality of each article we publish.
Find out more
ORIGINAL RESEARCH article
Front. Environ. Sci. , 05 July 2022
Sec. Soil Processes
Volume 10 - 2022 | https://doi.org/10.3389/fenvs.2022.905045
The influences and quantifications of soil crust traits on the infiltration, hydrodynamic of runoff, and erosion rate of sheet erosion under the combined effects of raindrop impact and sheet flow scouring need further study. Loessial soil from the Loess Plateau was tested to produce different antecedent crusts under simulated rainfall intensities (0.5, 1.0, 1.5, 2.0, and 2.5 mm/min, typical storm intensity in the area), and then the effects of antecedent crusts on sheet erosion processes were quantified at a rainfall intensity of 1.5 mm/min. The results showed that the bulk density and hardness of antecedent crusts were higher than those of soil. Particle sizes of crusts were smaller than those of soil at light rain intensity but larger under heavy rain intensity. The bulk density, hardness, and particle size D50 of the antecedent crust were all positively correlated with rainfall intensity, being well described by linear equations (R2 > 0.87), while the thickness was negatively linearly correlated with rainfall intensity (R2 = 0.88). Although the existence of antecedent crusts could decrease the infiltration and increase the runoff, resulting in the high flow velocity and stream power, antecedent crusts could still effectively reduce sheet erosion. The reductions in the average infiltration rate and average erosion rate and the increases of average flow velocity and stream power all increased with the increment of bulk density of antecedent crust. Relationships could be all well described by linear positive correlations (R2 > 0.79). When the bulk density of crust was enhanced by 27∼29%, the flow velocity and stream power could be increased by 8∼29% and 15∼70%, and the sheet erosion could be reduced by 61∼73%. The existence of crust could effectively reduce sheet erosion. These results could help understand the mechanism of the erosion process in the presence of physical crusts.
Soil physical crusts are a thin dense layer, with high strength, low porosity, and poor water conductivity, formed on the soil surface under the actions of rainfall and runoff (Miralles-Mellado et al., 2011; Hu et al., 2012; Barreto et al., 2019; Hardie and Almajmaie, 2019). The formation of soil crust can not only reduce soil infiltration rate but also has an important influence on the soil erosion process (Assouline, 2004; Pi et al., 2020). Soil crust is a common phenomenon in arid and semi-arid regions (Chen et al., 2013; Assouline et al., 2015; Chamizo et al., 2017). Approximately 35% of the lands in semi-arid areas are agricultural lands, the soils of which often have low contents of organic matter and aggregates (Chen and Cai, 2013), easily resulting in the formation of crust at the soil surface under intensive agriculture practices and the impact of raindrops (Cerdà, 2000; Cantón et al., 2009; Vaezi and Bahrami, 2014; Carr et al., 2015; Vaezi et al., 2017). The semi-arid climatic conditions and widely distributed loess materials in the Loess Plateau provide extremely favorable conditions for the generation of soil crusts. Meanwhile, sheet erosion is the initial and most important stage of slope erosion processes in the loess region. Research studies on the coupling relationship between soil crust characteristics and erosion on loess slopes can deeply reveal the mechanism of the slope erosion process, promote further development of the slope erosion theory, and provide an important scientific basis for soil and water loss control.
There are many factors affecting soil crust development, among which the characteristics of soil and rains play leading roles. Under the influence of rainfall splashing, physical crusts form in two ways: reorganization of soil particles induced by the continuous impact of raindrops (Bullard et al., 2018) and translocation and deposition of soil particles induced by raindrop impact and runoff (Avecilla et al., 2015). The prerequisite for crust formation is rainfall, especially the intensity. Bu et al. (2009) indicated that the main driving force of crust development for loessial soil was raindrop hitting and wetting dispersion. The greater the rainfall intensity, the stronger the impact and compaction of raindrops, and the greater the hardness and the thickness of the crusts (Liu and Jiang, 1988). Vaezi et al. (2017) concluded linear response relationships of both thickness and bulk density of crust to rainfall intensity. Wu and Fan, (2002) concluded that there was an exponential relationship between rainfall intensity and crust hardness. Liu and Jiang, (1988) figured out that crust hardness increased with rainfall kinetic energy. During the reorganization, translocation, and deposition of soil particles in the rainfall process, the particles on the soil surface are selected (Sadeghi et al., 2017, 2018; Kiani-Harchegani et al., 2019), which leads to different particle sizes of crust. The effects of rainfall intensity on the particle sizes of crust are the complex results of rain splash and runoff induced by rainfall. Chen et al. (2022a) indicated that the percentage of sand in crust decreased by 27% and those of silt and clay increased by 7 and 7%, respectively, compared with soil. Numerous research studies on the bulk density and hardness of crust are conducted, but studies about crust particle size related to rainfall intensity are rare. Moreover, quantified equations describing the crust traits and rainfall intensity need further study.
