- 1Programa de Pós-Graduação Em Ecologia e Recursos Naturais, Departamento de Biologia, Centro de Ciências, Universidade Federal Do Ceará, Fortaleza, Ceará, Brazil
- 2Programa de Pós-Graduação Em Ecologia e Evolução, Departamento de Ecologia, Instituto de Biologia Roberto Alcantara Gomes, Universidade Do Estado Do Rio de Janeiro, Rio de Janeiro, Brazil
- 3Programa de Pós-Graduação Em Ecologia, Conservação e Manejo da Vida Silvestre, Universidade Federal de Minas Gerais, Belo Horizonte, Minas Gerais, Brazil
- 4Programa de Pós-Graduação Em Ecologia, Instituto Nacional de Pesquisas da Amazônia, Manaus, Brazil
We are living in a fast-changing world promoted by anthropogenic actions and ecosystems’ functioning has been constantly changed. One example is the globally reduction of river flow that can lead to more than 50% of the rivers and streams around the world becoming intermittent. However, the relationship between environmental changes and biological communities has been limited to taxonomic approach, rather than the functional approach. Functional structure determines how individuals interact with the environment and evaluating the effects of hydrological changes in functional variation can elucidate the responses of aquatic biota under climate changes. Thus, we hypothesized that hydrological phases (dry, re-wetting and wet) would influence both environmental conditions and fish composition (i.e., taxonomic richness and functional structure) in one intermittent river. In addition, we expected that: 1) fish assemblages will have higher values of species richness and Functional Diversity indexes—Richness (FRic), Dispersion (FDis), Specialization (FSpe), and Originality (FOri) in drier phases (dry and re-wetting); and 2) higher values of Functional Diversity indexes related to evenness (FEve) and divergence (FDiv) in the wet phase, due to the predominance of species with high dispersal capacity when sites are connected. Sampling was conducted in the Cruxati river during the three hydrological phases along four sites (250 m each). Functional diversity analysis, involving traits of dispersion, life history and trophic ecology were used to apply Functional Diversity indexes. Indexes were compared from null models and all species were ordered in a multidimensional functional space using principal coordinate analysis (PCoA). In the dry phase, taxonomic richness and FRic between communities is higher, as well as FSpe and FOri, showing that each assemblage has unique characteristics with different strategies allowing the establishment in this hydrological phase. However, communities are less functionally dispersed. In the wet phase, when the river is connected, FDis between communities is higher and species abundances are more equitable with regular distribution in the functional space. Therefore, we conclude that local ecological processes (i.e., dynamics of hydrology) may promote the establishment of species according to their functional traits and thereby the functional structure of local assemblages.
Introduction
Predicting the consequences of climate and hydrological changes on biodiversity represents a primordial aim of modern community ecology since global climate changes are bringing unprecedented and extreme scenarios to the Neotropics (Pereira et al., 2010). However, our ability to accurately predict future changes has been limited by focusing on the taxonomic approach, such as species richness, rather than the functional approach, such as the life-history or habitat exploration traits of species (Jarzyna and Jetz, 2016). The functional structure of a community is defined as the distribution of species and their abundances in the functional space (Mouillot et al., 2013), considered as one better predictor of ecosystem functioning when compared with species diversity (Mouillot et al., 2011; Schmera et al., 2017). Because it is based on species traits, the functional structure determines how individuals interact with the environment (Violle et al., 2014) where groups of individuals with certain combinations of physiological–ecological adaptations are selected by the dynamics of local and regional environments (Dézerald et al., 2015). Thus, considering the functional approach in temporal studies can provide a more mechanistic relationship between the consequences of climate and hydrological changes on biodiversity patterns.
Functional diversity analyses should be approached as a tool for understanding the functioning and structure of biological communities, including stream fish (Manna et al., 2019). Quantifying the functional structure of communities involves the distribution of points (species) and their weights (abundances) in one multidimensional Euclidean space (Mouillot et al., 2013). From species distribution values, it is possible to obtain indices that will indicate different facets of functional diversity (Functional Richness—FRic, Functional Evenness—FEve, Functional Divergence - FDiv, Functional Dispersion—FDis, Functional Originality—FOri and Functional Specialization—FSpe) (Teresa et al., 2021). As functional diversity involves several complementary components, it is not possible to summarize the patterns in a single number and a multifaceted approach is needed (Villéger et al., 2008; Mouillot et al., 2013).
In aquatic systems, species distribution is strongly influenced by physical variables that characterize different types of habitats and hydrological regime that determine which species are prone to local coexist based on their functional traits (Manna et al., 2017; Manna et al., 2019; Rodrigues-Filho et al., 2020), especially for aquatic organisms such as fish that have an exclusively aquatic life cycle. The variability in local habitat conditions is striking drastic in intermittent rivers, and ephemeral streams (IRES), where streamflow ceases partially or completely at some point along their course (Datry et al., 2017). When flow ceases most of the reaches of the river can be completely dry or the remaining water can form isolated pools where fishes and other taxa can be found (Bonada et al., 2020). In isolated pools water temperatures are higher, solute concentrations are incremented, and pH and dissolved oxygen levels decrease (Boulton, 2003). These conditions are considered important environmental filters for fish species, selecting those having traits combinations with fine-tuned adjustment to explore the local harsh conditions. Specifically, small stream-lined fish species with high fecundity and short reproductivity cycles can grow fast during dry phases under favorable temporary conditions reaching larger population density and body size by the end of floods with greater chances of survival and dispersal under drought environmental conditions (Junk et al., 1989; Pease et al., 2012). Indeed, fish species tend to present specialized strategies to survive in dry phases, such as high trophic variability (characin species; Manna et al., 2019) and high fertility (killifishes; Rodrigues-Filho et al., 2020). On the other hand, in wet phases the local sites become connected and thereby allow species dispersal among them (Medeiros and Maltchik, 2001). As so, species with high dispersal capacity (greater size and absence of parental care; Comte and Olden, 2018) tend to become widely distributed among local communities, which in turn would increase the functional evenness. Thus, morphology and life-history traits strategies could therefore be expected to reflect adaptations to highly variable and unpredictable environments (Espírito-Santo et al., 2013; Borba et al., 2020).
Studies considering functional diversity on different spatial scales are increasingly known, nonetheless studies addressing temporal variation are still rare (Teresa et al., 2021; Vorste et al., 2021). In this context, we aim to investigate taxonomic and functional diversity responses of fish assemblage to temporal dynamics of IRES Cruxati River in Brazilian semiarid, over three years (2016–2018). For this purpose, we performed the hypothesis that hydrological phases (dry, re-wetting and wet) would influence both environmental conditions and fish composition (i.e., taxonomic richness and functional structure) of the IRES Cruxati River. We operationalize this hypothesis by testing the following questions: 1) Do the local environmental conditions of the IRES Cruxati River change over hydrological phases? 2) Do differences on taxonomic and functional structures change over hydrological phases? We expected to observe lower habitat availability and diversity in drier phases (dry and re-wetting) than in the wet phase. In addition, by assuming that fish fauna of naturally intermittent systems has different adaptations to hydrological fluctuations and local habitat variability (Rodrigues-Filho et al., 2020), we expected that different functionally assemblages will have higher values of species richness, FRic, FDis, FSpe, and FOri in drier phases (dry and re-wetting) and higher values of FEve and FDiv in the wet phase, due to the predominance of species with high dispersal capacity when sites are connected.
Materials and methods
Study area
Cruxati River (−3.406150° S −39.740449° W) is one of the main tributaries of the Mundaú River (about 20 m wide), it is one of the principal rivers of the litoral hydrographic basin; a coastal naturally intermittent basin that flows into the Atlantic Ocean. It forms a dendritic network divided by 475 tributaries, draining 1,264.22 km2 (Figure 1) (Duarte et al., 2021). Considering the distance from artificial barriers (nearest dam is ∼16 km distant from sampling sites) and capacity of dispersion by stream-dwelling fish, species movement should not be affected. From the 15 studied species, only two have migratory habits (Steindachnerina notonota and Prochilodus brevis), the others have potential to short movements (20-50 m), or displacement limited to ∼2 km (e.g., Mazzoni et al., 2018).