The crust is recognized by lower porosity, greater bulk density, and stronger soil strength (Lu et al., 2017). These features can lead to decreased infiltration rates and increased runoff (Souza et al., 2014). Jiang et al. (2018) demonstrated that the hydraulic conductivity of crusted soil was lower, supporting that physical crusts could reduce the infiltrating capacity (Wei et al., 2015). Bartling et al. (2017) assumed that surface crust had 1/5 of the hydraulic conductivity of non-crusted soil. Badorreck et al. (2013) evaluated the morphology of soil physical crusts and infiltration patterns and concluded that the crust could strongly affect the surface hydraulic properties and infiltration. Moore (1984) showed that infiltration of soil with surface crusting could be reduced by 70%. Chen et al. (2011) showed that there was a relatively significant dynamic response of runoff to crusts. Under the same rainfall condition, the crusts with higher strength can produce more runoff. Carmi et al. (2018) also suggested that raindrop energy had a major effect on the infiltration and runoff generation for naturally crusted loess soil, and the decrease in the infiltration rate was the result of the mechanical impact of raindrops on the soil surface (Souza et al., 2014). Chen et al. (2007) and Chen et al. (2013) found that crust-breaking could increase infiltration and reduce runoff yield. To date, no single soil water model can account for the effects of soil crust formation on soil hydrology (Nciizah et al., 2015; Hardie and Almajmaie, 2019). Flow velocity is a basic parameter to describe the dynamic of flow. Moreover, the hydrodynamic, including shear stress, stream power, and unit stream power, is a significant parameter to predict erosion. In general, the variations of flow velocity and hydrodynamic are consistent with those of runoff. To date, the quantifications of effects of crusts on runoff hydrodynamic are rare.
The formation of crusts also plays an important role in various types of erosion (splash erosion, rill erosion, and sheet erosion, etc.). On the one hand, the decrease of infiltration caused by crust can increase runoff and enhance the effect of runoff on erosion, thus increasing erosion (Kidron, 2007; Ries and Hirt, 2008; Bullard et al., 2018). On the other hand, the crusts formed on the surface have a dense layer, which can enhance the density and shear strength of the soil surface, thus reducing the impact of raindrops and runoff and erosion. Crusts may act as a physical barrier protecting the soil surface from the energy of raindrops and from runoff velocity, as pointed out by Descroix et al. (2001), Lane et al. (1997), and Maïga-Yaleu et al. (2013). For splash erosion, the effects of crusts were abundantly confirmed (Assouline, 2004; Bu et al., 2014; Pi et al., 2020). For rill erosion, studies have found that the existence of crusts may increase runoff kinetic energy, which can easily lead to rill generation, resulting in the increase of slope sediment yield by several or tens of times (Cai et al., 1998). Chen et al. (Forthcoming 2022b) notably indicated that crusts formed by rainfall at 30 min had the greatest rill detachment reduction benefit. For sheet erosion, Rajot et al. (2003) suggested that the development of a sieving crust for soils with < 5% clay may reduce erosion under a high rainfall intensity (40 ∼ 80 mm/h). Chen et al. (2011) verified that the development of crusts had both promoting and inhibiting effects on erosion through the rainfall experiment. Ma et al. (2022) showed that the crust of sloping farmland promoted runoff and inhibited slope sediment yield, and the total sediment yield was increased by 19.28 times after breaking the crusts. In laboratory simulation tests, the interaction between soil crust and splash erosion has been intensively discussed by many scholars, but the influence of soil crust on sheet erosion under the combined effects of the raindrop impact and sheet flow scouring needs further quantification.
The occurrence and development of sheet erosion and the development and evolution of crusts affect each other, and occur simultaneously, so it is difficult to observe both simultaneously. Based on the experiments of observing the characteristics of the crust before rainfall, while observing the characteristics of runoff and sediment after rainfall, the objectives of this study were to 1) explore the response of various traits of antecedent crust correlated to the rainfall intensity, 2) analyze the effects of different antecedent crusts on erosion, infiltration, and hydrodynamic processes of runoff, and 3) select the best trait to describe the crust and quantify the variations of the traits of crusts on the variations of erosion, infiltration, and hydrodynamic.
Experiments were conducted in Simulation Rainfall Hall of the State Key Laboratory of Soil Erosion and Dryland Farming on the Loess Plateau at the Institute of Soil and Water Conservation, the Chinese Academy of Science and Ministry of Water Resources, China. The tested soil was from Ansai County in the heartland of the Loess Plateau (a typically hilly and gully region). The area is a typical temperate semi-humid climate zone and a temperate semi-arid continental climate transition zone, with an annual precipitation of 505 mm and an annual mean temperature of 8.8°C. The soil was a silt loam (USDA) collected from 0 to 25 cm depth of the tillage layer, with particles < 0.001, 0.001–0.002, 0.002–0.01, 0.01–0.05, and 0.05–0.25 mm by 5.7, 2.8, 8.7, 53.6, and 29.2%, respectively.
Experimental plots were 1.2 m (length) × 0.4 m (width) × 0.25 m (depth), with adjustable gradients. A metal outlet at the lower end was set to collect runoff samples. The soil was packed to a depth of 20 cm in four 5-cm layers at a bulk density of 1.2 g/cm3 (same as that in the field cropland) and water content of 14% (a typical level during the flood season on the Loess Plateau when most erosion occurs). At the bottom, a depth of 5 cm natural sand was set to drain the infiltration water. Antecedent rainfalls were carried out to produce antecedent crusts at five rainfall intensities (0.5, 1.0, 1.5, 2.0, and 2.5 mm/min) and one slope gradient (5°). Blank control was carried out at a rainfall of 0.5 mm/min and a slope of 5°, the soil surface of which was covered by a 3-mm mesh screen. It could be approximately considered to produce no crust, as screens could eliminate raindrop kinetic energy and hitting effects on surface soil. All antecedent rainfalls stopped when the runoff occurred. The experiment design is shown in Figure 1.
About 20 h after the antecedent rainfalls, when the soil water content decreased to 20%, crusts were collected for measuring traits, including bulk density, thickness, and hardness. Samples were taken from the upper, middle, and lower slopes of the experimental plot by the customized cutting ring (5 cm inner diameter and 5 cm height). The underlying attached soil of these samples was carefully removed using a thin blade to obtain crusting. The bulk density of these samples was derived by the method of coating a thin film (Fan and Li, 2001), the thickness was determined using a digital caliper, and particle size D50 was measured using the EyeTech Particle Size and Shape Analyzer. The hardness of the surface soil crust was measured using a GY-3 hardness tester in the remained area of the same positions (upper, middle, and lower) on the slope, and then the average value was obtained.
About 20 h after the same antecedent rainfalls, the plots without measuring the traits of antecedent crusts were ready for the simulated rainfall experiments (1.5 mm/min, 15°). The duration was 45 min. Runoff samples were collected 1 and 3 min after the onset of runoff and then every 3 min until the end of the experiment. Simultaneously, the runoff velocity was measured by the dying method (KMnO4 solution) at two locations for each time interval. The runoff volumes were measured using a graduated cylinder and then left to sit. The clear supernatant was decanted, and the sediments were oven-dried at 105°C for 12 h and weighed. The runoff rate was defined as runoff depth per unit area per unit time, while the erosion rate was defined as sediment weight per unit area per unit time. The infiltration rate was defined as rainfall intensity minus runoff rate. Stream power (ω, W·m−2) can be calculated by:
where γ is bulk density of water (N·m−3), R is the hydraulic radius (m), J is the hydraulic gradient (J = sin θ, θ is the slope, º), and V is flow velocity (m·s−1).
The effects of antecedent crust on erosion were quantified by the relative percentage changes of four parameters (infiltration, erosion rate, flow velocity, and stream power) according to the comparison between antecedent-crusted soil and soil without the crust.
All data were analyzed using SPSS by one-way ANOVA and the least significant difference (LSD) tests. Statistical parameter R2 was used to evaluate the performance of new equations.
Table 1 shows the characteristics of crusts under various antecedent-rainfall intensities. The bulk densities of crusts were all significantly higher than those of soil, and (nonsignificantly) increased with rainfall intensity. The hardness of crusts was all significantly higher than that of soil and significantly increased with rainfall intensity. The thickness of crusts decreased with rainfall intensity, and the thickness of crusts caused by the heaviest and lightest rain intensity showed significant differences. The particle size D50 of crusts increased with rainfall intensity. Compared with soil, the particle sizes of crusts were smaller under light rain intensity but larger under heavy rain intensity Table 1.
The regression analysis was conducted on the variations of soil crust characteristics with antecedent rainfall intensity, and the relationships are shown in Table 2. The bulk density, hardness, and particle size D50 of the antecedent crusts were all positively correlated with antecedent rainfall intensity, being well described by linear equations (R2 > 0.87). However, the thickness was negatively correlated with rainfall intensity in the linear equation (R2 = 0.88), as shown in Table 2.
The studied soil was prone to form crusts due to the low aggregation (<1%). The crusts mainly resulted from the raindrop beating action and the deposition of entrained eroded soil particles suspended in runoff (Morin and Winkel, 1996), indicating that the rainfall intensity played an important role in the formation of crusts. Increasing rainfall intensity could increase the raindrop impact to induce greater soil compaction and sealing (Lu et al., 2016). Generally, the greater the rainfall intensity, the greater the kinetic energy of raindrops, the stronger the impact of raindrops on the soil surface, and the higher the characteristic values of the index such as bulk density and hardness of crust formation and development. The strength of crusts had been found to increase with cumulative rainfall (Freebairn et al., 1991; Fan et al., 2008; Feng et al., 2013; Nciizah and Wakindiki, 2014). Wu and Fan, (2002) concluded that there was an exponential relationship between rainfall intensity and crust hardness, which was different from the results in this study. Vaezi et al. (2017) also found a significantly positive relationship between crust thickness and rainfall intensity (T = 0.0044 I + 0.6008, R2 = 0.90) and between crust bulk density and rainfall intensity (B = 0.0054 I + 1.5072, R2 = 0.88), of which the equations were extremely similar with this study. For the thickness, the crust formed by antecedent rainfall was not fully developed, not getting enough runoff pressure and enough time to develop toward the depth. Therefore, it showed a negative correlation between the thickness and the antecedent rainfall intensity. However, seal thickness was strongly influenced by the rain amount impacting onto the soil surface, and the seal thickness and rate were well controlled by rainfall characteristics. Yan et al. (2015) indicated that the crust thickness linearly increased with increasing rainfall amounts. Armenise et al. (2018), Farres (1978) also observed that soil crust thickness increased with the cumulative rainfall. Feng et al. (2013) suggested a more suitable logarithmic relationship, which implied that crust development in response to rainfall was initially large and subsequently decreased with additional rainfall toward a given equilibrium.
Under the same slope (15°) and the same rainfall intensity (1.5 mm/min), the infiltration rates (IR) and erosion rates of soil with and without antecedent crusts are shown in Figure 2.
FIGURE 2. Effects of antecedent crust on infiltration and sheet erosion rates. Notes: A-R means antecedent rainfall intensity.
The infiltration rates all decreased rapidly and then tended to be gradually stable with durations. The infiltration rate of the soil without antecedent crusts was significantly higher than that of the soil with antecedent crusts. The turning point of the infiltration rate was about at 10th min, after which the infiltration rate tended to be gradually stable. The infiltration rates decreased with antecedent rainfall intensities. The higher the antecedent crust bulk density, the denser the topsoil and the smaller the pores, which could dramatically reduce infiltration, resulting in infiltration reduction. The results supported the notion that soil physical crusts could reduce the infiltrating capacity, which was proposed in previous studies (Bradford and Huang, 1993; Robinson and Philliphs, 2001; Kidron, 2007; Assouline et al., 2015; Jiang et al., 2018).