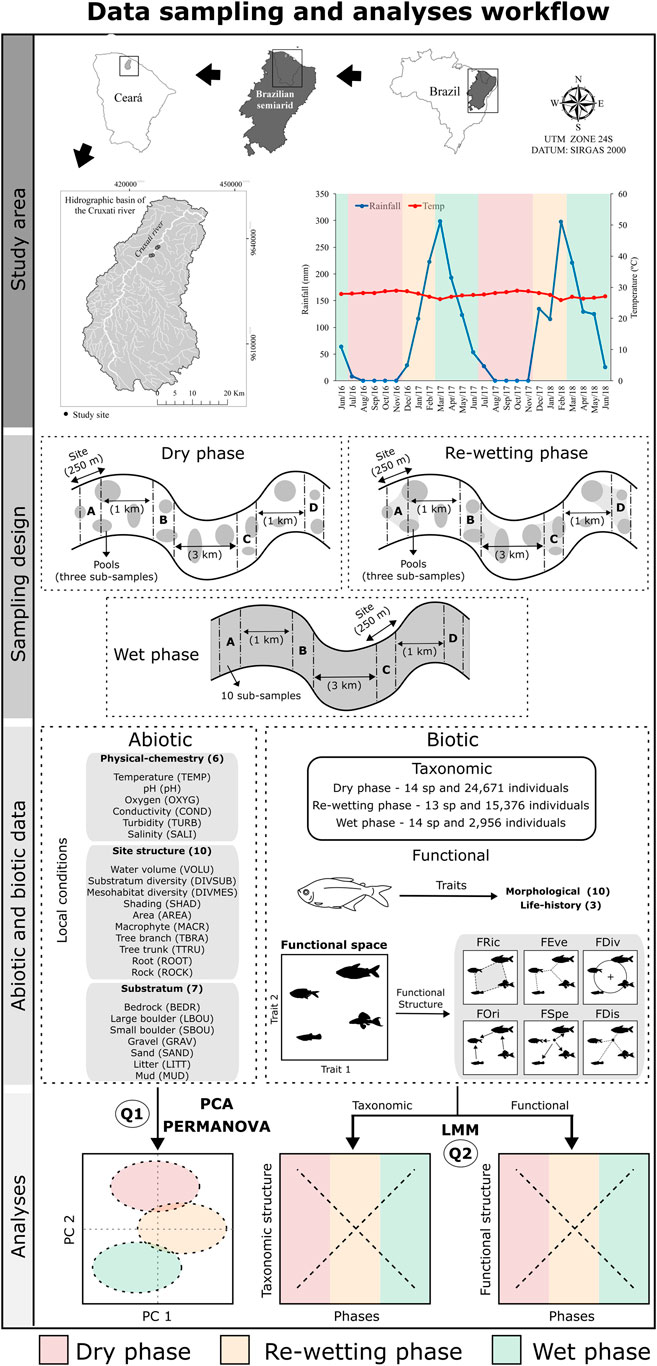
FIGURE 1. Methodological workflow to investigate the influence of hydrological phases (dry, re-wetting and wet) on environmental conditions and fish composition (taxonomic and functional structure) of the IRES Cruxati River, Ceará, Brazil. Representations of functional indexes in biotic data are adapted from Mouillot et al. (2013).
The catchment is located in a tropical semiarid climate zone where the annual precipitation in headwaters is about 1,102.5 mm and the mean annual temperature is 21.02°C, and downstream average monthly temperatures are 35.09°C in the driest and hottest period. The river flows four months a year (from March to June), when the flow ceases, the river breaks up into isolated pools of different sizes distributed along the dry bed. Some of these pools (approximately 40%) remain with water during the rest of the year while others dry completely, due to semiarid characteristics of soil and vegetation which increases the loss of water by evaporation. After rainy events the pools reconnect in the following year. From headwater up to downstream there are three vegetation formations (sensu Holdridge, 1947): 1) Most Forest in headwater from windward located at the highest altitudes (>700 m asl); 2) Dry Forest between 500 and 700 m asl; and 3) Very Dry Forest/Woodland at lower altitudes.
Sampling design and data sampling
Brazilian semiarid IRES are characterized by the extremes of flooding and total absence of water flow; this dynamic contributes to the high degree of spatial and temporal habitat heterogeneity (Maltchik and Medeiros, 2006). Due to the intermittent dynamics (Figure 1), we divided hydrological cycle into three phases: 1) dry (July-November), the flow of water ceases and river bed is fragmented into pools characterizing the longest phase of the hydrological cycle, it can last up to six months; 2) re-wetting (December-February), the remaining pools starts to fill again in the beginning of the rainy season; and 3) wet (March-June), river flows after torrential rains. The durations of the hydrological phases are not equitable, for this purpose we sampled twice as many times during the dry phase, because it is the longest phase of the hydrological cycle. In total, we sampled seventeen times between June 2016 and June 2018 (four samples during wet phase, eight samples during dry and five samples during re-wetting).
Samplings were performed in the middle reach of Cruxati River in four sampling sites (A-D, Figure 1) with an extension of 250 m each. In each sampling site were performed three sub-samples per pool during the dry and re-wetting phase and ten sub-samples, 25 m equidistant, during the wet phase (in the flowing river) (Figure 1), totaling 182 sub-samples or assemblages including all three hydrological phases. For each sub-sample, we obtained physical-chemicals (temperature, dissolved oxygen, conductivity, pH, turbidity, and salinity) and environmental (site structure and substratum composition) data (Taylor and Lienesch, 1995; Supplementary Table S1). These data were obtained three times to posteriorly use an average for each variable. We collected physical data with YSI Professional Plus Multiparameter Meter 7,000 and with Digimed AP2000 portable turbidimeter. In the dry phase, the volume of each pool was obtained through the multiplication of length, average width, and depth (Hauer and Lamberti, 2011).
Fish were sampled using seine nets (3.5 × 2.5 m, mesh size 5 mm), castnets (2 m height, mesh size 15 mm) and dip net (mesh size 5 mm). In each sub-sample, the nets were passed twice, and at inaccessible places for the seine net it was used castnet and dip net. After capture, fish were kept alive in a box covered in a 3 mm screen and immersed in water. Then, fishes were identified, counted, and returned to the water. Only 10 individuals of each species were euthanized in a solution of Eugenol (diluted in 92.8° alcohol in a proportion of 1:10) at 300 mg/L (Vidal et al., 2008) and fixed in 10% formalin. In the laboratory, the fish were preserved in 70% alcohol for further measurements of morphological functional traits (see below).
Functional structure
To assess the functional structure of fish assemblages, we selected 10 individuals of each species for all hydrological phases of the river and measured 13 functional traits related to food acquisition, dispersal, life history and trophic ecology (Supplementary Table S2). Morphological traits were obtained from ecomorphological measurements recorded using ImageJ software (https://imagej.nih.gov/ij/index.html), calculated according to Villéger et al. (2010). These measurements were then combined into 10 ecomorphological traits that were posteriorly centered to zero mean and unit standard deviation. Traits of life history (reproductive tactics and hypoxia tolerance) were obtained from the literature (Winemiller, 1991; Winemiller and Rose, 1992) and data from Fishbase (http://www.fishbase.org). Trophic traits were obtained through stomach analysis and quantification of food items by the volumetric method (Hyslop, 1980).
To construct the multidimensional space, we performed a principal coordinate analysis (PCoA) to reduce dimensionality and plot the species according to their traits (Villéger et al., 2008). Based on the protocol developed by Maire et al. (2015) to determine the number of axes that guarantee a high-quality functional space, we used the first four PCoA axes, using the function “quality_funct_space” available on Villéger’s website (http://villeger.sebastien. free. fr/Rscripts.html).