In the rainfall durations, the erosion rate of soil without crusts was much higher than that of the soil with antecedent crusts. The erosion rate of soil without crusts rapidly increased in the early 25 min and then tended to be stable. The erosion rates of soil with antecedent crusts gradually increased in early 25 min and then tended to be stable. Raindrop impact increased with rainfall intensity, inducing greater sealing and soil compaction, which contributed to less infiltration and more runoff and soil loss (Han et al., 2016; Vaezi et al., 2017). The greater the antecedent rainfall intensity, the lower the erosion rate. It verified that the antecedent crusts could effectively reduce erosion, and the erosion resistance of antecedent crusts was positively correlated with antecedent rainfall intensity. Yan et al. (2015) invalidated that the effects of crust on soil loss were closely related to the penetration resistance and confirmed that the crust could significantly increase the soil resistance to erosion, especially under rainfall intensity > 0.5 mm/h.
Flow velocity is the basic parameter to describe the dynamic of runoff. In addition, the hydrodynamic, including shear stress, stream power, and unit stream power, is a significant parameter to predict erosion. Either stream power or shear stress is usually recognized as the best predictor, so stream power was selected to describe the runoff hydrodynamic in this study. Under the same slope (15°) and the same rainfall intensity (1.5 mm/min), the flow velocity and stream power on the slopes of soil with and without antecedent crusts are shown in Figure 3.
FIGURE 3. Effect of antecedent crust on flow velocity and stream power. Notes: A-R means antecedent rainfall intensity.
The flow velocity all rapidly decreased in 10 or 15 min and then gradually tended to be stable with rainfall durations. The flow velocity on the soil surface without antecedent crusts was generally higher than that on the soil surface with antecedent crusts. The flow velocity under the heaviest rain intensity was always the maximum, and the velocity under the lightest rain intensity was always the minimum, but the velocity under three intermediate rainfall intensities changed irregularly. The traits of antecedent crusts were not constant, changing along with the rainfall process.
Raindrops can hit and damage crusts; however, crusts can also be developed by raindrop hit and runoff compaction (Chen et al., 1980; Onofiok and Singer, 1984). As a result, there is a destroyed—forming—destroyed evolution during the rainfall (Cai and Lu, 1996). This evolution leads to irregular changes. The effects of crusts on flow velocity were mainly reflected in the early period of rainfall. At the end of rainfall, the differences in flow velocities descended, showing that the influences of the antecedent crusts on flow velocity would gradually weaken and eliminate, which was mainly determined by the periodic development of crusts.
The stream power all rapidly increased in 10 or 15 min and then gradually tended to be stable with rainfall durations. The stream power for the soil without antecedent crusts was significantly lower than that for the soil with antecedent crusts. The stream power increased with antecedent rainfall intensity. The effects of crusts on stream power last for the entire rainfall process, not being confined to the early period of rainfall, which was different from the effects on flow velocity.
Compared with bare soil, the bulk density of crusts showed a significant rise, while the particle size D50 did not. There was no concept of thickness for soil without crusts. Soil crusts are the dense layer of the soil surface with high density and low porosity, which can improve the soil resistance to erosion and protect the underlying soil from being hit by raindrops and scoured out by runoff. Therefore, the bulk density of antecedent crusts was selected to study their effects on sheet erosion.
The relationships between increments of bulk density of the antecedent crusts and the corresponding reductions of infiltration, erosion rate, flow velocity, and stream power are shown in Figure 4. The reductions of the average infiltration rate and average erosion rate both increased with the increment of bulk density of antecedent crusts. Relationships could be well described by linear positive correlations (R2 > 0.79). There was a positive correlation between the average flow velocity and the bulk density of the antecedent crusts. The existence of antecedent crusts could decrease the infiltration and increase the runoff, resulting in the high flow velocity and stream power. When the bulk density of crusts was enhanced by 27–29%, the flow velocity and stream power could be increased by 8–29% and 15–70%, respectively, and sheet erosion could be reduced by 61–73%, as shown in Figure 4.
FIGURE 4. Relationships between percent changes of bulk density of antecedent crust and infiltration, erosion rates, flow velocity, and stream power.
Surface crust is an important factor affecting infiltration of water into soil profiles (Nciizah et al., 2015). The compaction of the soil surface to form a thin dense layer limits further entry of runoff (Bajracharya and Lal, 1999). In this study, the thickness became thinner with antecedent rainfall intensity due to the raindrop compaction, and the denser layer could prevent more infiltration and produce more runoff. As a result, bulk density, thickness, and hardness of crusts had influences on infiltration, flow velocity, and stream power (hydrodynamic of runoff). However, some authors have argued that the thickness had more influence than other properties like strength. McIntyre (1958) showed that a crust of only 0.1 mm thick may reduce the IR by more than 10 times. Wakindiki and Ben-Hur, (2002) observed that the infiltration of 0.1-mm-thick crust was almost two times more than that of 0.2-mm-thick crust.
Soil erosion is related to the erosion force (rainfall and runoff, etc.), topography (slope and slope length, etc.), and the surface soil characteristics and conditions. Erosion is a balance of the positive action of runoff and the negative action of soil resistance. In this experiment, the formation of the crust is an important factor in soil erosion. Crust formation directly changed the surface soil conditions, including low porosity, high bulk density, and great hardness and strength, which was consistent with the results indicated by Miralles-Mellado et al. (2011), Feng et al. (2013), Yan et al. (2015), and Vaezi et al. (2017). The high bulk density or hardness of crust strengthened the surface resistance to erosion, which could effectively reduce erosion (Assouline et al., 2015; Chamizo et al., 2017; Faist et al., 2017). However, crust formation weakened the infiltration and increased the runoff/flow velocity/stream power, which theoretically could exacerbate erosion. These are two completely contradictory effects in this study. In general theory, less infiltration means more runoff and more erosion. However, it showed that the larger the bulk density of the antecedent crust, the smaller the average infiltration rates and the erosion rates. Therefore, it could be reasonably speculated that the existence of crust could increase runoff, but the effect of a runoff increase was far less than that of a soil resistance increase on erosion. Conclusively, the existence of crusts could still reduce erosion, which indicated that the crusts could effectively enhance soil resistance and weaken erosion.
The properties of antecedent crusts induced by different antecedent rainfall intensities and the effects of antecedent crusts on sheet erosion processes were quantified on a loessial slope. Compared with soil without crusts, the bulk density and hardness of antecedent crusts were higher, and the particle sizes were smaller under light rain intensity but larger under heavy rain intensity. The bulk density, hardness, and particle size D50 of the antecedent crusts were all positively correlated with antecedent rainfall intensity, being well described by linear equations (R2 > 0.87). However, the thickness was negatively correlated with rainfall intensity in the linear equation (R2 = 0.88). In the rainfall durations, both the infiltration rate and erosion rate of soil without crusts were much higher than those of the soil with antecedent crusts. The flow velocity and stream power of the soil without crusts were generally higher than those of the soil with antecedent crusts. Although the existence of antecedent crusts could decrease infiltration and increase runoff, resulting in the high flow velocity and stream power, antecedent crusts could still effectively reduce erosion. The reductions in the average infiltration rate and average erosion rate and the increase in average flow velocity and stream power all increased with the increment of bulk density of antecedent crust. Relationships could be all well described by linear positive correlations (R2 > 0.79). When the bulk density of crusts was enhanced by 27∼29%, the flow velocity and stream power could be increased by 8∼29% and 15∼70%, respectively, and the sheet erosion could be reduced by 61∼73%. The existence of crusts could effectively reduce sheet erosion.
The raw data supporting the conclusion of this article will be made available by the authors, without undue reservation.
All authors listed have made a substantial, direct, and intellectual contribution to the work and approved it for publication.
Financial support for this research was provided by the National Natural Science Foundation of China-funded project (42077058, 41867015, 41601282, and 41830758), the Young Talent fund of University Association for Science and Technology in Shaanxi, China (20210705), the Fundamental Research Funds for Central Universities (GK202103150 and GK202001003), Shaanxi Provincial Key Research and Development Program (2021ZDLSF05-02), and Funds of Scientific Research Programs of State Key Laboratory of Soil Erosion and Dryland Farming on the Loess Plateau (A314021402-202109).
The authors declare that the research was conducted in the absence of any commercial or financial relationships that could be construed as a potential conflict of interest.
All claims expressed in this article are solely those of the authors and do not necessarily represent those of their affiliated organizations, or those of the publisher, the editors, and the reviewers. Any product that may be evaluated in this article, or claim that may be made by its manufacturer, is not guaranteed or endorsed by the publisher.
Armenise, E., Simmons, R. W., Ahn, S., Garbout, A., Doerr, S. H., Mooney, S. J., et al. (2018). Soil Seal Development under Simulated Rainfall: Structural, Physical and Hydrological Dynamics. J. Hydrology 556, 211–219. doi:10.1016/j.jhydrol.2017.10.073
Assouline, S. (2004). Rainfall-Induced Soil Surface Sealing: A Critical Review of Observations, Conceptual Models, and Solutions. Vadose Zone J. 3, 570–591. doi:10.2113/3.2.57010.2136/vzj2004.0570
Assouline, S., Thompson, S. E., Chen, L., Svoray, T., Sela, S., and Katul, G. G. (2015). The Dual Role of Soil Crusts in Desertification. J. Geophys. Res. Biogeosci. 120, 2108–2119. doi:10.1002/2015JG003185
Avecilla, F., Panebianco, J. E., and Buschiazzo, D. E. (2015). Variable Effects of Saltation and Soil Properties on Wind Erosion of Different Textured Soils. Aeolian Res. 18, 145–153. doi:10.1016/j.aeolia.2015.07.005
Badorreck, A., Gerke, H. H., and Hüttl, R. F. (2013). Morphology of Physical Soil Crusts and Infiltration Patterns in an Artificial Catchment. Soil Tillage Res. 129 (5), 1–8. doi:10.1016/j.still.2013.01.001
Bajracharya, R. M., and Lal, R. (1999). Land Use Effects on Soil Crusting and Hydraulic Response of Surface Crusts on a Tropical Alfisol. Hydrol. Process. 13 (1), 59–72. doi:10.1002/(sici)1099-1085(199901)13:1<59::aid-hyp675>3.0.co;2-1
Barreto, B. B., Siqueira, R. H. d. S., Rivera, F. P., Braga, R. A., Ferreira, M. M., and Horgan, G. (2019). Development of an Optical Technique for Characterizing Presence of Soil Surface Crusts. Comput. Electron. Agric. 167, 1050501–1050509. doi:10.1016/j.compag.2019.105050
Bartling, P. N. S., Ma, L., and Ahuja, L. R. (2017). RZWQM2 Agricultural Systems Model, User Guide. Fort Collins, Colorado, USA: USDA-ARS CARR Rangeland Resources and Systems Research Unit 2150 Centre Avenue Building D-200.