We computed six complementary indices to describe the functional structure of each fish assemblage: Functional Richness (FRic); Functional Evenness (FEve); Functional Divergence (FDiv); Functional Dispersion (FDis); Functional Specialization (FSpe); and Functional Originality (FOri), from the R function “multidimFD” (Mouillot et al., 2013). These indices were calculated using the number of sampled individuals (species abundance) for each hydrological phase and functional traits obtained from 10 individuals per species. The FRic represents the volume of the functional space occupied by each fish assemblage and is strongly related to local species richness (Villéger et al., 2008). On the other hand, the FEve and FDiv are abundance-weighted functional indices, representing the regularity of abundance distribution in the functional space and how species abundances diverge from the center of the functional space, respectively (Villéger et al., 2008). The FDis is the mean distance in multidimensional trait space of individual species to the centroid of all species in each community. This index is sensitive to species abundances by shifting the position of the centroid toward the more abundant species and weighting distances of individual species by their relative abundances (Laliberté and Legendre, 2010). The FSpe measures the functional specialization of the species pool, from the Euclidean distance of each species to the center of functional space (Villéger et al., 2010). Furthermore, FOri is expressed as the isolation of a species in the functional space occupied by a given community, since species are less original when showing combinations of characteristics similar to others (Mouillot et al., 2013). To scale up these species-level information of the FSpe and FOri to the community level, we calculated the mean value of these indices for each fish assemblage.
Data analysis
Differences on local environmental conditions between hydrological phases were tested using a Permutational Multivariate Analysis of Variance (PERMANOVA), using Euclidean distance. To illustrate the environmental differences, we carried out a Principal Component Analysis (PCA), using a correlation matrix.
To evaluate functional structure regionally, we began by examining the distribution of species through functional space for the three hydrological phases (dry, re-wetting, wet). We then plotted convex hulls of each hydrological phase within the functional space to examine whether the regional functional structure changed over phases. In addition, to examine which traits were most responsible for explaining the regional functional structure, we plotted the traits loadings (correlations of traits with PCoA axes) into functional space.
Locally, we assessed the importance of hydrological phases (dry, re-wetting and wet) on the functional structure of the fish assemblages using a linear mixed model (LMM). We first concatenated sub-samples into a single matrix with seven columns (species richness and functional indexes; response variables). The site identity (A-D, see Figure 1) and sampled months were treated as random factors to account for autocorrelation within sites and months, respectively (Zuur et al., 2009). We then built a global model testing for the effect of hydrological phases (dry, re-wetting, and wet) on species richness and functional structure.
We calculated Moran’s I values using the residuals of the global LMM models to evaluate if significant relationships are affected by spatial autocorrelation. In the case of significant effects of spatial correlation, we incorporated the argument “corAR1” to global models (Zuur et al., 2009). After that, the global models were compared against a null model assuming that the hydrological phases do not influence the response variables. For significant results (p < 0.05), we assume that the hydrological phases were essential to explaining the temporal variation in taxonomic and/or functional structure. Finally, when hydrological phases significantly influenced the variation of assemblages, we simplified the global model to test which hydrological phases were different from each other. Therefore, the simplified models contain the response variables that were significantly influenced by the hydrological phases as well as the identification of similar responses among phases.
We performed LMM with the “lme” function from the “lme4” package (Bates et al., 2015). We built a bar graph using the “lineplot.CI” function from the “sciplot” package (Morales and Development Core Team, 2020). The PERMANOVA and PCA for environmental variables were performed using the “vegan,” “ade4,” “stats” and “factoextra” packages. All data analyses were conducted in R software (R Core Team, 2021).
Results
Environmental variables
We detected significant differences in the physical-chemical and environmental parameters between hydrological phases (F = 5.44, p < 0.001). The first two principal components (PC) of the PCA accounted for 27.94% of total variation in environmental variables over the three hydrological phases. The overall environmental change was from water predominantly saline and with high conductivity in dry and re-wetting phases to tree branches-dominated with high substrate diversity and volume in wet phase (Table 1; Figure 2; Supplementary Table S3).
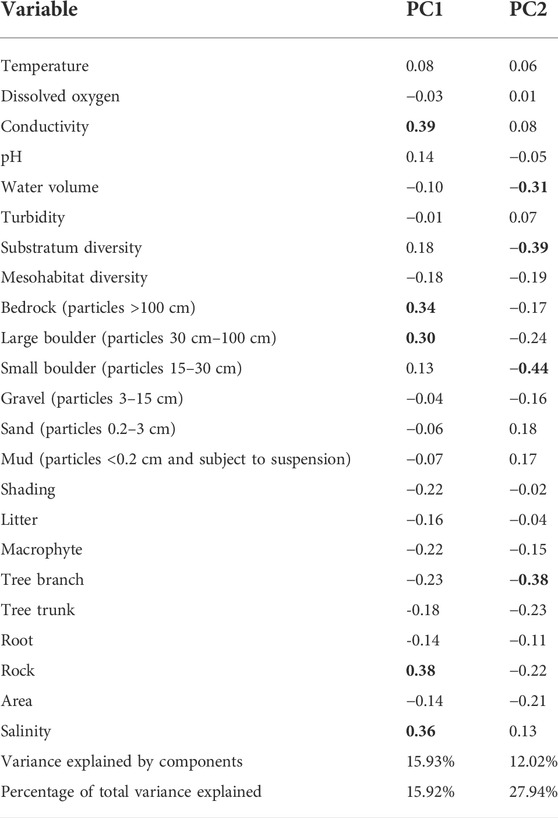
TABLE 1. Principal component analysis (PCA) loadings of physical-chemical and environmental variables. Values in bold indicate the most important variables (loadings > 0.3) to ordinate the hydrological phases.
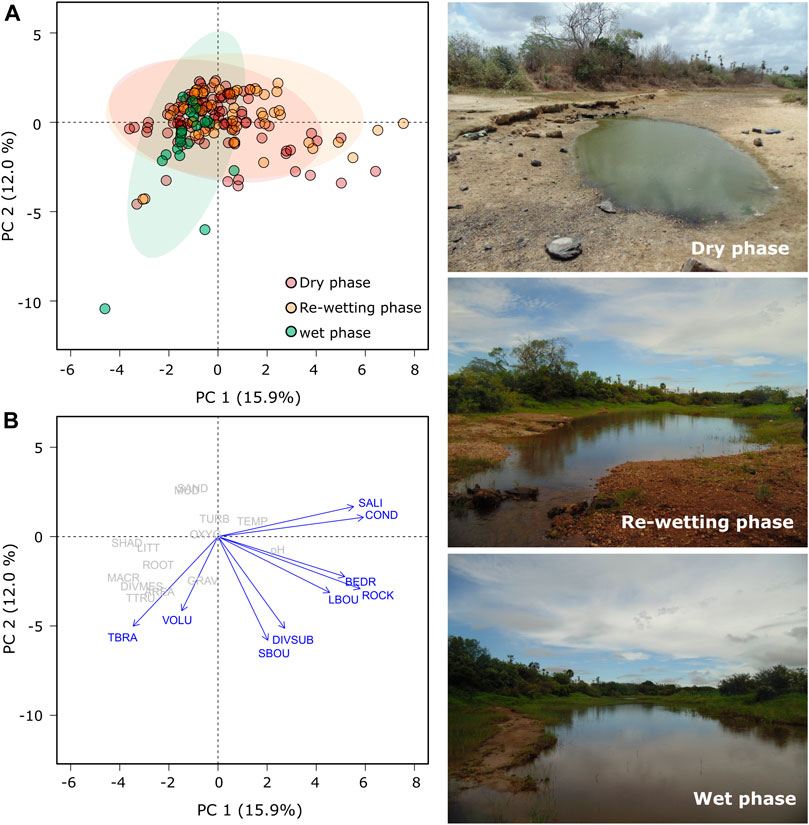
FIGURE 2. Principal component analysis of physical-chemical and environmental variables of each hydrological phase between 2016 and 2018 in Cruxati River, Ceará, Brazil. Photos captured between 2016 and 2017 at Site B (Teixeira, F.K.).
Functional structure of fish assemblages
A total of 43,003 individuals were collected belonging to 15 species (Table 2). Poecilia vivipara was the most abundant species (N = 18,436), followed by Serrapinnus heterodon (N = 14,249), representing 76% of total sampled species. These two species represented 74% of abundance in dry phase, 83% in re-wetting and 54% in wet. The less abundant species were Parotocinclus cearensis (N = 2) captured only in the wet phase and Characidum bimaculatum (N = 7) only in the dry phase. Considering all sampled species, fish abundance was higher during the dry phase (N = 24,671) followed by re-wetting (N = 15,376) and wet (N = 2,956) phases (Table 2). Species richness (S) was higher during dry (S = 14) and wet (S = 14) phases when compared to re-wetting (S = 13).
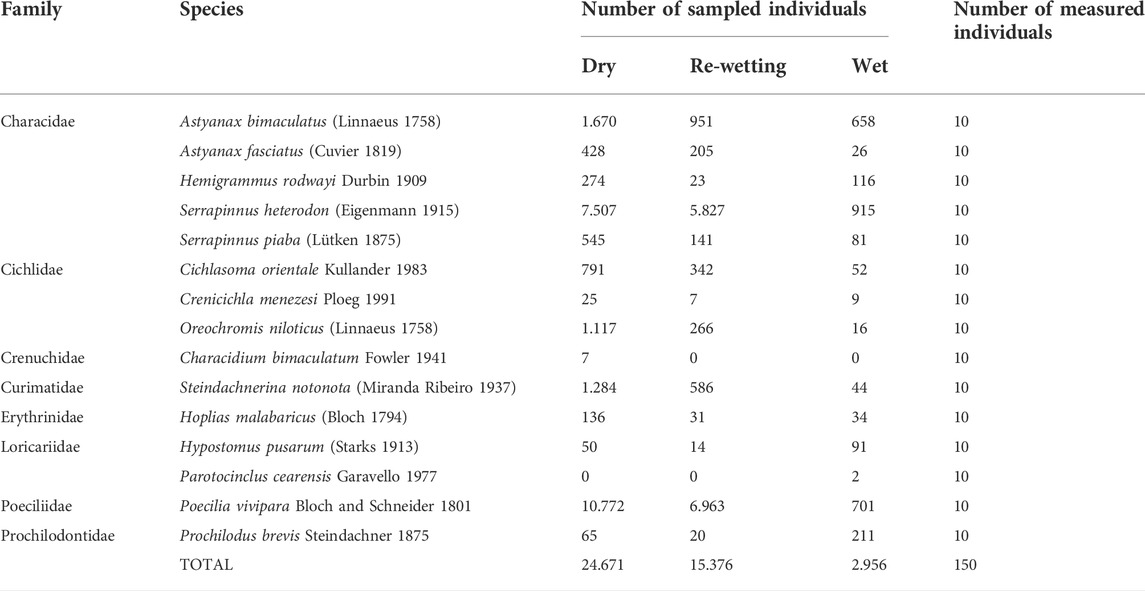
TABLE 2. List of species sampled on each hydrological phase in the Cruxati intermittent river, Ceará - Brazil, with respective abundance (number of sampled individuals) and number of individuals used for functional analysis.
The regional functional space did not differ between the hydrological phases, unlike the local taxonomic and functional structure (Figures 3A,C). In addition, the regional differences among species within hydrological phases are predominantly explained by morphological traits (Figure 3B). Higher mean values of oral gape position (Og_p = 1.06) and eye position (Ep = 1,04) were registered for P. vivipara while higher mean values of oral gape shape (Og_Sh = 1.75) for S. heterodon (Supplementary Table S4). These two species were also the most abundant in the three hydrological phases (Figure 3A; Table 2). Regarding functional traits reflecting swimming efficiency, the higher values of caudal peduncle throttling and fins surface to body size ratio were registered for Prochilodus brevis (Cp_Th = 2.62 and Fb_Ra = 207,884.59, Supplementary Table S2). This species presented substantial differences in abundance between hydrological phases (Figure 3A; Table 2) with higher numbers of individuals during the wet season (N = 211) revealing a high caudal propulsion efficiency in periods where the river is longitudinal connected.
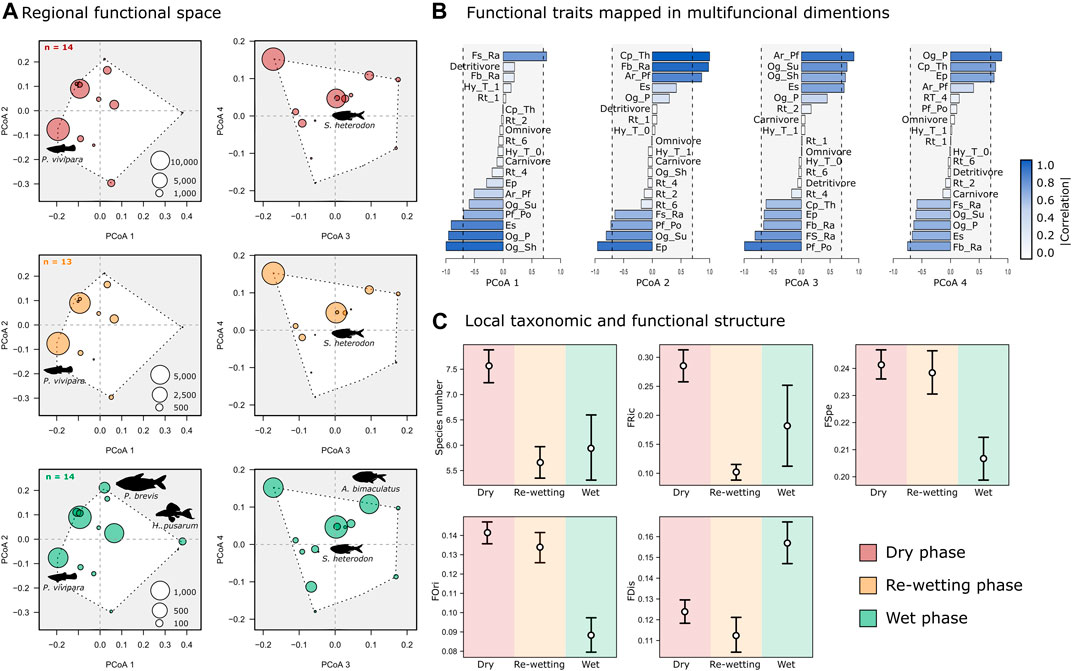
FIGURE 3. (A) Principal Coordinate Analysis (PCoA) of fish assemblages from each hydrological phase, representing the regional multidimensional functional space and species abundances (diameter of circles); (B) functional traits mapped in four multifuncional dimensions, the codes for each trait are described in Supplementary Table S1; and (C) differences on local taxonomic and functional structure according species number and functional diversity indices between the three hydrological intermittent phases (white circles indicate the mean of each index and black lines indicate values of standard deviation), using linear mixed models (LMM).
In opposite to the regional scale, we registered significant local differences on species richness (F = 11.1; p < 0.05), functional richness (FRic; F = 9.7; p < 0.05), functional specialization (FSpe; F = 3.7; p < 0.05) and functional originality (FOri; F = 8.0; p < 0.05) between hydrological phases of the intermittent river (Figure 3C; Table 3). Species richness and FRic values were higher in the dry phase while lower values were revealed during the re-wetting phase (Figure 3C). Additionally, the higher functional variation was detected during the wet phase for both indices (Table 3). In relation to the FSpe and the FOri indices, the higher values were detected during the dry and re-wetting phases (Figure 3C), while the FDis was higher in the wet phase (F = 4.6; p < 0.05; Figure 3C). The FDiv and FEve indices did not differ (F = 0.06; p = 0.94 and F = 0.6; p = 0.55, respectively) between the three phases of the hydrological cycle (Supplementary Figure S1).
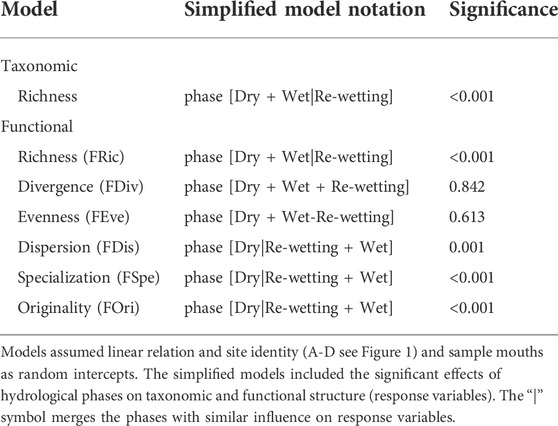
TABLE 3. Linear mixed models (LMM) on the taxonomic and functional structure of fish assemblages in the Cruxati intermittent river, Ceará - Brazil.