Bradford, J. M., and Huang, C. (1993). Comparison of Interrill Soil Loss for Laboratory and Field Procedures. Soil Technol. 6, 145–156. doi:10.1016/0933-3630(93)90003-w
Bu, C.-f., Wu, S.-f., and Yang, K.-b. (2014). Effects of Physical Soil Crusts on Infiltration and Splash Erosion in Three Typical Chinese Soils. Int. J. Sediment Res. 29 (4), 491–501. doi:10.1016/S1001-6279(14)60062-7
Bu, C. F., Cai, Q. G., Zhang, X. C., Chen, Q. J., and Wu, S. A. (2009). Mechanism and Erosion Effect of Development of Soil Crust of Loess. Acta Pet. Sin. 46 (01), 16–23. doi:10.3321/j.issn:0564-3929.2009.01.003
Bullard, J. E., Ockelford, A., Strong, C. L., and Aubault, H. (2018). Impact of Multi-Day Rainfall Events on Surface Roughness and Physical Crusting of Very Fine Soils. Geoderma 313, 181–192. doi:10.1016/j.geoderma.2017.10.038
Cai, Q. G., and Lu, Z. X. (1996). Experimental Study of Surface Crusts Process and Microfabric Analysis on Loess Soil. J. Basic Sci. Eng. 4 (4), 363–370.
Cai, Q. G., Wang, G. P., and Chen, Y. Z. (1998). Process and Simulation of Erosion and Sediment Yield in Loess Plateau. Beijing: Science Press.
Cantón, Y., Solé-Benet, A., Asensio, C., Chamizo, S., and Puigdefábregas, J. (2009). Aggregate Stability in Range Sandy Loam Soils Relationships with Runoff and Erosion. Catena 77, 192–199. doi:10.1016/j.catena.2008.12.011
Carmi, G., Abudi, I., and Berliner, P. (2018). An Experimental Study to Assess the Effect of the Energy and the Electrolyte Concentration of Rain Drops on the Infiltration Properties of Naturally Crusted Soils. J. Arid Environ. 152, 69–74. doi:10.1016/j.jaridenv.2018.01.009
Carr, P. M., Brevik, E. C., Horsley, R. D., and Martin, G. B. (2015). Long-Term No-Tillage Sequesters Soil Organic Carbon in Cool Semiarid Regions. Soil Horiz. 56 (6), 1–7. doi:10.2136/sh15-07-0016
Cerdà, A. (2000). Aggregate Stability against Water Forces under Different Climates on Agriculture Land and Scrubland in Southern Bolivia. Soil Tillage Res. 57 (3), 159–166. doi:10.1016/S0167-1987(00)00155-0
Chamizo, S., Rodríguez-Caballero, E., Román, J. R., and Cantón, Y. (2017). Effects of Biocrust on Soil Erosion and Organic Carbon Losses under Natural Rainfall. Catena 148, 117–125. doi:10.1016/j.catena.2016.06.017
Chen, L., Sela, S., Svoray, T., and Assouline, S. (2013). The Role of Soil-Surface Sealing, Microtopography, and Vegetation Patches in Rainfall-Runoff Processes in Semiarid Areas. Water Resour. Res. 49, 5585–5599. doi:10.1002/wrcr.20360
Chen, L., Wang, J., Wang, H., Xu, F., Xu, P., Yang, C., et al. (2022a). Variation in Soil Detachment Capacity of Structural and Sedimentary Crusts Induced by Simulated Rainfall Formed on Ridge and Furrow. Catena 211, 105971. doi:10.1016/j.catena.2021.105971
Chen, L., Yang, C., Zhang, Q., and Wang, J. (Forthcoming 2022b). Variations in the Disintegration Rate of Physical Crusts Induced by Artificial Rainfall in Different Alcohol Concentrations. Int. Soil Water Conservation Res.. doi:10.1016/j.iswcr.2022.03.006
Chen, Q. J., Cai, Q. G., and Liao, S. Y. (2007). Effects of Soil Surface Characteristics and Gradient on Runoff and Sediment Yield. J. Soil Water Conserv. 21 (2), 9–11. doi:10.13870/j.cnki.stbcxb.2007.02.003
Chen, Q. J., and Cai, Q. G. (2013). Response of Soil Surface Crusts on Soil Erosion in Loess under Simulated Rainfalls. J. Soil Water Conserv. 27 (4), 73–77. doi:10.13870/j.cnki.stbcxb.2013.04.029
Chen, Y., Tarchitzky, J., Brouwer, J., Morin, J., and Banin, A. (1980). Scanning Electron Microscope Observations on Soil Crusts and Their Formation. Soil Sci. 130, 49–55. doi:10.1097/00010694-198007000-00008
Chen, Z. F., Xia, Q., Shi, D. M., Jiang, G. Y. Y., and Guo, H. Z. (2011). Soil Surface Crust Characteristic and Response Feature to Slope Erosion Base on Simulated Rainfall. J. Soil Water Conserv. 25 (4), 6–11. doi:10.13870/j.cnki.stbcxb.2011.04.012
Descroix, L., Viramontes, D., Vauclin, M., Gonzalez Barrios, J. L., and Esteves, M. (2001). Influence of Soil Surface Features and Vegetation on Runoff and Erosion in the Western Sierra Madre (Durango, Northwest Mexico). Catena 43, 115–135. doi:10.1016/S0341-8162(00)00124-7
Faist, A. M., Herrick, J. E., Belnap, J., Van Zee, J. W., and Barger, N. N. (2017). Biological Soil Crust and Disturbance Controls on Surface Hydrology in a Semi-arid Ecosystem. Ecosphere 8 (3), e01691. doi:10.1002/ecs2.1691
Fan, W. B., and Li, X. J. (2001). Determining Unit Weight of Loess Crust with the Method of Coating a Thin Film. Soil water conservation Sci. Technol. Shanxi 3, 9–10. doi:10.3969/j.issn.1008-0120.2001.03.004