Discussion
We observed marked temporal variations in environmental conditions and biotic structure (taxonomic and functional) on the three hydrological phases. The intermittent dynamic modified the water’s physical and chemical characteristics as well as habitat diversity, influencing local communities which can be associated with drought characteristics such as warmer water, high salinity, and conductivity (Crook et al., 2010) (e.g., dry and re-wetting phases) that are harsh characteristics for aquatic species survival (Lennox et al., 2019). Despite the drought characteristics, we found high taxonomic richness and functional richness (FRic), specialization (FSpe) and originality (FOri) in dry and re-wetting phases, suggesting that Cruxati fish community display distinct functional characteristics capable of overcoming the local filters when flow ceases. Indeed, fish species of semiarid rivers showed high individual variability with high trophic specialization (Manna et al., 2019). Interestingly, we did not observe differences in the regional functional structure between hydrological phases (Figure 3A), which in turn may promote similar local functional structure patterns (Spasojevic et al., 2018). The regional-local decoupling suggests that the hydrological phases favor species with different trait combinations, acting as a strong environmental filter selecting some species over others regardless of the regional diversity in the Cruxati fish community.
Environmental variables and hydrological phases
The local environmental conditions of the IRES Cruxati River change over hydrological phases. In IRES, habitats expand and contract, changing the spatial arrangement, therefore hydrological connectivity shifts in response to surface discharge (Datry et al., 2016; Datry et al., 2017). In, wet phase was associated with tree branch and water volume confirming the conditions of higher water column observed in situations that intermittent rivers are longitudinal connected. In this hydrological phase, longitudinal connection among river-bed pools is an important factor in dispersal and maintenance of diversity in these systems (Maltchik and Medeiros, 2001). However, during dry phase, flow disrupts hydrological connectivity along longitudinal, lateral, and vertical dimensions, creating a highly dynamic mosaic of habitat patches (Boulton et al., 2017), forming isolated pools (dry and re-wetting) which will have higher conductivity and salinity as the pools dry (Bond et al., 2008). The flow absence in dry and re-wetting phases result in low rates of dissolved oxygen due to high utilization of dissolved oxygen for respiratory purposes of organisms. In this sense, the variability in environmental conditions during the hydrological phases acts as a habitat filter that has a marked influence on biological diversity (Maltchick and Medeiros 2006; Chase, 2007).
Functional responses to intermittent dynamics
On a regional scale, we did not find differences on functional structure and taxonomic diversity across hydrological phases (e.g., differences on water levels). Temporal variation on taxonomic diversity is determined by a balance between temporal environmental variability (seasonality) and the reliability of this variability (predictability) (Tonkin et al., 2017). Therefore, taxonomic composition and fish abundance in intermittent rivers may remain relatively stable and persistent across time even with extreme flooding or drying (Matthews et al., 2013). In addition to stability in fish composition, there is usually a low number of species in intermittent rivers (e.g., Africa and Australia) (Kerezsy et al., 2017). In this sense, over evolutionary time, the remain pool of species selected by environment filters have traits capable of increasing their survival (e.g., success on resources’ exploitation) and even traits that depend on these disturbances (e.g., water level acting as one driver of reproductive tactics) (Lytle and Poff, 2004).
Poecilia vivipara is the most abundant species in all hydrological phases due to several traits related to resistance that increasing the possibility of surviving in water with low oxygen concentration, and high variation in salinity and temperature (e.g., oral gape position, eye position, omnivory, salinity tolerance, and viviparous strategic) (Kramer & Mehegan 1981; Louvise and Monteiro, 2007; Silva et al., 2014; Rius et al., 2019). These traits associated with variability in reproductive strategies allow an easier establishment, producing large numbers of individuals (Louvise and Monteiro, 2007; Arcanjo et al., 2014; Rius et al., 2019). The combination of adaptable colonization and recruitment strategies allow most fish species to survive in intermittent rivers (Datry et al., 2017; Kerezsy et al., 2017). The second most abundant species was the omnivorous and shoal-forming Serrapinnus heterodon, also commonly found in semi-arid regions (Silva et al., 2014), including the Mundaú Basin (Teixeira et al., 2017; Terra et al., 2017). This species has a higher oral gape shape trait value, reflecting efficiency in capturing food items during all intermittent phases, as well as P. vivipara (Manna et al., 2019). The third most abundant species was Astyanax bimaculatus, a generalist and opportunistic species that has a wide capacity of changing the diet according to variations in the habitat and seasons in response to food resources availability (Silva et al., 2010; Manna et al., 2012). It is important to emphasize that during the dry phase we found higher abundance of individuals in the remain isolated pools; as other studies in IRES (e.g., Datry et al., 2016; Bogan et al., 2017). Increasing abundance of aquatic organisms can be associated with hard environmental conditions as decreased habitat area (e.g., Dewson et al., 2007; Datry et al., 2016), resilience traits and reproductive peak. The higher abundance of species in the dry phase is related to opportunistic strategists related to life history traits selected by flows’ variability (Winemiller and Rose, 1992; Mims and Olden, 2012). Perennial rivers are becoming intermittent due to recent drying events which cause higher local effects on taxonomic diversity of aquatic communities in relation to intermittent rivers that historically experience drying events (Crabot et al., 2020). The combination of resistance and resilience traits can explain this pattern, since communities are filtered by environmental conditions as flow that select the functional composition (Bonada et al., 2007; Chase, 2007; Bogan et al., 2017).
We found significant differences in the functional diversity indices (FRic, FSpe, FOri, and FDis) among hydrological phases, reflecting no stability in functional composition. As expected, assemblages with higher species richness were the ones with higher values of FRic, since functional richness varies in relation to species composition (Mouillot et al., 2013). Therefore, local effects were important on structuring fish assemblages during the dry phase. This can be explained by the high environmental heterogeneity in the formation of isolated pools (Banegas-Medina et al., 2021) and by unique traits adapted for drought events (Lytle and Poff, 2004).
Local processes as driver of functional diversity
We observed higher values of FSpe and FOri for each isolated pool during the dry phase when compared to re-wetting and wet phases. Hence, isolated assemblages can be composed by functionally specialist species with extreme trait combinations and high abundance (Villéger et al., 2010), and/or original species that do not share traits with functionally closely-related species (Mouillot et al., 2013). Additionally, the durability of the dry phase influences the degree of temporal effects in biotic communities, such as taxa with tolerance to drought (i.e., specialists) replacing species adapted to flowing conditions (Cid et al., 2017).
FSpe and FOri decreased during the wet season probably due to high water flow and hydrological conditions (e.g., Sánchez-Pérez et al., 2020), as well as FDis increased after the river reconnected. Communities are more functionally dispersed in this phase with species abundance more equitable and distant from the centroid considering the functional space. Therefore, when the river is connected, communities are regularly distributed in the functional space. Due to sensitivity to species abundance, FDis was lower in dry and re-wetting phases, where isolated pools have higher abundance with more species closer to the community centroid (Laliberté and Legendre, 2010). Lower values of FDis are already expected for environments that suffer hard disturbances (Mouillot et al., 2013). This sensitivity of the FDis to disturbances can be explained by the fact that changes in species abundance are faster than changes in species richness (e.g., local extinction) (Mouillot et al., 2013). This regularity in the higher functional dispersion of fish assemblages in the wet phase corroborates our hypothesis.