Fan, Y., Lei, T., Shainberg, I., and Cai, Q. (2008). Wetting Rate and Rain Depth Effects on Crust Strength and Micromorphology. Soil Sci. Soc. Am. J. 72, 1604–1610. doi:10.2136/sssaj2007.0334
Farres, P. (1978). Role of Time and Aggregate Size in the Crusting Process. Earth Surf. Proc. 3 (3), 243–254. doi:10.1002/esp.3290030304
Feng, G., Sharratt, B., and Vaddella, V. (2013). Windblown Soil Crust Formation under Light Rainfall in a Semiarid Region. Soil Tillage Res. 128, 91–96. doi:10.1016/j.still.2012.11.004
Freebairn, D. M., Gupta, S. C., and Rawls, W. J. (1991). Influence of Aggregate Size and Microrelief on Development of Surface Soil Crusts. Soil Sci. Soc. Am. J. 55, 188–195. doi:10.2136/sssaj1991.03615995005500010033x
Hardie, M., and Almajmaie, A. (2019). Measuring and Estimating the Hydrological Properties of a Soil Crust. J. Hydrology 574, 12–22. doi:10.1016/j.jhydrol.2019.04.031
Han, Y. G., Fan, Y. T., Xin, Z. B., Wang, L., Cai, Q. G., and Wang, X. Y. (2016). Effects of Wetting Rate and Simulated Rain Duration on Soil Crust Formation of Red Loam. Environ. Earth Sci. 75, 149. doi:10.1007/s12665-015-4901-x
Hu, X., Liu, L.-Y., Li, S.-J., Cai, Q.-G., Lü, Y.-L., and Guo, J.-R. (2012). Development of Soil Crusts under Simulated Rainfall and Crust Formation on a Loess Soil as Influenced by Polyacrylamide. Pedosphere 22, 415–424. doi:10.1016/s1002-0160(12)60027-7
Jiang, Z.-Y., Li, X.-Y., Wei, J.-Q., Chen, H.-Y., Li, Z.-C., Liu, L., et al. (2018). Contrasting Surface Soil Hydrology Regulated by Biological and Physical Soil Crusts for Patchy Grass in the High-Altitude Alpine Steppe Ecosystem. Geoderma 326, 201–209. doi:10.1016/j.geoderma.2018.04.009
Kiani-Harchegani, M., Sadeghi, S. H., Singh, V. P., Asadi, H., and Abedi, M. (2019). Effect of Rainfall Intensity and Slope on Sediment Particle Size Distribution during Erosion Using Partial Eta Squared. Catena 176, 65–72. doi:10.1016/j.catena.2019.01.006
Kidron, G. J. (2007). Millimeter-scale Microrelief Affecting Runoff Yield over Microbiotic Crust in the Negev Desert. Catena 70, 266–273. doi:10.1016/j.catena.2006.08.010
Lane, L. J., Hernandez, M., and Nichols, M. (1997). Processes Controlling Sediment Yield from Watersheds as Functions of Spatial Scale. Environ. Model. Softw. 12, 355–369. doi:10.1016/s1364-8152(97)00027-3
Liu, Z., and Jiang, Z. S. (1988). Study on the Effect of Raindrop on Loess Crust. Bull. Soil Water Conserv. 8 (1), 62–64.
Lu, J., Zheng, F., Li, G., Bian, F., and An, J. (2016). The Effects of Raindrop Impact and Runoff Detachment on Hillslope Soil Erosion and Soil Aggregate Loss in the Mollisol Region of Northeast China. Soil Tillage Res. 161, 79–85. doi:10.1016/j.still.2016.04.002
Lu, P., Xie, X., Wang, L., and Wu, F. (2017). Effects of Different Spatial Distributions of Physical Soil Crusts on Runoff and Erosion on the Loess Plateau in China. Earth Surf. Process. Landforms 42 (13), 2082–2089. doi:10.1002/esp.4175
Ma, J. L., Xu, H., Wang, B., Zhang, B. Q., and Dan, C. H. (2022). Effect of Soil Physical Crust on Runoff and Sediment Yield on Sloping Farmland of the Loess Plateau. J. Soil Water Cons. 36 (1), 45–49. doi:10.13870/j.cnki.stbcxb.2022.01.007
Maïga-Yaleu, S., Guiguemde, I., Yacouba, H., Karambiri, H., Ribolzi, O., Bary, A., et al. (2013). Soil Crusting Impact on Soil Organic Carbon Losses by Water Erosion. Catena 107, 26–34. doi:10.1016/j.catena.2013.03.006
McIntyre, D. S. (1958). Permeability Measurements of Soil Crusts Formed by Raindrop Impact. Soil Sci. 85, 185–189. doi:10.1097/00010694-195804000-00002
Miralles-Mellado, I., Cantón, Y., and Solé-Benet, A. (2011). Two-dimensional Porosity of Crusted Silty Soils: Indicators of Soil Quality in Semiarid Rangelands? Soil Sci. Soc. Am. J. 75 (4), 1330–1342. doi:10.2136/sssaj2010.0283
Moore, D. C. (1984). Effect of Surface Sealing on Infiltration. Trans. ASAE. 24, 1546–1552. doi:10.13031/2013.34488
Morin, J., and Winkel, J. (1996). The Effect of Raindrop Impact and Sheet Erosion on Infiltration Rate and Crust Formation. Soil Sci. Soc. Am. J. 60, 1223–1227. doi:10.2136/sssaj1996.03615995006000040038x
Nciizah, A. D., Wakindiki, I. I. C., and Wakindiki, I. C. (2015). Soil Sealing and Crusting Effects on Infiltration Rate: a Critical Review of Shortfalls in Prediction Models and Solutions. Archives Agron. Soil Sci. 61 (9), 1211–1230. doi:10.1080/03650340.2014.998203
Nciizah, A., and Wakindiki, I. (2014). Rainfall Pattern Effects on Crusting, Infiltration and Erodibility in Some South African Soils with Various Texture and Mineralogy. Wsa 40, 57–64. doi:10.4314/wsa.v40i1.7