Water flow variations can lead to changes in species abundance throughout the hydrological phases (Medeiros and Maltchik, 2001). For example, the increase in abundance of Hypostomus pusarum during the wet phase can be reflected by high resilience reported to Loricariidae species (Power, 2003), indicating potential resistance to extreme drought events followed by recolonization when the water level rises. Prochilodus brevis was more abundant in wet phase, which is explained by its migratory habits related to dispersion traits as caudal peduncle throttling and fins surface to body size ratio, which allow caudal propulsion efficiency through reduction of drag and acceleration and/or maneuverability efficiency (Villéger et al., 2010). In the semiarid region, the rainfall acts as a trigger to the reproductive cycle of this species (Gurgel et al., 2012) causing spawning migration during the rainy season (Nelson, 1994). However, small stream-dwelling fish have longitudinal movements limited to two kilometers, characterizing most species with short distance displacement (e.g., Mazzoni et al., 2018), which can explain the low abundance of most species during re-wetting and wet phases. Traits related to Eurytopic guild combined with limited displacement, which includes species with high tolerance to low dissolved oxygen concentrations, may explain high abundance of some species during the dry phase (e.g., P. vivipara) (Welcomme et al., 2006).
However, global climatic changes have been modifying the rainfall regime in semiarid regions, altering the reproductive process of fish species. These climatic alterations favor the expansion of drying over time and space in naturally intermittent river regimes with prolonged dry phase (Larned et al., 2010; Datry et al., 2021). Temporal nestedness increased in naturally intermittent rivers as climatic aridity increased, showing that harsh environmental conditions associated with global changes may further reduce functional biodiversity at these sites even if they are already intermittent (Vorste et al., 2021). In addition to the increased water demands for human use (Larned et al., 2010), naturally intermittent systems may serve as models of future predictions in cases of water scarcity. This highlights the need to consider climatic alterations in studies investigating the ecological responses to stress and disturbance in dynamic river systems (Vorste et al., 2021).
We conclude that local ecological processes (i.e., dynamics of hydrology) may promote the establishment of species according to their functional traits and thereby the functional structure of local assemblages. Fish species also change their distributions at different spatial scales in response to seasonal changes in flow and habitat availability (Cid et al., 2017). Interestingly, local effects may influence the responses of functional fish traits (more than regional diversity) when temporal dynamics within the same region were investigated, contradicting the inferences obtained at regional, or global level (Stefani et al., 2020). Therefore, in a region with low species richness that does not substantially change over a long time, local processes are important to explain the functional structure of fish assemblages.
Data availability statement
The original contributions presented in the study are included in the article/Supplementary Material, further inquiries can be directed to the corresponding author.
Ethics statement
This study is in strict accordance with recommendations of the Ethical committee of Federal University of Ceará (UFC) (CEUA 4629200318 and CEUA 7344060218). All the sampling complied with current Brazilian laws, and IBAMA SISBIO through special license 61,276-1 issued to CFR.
Author contributions
MG-S, FT and CR conceived the ideas and designed methodology; FT collected the data; MG-S, LM, and CF analyzed the data; MG-S, LM and CR led the writing of the manuscript. All authors contributed critically to the discussion on the results and gave final approval for submission. CF also led the writing of the manuscript.
Funding
This study was financed in part by the Coordenação de Aperfeiçoamento de Pessoal de Nível Superior—Brasil (CAPES)—Finance Code 001 (MG-S and FT), and funded by CNPq universal (420617/2016-7), Programa de Apoio a Núcleos Emergentes PRONEM/FUNCAP/CNPq (PNE-0112-00026.01.00/16), FUNBIO (02/2019) and Teaching Support Scholarship/PAPD-UERJ/2019.
Acknowledgments
The authors are thankful to Maria Rita N. Duarte for the elaboration of the map (Figure 1). All the members involved in this study are to be acknowledged here.
Conflict of interest
The authors declare that the research was conducted in the absence of any commercial or financial relationships that could be construed as a potential conflict of interest.
Publisher’s note
All claims expressed in this article are solely those of the authors and do not necessarily represent those of their affiliated organizations, or those of the publisher, the editors and the reviewers. Any product that may be evaluated in this article, or claim that may be made by its manufacturer, is not guaranteed or endorsed by the publisher.
Supplementary material
The Supplementary Material for this article can be found online at: https://www.frontiersin.org/articles/10.3389/fenvs.2022.903974/full#supplementary-material
References
Arcanjo, R. B., de Souza, L. P., Rezende, C. F., and Silva, J. R. (2014). Embryonic development and nourishment in the viviparous fish P oecilia vivipara (cyprinodontiformes: P oeciliidae). Acta Zool. 95 (4), 493–500. doi:10.1111/azo.12046
Banegas-Medina, A., Montes, I. Y., Tzoraki, O., Brendonck, L., Pinceel, T., Diaz, G., et al. (2021). Hydrological, environmental and taxonomical heterogeneity during the transition from drying to flowing conditions in a Mediterranean intermittent river. Biology 10 (4), 316. doi:10.3390/biology10040316
Bates, D., Maechler, M., Bolker, B., and Walker, S. (2015). Fitting linear mixed-effects models using lme4. J. Stat. Softw. 67, 1–48. doi:10.18637/jss.v067.i01
Bogan, M. T., Chester, E. T., Datry, T., Murphy, A. L., Robson, B. J., Ruhi, A., et al. (2017). “Resistance, resilience, and community recovery in IRs and ephemeral streams,” in Intermittent rivers and ephemeral streams: Ecology and management. Editors T. Datry, N. Bonada, and A. Boulton (London, United Kingdom: Elsevier Academic Press). doi:10.1016/C2015-0-00459-2
Bonada, N., Cañedo-Argüelles, M., Gallart, F., von Schiller, D., Fortuño, P., Latron, J., et al. (2020). Conservation and management of isolated pools in temporary rivers. Water 12 (10), 2870. doi:10.3390/w12102870
Bonada, N., Rieradevall, M., and Prat, N. (2007). Macroinvertebrate community structure and biological traits related to flow permanence in a Mediterranean river network. Hydrobiologia 589 (1), 91–106. doi:10.1007/s10750-007-0723-5
Bond, N. R., Lake, P. S., and Arthington, A. H. (2008). The impacts of drought on freshwater ecosystems: An Australian perspective. Hydrobiologia 600 (1), 3–16. doi:10.1007/s10750-008-9326-z
Borba, G. C., Costa, F. R., Espírito-Santo, H. M., Leitão, R. P., Dias, M. S., and Zuanon, J. (2020). Temporal changes in rainfall affect taxonomic and functional composition of stream fish assemblages in central Amazonia. Freshw. Biol. 66 (4), 753–764. doi:10.1111/fwb.13675
Boulton, A. J. (2003). Parallels and contrasts in the effects of drought on stream macroinvertebrate assemblages. Freshw. Biol. 48 (7), 1173–1185. doi:10.1046/j.1365-2427.2003.01084.x
Boulton, A. J., Rolls, R. J., Jaeger, K. L., and Datry, T. (2017). “Hydrological connectivity in intermittent rivers and ephemeral streams,” in Intermittent rivers and ephemeral streams: Ecology and management. Editors T. Datry, N. Bonada, and A. Boulton (London, United Kingdom: Elsevier Academic Press). doi:10.1016/C2015-0-00459-2
Chase, J. M. (2007). Drought mediates the importance of stochastic community assembly. Proc. Natl. Acad. Sci. U. S. A. 104 (44), 17430–17434. doi:10.1073/pnas.0704350104
Cid, N., Bonada, N., Carlson, S. M., Grantham, T. E., Gasith, A., and Resh, V. H. (2017). High variability is a defining component of Mediterranean-climate rivers and their biota. Water 9 (1), 52. doi:10.3390/w9010052
Comte, L., and Olden, D. J. (2018). Evidence for dispersal syndromes in freshwater fishes. Proc. R. Soc. B 285, 20172214. doi:10.1098/rspb.2017.2214
Crabot, J., Polášek, M., Launay, B., Pařil, P., and Datry, T. (2020). Drying in newly intermittent rivers leads to higher variability of invertebrate communities. Freshw. Biol. 66 (4), 730–744. doi:10.1111/fwb.13673
Crook, D. A., Reich, P., Bond, N. R., McMaster, D., Koehn, J. D., and Lake, P. S. (2010). Using biological information to support proactive strategies for managing freshwater fish during drought. Mar. Freshw. Res. 61 (3), 379–387. doi:10.1071/MF09209
Datry, T., Allen, D., Argelich, R., Barquin, J., Bonada, N., Boulton, A., et al. (2021). Securing biodiversity, functional integrity, and ecosystem services in drying river networks (DRYvER). Res. Ideas Outcomes 7, e77750. doi:10.3897/rio.7.e77750
Datry, T., Bonada, N., and Boulton, A. (2017). Intermittent rivers and ephemeral streams: Ecology and management. London, United Kingdom: Elsevier Academic Press. doi:10.1016/C2015-0-00459-2
Datry, T., Bonada, N., and Heino, J. (2016). Towards understanding the organisation of metacommunities in highly dynamic ecological systems. Oikos 125 (2), 149–159. doi:10.1111/oik.02922
Dewson, Z. S., James, A. B., and Death, R. G. (2007). A review of the consequences of decreased flow for instream habitat and macroinvertebrates. J. North Am. Benthol. Soc. 26 (3), 401–415. doi:10.1899/06-110.1
Dézerald, O., Céréghino, R., Corbara, B., Dejean, A., and Leroy, C. (2015). Functional trait responses of aquatic macroinvertebrates to simulated drought in a Neotropical bromeliad ecosystem. Freshw. Biol. 60 (9), 1917–1929. doi:10.1111/fwb.12621
Duarte, M. R. N., Pereira, T. M., Lima, P. D. F., Pereira, E. C. B., Lopes, F. B., and Rezende, C. F. (2021). Dinâmica limnológica em reservatório artificial e rio intermitente na região semiárida em função do uso e ocupação do solo. Rev. Ciência Agronômica 52 (1). doi:10.5935/1806–6690.20210010
Espírito–Santo, H. M., Rodriguez, M. A., and Zuanon, J. (2013). Reproductive strategies of Amazonian stream fishes and their fine–scale use of habitat are ordered along a hydrological gradient. Freshw. Biol. 58 (12), 2494–2504. doi:10.1111/fwb.12225
Gurgel, L. D. L., Verani, J. R., and Chellappa, S. (2012). Reproductive ecology of Prochilodus brevis an endemic fish from the semiarid region of Brazil. Sci. World J. 2012, 1–7. doi:10.1100/2012/810532