Onofiok, O., and Singer, M. J. (1984). Scanning Electron Microscope Studies of Surface Crusts Formed by Simulated Rainfall. Soil Sci. Soc. Am. J. 48, 1137–1143. doi:10.2136/sssaj1984.03615995004800050037x
Pi, H., Huggins, D. R., and Sharratt, B. (2020). Influence of Clay Amendment on Soil Physical Properties and Threshold Friction Velocity within a Disturbed Crust Cover in the Inland Pacific Northwest. Soil Tillage Res. 202, 104659. doi:10.1016/j.still.2020.104659
Rajot, J. L., Alfaro, S. C., Gomes, L., and Gaudichet, A. (2003). Soil Crusting on Sandy Soils and its Influence on Wind Erosion. Catena 53, 1–16. doi:10.1016/S0341-8162(02)00201-1
Ries, J. B., and Hirt, U. (2008). Permanence of Soil Surface Crusts on Abandoned Farmland in the Central Ebro Basin/Spain. Catena 72, 282–296. doi:10.1016/j.catena.2007.06.001
Robinson, D. A., and Phillips, C. P. (2001). Crust Development in Relation to Vegetation and Agricultural Practice on Erosion Susceptible, Dispersive Clay Soils from Central and Southern Italy. Soil Tillage Res. 60, 1–9. doi:10.1016/s0167-1987(01)00166-0
Sadeghi, S. H., Kiani Harchegani, M., and Asadi, H. (2017). Variability of Particle Size Distributions of Upward/Downward Splashed Materials in Different Rainfall Intensities and Slopes. Geoderma 290, 100–106. doi:10.1016/j.geoderma.2016.12.007
Sadeghi, S. H., Singh, V. P., Kiani-Harchegani, M., and Asadi, H. (2018). Analysis of Sediment Rating Loops and Particle Size Distributions to Characterize Sediment Source at Mid-sized Plot Scale. Catena 167, 221–227. doi:10.1016/j.catena.2018.05.0010.1016/j.catena.2018.05.002
Souza, E. S., Antonino, A. C. D., Heck, R. J., Montenegro, S. M. G. L., Lima, J. R. S., Sampaio, E. V. S. B., et al. (2014). Effect of Crusting on the Physical and Hydraulic Properties of a Soil Cropped with Castor Beans (Ricinus communis L.) in the Northeastern Region of Brazil. Soil Tillage Res. 141, 55–61. doi:10.1016/j.still.2014.04.004
Vaezi, A. R., Ahmadi, M., and Cerdà, A. (2017). Contribution of Raindrop Impact to the Change of Soil Physical Properties and Water Erosion under Semi-arid Rainfalls. Sci. Total Environ. 583, 382–392. doi:10.1016/j.scitotenv.2017.01.078
Vaezi, A. R., and Bahrami, H. A. (2014). Relationship between Soil Productivity and Erodibility in Rainfed Wheat Lands in Northwestern Iran. J. Agric. Sci. Technol. 16, 1455–1466. http://jast.modares.ac.ir/article-23-4666-en.html.
Wakindiki, I. I. C., and Ben-Hur, M. (2002). Soil Mineralogy and Texture Effects on Crust Micromorphology, Infiltration, and Erosion. Soil Sci. Soc. Am. J. 66, 897–905. doi:10.2136/sssaj2002.8970
Wei, W., Yu, Y., and Chen, L. (2015). Response of Surface Soil Hydrology to the Micro-pattern of Bio-Crust in a Dry-Land Loess Environment, China. PLoS One 10, e0133565. doi:10.1371/journal.pone.0133565
Wu, F. Q., and Fan, W. B. (2002). Analysis on Factors Affecting Soil Crust Formation on Slope Farmland. J. Soil Water Conserv. 16 (1), 33–36. doi:10.13870/j.cnki.stbcxb.2002.01.008
Keywords: physical crust, sheet erosion, hydrodynamics, runoff, loessial soil
Citation: Liu J, Qi X, Ma C, Wang Z and Li H (2022) Response of Sheet Erosion to the Characteristics of Physical Soil Crusts for Loessial Soils. Front. Environ. Sci. 10:905045. doi: 10.3389/fenvs.2022.905045
Received: 26 March 2022; Accepted: 24 May 2022;
Published: 05 July 2022.
Edited by:
Ilan Stavi, the Dead Sea and Arava Science Center, IsraelReviewed by:
Ning Chen, Lanzhou University, ChinaCopyright © 2022 Liu, Qi, Ma, Wang and Li. This is an open-access article distributed under the terms of the Creative Commons Attribution License (CC BY). The use, distribution or reproduction in other forums is permitted, provided the original author(s) and the copyright owner(s) are credited and that the original publication in this journal is cited, in accordance with accepted academic practice. No use, distribution or reproduction is permitted which does not comply with these terms.
*Correspondence: June Liu, bGl1anVuZTVAc25udS5lZHUuY24=
Disclaimer: All claims expressed in this article are solely those of the authors and do not necessarily represent those of their affiliated organizations, or those of the publisher, the editors and the reviewers. Any product that may be evaluated in this article or claim that may be made by its manufacturer is not guaranteed or endorsed by the publisher.
Research integrity at Frontiers
Learn more about the work of our research integrity team to safeguard the quality of each article we publish.