F. R. Hauer, and G. A. Lamberti (Editors) (2011). Methods in stream ecology (Cambridge, MA, USA: Academic Press).
Holdridge, L. R. (1947). Determination of world plant formations from simple climatic data. Science 105 (2727), 367–368. doi:10.1126/science.105.2727.367
Hyslop, E. J. (1980). Stomach contents analysis—A review of methods and their application. J. Fish. Biol. 17 (4), 411–429. doi:10.1111/j.1095–8649.1980.tb02775.x
Jarzyna, M. A., and Jetz, W. (2016). Detecting the multiple facets of biodiversity. Trends Ecol. Evol. 31, 527–538. doi:10.1016/j.tree.2016.04.002
Junk, W. J., Bayley, P. B., and Sparks, R. E. (1989). The flood pulse concept in river-floodplain systems. Can. special Publ. Fish. aquatic Sci. 106 (1), 110–127.
Kerezsy, A., Gido, K., Magalhães, M. F., and Skelton, P. H. (2017). “The biota of intermittent rivers and ephemeral streams: Fishes,” in Intermittent rivers and ephemeral streams: Ecology and management. Editors T. Datry, N. Bonada, and A. Boulton (London, United Kingdom: Elsevier Academic Press). doi:10.1016/C2015-0-00459-2
Kramer, D. L., and Mehegan, J. P. (1981). Aquatic surface respiration, an adaptive response to hypoxia in the guppy, Poecilia reticulata (Pisces, Poeciliidae). Environ. Biol. Fishes 6 (3), 299–313. doi:10.1007/BF00005759
Laliberté, E., and Legendre, P. (2010). A distance-based framework for measuring functional diversity from multiple traits. Ecology 91 (1), 299–305. doi:10.1890/08-2244.1
Larned, S. T., Datry, T., Arscott, D. B., and Tockner, K. (2010). Emerging concepts in temporary‐river ecology. Freshw. Biol. 55 (4), 717–738. doi:10.1111/j.1365-2427.2009.02322.x
Lennox, R. J., Crook, D. A., Moyle, P. B., Struthers, D. P., and Cooke, S. J. (2019). Toward a better understanding of freshwater fish responses to an increasingly drought-stricken world. Rev. Fish. Biol. Fish. 29 (1), 71–92. doi:10.1007/s11160-018-09545-9
Louvise, J., and Monteiro, L. R. (2007). Size and fecundity variation in populations of Poecilia vivipara Block & Schneider (Teleostei; Poeciliidae) inhabiting an environmental gradient. J. Fish. Biol. 71, 1799–1809. doi:10.1111/j.1095-8649.2007.01653.x
Lytle, D. A., and Poff, N. L. (2004). Adaptation to natural flow regimes. Trends Ecol. Evol. 19 (2), 94–100. doi:10.1016/j.tree.2003.10.002
Maire, E., Grenouillet, G., Brosse, S., and Villéger, S. (2015). How many dimensions are needed to accurately assess functional diversity? A pragmatic approach for assessing the quality of functional spaces. Glob. Ecol. Biogeogr. 24 (6), 728–740. doi:10.1111/geb.12299
Maltchik, L., and Medeiros, E. S. F. (2006). Conservation importance of semi-arid streams in north-eastern Brazil: Implications of hydrological disturbance and species diversity. Aquat. Conserv. 16 (7), 665–677. doi:10.1002/aqc.805
Maltchik, L., and Medeiros, E. S. F. (2001). Does hydrological stability influence biodiversity and community stability? A theoretical model for lotic ecosystems from the Brazilian semiarid region. Ciência Cult. 53 (1), 44–48.
Manna, L. R., Rezende, C. F., and Mazzoni, R. (2017). Effect of body size on microhabitat preferences in stream-dwelling fishes. J. Appl. Ichthyol. 33 (2), 193–202. doi:10.1111/jai.13320
Manna, L. R., Rezende, C. F., and Mazzoni, R. (2012). Plasticity in the diet of Astyanax taeniatus in a coastal stream from south-east Brazil. Braz. J. Biol. 72 (4), 919–928. doi:10.1590/s1519-69842012000500020
Manna, L. R., Villéger, S., Rezende, C. F., and Mazzoni, R. (2019). High intraspecific variability in morphology and diet in tropical stream fish communities. Ecol. Freshw. Fish. 28 (1), 41–52. doi:10.1111/eff.12425
Matthews, W. J., Marsh-Matthews, E., Cashner, R. C., and Gelwick, F. (2013). Disturbance and trajectory of change in a stream fish community over four decades. Oecologia 173 (3), 955–969. doi:10.1007/s00442-013-2646-3
Mazzoni, R., Pinto, M. P., Iglesias-Rios, R., and Costa, R. (2018). Fish movement in an atlantic forest stream. Neotrop. Ichthyol. 16 (1), e170065. doi:10.1590/1982-0224-20170065
Medeiros, E. S., and Maltchik, L. (2001). Fish assemblage stability in an intermittently flowing stream from the Brazilian semiarid region. Austral Ecol. 26 (2), 156–164. doi:10.1046/j.1442-9993.2001.01099.x
Mims, M. C., and Olden, J. D. (2012). Life history theory predicts fish assemblage response to hydrologic regimes. Ecology 93 (1), 35–45. doi:10.1890/11-0370.1
Morales, M., and Development Core Team, R. (2020). Sciplot: Scientific graphing functions for factorial designs. R Package version 1.2-0. Available at: https://CRAN.R-project.org/package=sciplot.
Mouillot, D., Graham, N. A., Villéger, S., Mason, N. W., and Bellwood, D. R. (2013). A functional approach reveals community responses to disturbances. Trends Ecol. Evol. 28 (3), 167–177. doi:10.1016/j.tree.2012.10.004
Mouillot, D., Villéger, S., Scherer-Lorenzen, M., and Mason, N. W. H. (2011). Functional structure of biological communities predicts ecosystem multifunctionality. PLoS ONE 6 (3), e17476. doi:10.1371/journal.pone.0017476
Pease, A. A., González-Díaz, A. A., Rodiles-Hernández, R., and Winemiller, K. O. (2012). Functional diversity and trait–environment relationships of stream fish assemblages in a large tropical catchment. Freshw. Biol. 57 (5), 1060–1075. doi:10.1111/j.1365-2427.2012.02768.x
Pereira, H. M., Leadley, P. W., Proença, V., Alkemade, R., Scharlemann, J. P. W., Fernandez-Manjarrés, J. F., et al. (2010). Scenarios for global biodiversity in the 21st century. Science 330, 1496–1501. doi:10.1126/science.1196624
Power, M. E. (2003). Life cycles, limiting factors, and behavioral ecology of four loricariid catfishes in a Panamanian stream. Catfishes 2, 581–600.
R Core Team (2021). R: A language and environment for statistical computing. Vienna, Austria: R Foundation for Statistical Computing. Availabel at: https://www.R-project.org/.
Rius, B. F., Petry, A. C., Langerhans, R. B., Figueiredo-Barros, M. P., Bozelli, R. L., Honda, L. K., et al. (2019). Correlates of life-history variation in the livebearing fish Poecilia vivipara (Cyprinodontiformes: Poeciliidae) inhabiting an environmental gradient. Biol. J. Linn. Soc. Lond. 126 (3), 436–446. doi:10.1093/biolinnean/bly208
Rodrigues-Filho, C. A., Gurgel-Lourenço, R. C., Ramos, E. A., Novaes, J. L., Garcez, D. S., Costa, R. S., et al. (2020). Metacommunity organization in an intermittent river in Brazil: The importance of riverine networks for regional biodiversity. Aquat. Ecol. 54 (1), 145–161. doi:10.1007/s10452-019-09732-1
Sánchez-Pérez, A., Oliva-Paterna, F. J., Colin, N., Torralva, M., and Górski, K. (2020). Functional response of fish assemblage to multiple stressors in a highly regulated Mediterranean river system. Sci. Total Environ. 730, 138989. doi:10.1016/j.scitotenv.2020.138989
Schmera, D., Heino, J., Podani, J., Erős, T., and Dolédec, S. (2017). Functional diversity: A review of methodology and current knowledge in freshwater macroinvertebrate research. Hydrobiologia 787 (1), 27–44. doi:10.1007/s10750-016-2974-5
Silva, M. J. D., Figueiredo, B. R., Ramos, R. T., and Medeiros, E. S. (2010). Food resources used by three species of fish in the semi-arid region of Brazil. Neotrop. Ichthyol. 8 (4), 819–825. doi:10.1590/s1679-62252010005000010
Silva, M. J. D., Ramos, T. P. A., Diniz, V. D., Ramos, R. T. D. C., and Medeiros, E. S. F. (2014). Ichthyofauna of seridó/borborema: A semi-arid region of Brazil. Biota Neotrop. 14. doi:10.1590/1676-06032014007713
Spasojevic, M. J., Catano, C. P., LaManna, J. A., and Myers, J. A. (2018). Integrating species traits into species pools. Ecology 99 (6), 1265–1276. doi:10.1002/ecy.2220
Stefani, F., Schiavon, A., Tirozzi, P., Gomarasca, S., and Marziali, L. (2020). Functional response of fish communities in a multistressed freshwater world. Sci. Total Environ. 740, 139902. doi:10.1016/j.scitotenv.2020.139902
Taylor, C. M., and Lienesch, P. W. (1995). Environmental correlates of distribution and abundance forLythrurus snelsoni: A range-wide analysis of an endemic fish species. Southwest. Nat. 40, 373–378.
Teixeira, F. K., Ramos, T. P. A., Paiva, R. E. C. D., Távora, M. A., Lima, S. M. Q., and Rezende, C. F. (2017). Ichthyofauna of Mundaú river basin, Ceará state, northeastern Brazil. Biota Neotrop. 17, 1–9. doi:10.1590/1676-0611-BN-2016-0174
Teresa, F. B., Rodrigues-Filho, C. A. S., and Leitão, R. P. (2021). Diversidade funcional de comunidades de peixes de riacho. Oecol. Aust. 25 (2), 415–432. doi:10.4257/oeco.2021.2502.12
Terra, B. F., Teixeira, F. K., and Rezende, C. F. (2017). Length–weight relationships of 10 freshwater fish species from an intermittent river basin, semi-arid region, Brazil. J. Appl. Ichthyol. 33 (4), 832–834. doi:10.1111/jai.13357
Tonkin, J. D., Bogan, M. T., Bonada, N., Rios‐Touma, B., and Lytle, D. A. (2017). Seasonality and predictability shape temporal species diversity. Ecology 98 (5), 1201–1216. doi:10.1002/ecy.1761
Vidal, L. V. O., Albinati, R. C. B., Albinati, A. C. L., Lira, A. D. D., Almeida, T. R. D., and Santos, G. B. (2008). Eugenol como anestésico para a tilápia-do-nilo. Pesq. Agropec. Bras. 43, 1069–1074. doi:10.1590/S0100-204X2008000800017
Villéger, S., Mason, N. W., and Mouillot, D. (2008). New multidimensional functional diversity indices for a multifaceted framework in functional ecology. Ecology 89 (8), 2290–2301. doi:10.1890/07-1206.1
Villéger, S., Miranda, J. R., Hernández, D. F., and Mouillot, D. (2010). Contrasting changes in taxonomic vs. functional diversity of tropical fish communities after habitat degradation. Ecol. Appl. 20 (6), 1512–1522. doi:10.1890/09-1310.1
Violle, C., Reich, P. B., Pacala, S. W., Enquist, B. J., and Kattge, J. (2014). The emergence and promise of functional biogeography. Proc. Natl. Acad. Sci. U. S. A. 111 (38), 13690–13696. doi:10.1073/pnas.1415442111
Vorste, R. V., Stubbington, R., Acuña, V., Bogan, M. T., Bonada, N., Cid, N., et al. (2021). Climatic aridity increases temporal nestedness of invertebrate communities in naturally drying rivers. Ecography 44 (6), 860–869. doi:10.1111/ecog.05349
Welcomme, R. L., Winemiller, K. O., and Cowx, I. G. (2006). Fish environmental guilds as a tool for assessment of ecological condition of rivers. River Res. Appl. 22 (3), 377–396. doi:10.1002/rra.914
Winemiller, K. O. (1991). Ecomorphological diversification in lowland freshwater fish assemblages from five biotic regions. Ecol. Monogr. 61 (4), 343–365. doi:10.2307/2937046
Winemiller, K. O., and Rose, K. A. (1992). Patterns of life-history diversification in north American fishes: Implications for population regulation. Can. J. Fish. Aquat. Sci. 49 (10), 2196–2218. doi:10.1139/f92-242
Keywords: functional diversity, climate changes, temporary streams, drying rivers networks, semiarid climate
Citation: Gonçalves-Silva M, Manna LR, Rodrigues-Filho CAS, Teixeira FK and Rezende CF (2022) Effect of drying dynamics on the functional structure of a fish assemblage from an intermittent river network. Front. Environ. Sci. 10:903974. doi: 10.3389/fenvs.2022.903974
Received: 24 March 2022; Accepted: 26 July 2022;
Published: 31 August 2022.
Edited by:
Simone Jaqueline Cardoso, Juiz de Fora Federal University, BrazilReviewed by:
Vandick Silva Batista, Federal University of Alagoas, BrazilFrancisco Martinez-Capel, Universitat Politècnica de València, Spain
Copyright © 2022 Gonçalves-Silva, Manna, Rodrigues-Filho, Teixeira and Rezende. This is an open-access article distributed under the terms of the Creative Commons Attribution License (CC BY). The use, distribution or reproduction in other forums is permitted, provided the original author(s) and the copyright owner(s) are credited and that the original publication in this journal is cited, in accordance with accepted academic practice. No use, distribution or reproduction is permitted which does not comply with these terms.
*Correspondence: Luisa Resende Manna, luisamanna@gmail.com